- 1Department of Neurology, First Hospital of Jilin University, Chang Chun, China
- 2Clinical Trial and Research Center for Stroke, Department of Neurology, First Hospital of Jilin University, Chang Chun, China
Background: Patients with depression tend to have various comorbid neurological symptoms, but the mechanisms remain unclear. The purpose of this study was to analyze the characteristics of dynamic cerebral autoregulation in depressed patients.
Methods: Patients (aged ≥ 18 years) who were diagnosed with depression [17-item Hamilton Depression Rating Scale (HAMD) > 17] or suspected of depression (HAMD > 7) were enrolled in this study. Medically healthy volunteers were recruited as controls. The subjects also received the 7-item HAMD. We simultaneously recorded noninvasive continuous arterial blood pressure and bilateral middle cerebral artery blood flow velocity from each subject. Cerebral autoregulation was assessed by analyzing the phase difference using transfer function analysis.
Results: This study enrolled 54 patients with suspected depression, 45 patients with depression, and 48 healthy volunteers. The mean phase difference values were significantly lower in the patients with depression (F = 9.071, P < 0.001). In the multiple regression analysis, depression was negatively correlated with the phase difference values.
Conclusions: Dynamic cerebral autoregulation was compromised in patients with depression and negatively correlated with the depression score. Improving dynamic cerebral autoregulation may be a potential therapeutic method for treating the neurological symptoms of depression.
Introduction
Depression is the most common psychiatric disorder, a leading cause of disability, and affects nearly 15% of the population (1, 2). Core features of this disorder include depressed mood, loss of interest or pleasure, irritability, change in appetite and sleep, and neurocognitive dysfunctions (3, 4). In addition to suicide ideation and behavior, patients with depression also tend to have comorbid medical illnesses, such as cancer, cardiovascular diseases, and diabetes (5, 6). Depression is associated with an increased risk of stroke morbidity and mortality. These combined conditions generally worsen patient outcomes (7–12).
Despite the prevalence of depression and its considerable burden on global health, knowledge about its pathogenesis remains rudimentary. Previous studies have revealed global and regional changes in the cerebral blood flow of patients with depression compared to healthy individuals (13–15). Cerebral blood flow abnormalities in depression differ in patients, with a varying age of onset (16), disparate responses to antidepressant treatment (17), and diverse family histories (18). Longitudinal research also shows the apparent elevation of regional cerebral perfusion in remissive depression compared to current depression (19). The mechanism of the unusual cerebral blood flow in depressed patients is complex and incompletely understood, and cerebral autoregulation may play a role.
Cerebral autoregulation is the innate ability to maintain appropriate brain perfusion during blood pressure changes. It can be dynamically assessed with transfer function analysis (TFA) between spontaneous fluctuations of arterial blood pressure (ABP) and cerebral blood flow velocity (CBFV) (20, 21). To date, cerebral autoregulation has not been well analyzed in patients with depression. In the present study, we hypothesize that dynamic cerebral autoregulation is compromised in patients with depression, and we use TFA to assess dynamic cerebral autoregulation in depressed patients and explore its relationship with the degree of depression.
Methods
Participants and Clinical Assessment
Patients whose first complaint was poor sleep and with 17-item Hamilton Depression Rating Scale (HAMD) scores > 7 were included from the Department of Neurology, First Hospital of Jinlin University, from September 2017 to June 2018. Two blinded clinical psychiatrists evaluated the patients’ mental health status. All patients had never been treated with antidepressants before. Patients with a history of cerebrovascular diseases (that is, cerebrovascular stenosis and stroke), frequent arrhythmias, anemia and unstable blood pressure, and hyperthyroidism were excluded from the study as controls. The patients with hypertension or diabetes took medications, and their blood pressure and blood glucose levels were well controlled. These patients were divided into two groups, those with depression (HAMD ≥ 17) and those suspected of depression (17 > HAMD ≥ 7). Physical health status was assessed using a questionnaire covering cardiovascular, nervous system, thyroid, and metabolic diseases, and information regarding age, smoking, and drinking habits. A total of 48 medically and psychiatrically healthy volunteers were recruited as controls. Liver and kidney function, blood glucose, blood lipid, blood pressure, electrocardiography, transcranial Doppler (EMS-9 PB, Delica, Shenzhen, China), and carotid ultrasound (IU22, Phillips, Andover, MA, USA) tests were used to exclude subjects who did not meet the study standards.
Cerebral Autoregulation Assessment Monitoring
Before the dynamic cerebral autoregulation examination, all of the patients were instructed to avoid caffeine, nicotine, alcohol, and all kinds of sleep medications for at least 24 h. The assessments were performed in a quiet, dedicated monitoring room with minimal external stimuli. The subjects were instructed to breathe spontaneously and assumed a supine position with a head elevation of 30° when baseline ABP (automatic blood pressure monitor, Omron 711) was measured. Signals were recorded after a 10-min rest. Beat-to-beat ABP was noninvasively recorded through servo-controlled finger plethysmography (Finometer Model 1, FMS, Netherlands), and continuous bilateral middle cerebral artery blood flow velocity was recorded with 2-MHz probes with an insonation depth of 45 to 60 mm attached to a customized head frame (MultiDop X2, DWL, Sipplingen, Germany). Stable end-tidal carbon dioxide (CO2) levels were confirmed through a capnograph with a face mask attached to the nasal cannula. Each participant’s blood pressure and blood flow velocity were recorded for 10 min. The data were then stored for further dynamic cerebral autoregulation examination analysis.
Analysis of Dynamic Cerebral Autoregulation
The recorded data were analyzed blindly using a laptop computer equipped with MATLAB (MathWorks, Natick, MA, USA). The beat-to-beat alignment of the data was acquired using a cross-correlation function to eliminate possible time lags. By using a cross-correlation function between ABP and CBFV, we may calculate the correlation at each time lag (by sample). We can then find the time lag with the maximum correlation, suggesting that the two signals are synchronized at this time lag. This is considered as the time delay between ABP and CBFV, which is likely caused by the data acquisition devices. We used a third-order Butterworth low-pass filter (cutoff at 0.5 Hz) as an anti-aliasing filter before downsampling the data to 1 Hz. A TFA was applied for evaluating cerebral autoregulation (22). TFA is a frequency domain analysis that calculates the “phase shift” between the CBFV and blood pressure waveforms in the 0.06–0.12 Hz frequency domain to evaluate cerebral autoregulation where the derived parameters were considered most relevant to autoregulation hemodynamics (23). In the current study, a phase shift was accepted for later statistical analysis only if the calculated coherence of one measurement was >0.49 within 0.06–0.12 Hz (24–26), in order to ensure that there was at least 49% linearity between ABP and CBFV. Otherwise, it is invalid to use TFA for the assessment, as it is a linear model.
Statistical Analysis
The statistical data were analyzed using Statistical Program for Social Sciences version 21.0 (SPSS, IBM, West Grove, PA, USA). Continuous and discrete variables were respectively compared between the patients and healthy controls using analysis of variance. Liner multiple regression was used to explore the association between the phases and characteristics of the patients. The relationships between the phase difference values and the HAMD scores were analyzed using Spearman’s rank-order correlation analysis. Multiple linear regression analysis was used to investigate the effects of the covariates on the phase difference. P values < 0.05 were considered statistically significant.
Results
Baseline Characteristics and Phase Difference
The characteristics of the participants are listed in Table 1. This study analyzed 45 patients (median age = 47.95 ± 13.30 years, 12 males) with depression, 154 (median age = 47.19 ± 4.48 years, 57 males) suspected of depression, and 48 healthy controls (median age = 47.29 ± 12.24 years, 19 males). The prevalence of hypertension and hyperlipidemia in the patients with depression and those suspected of depression was higher than in the control group.
There was no significant difference between left and right phase difference values in the patients and the controls. The phases in the patients suspected of depression and in those with depression were significantly lower than in the corresponding hemispheres of the healthy controls (Table 1, Figure 1).
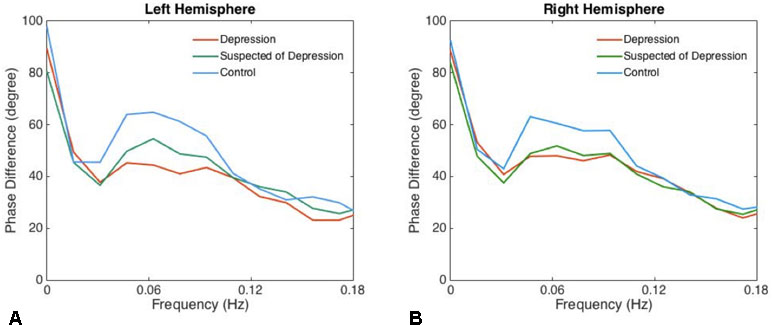
Figure 1 The phase differences of left (A) and right (B) derived from the transfer function within significant interval 0.06–0.12 Hz are plotted. Phase difference values (parameter of dynamic cerebral autoregulation) were significantly compromised in patients with depression and suspected of depression compared with the controls, indicating an impairment of dynamic cerebral autoregulation in patients with depression.
Multiple Linear Regression Analysis
Age, sex, diabetes, hypertension, hyperlipidemia, tobacco smoking habits, drinking habits, left middle cerebral artery, right middle cerebral artery, mean ABP, and heart rate did not influence the phase averages. However, when the level of depression increased (as evaluated by the HAMD score), the phase difference values were negatively correlated to the HAMD scores (7–17: 95% CI −13.825 to −2.911, P = 0.003; 17: 95% CI −19.725 to −5.802, P < 0.001) (Table 2).
Discussion
This study found that dynamic cerebral autoregulation was impaired in patients with depression and the phase difference value was negatively correlated with the HAMD score. Higher levels of depressive symptoms were associated with increased risk of neurological diseases such as stroke or TIA (27). The patients with depression also suffered from dizziness (28). Neurological diseases in patients with depression can be connected to impaired dynamic cerebral autoregulation.
The potential mechanisms underlying this phenomenon are unknown, but there are theoretical possibilities. Recent studies showed that the modulation of neurotransmitters can malfunction in depression (29, 30), and neurotransmitters such as serotonins have a major impact on cerebral vessel tone and could affect cerebral autoregulation (31, 32). In addition, the clinical evidence suggests increased pro-inflammatory markers in patients with depression (increased TNF-α, CRP, and IL-6) (33, 34). These inflammatory factors (35, 36) also can affect the endothelial cells and impair cerebral autoregulation (37). Therefore, depression may influence dynamic cerebral autoregulation through neuroendocrine and immunological/inflammation pathways.
Although various studies (38, 39) indicated that diabetes and hypertension are related to dynamic cerebral autoregulation, we did not find that they have significant influence on cerebral autoregulation. One possible explanation for this disparate finding might be related to the incidence of diabetes and hypertension. This study has a low rate of diabetes and hypertension, and we need to have a larger sample size to further explore their relationship.
In this study, the phase values were correlated with the depression levels. As the HAMD scores increased, the phase difference values (dynamic cerebral autoregulation) tended to decrease. The potential mechanisms are unclear. We supposed that the level of serotonins might be a potential mechanism to explain the relationship between the HAMD scores and the phase values. It has been proven that dysfunction of serotonin is related to major depressive disorder (40). Serotonins also have an impact on cerebrovascular function (41). The study of Edvinsson et al. suggested that serotoninergic projections played a significant role in the regulation of cerebral microvascular tone (42). Nevertheless, a study using PET found that citalopram (a selective serotonin reuptake inhibitor) led to alteration of cerebral hemodynamics (32). In addition, the negative correlation between phase values and the HAMD scale suggests the potential impact of depressive symptoms on dynamic cerebral autoregulation.
The impairment of dynamic cerebral autoregulation in depression indicates that cerebral vascular function may be a therapeutic target of depression. Therefore, improving dynamic cerebral autoregulation may potentially alleviate the neurological symptoms in patients with depression.
This study has some limitations. This was an observational study without in-depth research mechanisms. In addition, the sample size is relatively small. In the future, larger sample sizes and animal studies are needed. In terms of the TFA method, a phase shift is considered valid for further statistical analysis only when the linearity between ABP and CBFV is greater than 49% (24), and TFA is currently the only method that has been studied by multiple centers and standardized by a white paper for the assessment of cerebral autoregulation (23).
Conclusions
Dynamic cerebral autoregulation was compromised in patients with depression and negatively correlated with depression scores. The mechanism of impaired cerebral autoregulation may play a role not only in the development of cerebrovascular diseases but also as a potential therapeutic method for treating the neurological symptoms of depression.
Ethics Statement
This study was carried out in accordance with the recommendations of the Declaration of Helsinki and the Ethics Committee of the First Hospital of Jilin University with written informed consent from all subjects. All subjects gave written informed consent in accordance with the Declaration of Helsinki. The protocol was approved by the Ethics Committee of the First Hospital of Jilin University.
Author Contributions
YY, ML, and ZG drafted the manuscript. ZG and ML revised the manuscript. SL, XS, and HM drew the figures. ZW, PZ, HJ was in charge of acquisition of data. YQ and SL performed the data analysis. ML and PZ performed the statistical analysis. XS and YY conceived and designed the manuscript. All authors read and approved the final manuscript.
Funding
This article was supported by the National Natural Science Foundation of China (Grant No. 81571123), the National Key R&D Program of China (2016YFC1301600) and JLUSTIRT (2017TD-12) to YY.
Conflict of Interest Statement
The authors declare that the research was conducted in the absence of any commercial or financial relationships that could be construed as a potential conflict of interest.
References
1. Oakes P, Loukas M, Oskouian RJ, Tubbs RS. The neuroanatomy of depression: a review. Clin Anat (2017) 30(1):44–9. doi: 10.1002/ca.22781
2. McCarron RM, Vanderlip ER, Rado J. Depression. Ann Intern Med (2016) 165(7):ITC49–ITC64. doi: 10.7326/AITC201610040
3. Krishnan V, Nestler EJ. The molecular neurobiology of depression. Nature (2008) 455(7215):894–902. doi: 10.1038/nature07455
4. American Psychiatric Association. Diagnostic and statistical manual of mental disorders (DSM-5®). Washington, DC: American Psychiatric Pub (2013). doi: 10.1176/appi.books.9780890425596
5. Smith DJ, Court H, McLean G, Martin D, Langan Martin J, Guthrie B, et al. Depression and multimorbidity: a cross-sectional study of 1,751,841 patients in primary care. J Clin Psychiatry (2014) 75(11):1202–8; quiz 8. doi: 10.4088/JCP.14m09147
6. Kessler RC, Birnbaum HG, Shahly V, Bromet E, Hwang I, McLaughlin KA, et al. Age differences in the prevalence and co-morbidity of DSM-IV major depressive episodes: results from the WHO World Mental Health Survey Initiative. Depress Anxiety (2010) 27(4):351–64. doi: 10.1002/da.20634
7. Chida Y, Hamer M, Wardle J, Steptoe A. Do stress-related psychosocial factors contribute to cancer incidence and survival? Nat Clin Pract Oncol (2008) 5(8):466–75. doi: 10.1038/ncponc1134
8. Jackson JL, DeZee K, Berbano E. Can treating depression improve disease outcomes? Ann Intern Med (2004) 140(12):1054–6. doi: 10.7326/0003-4819-140-12-200406150-00017
9. Ramasubbu R, Patten SB. Effect of depression on stroke morbidity and mortality. Can J Psychiatry (2003) 48(4):250–7. doi: 10.1177/070674370304800409
10. Black SA, Markides KS, Ray LA. Depression predicts increased incidence of adverse health outcomes in older Mexican Americans with type 2 diabetes. Diabetes Care (2003) 26(10):2822–8. doi: 10.2337/diacare.26.10.2822
11. Barefoot JC, Helms MJ, Mark DB, Blumenthal JA, Califf RM, Haney TL, et al. Depression and long-term mortality risk in patients with coronary artery disease. Am J Cardiol (1996) 78(6):613–7.
12. Pan A, Sun Q, Okereke OI, Rexrode KM, Hu FB. Depression and risk of stroke morbidity and mortality: a meta-analysis and systematic review. JAMA (2011) 306(11):1241–9. doi: 10.1001/jama.2011.1282
13. Wang Y, Zhang H, Tang S, Liu X, O’Neil A, Turner A, et al. Assessing regional cerebral blood flow in depression using 320-slice computed tomography. PLoS One (2014) 9(9):e107735. doi: 10.1371/journal.pone.0107735
14. Vakilian A, Iranmanesh F. Assessment of cerebrovascular reactivity during major depression and after remission of disease. Ann Indian Acad Neurol (2010) 13(1):52–6. doi: 10.4103/0972-2327.61278
15. Monkul ES, Silva LA, Narayana S, Peluso MA, Zamarripa F, Nery FG, et al. Abnormal resting state corticolimbic blood flow in depressed unmedicated patients with major depression: a (15)O-H(2)O PET study. Hum Brain Mapp (2012) 33(2):272–9. doi: 10.1002/hbm.21212
16. Liao W, Wang Z, Zhang X, Shu H, Wang Z, Liu D, et al. Cerebral blood flow changes in remitted early- and late-onset depression patients. Oncotarget (2017) 8(44):76214–22. doi: 10.18632/oncotarget.19185
17. Lui S, Parkes LM, Huang X, Zou K, Chan RCK, Yang H, et al. Depressive disorders: focally altered cerebral perfusion measured with arterial spin-labeling MR imaging. Radiology (2009) 251(2):476–84. doi: 10.1148/radiol.2512081548
18. Wang S, Wang L, Jing P, Guo P, Zheng W, Li J, et al. Aberrant patterns of brain cerebral blood flow in Chinese han first-episode drug-naive depressive patients with and without a family history of depression. Oncotarget (2017) 8(45):79906–13. doi: 10.18632/oncotarget.20306
19. Colloby SJ, Firbank MJ, He J, Thomas AJ, Vasudev A, Parry SW, et al. Regional cerebral blood flow in late-life depression: arterial spin labelling magnetic resonance study. Br J Psychiatry (2012) 200(2):150–5. doi: 10.1192/bjp.bp.111.092387
21. Panerai RB. Assessment of cerebral pressure autoregulation in humans—a review of measurement methods. Physiol Meas (1998) 19(3):305–38. doi: 10.1088/0967-3334/19/3/001
22. Zhang R, Zuckerman JH, Giller CA, Levine BD. Transfer function analysis of dynamic cerebral autoregulation in humans. Am J Physiol (1998) 274(1 Pt 2):H233–41. doi: 10.1152/ajpheart.1998.274.1.H233
23. Claassen JA, Meel-van den Abeelen AS, Simpson DM, Panerai RB. Transfer function analysis of dynamic cerebral autoregulation: a white paper from the International Cerebral Autoregulation Research Network. J Cereb Blood Flow Metab (2016) 36(4):665–80. doi: 10.1177/0271678X15626425
24. Reinhard M, Muller T, Guschlbauer B, Timmer J, Hetzel A. Transfer function analysis for clinical evaluation of dynamic cerebral autoregulation—a comparison between spontaneous and respiratory-induced oscillations. Physiol Meas (2003) 24(1):27–43. doi: 10.1088/0967-3334/24/1/303
25. Ma H, Guo ZN, Sun X, Liu J, Lv S, Zhao L, et al. Hematoma volume is a predictive factor of disturbed autoregulation after spontaneous intracerebral hemorrhage. J Neurol Sci (2017) 382:96–100. doi: 10.1016/j.jns.2017.09.035
26. Kuo TB, Chern CM, Sheng WY, Wong WJ, Hu HH. Frequency domain analysis of cerebral blood flow velocity and its correlation with arterial blood pressure. J Cereb Blood Flow Metab (1998) 18(3):311–8. doi: 10.1097/00004647-199803000-00010
27. Everson-Rose SA, Roetker NS, Lutsey PL, Kershaw KN, Longstreth WT Jr., Sacco RL, et al. Chronic stress, depressive symptoms, anger, hostility, and risk of stroke and transient ischemic attack in the multi-ethnic study of atherosclerosis. Stroke (2014) 45(8):2318–23. doi: 10.1161/STROKEAHA.114.004815
28. Kim SK, Kim YB, Park IS, Hong SJ, Kim H, Hong SM. Clinical Analysis of dizzy patients with high levels of depression and anxiety. J Aud Otol (2016) 20(3):174–8. doi: 10.7874/jao.2016.20.3.174
29. Ghasemi M, Phillips C, Fahimi A, McNerney MW, Salehi A. Mechanisms of action and clinical efficacy of NMDA receptor modulators in mood disorders. Neurosci Biobehav Rev (2017) 80:555–72. doi: 10.1016/j.neubiorev.2017.07.002
30. Niciu MJ, Henter ID, Sanacora G, Zarate CA Jr. Glial abnormalities in substance use disorders and depression: does shared glutamatergic dysfunction contribute to comorbidity? World J Biol Psychiatry (2014) 15(1):2–16. doi: 10.3109/15622975.2013.829585
31. Hilz MJ, Stemper B, Heckmann JG, Neundorfer B. [Mechanisms of cerebral autoregulation, assessment and interpretation by means of transcranial Doppler sonography]. Fortschr Neurol Psychiatr (2000) 68(9):398–412. doi: 10.1055/s-2000-11798
32. Geday J, Hermansen F, Rosenberg R, Smith DF. Serotonin modulation of cerebral blood flow measured with positron emission tomography (PET) in humans. Synapse (2005) 55(4):224–9. doi: 10.1002/syn.20112
33. Steiner J, Bogerts B, Sarnyai Z, Walter M, Gos T, Bernstein HG, et al. Bridging the gap between the immune and glutamate hypotheses of schizophrenia and major depression: potential role of glial NMDA receptor modulators and impaired blood–brain barrier integrity. World J Biol Psychiatry (2012) 13(7):482–92. doi: 10.3109/15622975.2011.583941
34. Laske C, Zank M, Klein R, Stransky E, Batra A, Buchkremer G, et al. Autoantibody reactivity in serum of patients with major depression, schizophrenia and healthy controls. Psychiatr Res (2008) 158(1):83–6. doi: 10.1016/j.psychres.2006.04.023
35. Howren MB, Lamkin DM, Suls J. Associations of depression with C-reactive protein, IL-1, and IL-6: a meta-analysis. Psychosom Med (2009) 71(2):171–86. doi: 10.1097/PSY.0b013e3181907c1b
36. White RP, Vallance P, Markus HS. Effect of inhibition of nitric oxide synthase on dynamic cerebral autoregulation in humans. Clin Sci (Lond) (2000) 99(6):555–60. doi: 10.1042/cs0990555
37. Armstead WM, Hekierski H, Pastor P, Yarovoi S, Higazi AA, Cines DB. Release of IL-6 after stroke contributes to impaired cerebral autoregulation and hippocampal neuronal necrosis through NMDA receptor activation and upregulation of ET-1 and JNK. Transl Stroke Res (2018). doi: 10.1007/s12975-018-0617-z
38. Eames PJ, Blake MJ, Panerai RB, Potter JF. Cerebral autoregulation indices are unimpaired by hypertension in middle aged and older people. Am J Hypertens (2003) 16(9 Pt 1):746–53. doi: 10.1016/S0895-7061(03)00947-6
39. Vianna LC, Deo SH, Jensen AK, Holwerda SW, Zimmerman MC, Fadel PJ. Impaired dynamic cerebral autoregulation at rest and during isometric exercise in type 2 diabetes patients. Am J Physiol Heart Circ Physiol (2015) 308(7):H681–7. doi: 10.1152/ajpheart.00343.2014
40. Ruhe HG, Mason NS, Schene AH. Mood is indirectly related to serotonin, norepinephrine and dopamine levels in humans: a meta-analysis of monoamine depletion studies. Mol Psychiatry (2007) 12(4):331–59. doi: 10.1038/sj.mp.4001949
41. Ueda Y, Walker SA, Povlishock JT. Perivascular nerve damage in the cerebral circulation following traumatic brain injury. Acta Neuropathol (2006) 112(1):85–94. doi: 10.1007/s00401-005-0029-5
Keywords: depression, dynamic cerebral autoregulation, transcranial Doppler, transfer function, cerebral hemodynamics
Citation: Luo M-Y, Guo Z-N, Qu Y, Zhang P, Wang Z, Jin H, Ma H-Y, Lv S, Sun X and Yang Y (2019) Compromised Dynamic Cerebral Autoregulation in Patients With Depression. Front. Psychiatry 10:373. doi: 10.3389/fpsyt.2019.00373
Received: 14 October 2018; Accepted: 13 May 2019;
Published: 31 May 2019.
Edited by:
Julian Macoveanu, Copenhagen University Hospital, DenmarkReviewed by:
Anirban Dutta, University at Buffalo, United StatesJanusz Skrzypecki, Medical University of Warsaw, Poland
Copyright © 2019 Luo, Guo, Qu, Zhang, Wang, Jin, Ma, Lv, Sun and Yang. This is an open-access article distributed under the terms of the Creative Commons Attribution License (CC BY). The use, distribution or reproduction in other forums is permitted, provided the original author(s) and the copyright owner(s) are credited and that the original publication in this journal is cited, in accordance with accepted academic practice. No use, distribution or reproduction is permitted which does not comply with these terms.
*Correspondence: Yi Yang, doctoryangyi@163.com; Xin Sun, sjnksunxin@163.com
†These authors have contributed equally to this work.