- 1Division of Neurosurgery, Department of Surgery, University Health Network and University of Toronto, Toronto, ON, Canada
- 2The Department of Fundamental Neuroscience, The University of Lausanne, Lausanne, Switzerland
- 3INSERM, UMR-S 839, Paris, France
- 4Sunnybrook Research Institute, Sunnybrook Health Sciences Centre, University of Toronto, Toronto, ON, Canada
- 5Joint Department of Medical Imaging, University of Toronto, Toronto, ON, Canada
The habenula is a small bilateral epithalamic structure that plays a key role in the regulation of the main monoaminergic systems. It is implicated in many aspects of behavior such as reward processing, motivational behavior, behavioral adaptation, and sensory integration. A role of the habenula has been indicated in the pathophysiology of a number of neuropsychiatric disorders such as depression, addiction, obsessive-compulsive disorder, and bipolar disorder. Neuromodulation of the habenula using deep brain stimulation (DBS) as potential treatment has been proposed and a first successful case of habenula DBS was reported a decade ago. To provide an overview of the current state of habenula DBS in human subjects for the treatment of neuropsychiatric disorders we conducted a systematic review of both the published literature using PUBMED and current and past registered clinical trials using ClinicalTrials.gov as well as the International Clinical Trials Registry Platform. Using PRISMA guidelines five articles and five registered clinical trials were identified. The published articles detailed the results of habenula DBS for the treatment of schizophrenia, depression, obsessive-compulsive disorder, and bipolar disorder. Four are single case studies; one reports findings in two patients and positive clinical outcome is described in five of the six patients. Of the five registered clinical trials identified, four investigate habenula DBS for the treatment of depression and one for obsessive-compulsive disorder. One trial is listed as terminated, one is recruiting, two are not yet recruiting and the status of the fifth is unknown. The planned enrollment varies between 2 to 13 subjects and four of the five are open label trials. While the published studies suggest a potential role of habenula DBS for a number of indications, future trials and studies are necessary. The outcomes of the ongoing clinical trials will provide further valuable insights. Establishing habenula DBS, however, will depend on successful randomized clinical trials to confirm application and clinical benefit of this promising intervention.
Introduction
Neurological and psychiatric brain disorders emerge from the aberrant activity in brain circuits (1–4). Deep Brain Stimulation (DBS) employs precisely placed electrodes to deliver current to specific brain structures in order to modulate these dysfunctional circuits (1, 5). To date, well over 200,000 patients world-wide have been treated with DBS, most commonly for the management of movement disorders, such as Parkinson's disease (PD) (1). DBS offers advantages over other neuromodulatory treatments as it is non-lesional, reversible, and stimulation parameters can be adjusted as needed. The effectiveness of DBS depends upon appropriately and selectively stimulating and modulating the intended brain circuit(s) and is contingent on the selection of the optimal anatomical target and fine tuning the stimulation. For each DBS patient, stimulation parameters have to be individually optimized to maximize clinical benefits and minimize side-effects. This parameter optimization–or “programming”–, however, remains an empirical trial-and-error process that necessitates repeated clinic visits and is thus time- and resource-intensive (6) for both the patients and healthcare systems. A number of brain structures have been proposed as targets for DBS with multiple potential targets identified for most conditions (3, 7–12).
The habenula (Hb) is a relatively new DBS target that has been proposed to treat various psychiatric disorders, including depression (7, 13). It is a small bilateral epithalamic structure located adjacent to the posterior commissure in humans (14, 15) (Figure 1A). Invasive studies using animal models have shown that the Hb has extensive direct connections with the hypothalamus, brainstem nuclei, basal ganglia and limbic areas–the stria medullaris being the main afferent and the fasciculus retroflexus the main efferent fiber bundle (Figure 1B; Table 1) (17–19, 25–28). Studies in humans using imaging and electrophysiological techniques have shown multiple additional cortical and cerebellar regions to be functionally connected to the habenula (Figure 1B; Table 1) (20, 22–24, 28). It plays a key role in controlling the dopaminergic, serotonergic and noradrenergic systems (25, 27, 29–32). The Hb thus has a unique position regulating the three main monoaminergic systems.
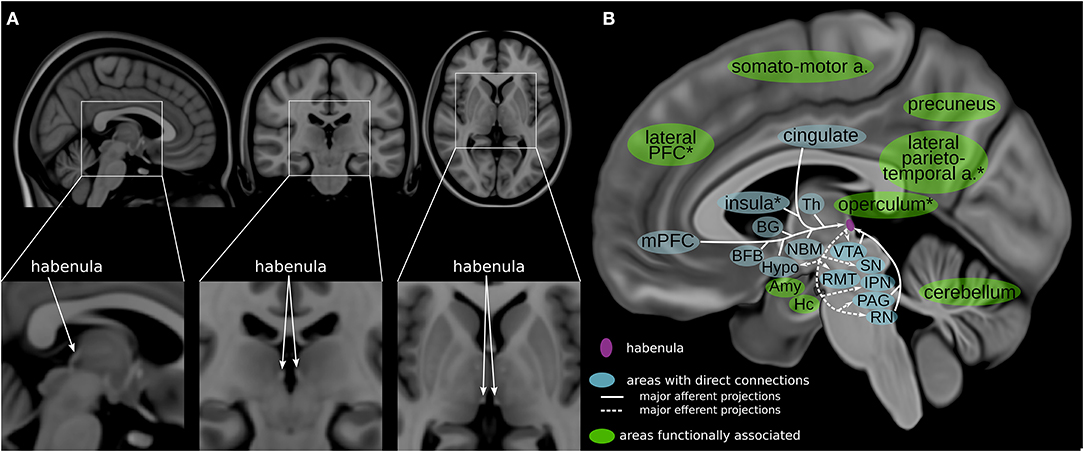
Figure 1. Habenula anatomy and connections. (A) The human habenula illustrated in orthogonal slices using the MNI template brain (MNI152b) (16). (B) Highlighting regions connected with the habenula (17–20) illustrated using a medial view of a high-resolution, high contrast template (21). The habenula is highlighted in purple. Areas with direct connections to the habenula are highlighted in blue; areas that are functionally associated with the habenula are highlighted in green. a, areas; Amy, amygdala; BFB, basal forebrain; BG, basal ganglia; Hc, hippocampus; Hypo, hypothalamus; IPN, interpeduncular nucleus; mPFC, medial prefrontal cortex; NBM, nucleus basalis of Meynert; PAG, periaqueductal gray; PFC, prefrontal cortex; RMT, rostromedial tegmental nucleus; RN, raphe nuclei; SN, substantia nigra; Th, thalamus; VTA, ventral tegmental area; *approximate/example position of the region.
Evolutionarily preserved across vertebrae, the Hb can be divided into a medial and a lateral Hb. The medial Hb is composed mainly of glutamate producing neurons that exert influence over the serotonergic system and are involved in emotional response selection (28, 33–35). The lateral Hb (LHb) is composed mainly of glutamate producing neurons that control midbrain dopaminergic neurons and is involved in reward related behavior (17, 31). Pioneering research analyzing the role of the Hb in processing reward related information by Hikosaka and others demonstrated that the LHb plays a key role in controlling adaptive behaviors (17). Neuronal activity in the LHb diminishes upon presentation of rewards, while activity increases after the presentation of aversive stimuli or cues predicting them (36–39). A series of studies indicate that maladaptations within the LHb underlie behavioral symptoms of major depression. Indeed, several cellular, and synaptic adaptations are causally linked to LHb hyperactivity, which consequently drives the expression of anhedonia and behavioral despair, which are typical aspects of mood disorders (40–42). Playing a key role in the control of all three major monoaminergic transmitter systems, the Hb controls a wide range of behavior beyond reward processing and depressive symptoms. The habenula has been connected to social interaction, motivational behavior, behavioral adaptation, pain processing and sensory integration, motor activity, memory, sleep, and circadian rhythm (17–19, 22, 43–46).
In a first seminal study, DBS within the Hb successfully ameliorated depressive symptoms in a patient where classic pharmacological treatment had failed (47). The components of an implanted DBS system and an example electrode targeting the Hb are illustrated in Figures 2A,B. These findings prompted a series of studies to assess whether DBS was similarly effective in animal models and to evaluate the underlying therapeutic mechanisms. Using a rodent model of depression named learned helplessness, an initial study reported an increased synaptic excitation onto LHb neurons concomitantly with increased neuronal activity of LHb cells with respect to control rats (41, 42). Adapted DBS-like electrodes were then inserted in the LHb, and high frequency stimulation, similar to that employed in humans, normalized the depressive-like state typical in learned helplessness rats (41). Importantly, DBS potently produced a time-locked collapse of glutamatergic transmission onto LHb neurons (41). This initial finding represented an initial indication that changes in cellular function in the LHb causally linked to depressive states, and that LHb-targeted DBS could represent a therapeutically-relevant strategy. In support of this, a different study employed early life stress to drive the emergence of depressive-like symptoms in adulthood that included defects in coping strategies and anhedonia (50). Mechanistically, the behavioral changes were associated with a reduction in the postsynaptic function of the metabotropic GABAb receptors (Figure 2C). The metabotropic GABAb receptor is a key cellular module for the maintenance of neuronal activity within the LHb (40). The reduction in GABAb function led to higher neuronal firing activity in LHb neurons (40, 50).
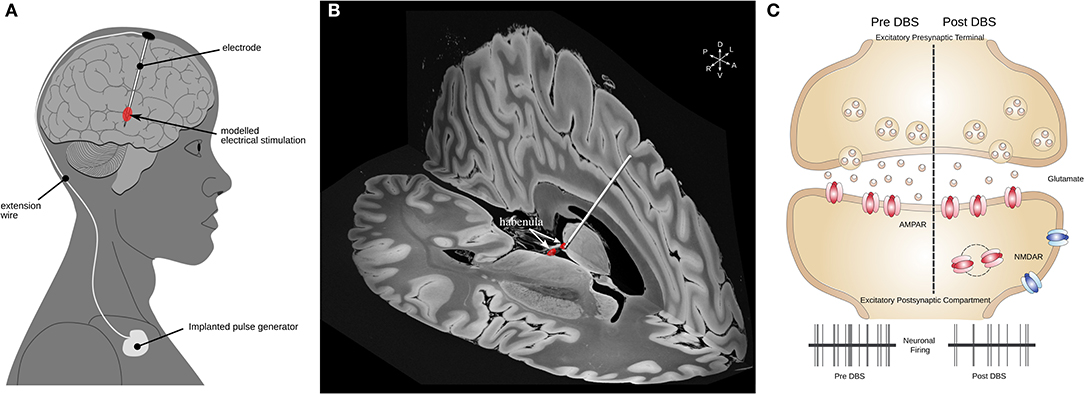
Figure 2. Habenular DBS and its mechanisms of action. (A) Schematic illustrating the components of a DBS system. (B) 3-D example image of a DBS electrode targeting the left Hb (red) illustrated in MNI152 space (16) using slices from a 100 micron resolution, FLASH 7 Tesla brain (48) electrode placement performed using Lead-DBS (49). (C) Mechanisms of action of DBS in LHb. A schematic of excitatory synapse is presented. Human-inspired DBS protocols in rodents lead to a reduction in glutamate release, which subsequently reduces the neuronal activity of LHb neurons (41, 50). DBS, deep brain stimulation; Hb, habenula; LHb, lateral habenula; MNI, Montreal Neurological Institute.
The authors used DBS-like electrodes in acute slices to show that this also causes a presynaptic reduction in glutamate release, as well as efficient reduction in neuronal firing rate assessed using in vivo recordings (50). Further, the use of DBS in behaving animals submitted to early life stress produced a normalization of the depressive-like state compared to non-stimulated animals (50). These studies not only unravel mechanisms of action of DBS in the LHb but support its use for therapeutically relevant interventions.
The potential of the Hb as a target for DBS goes beyond the context of depression. For example, DBS of LHb in rats reduced sucrose seeking and cocaine seeking behavior (51, 52) consistent with the putative role of Hb in addiction (17, 34, 53, 54). Furthermore, studies have demonstrated that the Hb plays a role in the pathophysiology of a number of neuropsychiatric disorders beyond depression and addiction such as schizophrenia (15, 55, 56), bipolar disorder (BD) (15, 33, 57–59), obsessive-compulsive disorder (OCD) (60, 61) and autism (62).
Given the multitude of potential therapeutic applications of Hb DBS, we conducted a systematic review of both the published human literature and the registered clinical trials to provide an overview of the current status of Hb DBS.
Methods
This systematic review was performed according to PRISMA guidelines (Figure 3). In March 2021, a literature search was conducted for original articles using PubMed/MEDLINE with the following search term: “habenula” AND (“DBS” OR “deep brain stimulation” OR “neuromodulation” OR “stimulation” OR “electrical stimulation”). No restrictions were placed on the publication date. No duplicates were found. Articles written in languages other than English, protocols, reviews, and opinion pieces as well as articles describing techniques other than DBS were excluded. All relevant articles were selected for full-text review and had to meet the following inclusion and exclusion criteria: (I) inclusion (articles reporting on the clinical outcome of Hb deep brain stimulation in humans) and (II) exclusion (studies reporting preclinical data; deep brain stimulation targeting a different brain region; articles reporting a patient population described previously).
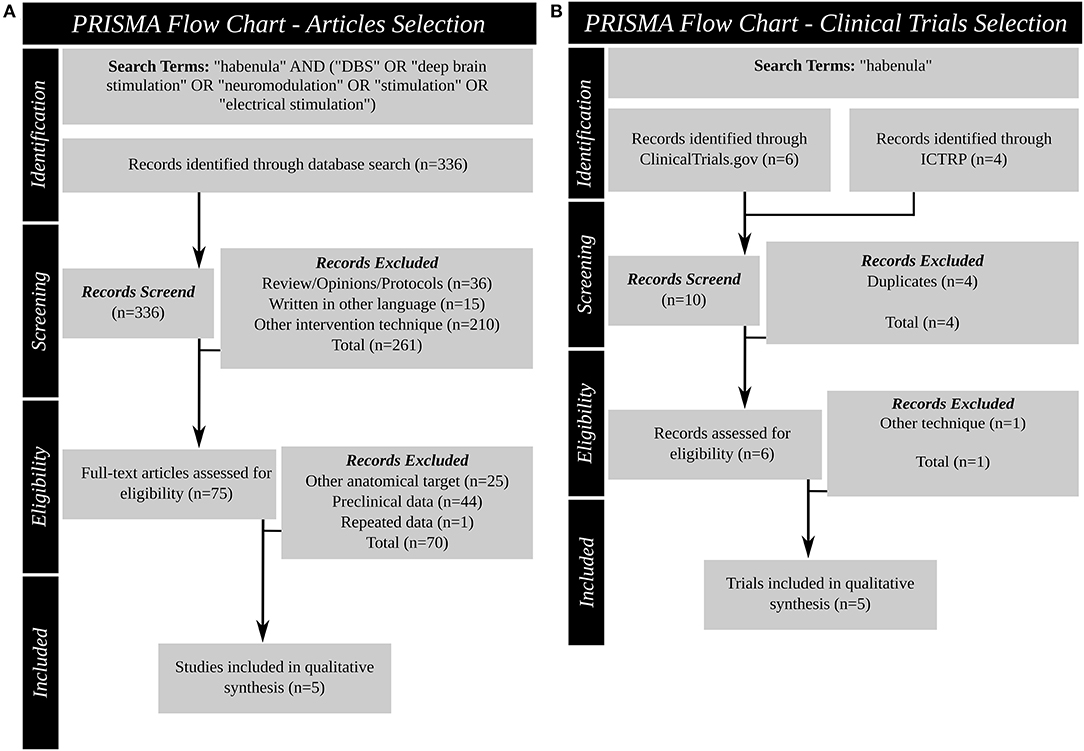
Figure 3. Systematic review PRISMA figures. (A): Article search (B): Clinical trial database search. DBS, deep brain stimulation; ICTRP, international clinical trials registry platform; PRISMA, Preferred Reporting Items for Systematic Reviews and Meta-Analyses.
Also in March 2021 two publicly available clinical trial databases were queried for past and ongoing clinical trials using “habenula” as search term: ClinicalTrials.gov (https://clinicaltrials.gov/) provided by the US National Library of Medicine, and the International Clinical Trials Registry Platform (ICTRP; https://www.who.int/ictrp/en/; https://apps.who.int/trialsearch/) of the World Health Organization (WHO). All entries since inception of the databases were queried. Duplicates were excluded. All relevant trials were selected for review and had to meet the inclusion and exclusion criteria: (I) inclusion (deep brain stimulation targeting the Hb as primary intervention) and (II) exclusion (intervention other than DBS).
Studies and trials were separately screened (JG and AT) and disagreement was resolved by consensus.
Results
Of the 336 items identified in the search, 36 were reviews, technical protocols or opinion pieces, 15 were not written in English, and 210 described an intervention other than DBS (e.g., optogenetic stimulation, neurochemical stimulation). The full text search of the remaining 75 studies 25 did not target the Hb, 44 described preclinical experiments and one study described data reported in an earlier manuscript. After screening, this systematic review identified five articles (Table 2) (47, 63–66) that satisfied the inclusion criteria. These articles reported the results of DBS targeting the Hb for four different indications: schizophrenia (63), treatment resistant major depressive disorder (TRD) (47, 65, 66), OCD (45) and BD (46).
Table 3 outlines the evidence and rationale the studies provide for using Hb DBS as treatment in each of the various psychiatric conditions.
Four of the five were single case studies (47, 64–66), one was a small case series of two patients (63). Clinical changes ranged from −9.5 to 100% symptom improvement in the overall six patients treated, with positive clinical outcome (improvement ≧31.7%) reported in 5 patients. The patients ranged in age from 21 to 64 years, disease duration at time of surgery varied from 4 to 46 years, and five of the six were male. One patient dropped out after 18 weeks for non-medical reasons (47) and all other patients were observed for at least >10 months. In all cases reported, Hb DBS stimulation was delivered via bilateral electrodes using a monopolar configuration and a pulse-width of 60 μs. Frequency of stimulation spanned from 60 to 160 Hz and voltage ranged from 1.35 to 10.5V. Studies describe numerous side effects of acute stimulation, all of which were subsequently controlled by altering the stimulation parameters (e.g., lower voltage, change contact, change frequency).
In addition to published articles, the search found 10 trial entries in the two clinical trial databases. The search results of these clinical trial databases provide insight into the current research investigating the habenula as a DBS target. Four duplicates were excluded and the records revealed that one trial did not use Hb DBS. Therefore, five clinical trials (Table 4) (NCT03463590, NCT03347487, NCT03254017, NCT01798407, NCT03667872) were identified using Hb DBS for two different indications: TRD (NCT03347487, NCT03254017, NCT01798407, NCT03667872) and OCD (NCT03463590). One trial (NCT01798407) is a non-randomized trial using quadruple masking, the other four are open label trials. Two of the publications identified in the literature search are associated with two of the clinical trials found: the study of Wang and colleagues (66) with NCT03667872 and the work of Zhang and colleagues (65) with NCT03254017. One trial is currently recruiting, one has been terminated, two are not yet recruiting and the status of the last is unknown. Bilateral Hb DBS is planned in all five trials and planned enrollment ranges from 2 to 13 patients, or 6 to 13 in the trials not terminated. China is the country of origin of four, the United states of one trial. All trials plan to recruit adults (from 18 or 21 years to 65 or 70 years of age) of both sexes.
Discussion
This literature review highlights the encouraging preliminary outcomes of Hb DBS for a variety of treatment-resistant psychiatric conditions (schizophrenia, BD, OCD, and TRD) and tentatively suggests it could be a viable therapeutic option for these conditions in the future. However, more comprehensive cases series and sham-controlled clinical trials with larger enrollment are necessary to confirm the effectiveness of Hb DBS. The review of the clinical trial databases revealed that there are a number of such trials currently underway. These larger trials will allow for a better understanding of the patient characteristics associated with greater Hb DBS benefits. For example, the authors of the Hb DBS trial for schizophrenia speculate that decreased symptom severity and shorter disease duration might play a role in determining treatment response (63). As the Hb is involved in the major neurotransmitter systems, which are the target of the most common pharmacotherapies used in these patient populations (i.e., antidepressants and antipsychotics), detailed reporting of the medication regime of patients (both before and repeatedly during Hb DBS treatment) would provide valuable information to elucidate the treatment mechanism of action. Different medication regimes as well as differences in lead localization might play a role in the great variance of DBS stimulation parameters observed in the reported studies: stimulation voltage between 1.35 and 10.5 V and frequency between 60 and 160 Hz were reported. It remains unclear if high- or low-frequency stimulation is most beneficial. For example, while Zhang and colleagues (65) report a marked improvement when switching from high- to low- frequency stimulation, Wang and colleagues (66) report good clinical outcome over 3 months with high-frequency stimulation.
Beyond the need for more additional clinical evidence, there are several challenges that should be overcome if Hb DBS is to become a useful therapeutic tool. First, greater insight into the mechanism of action of this therapy is required. This is especially important given the variety of different rationales and potential mechanisms that have been proposed for Hb DBS (Table 3). Further work with preclinical models will be needed to robustly test these proposals in the context of the various neuropsychiatric diseases. Second, given the critical relationship between the precise location and nature of the electric field and clinical response to DBS (78), knowledge of how best to target this relatively small structure and subsequently select the optimal stimulation parameters is needed. This is particularly relevant for psychiatric DBS indications given that established clinical programming algorithms of the sort employed for movement disorder patients rely largely on immediate and objective clinical feedback following parameter adjustment (e.g., improved tremor or rigidity in PD patients) (79, 80). These strategies may not be suitable for Hb DBS, where authors such as Sartorius and colleagues have reported a delay of 4 months between initiating DBS treatment and observing antidepressant effect (47). Indeed, two of the five studies report that determining the optimal stimulation parameters was time-consuming [taking 9 months for Zhang et al. (65); 8 months for Wang et al. (63)]. As such, Hb DBS–like other types of psychiatric DBS (81)–will likely benefit greatly from the identification of robust electrophysiological or neuroimaging biomarkers of efficacious stimulation. Furthermore, while most preclinical work supporting the potential use of Hb DBS in neuropsychiatric disorders has specifically focused on the function and connections of the LHb, the small size and close proximity of LHb and medial Hb make it difficult to precisely target (or visualize) only LHb in humans. Future clinical studies should therefore report DBS targeting and electrical field modeling with the utmost precision in order to evaluate the relationship between efficacy and the precise locus of stimulation within the Hb.
An array of experimental techniques may be useful for elucidating the mechanism of action and optimal treatment parameters for Hb DBS. Animal model and in vitro research using microelectrode recording, microdialysis, and optogenetic approaches have previously uncovered important insights into the neuronal and synaptic mechanisms of DBS for movement disorders and could be similarly applied here. Prior preclinical microelectrode recording work, for example, has shown that high frequency subthalamic stimulation leads to decreased neuronal activity in interconnected deep motor nuclei (82, 83) and suppressed local activity within the target structure (84). Microdialysis studies, which permit measurement of local neurotransmitter levels, indicate that high frequency stimulation is also accompanied by increased extracellular levels of GABA (85, 86). Optogenetic work, meanwhile, has provided further insight into the action of DBS at the ion channel level (87–89) and allowed investigations of the precise neuronal circuits underlying DBS responses (90, 91). These research modalities can be complemented by functional and molecular neuroimaging techniques such as positron emission tomography (PET), single photon emission computed tomography (SPECT), and functional magnetic resonance imaging (fMRI), which can be readily performed in patients and which capture information simultaneously from across the brain, thereby explicating the network-level effects of DBS (92–95). Indeed, recent fMRI studies have characterized brain-wide fingerprints of optimal subthalamic DBS for PD with respect to stimulation parameters such as contact, voltage, and frequency (96, 97). To this end, Zhang and colleagues used fMRI at various settings compared to a healthy control group (65) while Wang and colleagues conducted LFP recordings paired with a systematic assessment of the effects of stimulation (66).
Going forward, studies using these varying techniques will hopefully further elucidate the role of the Hb in psychiatric disease, advancing understanding of how best to modulate this structure and guiding optimization of DBS targeting and stimulation parameters. Ultimately, successful randomized, clinical trials with appropriate enrollment levels will be necessary to firmly establish the clinical benefit and optimal application of Hb DBS. Nevertheless, the reported studies (47, 63–66), albeit only reporting on a small number of patients, demonstrate that Hb DBS has potential for a multitude of clinical indications.
Data Availability Statement
The original contributions presented in the study are included in the article/supplementary material, further inquiries can be directed to the corresponding author.
Author Contributions
JG, MM, and AML: design of the work. JG, MM, and AT: acquisition of data. JG and MM: analysis of data and drafting the manuscript. JG, MM, GE, AL, FG, AB, and AML: interpretation of data. JG, MM, GE, AL, AT, FG, AB, and AML: critical revision of the manuscript. All authors approved the final version of the manuscript.
Conflict of Interest
The authors declare that the research was conducted in the absence of any commercial or financial relationships that could be construed as a potential conflict of interest.
Publisher's Note
All claims expressed in this article are solely those of the authors and do not necessarily represent those of their affiliated organizations, or those of the publisher, the editors and the reviewers. Any product that may be evaluated in this article, or claim that may be made by its manufacturer, is not guaranteed or endorsed by the publisher.
References
1. Lozano AM, Lipsman N. Probing and regulating dysfunctional circuits using deep brain stimulation. Neuron. (2013) 77:406–24. doi: 10.1016/j.neuron.2013.01.020
2. Fox MD. Mapping symptoms to brain networks with the human connectome. N Engl J Med. (2018) 379:2237–45. doi: 10.1056/NEJMra1706158
3. Lozano AM, Lipsman N, Bergman H, Brown P, Chabardes S, Chang JW, et al. Deep brain stimulation: current challenges and future directions. Nat Rev Neurol. (2019) 15:148–60. doi: 10.1038/s41582-018-0128-2
4. Bonelli RM, Cummings JL. Frontal-subcortical circuitry and behavior. Dialogues Clin Neurosci. (2007) 9:141–51. doi: 10.31887/DCNS.2007.9.2/rbonelli
5. Hammond C, Ammari R, Bioulac B, Garcia L. Latest view on the mechanism of action of deep brain stimulation. Mov Disord. (2008) 23:2111–21. doi: 10.1002/mds.22120
6. Koeglsperger T, Palleis C, Hell F, Mehrkens JH, Bötzel K. Deep brain stimulation programming for movement disorders: current concepts and evidence-Based strategies. Front Neurol. (2019) 10:410. doi: 10.3389/fneur.2019.00410
7. Hauptman JS, DeSalles AAF, Espinoza R, Sedrak M, Ishida W. Potential surgical targets for deep brain stimulation in treatment-resistant depression. Neurosurg Focus. (2008) 25:E3. doi: 10.3171/FOC/2008/25/7/E3
8. Lee D, Dallapiazza R, De Vloo P, Lozano A. Current surgical treatments for parkinson's disease and potential therapeutic targets. Neural Regeneration Res. (2018) 13:1342. doi: 10.4103/1673-5374.235220
9. Honey CR, Hamani C, Kalia SK, Sankar T, Picillo M, Munhoz RP, et al. Deep brain stimulation target selection for parkinson's disease. Can J Neurol Sci. (2017) 44:3–8. doi: 10.1017/cjn.2016.22
10. Sullivan CRP, Olsen S, Widge AS. Deep brain stimulation for psychiatric disorders: from focal brain targets to cognitive networks. Neuroimage. (2021) 225:117515. doi: 10.1016/j.neuroimage.2020.117515
11. Holtzheimer PE, Mayberg HS. Deep brain stimulation for psychiatric disorders. Annu Rev Neurosci. (2011) 34:289–307. doi: 10.1146/annurev-neuro-061010-113638
12. Lee DJ, Lozano CS, Dallapiazza RF, Lozano AM. Current and future directions of deep brain stimulation for neurological and psychiatric disorders. J Neurosurg. (2019) 131:333–42. doi: 10.3171/2019.4.JNS181761
13. Sartorius A, Henn FA. Deep brain stimulation of the lateral habenula in treatment resistant major depression. Med Hypotheses. (2007) 69:1305–8. doi: 10.1016/j.mehy.2007.03.021
15. Germann J, Gouveia FV, Martinez RCR, Zanetti MV, de Souza Duran FL, Chaim-Avancini TM, et al. Fully automated habenula segmentation provides robust and reliable volume estimation across large magnetic resonance imaging datasets, suggesting intriguing developmental trajectories in psychiatric disease. Biol Psychiatry Cogn Neurosci Neuroimaging. (2020) 5:923–9. doi: 10.1016/j.bpsc.2020.01.004
16. Fonov VS, Evans AC, McKinstry RC, Almli CR, Collins DL. Unbiased nonlinear average age-appropriate brain templates from birth to adulthood. Neuroimage. (2009) 47:S102. doi: 10.1016/S1053-8119(09)70884-5
17. Hikosaka O. The habenula: from stress evasion to value-based decision-making. Nat Rev Neurosci. (2010) 11:503–13. doi: 10.1038/nrn2866
18. Hu H, Cui Y, Yang Y. Circuits and functions of the lateral habenula in health and in disease. Nat Rev Neurosci. (2020) 21:277–95. doi: 10.1038/s41583-020-0292-4
19. Mizumori SJY, Baker PM. The lateral habenula and adaptive behaviors. Trends Neurosci. (2017) 40:481–93. doi: 10.1016/j.tins.2017.06.001
20. Ely BA, Stern ER, Kim J-W, Gabbay V, Xu J. Detailed mapping of human habenula resting-state functional connectivity. Neuroimage. (2019) 200:621–34. doi: 10.1016/j.neuroimage.2019.06.015
21. Neudorfer C, Germann J, Elias GJB, Gramer R, Boutet A, Lozano AM. A high-resolution in vivo magnetic resonance imaging atlas of the human hypothalamic region. Sci Data. (2020) 7:305. doi: 10.1038/s41597-020-00644-6
22. Torrisi S, Nord CL, Balderston NL, Roiser JP, Grillon C, Ernst M. Resting state connectivity of the human habenula at ultra-high field. Neuroimage. (2017) 147:872–9. doi: 10.1016/j.neuroimage.2016.10.034
23. Aizawa H, Yanagihara S, Kobayashi M, Niisato K, Takekawa T, Harukuni R, et al. The synchronous activity of lateral habenular neurons is essential for regulating hippocampal theta oscillation. J Neurosci. (2013) 33:8909–21. doi: 10.1523/JNEUROSCI.4369-12.2013
24. Damborská A, Honzírková E, Barteček R, Horínková J, Fedorová S, Ondruš Š, et al. Altered directed functional connectivity of the right amygdala in depression: high-density eEG study. Sci Rep. (2020) 10:4398. doi: 10.1038/s41598-020-61264-z
25. Herkenham M, Nauta WJ. Afferent connections of the habenular nuclei in the rat. A horseradish peroxidase study, with a note on the fiber-of-passage problem. J Comp Neurol. (1977) 173:123–46. doi: 10.1002/cne.901730107
26. Kim U, Lee T. Topography of descending projections from anterior insular and medial prefrontal regions to the lateral habenula of the epithalamus in the rat. Eur J Neurosci. (2012) 35:1253–69. doi: 10.1111/j.1460-9568.2012.08030.x
27. Herkenham M, Nauta WJ. Efferent connections of the habenular nuclei in the rat. J Comp Neurol. (1979) 187:19–47. doi: 10.1002/cne.901870103
28. Namboodiri VMK, Rodriguez-Romaguera J, Stuber GD. The habenula. Curr Biol. (2016) 26:R873–7. doi: 10.1016/j.cub.2016.08.051
29. Jhou TC, Geisler S, Marinelli M, Degarmo BA, Zahm DS. The mesopontine rostromedial tegmental nucleus: a structure targeted by the lateral habenula that projects to the ventral tegmental area of tsai and substantia nigra compacta. J Comp Neurol. (2009) 513:566–96. doi: 10.1002/cne.21891
30. Wang R, Aghajanian G. Physiological evidence for habenula as major link between forebrain and midbrain raphe. Science. (1977) 197:89–91. doi: 10.1126/science.194312
31. Stephenson-Jones M, Floros O, Robertson B, Grillner S. Evolutionary conservation of the habenular nuclei and their circuitry controlling the dopamine and 5-hydroxytryptophan (5-HT) systems. Proc Natl Acad Sci USA. (2012) 109:E164–73. doi: 10.1073/pnas.1119348109
32. Purvis EM, Klein AK, Ettenberg A. Lateral habenular norepinephrine contributes to states of arousal and anxiety in male rats. Behav Brain Res. (2018) 347:108–15. doi: 10.1016/j.bbr.2018.03.012
33. Fakhoury M. The habenula in psychiatric disorders: more than three decades of translational investigation. Neurosci Biobehav Rev. (2017) 83:721–35. doi: 10.1016/j.neubiorev.2017.02.010
34. McLaughlin I, Dani JA, De Biasi M. The medial habenula and interpeduncular nucleus circuitry is critical in addiction, anxiety, and mood regulation. J Neurochem. (2017) 142 Suppl 2:130–43. doi: 10.1111/jnc.14008
35. Lipina TV, Khrapova MV, Serykh A, Dubrovina NI, Petrova ES, Mikhnevich N, et al. The increased density of the habenular neurons, high impulsivity, aggression and resistant fear memory in disc1-Q31 l genetic mouse model of depression. Behav Brain Res. (2020) 392:112693. doi: 10.1016/j.bbr.2020.112693
36. Matsumoto M, Hikosaka O. Representation of negative motivational value in the primate lateral habenula. Nat Neurosci. (2009) 12:77–84. doi: 10.1038/nn.2233
37. Lecca S, Meye FJ, Trusel M, Tchenio A, Harris J, Schwarz MK, et al. Aversive stimuli drive hypothalamus-to-habenula excitation to promote escape behavior. eLife. (2017) 6:e30697. doi: 10.7554/eLife.30697
38. Lecca S, Namboodiri VMK, Restivo L, Gervasi N, Pillolla G, Stuber GD, et al. Heterogeneous habenular neuronal ensembles during selection of defensive behaviors. Cell Rep. (2020) 31:107752. doi: 10.1016/j.celrep.2020.107752
39. Nuno-Perez A, Trusel M, Lalive AL, Congiu M, Gastaldo D, Tchenio A, et al. Stress undermines reward-guided cognitive performance through synaptic depression in the lateral habenula. Neuron. (2021) 109:947–56.e5. doi: 10.1016/j.neuron.2021.01.008
40. Lecca S, Pelosi A, Tchenio A, Moutkine I, Lujan R, Hervé D, et al. Rescue of GABAB and GIRK function in the lateral habenula by protein phosphatase 2A inhibition ameliorates depression-like phenotypes in mice. Nat Med. (2016) 22:254–61. doi: 10.1038/nm.4037
41. Li B, Piriz J, Mirrione M, Chung C, Proulx CD, Schulz D, et al. Synaptic potentiation onto habenula neurons in the learned helplessness model of depression. Nature. (2011) 470:535–9. doi: 10.1038/nature09742
42. Li K, Zhou T, Liao L, Yang Z, Wong C, Henn F, et al. βCaMKII in lateral habenula mediates core symptoms of depression. Science. (2013) 341:1016–20. doi: 10.1126/science.1240729
43. Baker PM, Jhou T, Li B, Matsumoto M, Mizumori SJY, Stephenson-Jones M, et al. The lateral habenula circuitry: reward processing and cognitive control. J Neurosci. (2016) 36:11482–88. doi: 10.1523/JNEUROSCI.2350-16.2016
44. Benekareddy M, Stachniak TJ, Bruns A, Knoflach F, von Kienlin M, Künnecke B, et al. Identification of a corticohabenular circuit regulating socially directed behavior. Biol Psychiatry. (2018) 83:607–17. doi: 10.1016/j.biopsych.2017.10.032
45. Boulos LJ, Darcq E, Kieffer BL. Translating the habenula—from rodents to humans. Biol Psychiatry. (2017) 81:296–305. doi: 10.1016/j.biopsych.2016.06.003
46. van Kerkhof LWM, Damsteegt R, Trezza V, Voorn P, Vanderschuren LJMJ. Functional integrity of the habenula is necessary for social play behaviour in rats. Eur J Neurosci. (2013) 38:3465–75. doi: 10.1111/ejn.12353
47. Sartorius A, Kiening KL, Kirsch P, von Gall CC, Haberkorn U, Unterberg AW, et al. Remission of major depression under deep brain stimulation of the lateral habenula in a therapy-refractory patient. Biol Psychiatry. (2010) 67:e9–11. doi: 10.1016/j.biopsych.2009.08.027
48. Bianciardi M, Strong C, Toschi N, Edlow BL, Fischl B, Brown EN, et al. A probabilistic template of human mesopontine tegmental nuclei from in vivo 7 t MRI. Neuroimage. (2018) 170:222–30. doi: 10.1016/j.neuroimage.2017.04.070
49. Horn A, Li N, Dembek TA, Kappel A, Boulay C, Ewert S, et al. Lead-DBS v2: towards a comprehensive pipeline for deep brain stimulation imaging. Neuroimage. (2019) 184:293–316. doi: 10.1016/j.neuroimage.2018.08.068
50. Tchenio A, Lecca S, Valentinova K, Mameli M. Limiting habenular hyperactivity ameliorates maternal separation-driven depressive-like symptoms. Nat Commun. (2017) 8:1135. doi: 10.1038/s41467-017-01192-1
51. Friedman A, Lax E, Dikshtein Y, Abraham L, Flaumenhaft Y, Sudai E, et al. Electrical stimulation of the lateral habenula produces enduring inhibitory effect on cocaine seeking behavior. Neuropharmacology. (2010) 59:452–9. doi: 10.1016/j.neuropharm.2010.06.008
52. Friedman A, Lax E, Dikshtein Y, Abraham L, Flaumenhaft Y, Sudai E, et al. Electrical stimulation of the lateral habenula produces an inhibitory effect on sucrose self-administration. Neuropharmacology. (2011) 60:381–7. doi: 10.1016/j.neuropharm.2010.10.006
53. Lecca S, Meye FJ, Mameli M. The lateral habenula in addiction and depression: an anatomical, synaptic and behavioral overview. Eur J Neurosci. (2014) 39:1170–8. doi: 10.1111/ejn.12480
54. Yadid G, Gispan I, Lax E. Lateral habenula deep brain stimulation for personalized treatment of drug addiction. Front Hum Neurosci. (2013) 7:806. doi: 10.3389/fnhum.2013.00806
55. Stopper CM, Floresco SB. Dopaminergic circuitry and risk/reward decision making: implications for schizophrenia. Schizophr Bull. (2015) 41:9–14. doi: 10.1093/schbul/sbu165
56. Shepard PD, Holcomb HH, Gold JM. Schizophrenia in translation: the presence of absence: habenular regulation of dopamine neurons and the encoding of negative outcomes. Schizophr Bull. (2006) 32:417–21. doi: 10.1093/schbul/sbj083
57. Loonen AJM, Kupka RW, Ivanova SA. Circuits regulating pleasure and happiness in bipolar disorder. Front Neural Circuits. (2017) 11:35. doi: 10.3389/fncir.2017.00035
58. Savitz JB, Nugent AC, Bogers W, Roiser JP, Bain EE, Neumeister A, et al. Habenula volume in bipolar disorder and major depressive disorder: a high-resolution magnetic resonance imaging study. Biol Psychiatry. (2011) 69:336–43. doi: 10.1016/j.biopsych.2010.09.027
59. Schafer M, Kim J-W, Joseph J, Xu J, Frangou S, Doucet GE. Imaging habenula volume in schizophrenia and bipolar disorder. Front Psychiatry. (2018) 9:456. doi: 10.3389/fpsyt.2018.00456
60. Loonen AJM, Ivanova SA. Consider role of glutamatergic habenula-projecting globus pallidus in OCD. Pharmacopsychiatry. (2019) 52:203–4. doi: 10.1055/a-0835-6447
61. Stein DJ, Costa DLC, Lochner C, Miguel EC, Reddy YCJ, Shavitt RG, et al. Obsessive-compulsive disorder. Nat Rev Dis Primers. (2019) 5:52. doi: 10.1038/s41572-019-0102-3
62. Germann J, Gouveia FV, Brentani H, Bedford SA, Tullo S, Chakravarty MM, et al. Involvement of the habenula in the pathophysiology of autism spectrum disorder. medRxiv. (2021) doi: 10.1101/2021.04.08.21255122
63. Wang Y, Zhang C, Zhang Y, Gong H, Li J, Jin H, et al. Habenula deep brain stimulation for intractable schizophrenia: a pilot study. Neurosurg Focus. (2020) 49:E9. doi: 10.3171/2020.4.FOCUS20174
64. Zhang C, Zhang Y, Li D, Deng Z, Nuttin B, Voon V, et al. Habenular stimulation for neurosurgery resistant obsessive-Compulsive disorder: a Case report. Front Psychiatry. (2020) 11:29. doi: 10.3389/fpsyt.2020.00029
65. Zhang C, Kim S-G, Li D, Zhang Y, Li Y, Husch A, et al. Habenula deep brain stimulation for refractory bipolar disorder. Brain Stimul. (2019) 12:1298–300. doi: 10.1016/j.brs.2019.05.010
66. Wang Z, Cai X, Qiu R, Yao C, Tian Y, Gong C, et al. Case report: lateral habenula deep brain stimulation for treatment-Resistant depression. Front Psychiatry. (2020) 11:616501. doi: 10.3389/fpsyt.2020.616501
67. Proulx CD, Hikosaka O, Malinow R. Reward processing by the lateral habenula in normal and depressive behaviors. Nat Neurosci. (2014) 17:1146–52. doi: 10.1038/nn.3779
68. Lawson RP, Nord CL, Seymour B, Thomas DL, Dolan RJ, Dayan P, et al. Habenula responses during appetitive and aversive conditioning in major depressive disorder. J Neurol Neurosurg Psychiatry. (2014) 85:e3. doi: 10.1136/jnnp-2014-308883.31
69. Ambrosi E, Arciniegas DB, Curtis KN, Patriquin MA, Spalletta G, Sani G, et al. Resting-state functional connectivity of the habenula in mood disorder patients with and without suicide-Related behaviors. J Neuropsychiatry Clin Neurosci. (2019) 31:49–56. doi: 10.1176/appi.neuropsych.17120351
70. Zhang L, Wang H, Luan S, Yang S, Wang Z, Wang J, et al. Altered volume and functional connectivity of the habenula in schizophrenia. Front Hum Neurosci. (2017) 11:636. doi: 10.3389/fnhum.2017.00636
71. Sandyk R. Pineal and habenula calcification in schizophrenia. Int J Neurosci. (1992) 67:19–30. doi: 10.3109/00207459208994773
72. Li J, Yang S, Liu X, Han Y, Li Y, Feng J, et al. Hypoactivity of the lateral habenula contributes to negative symptoms and cognitive dysfunction of schizophrenia in rats. Exp Neurol. (2019) 318:165–73. doi: 10.1016/j.expneurol.2019.05.005
73. Fineberg NA, Apergis-Schoute AM, Vaghi MM, Banca P, Gillan CM, Voon V, et al. Mapping compulsivity in the dSM-5 obsessive compulsive and related disorders: cognitive domains, neural circuitry, and treatment. Int J Neuropsychopharmacol. (2018) 21:42–58. doi: 10.1093/ijnp/pyx088
74. Isobe M, Vaghi M, Fineberg NA, Apergis-Schoute AM, Bullmore ET, Sahakian BJ, et al. Set-shifting-related basal ganglia deformation as a novel familial marker of obsessive–compulsive disorder. Br J Psychiatry. (2021) 17: 249–60. doi: 10.1192/bjp.2021.45
75. Goodwin GM. The overlap between anxiety, depression, and obsessive-compulsive disorder. Dialogues Clin Neurosci. (2015) 17:249–60. doi: 10.31887/DCNS.2015.17.3/ggoodwin
76. Heinz A, Schlagenhauf F. Dopaminergic dysfunction in schizophrenia: salience attribution revisited. Schizophr Bull. (2010) 36:472–85. doi: 10.1093/schbul/sbq031
77. Barr LC, Goodman WK, Price LH. The serotonin hypothesis of obsessive compulsive disorder. Int Clin Psychopharmacol. (1993) 8(Suppl. 2):79–82. doi: 10.1097/00004850-199311002-00011
78. Elias GJB, Boutet A, Joel SE, Germann J, Gwun D, Neudorfer C, et al. Probabilistic mapping of deep brain stimulation: insights from 15 years of therapy. Ann Neurol. (2020) 89: 426–43. doi: 10.1002/ana.25975
79. Rizzone M. Deep brain stimulation of the subthalamic nucleus in parkinson's disease: effects of variation in stimulation parameters. J Neurol Neurosurg Psychiatry. (2001) 71:215–9. doi: 10.1136/jnnp.71.2.215
80. Volkmann J, Herzog J, Kopper F, Deuschl G. Introduction to the programming of deep brain stimulators. Mov Disord. (2002) 17(Suppl. 3):S181–7. doi: 10.1002/mds.10162
81. Bari AA, Mikell CB, Abosch A, Ben-Haim S, Buchanan RJ, Burton AW, et al. Charting the road forward in psychiatric neurosurgery: proceedings of the 2016 American society for stereotactic and functional neurosurgery workshop on neuromodulation for psychiatric disorders. J Neurol Neurosurg Psychiatry. (2018) 89:886–96. doi: 10.1136/jnnp-2017-317082
82. Benazzouz A, Piallat B, Pollak P, Benabid AL. Responses of substantia nigra pars reticulata and globus pallidus complex to high frequency stimulation of the subthalamic nucleus in rats: electrophysiological data. Neurosci Lett. (1995) 189:77–80. doi: 10.1016/0304-3940(95)11455-6
83. Benazzouz A, Gao DM, Ni ZG, Piallat B, Bouali-Benazzouz R, Benabid AL. Effect of high-frequency stimulation of the subthalamic nucleus on the neuronal activities of the substantia nigra pars reticulata and ventrolateral nucleus of the thalamus in the rat. Neuroscience. (2000) 99:289–95. doi: 10.1016/S0306-4522(00)00199-8
84. Beurrier C, Bioulac B, Audin J, Hammond C. High-frequency stimulation produces a transient blockade of voltage-gated currents in subthalamic neurons. J Neurophysiol. (2001) 85:1351–6. doi: 10.1152/jn.2001.85.4.1351
85. Windels F, Bruet N, Poupard A, Feuerstein C, Bertrand A, Savasta M. Influence of the frequency parameter on extracellular glutamate and gamma-aminobutyric acid in substantia nigra and globus pallidus during electrical stimulation of subthalamic nucleus in rats. J Neurosci Res. (2003) 72:259–67. doi: 10.1002/jnr.10577
86. Moro E, Esselink RJA, Xie J, Hommel M, Benabid AL, Pollak P. The impact on Parkinson's disease of electrical parameter settings in STN stimulation. Neurology. (2002) 59:706–13. doi: 10.1212/WNL.59.5.706
87. Gradinaru V, Mogri M, Thompson KR, Henderson JM, Deisseroth K. Optical deconstruction of parkinsonian neural circuitry. Science. (2009) 324:354–9. doi: 10.1126/science.1167093
88. Gunaydin LA, Yizhar O, Berndt A, Sohal VS, Deisseroth K, Hegemann P. Ultrafast optogenetic control. Nat Neurosci. (2010) 13:387–92. doi: 10.1038/nn.2495
89. Yu C, Cassar IR, Sambangi J, Grill WM. Frequency-specific optogenetic deep brain stimulation of subthalamic nucleus improves parkinsonian motor behaviors. J Neurosci. (2020) 40:4323–34. doi: 10.1523/JNEUROSCI.3071-19.2020
90. Mastro KJ, Zitelli KT, Willard AM, Leblanc KH, Kravitz AV, Gittis AH. Cell-specific pallidal intervention induces long-lasting motor recovery in dopamine-depleted mice. Nat Neurosci. (2017) 20:815–23. doi: 10.1038/nn.4559
91. Creed M, Pascoli VJ, Lüscher C. Addiction therapy. Refining deep brain stimulation to emulate optogenetic treatment of synaptic pathology. Science. (2015) 347:659–64. doi: 10.1126/science.1260776
92. Logothetis NK, Pauls J, Augath M, Trinath T, Oeltermann A. Neurophysiological investigation of the basis of the fMRI signal. Nature. (2001) 412:150–7. doi: 10.1038/35084005
93. Boutet A, Jain M, Gwun D, Rusjan P, Neudorfer C, Elias GJB, et al. “Modulation of CNS functions by deep brain stimulation: insights provided by molecular imaging. In: PET SPECT in Neurology. Dierckx RAJO, Ottee A, de Vries EFJ, van waarde A, Leenders KL, editors. Cham: Springer International Publishing (2021). p. 1177–244. doi: 10.1007/978-3-030-53168-3_46
94. Ko JH, Tang CC, Eidelberg D. Brain stimulation and functional imaging with fMRI and PET. Handb Clin Neurol. (2013) 116:77–95. doi: 10.1016/B978-0-444-53497-2.00008-5
95. Jech R. Functional imaging of deep brain stimulation: fMRI, SPECT, and PET. In: Tarsy D, Vitek JL, Starr PA, Okun MS, editors. Deep Brain Stimulation in Neurological and Psychiatric Disorders. Totowa, NJ: Humana Press (2008). p. 179–201. doi: 10.1007/978-1-59745-360-8_9
96. Boutet A, Madhavan R, Elias GJB, Joel SE, Gramer R, Ranjan M, et al. Predicting optimal deep brain stimulation parameters for Parkinson's disease using functional mRI and machine learning. Nat Commun. (2021) 12:1–13. doi: 10.1038/s41467-021-23311-9
Keywords: habenula, deep brain stimulation, clinical trial, depression, obsessive-compulsive disorder, schizophrenia, bipolar disorder
Citation: Germann J, Mameli M, Elias GJB, Loh A, Taha A, Gouveia FV, Boutet A and Lozano AM (2021) Deep Brain Stimulation of the Habenula: Systematic Review of the Literature and Clinical Trial Registries. Front. Psychiatry 12:730931. doi: 10.3389/fpsyt.2021.730931
Received: 25 June 2021; Accepted: 21 July 2021;
Published: 17 August 2021.
Edited by:
Wenbin Guo, Central South University, ChinaReviewed by:
Dianyou Li, Shanghai Jiao Tong University, ChinaGiacomo Grassi, University of Florence, Italy
Shyam Sundar Arumugham, National Institute of Mental Health and Neurosciences (NIMHANS), India
Copyright © 2021 Germann, Mameli, Elias, Loh, Taha, Gouveia, Boutet and Lozano. This is an open-access article distributed under the terms of the Creative Commons Attribution License (CC BY). The use, distribution or reproduction in other forums is permitted, provided the original author(s) and the copyright owner(s) are credited and that the original publication in this journal is cited, in accordance with accepted academic practice. No use, distribution or reproduction is permitted which does not comply with these terms.
*Correspondence: Andres M. Lozano, bG96YW5vQHVobnJlc2VhcmNoLmNh
†These authors have contributed equally to this work