- 1Department of Anthropology, Pennsylvania State University, University Park, PA, United States
- 2Huck Institutes of the Life Sciences, Pennsylvania State University, University Park, PA, United States
In the 21st century, neuropsychiatric disorders (NPDs) are on the rise, yet the causal mechanisms behind this global epidemic remain poorly understood. A key to these unknowns may lie within the vast communities of bacteria, fungi, and viruses in the body (microbiota), which are intimately linked with health and disease. NPDs were recently shown to be connected to gut microbiota, which can communicate with and influence the brain through the Gut-Brain-Axis (GBA). Parallel studies examining oral microbiota and their connections to the brain also suggest that microbes in the mouth can similarly influence NPD outcomes. However, the mechanisms and pathways that illuminate how oral microbiota and brain communicate in NPDs remain unknown. Here, we review identified mechanisms and pathways that oral microbiota use to engage the brain, and we lay the theoretical foundation for an oral-microbiota-brain axis (OMBA). Specifically, we examine established neuroinflammatory and immune system activation responses that underpin interactions between the oral microbiota and the central nervous system (CNS), detailing four specific mechanisms: (1) microbial and metabolite escape, (2) neuroinflammation, (3) CNS signaling, and (4) response to neurohormones. We then scrutinize why including the OMBA, in addition to the GBA, is critically needed to elucidate specific causal relationships between microbial dysbiosis and observed NPD development and progression. Furthermore, we argue for comprehensive, interdisciplinary approaches that integrate lab-based microbiome research and population-level studies that examine the OMBA to improve NPDs. We specifically identify key anthropological perspectives that integrate sociocultural, epidemiological, genetic, and environmental factors that shape the oral microbiome and its interactions with NPDs. Together, future studies of the OMBA in conjunction with interdisciplinary approaches can be used to identify NPD risks and improve outcomes, as well as develop novel intervention and treatment strategies.
Introduction
Microbiome research has been driven by new revelations regarding the complex interactions which contribute to human health and disease, particularly in the global rise of neuropsychiatric disorders (NPDs) and non-communicable diseases (NCDs) (1). Mental health has long been a confounding subject for researchers and practitioners of health and medicine, and breakthroughs in treatment and preventative measures are slow. This is largely due to the lack of understanding regarding the causal factors of neurophysiological and psychiatric disorders and is compounded by the complex physiological, genetic, social, and environmental factors that each contribute to mental and neurological health (2).
Increasingly, researchers and health experts recognize that no one factor, or microbe, can be exclusively to blame in NCD or NPD development, but recent findings have shown that the brain is much more connected to systemic health than previously believed. For example, the microbial communities within the body (microbiota) can play an integral role in NCDs, such as obesity and diabetes, and affect mental and neurological health (1–4). This research has predominantly focused on the GI tract, which contains most of the microbiota associated with the human body, both in numbers and species diversity (5). As a result, the primary findings in the context of microbe-brain interactions are facilitated through the Gut-Brain-Axis (GBA). The GBA refers to the complex bidirectional interactions and processes utilized by the gut microbiome and brain to communicate with one another (6). Broadly, the purpose of the GBA is to integrate gut functions with the brain’s emotional and cognitive centers. This is achieved through numerous mechanisms, including immune activation, entero-endocrine signaling, intestinal permeability, and enteric reflex (6, 7). Interest in the GBA and its connections to human health and behavior has led to an emerging field of study known as microbial endocrinology, which has sought to elucidate the functional pathways through which gut microbiota engage in the GBA (1, 2, 8–10). The primary pathway that allows interactions between the central nervous system and gut is primarily facilitated by hormones and mediated by the long branching vagus nerve (11).
As bacteria possess hormone receptors and perform and utilize quorum sensing, they can sense and produce autoinducer (AI) molecules, which function within bacteria in a similar manner to hormones in the host by regulating functions of bacterial growth, motility, and virulence (11, 12). It was further discovered that these AI molecules can also modulate host cell transduction and engage in crosstalk with host hormones which activate signaling pathways within the host organism (11, 13). The ability of AI molecules and hormones to interact in a similar fashion with both host and microbe forms the foundation of their communication and influence on one another. Commensal bacteria in the gut directly act on host hormones by producing and secreting hormones linked to host metabolism, immunity, and behavior ((7), and (11)). For example, a study by Iyer et al. (14) found that gut microbiota can influence the central nervous system (CNS) through production of hormones and other related molecules, such as serotonin, acetylcholine, histamine, and melatonin, which act as local neurotransmitters. Bacterial neuro-immuno-endocrine mediators are also involved in the breakdown of dietary components (15), immune system education, human development (16–18), and toxin degradation (11). As the microbe can modulate the host through AI, the host is also able to influence and alter gut microbiota through hormone production. A study conducted by Mudd et al. (19) reported catecholamines can modulate bacterial gene expression, with serum cortisol mediating the relationship between fecal Ruminococcus and brain N-acetylaspartate (NAA). The results indicated that the prescence of Ruminococcus was negatively associated with serum cortisol levels and brain NAA, a biomarker for neurological health. This can in turn affect the health of the host.
Microbial endocrinology and GBA research lay an important foundation for understanding functional processes of microbe–CNS interactions. This communication pathway between host and microbiome is not likely unique to the gut microbiome alone. It has been noted that functional pathways are conserved across microbiome sites and species composition of communities (16). Given that some microbes are tightly co-evolved with their hosts standpoint, it is logical that the microbiome can be in constant communication with the host’s neurophysiological system (20). Furthermore, the ability of bacteria to synthesize and recognize the same neurotransmitters that are found in the host suggests a bidirectional environment where the microbiome can influence the host and the host influence the microbiome. This communication between host and microbiome is mediated by a commonly shared evolutionary pathway of intercellular signaling (20). This once again indicated that host systems and microbial communities are not isolated from one another and supports the argument that there are tangible physiological pathways affecting mental health and illness associated with microbiota.
Although the GBA research has rapidly enhanced our understanding of microbe and CNS interactions, this research often focuses only on the lower digestive tract and neglects another important environment: the oral microbiome. The mouth is the beginning of the digestive system and a primary entry point for all things (microbial and otherwise) to access the internal body. As with the gut, oral microbiome research is shifting to perspectives focused on holistic, systems-level understandings of its functions and interactions with our bodies (21–24). Recent findings have shown that oral microbiome health is not only an indicator of oral health problems, such as caries and periodontal disease, but is also a key player in systemic disease, including obesity, diabetes, and neurological and psychiatric disorders (25–28). Indeed, oral microbial communities are likely involved in the same complex, bidirectional crosstalk between the brain and central nervous system as the gut microbiome. Although the oral microbiome has been comparatively less researched than the gut, current published literature shows promising complementary results to gut microbiome research, which points to similar neuro-immuno-chemical processes in host-oral microbe interactions.
The microbial diversity of bacteria, fungi, and viruses present in the mouth is second only to that of the gut and has led to more questions regarding the importance of these diverse and complex microbial networks in host health and development (29). There is established recognition among the medical, dental, and periodontal research fields that the oral microbiome plays an important role in systemic health (30). For example, disruption of the oral microbiome is a contributing factor to several chronic diseases across the body, including endocarditis, rheumatoid arthritis, and osteoporosis (31–34). The extent of oral microbial importance to health goes beyond the potential for bacterial pathogenesis infection. Oral health, gum disease, and periodontitis have been found to play a direct role in the development and progression of non-communicable diseases (NCDs), such as obesity (35), diabetes (36), and a number of cancers, including colorectal and esophageal cancer (37). With these insights, attention on the oral microbiome and its corollary relationship to systemic disease has focused on its exciting potential for early diagnostic purposes and intervention of NCDs. For example, Willis and Gabaldón (37) suggest that the presence of Lactobacillus in the mouth is associated with dental carries, and therefore, it could be used as an ancillary indicator of poor oral hygiene, which has a strong relationship to cancer development. In another example, Xiao et al. (38) notes the Craig et al. (35) study, where the ratio of Firmicutes-to-Bacteroidetes in the oral microbiota in children was strongly associated with weight gain. They conclude that the oral microbiome could be a valuable tool in assessing obesity risk. However, Xiao et al. (38) also concedes that we have yet to determine the direct mechanism underlying this relationship.
The results of these studies demonstrate that oral microbiota are directly involved in NPDs, and as with NCDs, exploration into diagnostic and intervention and treatment purposes is necessary. Current literature has posited the potential meanings for associations between certain oral species and NPDs, but there is yet to be a comprehensive attempt at compiling the direct mechanisms by which the oral microbiota affect the host CNS, and thereby cause or contribute to NPDs. This is a critical step in bringing the suggested benefits of oral microbiome research for health into actual medical practice.
Where previous reviews have focused on the indirect implications of oral microbial research in assessing risk for NPD and NCD development, this review will examine new research illuminating the direct causal mechanisms by which oral microbiota contribute to disease development and progression. Drawing on these studies, we propose a theoretical basis for an oral-microbiota-brain axis (OMBA): (1) microbial and metabolite escape, (2) neuroinflammation, (3) CNS signaling, and (4) response to neurohormones (Figure 1). In addition, we explore how applying this OMBA framework can help to connect microbial dysbiosis to NPD progression and outcome. Lastly, we will also argue that OMBA research needs to move beyond studies in microbiology, psychiatric, and nutritional health sciences and include anthropological methods and approaches to the study of the oral microbiome and mental health and discuss some potential oral microbiome-based solutions with implications for NPD treatment. For a summary highlighting the key studies and findings discussed in this review, please see Table 1.
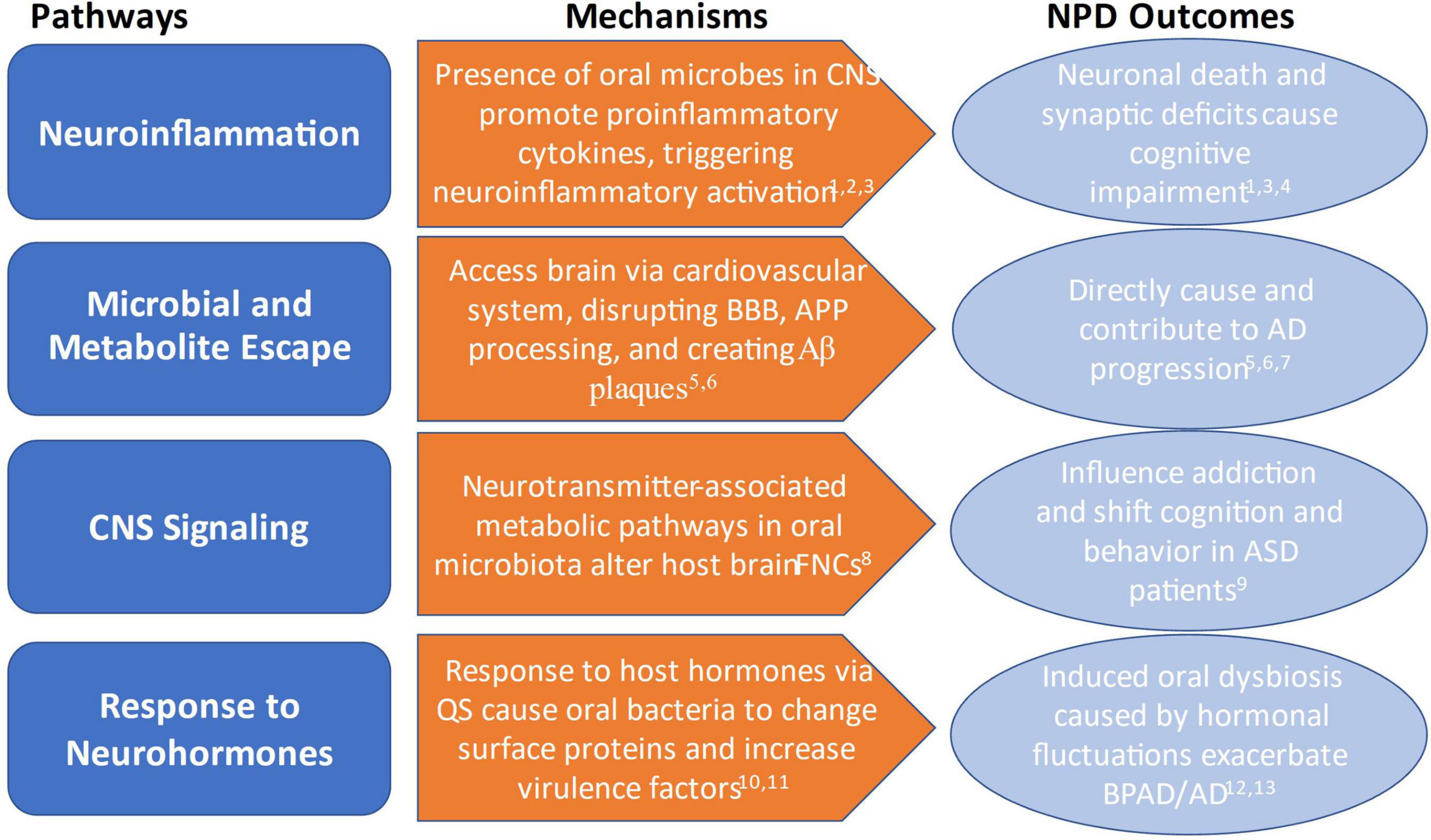
Figure 1. Overview of mechanisms by which oral microbiota influence and contribute to NPD outcomes. 1Xue et al. (25); 2Xia et al. (43); 3Dinarello et al. (94); 4Ding et al. (45); 5Bulgart et al. (39); 6Watanabe et al. (40); 7Dominy et al. (42); 8Lin et al. (26); 9Olsen and Hicks (50); 10Jentsch et al. (56); 11Duran-Pinedo et al. (30); 12Cunha et al. (28); 13Vieira et al. (33).
Microbial and Metabolite Escape and Disease
Many microbial species are site-specific in the host body, where they perform beneficial functions and are not typically associated with disease. However, when microbes escape from their niche and travel to other locations, they can cause disease elsewhere in the body by disrupting bioprocesses and secreting biproducts (e.g., metabolites), which can be toxic in some conditions. Microorganisms that colonize the body in the wrong spot can be a mechanism that underpins disease. This may be more critical in times of disease when oral microbial communities are ‘dysbiosed’ and certain potentially pathogenic microbes are in higher abundance.
Through the bloodstream, these escaped oral microbes and their metabolites travel to new regions of the body, where they can aggregate in different tissues causing diseases such as endocarditis and rheumatoid arthritis (31, 34). Oral microbiota can also make it to the brain via the cardiovascular system, where they are thought to be directly responsible for disruption of vital neurological functions and degradation of the brain through accumulation of toxic bioproducts (39). In mice, the collagen-binding activity of cnm-positive Streptococcus mutans, an oral microbe associated with dental carries, can leave the oral cavity in the blood and induce cerebral hemorrhaging by disrupting the blood–brain barrier (BBB). Endothelial cells of cerebral vessels are damaged during hemorrhage, exposing collagen on the surface of the blood vessel. If cnm-positive S. mutans is present in the bloodstream, its collagen binding activity can further cause hemorrhaging by adhering to and damaging the vascular endothelium in the brain (40). Similarly, the bacterium Porphyromonas gingivalis exists in many orally healthy people (41) but can be a key pathogen in the development and progression of periodontitis. However, P. gingivalis has been known to escape via the bloodstream and make its way to the brain, where it colonizes and releases neurotoxic proteases called gingipains. These metabolite gingipains are involved in disruption of the transmembrane protein Amyloid precursor protein (APP) processing, which is responsible for synaptic stability and neuronal growth and protection (39). This causes the improper cleaving of APP and creates amyloid beta (Aβ) protein plaques that in turn instigate neuronal death (39, 42).
The cascading affects caused by microbial and metabolite escape to the brain can directly result in disease. In individuals with Alzheimer’s Disease (AD), oral microbes, such as P. gingivalis, can directly affect disease progression and outcomes through the disruption of APP processing and subsequent Aβ accumulation (39, 42). AD is a progressive neurodegenerative disease demarcated by individuals that experiences both short- and long-term memory loss, mood swings, forgetfulness, and impaired decision making (39). Increased Aβ production leads to plaque buildup and increased the levels of phosphorylated tau, which is a protein essential for dendritic and axonal processes (39, 42). While oral microbial escape is now well appreciated, further research investigating which species are capable of escape and how they modify the CNS is critically needed, given the severe reactions associated with their escape in other diseases, such as cardiovascular disease.
Neuroinflammation
The presence of oral microbes and their byproducts within the bloodstream and CNS can have detrimental effects on the brain by stimulating the body’s neuroinflammatory response. During this process, expression of inflammatory mediators such as pro-inflammatory cytokines are promoted (25), mounting a sometimes mild, but constant immune response. Systemic inflammation can induce alterations in neurovascular functions, resulting in an increase in the blood–brain barrier permeability, reduction of nutrients, and buildup of toxins within the brain (43).
Oral microbes associated with dysbiosis and oral disease play critical roles in the production of pro-inflammatory cytokines, both in localized tissues and systemically (25). Proinflammatory cytokines, such as interleukin 1 (IL-1) and tumor necrosis factor (TNF), are molecules that can intensify a host’s immune response and are responsible for producing fever, inflammation, and tissue destruction (44). P. gingivalis has been shown to cause the body to release pro-cytokines TNF-α, IL-6, and IL-1β, resulting in neuroinflammation (45) during chronic periodontal disease (CP). CP is a polymicrobial condition which causes dysbiosis within the oral microbiome and is responsible for immune system activation (25). In fact, CP has been additionally linked to increased bacterial lipopolysaccharide (LPS) levels in the brain, which indicate the presence of bacteria and disruption of the BBB. With elevated LPS levels, expression of the host receptor toll-like receptor 4 (TLR4) also increases, triggering neuroinflammatory activation (25).
Neuroinflammation is likely to play key roles in the relationships between oral microbes and the CNS. A key example is how neuroinflammation associates with cognitive impairment (CI). CI is a pathological state associated with marked cognitive and neurological decline (46). It has been surmised that CP-induced neuroinflammation causes neuronal loss and synaptic deficits. This is crucial, as neural and synapse health are vital in maintaining normal cognitive function (25). As CP is a polymicrobial disease, it is likely that more species, beyond P. gingivalis, can contribute to neuroinflammation. Further research is needed to identify these oral microbes, explore the microbial mechanisms used to trigger this immune response during CP, and understand the levels of activation required to influence the CNS.
Furthermore, oral microbial involvement in NPDs may also be linked with broader systemic inflammation. Investigations of oral microbial involvement in neuroinflammation has found that oral dysbiosis can disrupt the GBA, causing gut inflammation and further contributing to systemic inflammatory response in the host (25). The cascading effect of oral microbial dysbiosis on the GBA may explain why chronic inflammatory diseases have been shown to co-occur with NPDs. For example, research on Inflammatory Bowel Disease (IBD), a group of disorders involving chronic inflammation of the digestive tract, noted that individuals with IBD had worse oral health and higher risk of periodontitis than those who did not have IBD (47). Other studies of IBD have found increased incidence, and prevalence of psychiatric disorders such as depression, anxiety, and bipolar disorders have also been noted in the IBD population (48). Further research into the influence of oral microbiota in chronic inflammatory diseases and NPD outcomes is needed to this point.
Central Nervous System Signaling
Recent findings have shed light on the ways that oral microbes can negatively influence neurological processes and shape cognition and behavior. However, oral microbial species may be vital to brain function, and therefore, altering microbial community composition could affect this by acting on specific neural pathways in the brain. Functional network connectivity (FNC) refers to the temporally dependent activation patterns of anatomically separate brain regions (49). These networks allow for different parts of the brain to communicate with one another and are responsible for functions such as cognitive-control, attention, and rapid information processing (26).
Analysis of FNC and oral microbial metabolic pathways in smokers identified enrichment of neurotransmitter-associated pathways in microbiota. These pathways include tyrosine metabolism and production of glutamate-glutamine and glutamatergic synapse. The production of neurotransmitters from the glutamine and glutamate pathway is stimulated by smoking and is involved in the reward circuit neural functions (26). In this way, oral microbiota could directly influence the reward pathways in the brain pertaining to smoking behavior and dependency, therefore altering the typical interactions between oral microbes and FNC (26). The FNC between networks involved in cognitive control and information processing were weaker in smokers, suggesting that oral microbiota may also play a role in dysregulating neurocognitive ability (26).
Breakthroughs in determining specific interactions between oral microbiota and brain circuit function are potentially valuable for studying psychiatric disorders as well. Research recently described an association between oral microbiota and Autism Spectrum Disorder (ASD). Research has indicated that children with ASD have lower oral bacterial diversity than their non-ASD peers, which is consistent with findings from the gut (50). The genera Haemophilus in saliva and Streptococcus in dental plaque were significantly more abundant in children with ASD, whereas Prevotella, Selenomonas, Actinomyces, Porphyromonas, and Fusobacterium were reduced. A depletion of the Prevotellaceae family co-occurrence network was also detected in plaque from ASD patients (50). This could point to the role of certain oral microbiota in exacerbating ASD symptoms, as ASD patients were noted to have increased abundances of bacteria associated with periodontal disease, immune activation, and inflammatory response. Further research utilizing study designs similar to that of Lin et al. (26) may illuminate causal factors of oral microbiota in shifting cognition and behavior in ASD patients. Downstream research using gnotobiotic mice or targeting the depletion of certain microbes in model systems may also provide further insights into how microbes contribute to routine brain function.
Another potential, yet understudied, mechanism by which oral microbiota may interact with the CNS is through afferent signaling via the vagus and trigeminal nerve complex. The vagus nerve runs from the brain to the abdomen and is responsible for relaying signals between the digestive tract and CNS. Research regarding gut microbiota interaction with the GBA has established that the vagus nerve is able to sense gut microbial metabolites via afferent fibers and transfer this information to the CNS (51). The trigeminal nerve is similarly responsible for innervating and relaying signals between the brain and oral and nasal cavities, and also contains connections to the vagus nerve via the main sensory nucleus (52). Therefore, it is likely that the same afferent signaling between gut microbes and the brain could very well be occurring within the mouth via the vagus and trigeminal nerve complex.
Response to Neurohormones and Neuroendocrine Signaling
Host hormones signal to the host and simultaneously influence the oral microbiota. Much of this research centers on the hypothalamic pituitary adrenal (HPA) axis, which is primarily responsible for coordinating the body’s response to stress through the release of catecholamines, such as cortisol. Cortisol is a primary regulatory factor in numerous host systems, including immune response, host growth, and development (53).
A number of studies examined the interactions between the oral microbiome and cortisol and showed host modulation of oral bacterial gene expression through cortisol (54, 55). Through quorum sensing, bacteria may use catecholamine hormones as siderophores—molecules which bind and transport iron. As such, catecholamines may also cause bacteria to change outer surface proteins and increase cytotoxic activity and other virulence factors (56). Exposure to increased levels of cortisol have been shown to oral microbial composition and caused upregulation of virulence factors in certain microbial species involved in periodontitis and carries, including Fusobacterium species and P. gingivalis. These shifts in oral microbial community composition are associated with increased inflammation associated with gingivitis and periodontal disease (30, 56).
Microbial response to hormones may also play a part in exacerbating psychiatric disorders, such as bipolar affective personality disorder (BAPD) (28). Differences in oral microbiome composition were reported in individuals with BAPD compared to those without, and an association between increased risk of CP was identified in those with BAPD versus healthy individuals (28). In participants with BAPD and CP, the abundance of Aggregatibacter actinomycetemcomitans and P. gingivalis counts were significantly higher, and periodontitis was strongly associated with the total bacterial load and the depressive phase of BPAD, which also impacts catecholamine levels (28). This may suggest that CP induced by oral microbial response to shifts in hormones may exacerbate the symptoms of BPAD, and vice versa. In addition, PD may also be associated with neuroendocrine changes due to aging and cognitive impairment leading to AD. The primary factor responsible is thought to be an increase in cortisol with age, which induces oral microbial dysbiosis leading to PD (57). Women have an increased risk of developing PD and oral inflammation in old age (33), which may be due to decreases in estrogen (an important immune regulator) during menopause and increases in cortisol with aging (33). The immune deficits observed with lower estrogen, combined with increased cortisol levels, could be vital cues in changing oral microbial composition and inducing PD-associated dysbiosis. This may also be a responsible factor for why a large number of AD patients are female (33). However, much more research is needed to establish the functional mechanisms behind these relationships and explore how oral microbiota may respond to a plethora of different hormones.
Anthropological Insights Into Microbiome Research With Implications for Neuropsychiatric Disorders
Establishing the direct functional pathways through which the human microbiome affects health outcomes, especially in the nervous system, is vital, but it is only one piece of the puzzle. As noted above, the oral microbiome is influenced by the host but is also shaped by our lifestyles (41), environments (58), diets (59), and exposures to toxins (26). Oral microbial dysbiosis associated with disease can be triggered by changes brought about through environmental disturbance and dietary shifts, leading to host disease (58, 60). Thus, understanding the human factors that shape our microbiome is likely to be an important mediator of NPD disease outcomes.
Currently, most microbiota studies, regardless of disease state, are from Industrialized people from the United States of America or China (61) and include data from Western, educated, industrialized, rich and democratic (WEIRD) populations (37). Despite making up less than 20% of the world population, “WEIRD” populations have historically accounted for 60–90% of subjects in psychological studies (62). As behavioral presentations of mental illness are heavily influenced and filtered through our sociocultural experiences, models linking oral microbiota and NPDs that are built using only a narrow range of participants are inadequate to fully understand and diagnose NPDs, nor understand how different oral microbiota compositions influence NPDs and other diseases (1, 63). While psychiatry has made a push to include models which incorporate culture as a factor which influences peoples’ experience of illness, there is still a need for theory that addresses the complex interactions between sociocultural and biological factors which produce NPDs across the diversity of human experiences (64). Anthropology can provide this missing theoretical framework to address this, and thus contribute to the perspective in current microbiome and mental health research, by situating lifestyle factors such as diet, behavior, and environment within the context of NPDs and the oral microbiome.
Anthropological perspectives allow researchers to disentangle the factors that shape microbial diversity and community composition of the oral microbiome (26, 41, 65), which in turn can influence NPDs. For example, NPDs are being investigated with particular focus on nutrition and diet (1, 10), but there is limited information on how this links to microbial shifts associated with lifeways (e.g., culture). Some of this research has been done using interdisciplinary methods between psychiatry and nutrition, dubbed “nutritional psychiatry,” as this field focuses on the influences of diet on the GBA as an integral factor in neuropsychiatric disorders (10); however, this work has excluded the OMBA, especially in the context of diverse lifeways lived by different Indigenous people globally. This is critical, as large-scale dietary differences have been shown to influence oral microbiota, as they do gut microbiota. For example, oral microbial samples from hunter gatherer and traditional farming communities in the Philippines revealed that, when compared to data from individuals living on a Western diet, the abundance of core microbial oral species were significantly correlated with subsistence strategy (41). Other examples have demonstrated that some Indigenous and traditional hunter-gatherer populations carry higher loads of potential oral pathogens that are linked to PD, such as Prevotella, Porphyromonas, Treponema, and Tannerella, than traditional farmers (41, 60, 66, 67), despite having less PD in those hunter-gatherer communities. As such, research examining intertwining dietary and nutritional differences in the context of human cultural, lifestyle, and environmental differences is needed to better understand the relationships between OMBA and NPDs.
Similarly, anthropology can help improve our understanding of OMBA and NPD linkages by examining how different histories and lifeways affect oral diseases that may differentially influence health, such as caries or periodontal disease. For example, although there is still limited research regarding oral microbial diversity among Indigenous groups, analyses of oral microbiota from Native American individuals indicated that the microbial communities of Indigenous participants significantly differed from their non-native counterparts, including high abundances of the genus Prevotella, which have been implicated in periodontal disease (68). In addition, it is also established that Aboriginal Australians and Torres Strait Islanders also suffer from higher incidences of NPDs and NCDs than their non-Indigenous counterparts (69). Recent research suggests that this health disparity may be complicated by the higher prevalence of oral disease, such as tooth decay and moderate to severe periodontal disease, experienced by Indigenous Australians in comparison to non-Indigenous Australians (69). These systemic differences were correlated with established differences microbial diversity and composition of Indigenous and non-Indigenous Australians, suggesting that these differences in oral microbiota-related diseases may be specific to Indigenous Australians via a unique oral microbiome (69). This oral microbial dysbiosis may be the result of evolutionary or sociocultural differences or, more likely, are reinforced by economic and social inequalities that result in greater burdens of disease. Higher densities of oral disease in certain populations or groups of people could result in higher incidences of downstream systemic diseases. While this observation shapes our understanding of how oral disease may be linked to mental health outcomes, this relationship between microbes and disease is likely to be unique across different human populations and therefore need an anthropological lens.
Anthropology also provides a structure by which to examine sociocultural factors effecting oral and mental health. For example, social and community interactions impact oral microbiome composition. Close family members and romantic partners possess similar microbial compositions to one another, likely facilitated by similar exposures as well as transferring of microbes via direct contact (70, 71). This is important to consider, as these could contribute to an individuals’ oral microbial composition and health. For example, evidence suggests that offspring of parents with periodontitis are preferentially colonized by bacteria known to be involved in periodontitis, including P. gingivalis, A. actinomycetemcomitans, Streptococcus parasanguinis, and Fusobacterium nucleatum (71). Furthermore, plaque control did not appear to change this, suggesting that interventions at the individual level through dental hygiene may not be adequate (71). Microbial inheritance from parent to child is an important aspect of periodontal disease, and thus may influence NPD risk and outcomes. As clinicians often place the emphasis on oral hygiene as the solution to slowing periodontitis, and now potentially NPDs, other contributing factors, such as inherited microbes, may not be within the individual’s control and need further mitigation.
Examination of sociocultural influences on the oral microbiome may also be extended to gender. Very recent studies have indicated significant differences in oral microbial community composition and abundances between adolescent girls and boys and elderly men and women (72, 73). However, it should be noted that these findings are likely confounded by biological factors of sex. Research has also found that sex and gender are major influencing factors in NPD presentation and outcome (74). This has led to a call for inclusion of gender and sex in a more tailored approach to NPD diagnosis and treatment (74). Therefore, it is important that such research be expanded to include the wider spectrum of gender identities in order to assess how the social constructs of gender may play a role in shaping the relationship between oral microbiota and NPDs.
Further to this, larger sociocultural influences, such as social and economic injustice that can increase periodontitis occurrence and exacerbate NPDs, can also be better understood with an anthropological lens. Anthropologists have drawn heavily on structural violence theory – a tripartite model of cultural violence, structural violence, and direct violence (75) that results from the institutionalization of cultural power structures and blocks access to care, opportunities, and resources, and marginalize specific populations—to identify and understand why some groups are disproportionately affected by oral diseases and vulnerable to NPDs. For example, Indigenous populations experience worse health, higher NCD and NPD burdens, and larger oral disease prevalence than non-Indigenous populations (69, 76). Studies examining the relative oral health disparities between Indigenous and non-Indigenous persons from New Zealand, Brazil, Australia, Canada, and the United States have found that Indigenous people consistently experience worse oral health than their non-Indigenous counterparts regardless of location (77, 78). Experiencing higher levels of structural violence could influence microbial exposure, colonization, and microbiome responses (i.e., microbiome’s response to stress hormones), which would ultimately alter systemic disease outcomes and therefore predispose Indigenous peoples to higher NCD and NPD burden. Further, early life stress may also contribute indirectly to NPD development later in life via the oral microbiome by shifting microbiota composition toward a disease-associated state (71, 78, 79). Without research and intervention efforts that examine sociocultural and environmental exposures across different settings, these health gaps will continue to exist. Figure 2, summarizes these concepts regarding the relationship between the OMBA and sociocultural and environmental factors.
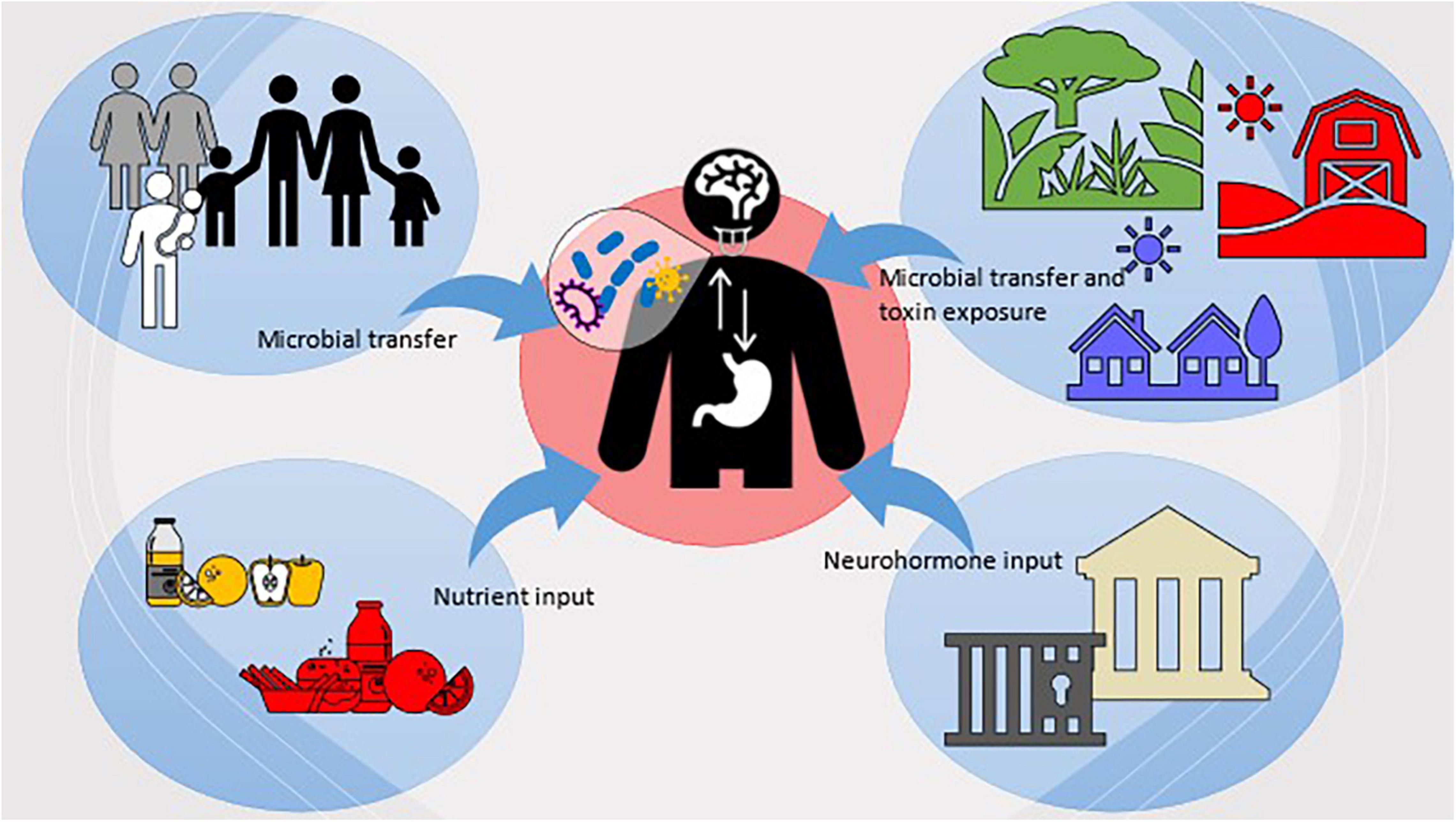
Figure 2. Visualization of the sociocultural and environmental factors which influence host microbiota and the OMBA.
Solutions and Preventative Measures in Treating Neuropsychiatric Disorders Through the Oral Microbiota
Expanding our understanding of the environmental factors and microbial taxa associated with a healthy oral microbiome will open new avenues for intervention and prevention of NPDs. However, this knowledge should also underpin novel strategies to modulate or alter the microbiota to limit disease. Several tools already exist to manipulate or modulate oral microbiota, albeit with varied effectiveness, while new tools and pharmaceutical interventions are currently being developed. These single approaches or combined approaches may provide unique solutions or novel intervention therapies in how health practitioners think about preventing and addressing NPDs.
Antimicrobials are our first line of defense against pathogenic infection and work to eliminate specific species (i.e., phage treatment) or whole groups or families of microorganisms (i.e., antibiotics). Removing microbes found to be associated with NPDs may be a new way of addressing the symptoms or severity of NPDs in the future. However, antibiotics administered orally have been shown to have little impact on oral microbiota (80), suggesting that topical or direct application of antibiotics in the oral cavity may be more effective. Further, phage therapies designed against oral microbes have had little efficacy (i.e., against F. nucleatum). As such, encouraging growth of beneficial or potentially non-pathogenic microbes in the mouth may be a more appropriate way to modify the oral microbiota in support of addressing NPDs.
Probiotics have been increasing in popularity over the past decade as a form of health maintenance and disease prevention (81). Some research has indicated that Lactobacillus and Bifidobacterium spp. can aid in controlling the growth of cariogenic streptococci in the mouth (82). However, the effectiveness of probiotics often only lasts as long as the user is actively taking them, suggesting that the bacteria need additional factors to successfully colonize the oral cavity (83). Furthermore, most clinical trialing of these probiotics occurs within WEIRD populations, making it difficult to speak to their efficacy across populations. Identifying new microbial strains which contribute to oral health in individuals, as well as the environmental factors which dictate their success, will be vital in creating more beneficial probiotics to treat oral disease, and potentially act as preventatives for NPDs.
A novel therapy that seeks to address key holes in probiotic research is the implementation of microbial transplantations. Microbiota from a healthy donor are transplanted or seeded into the body of the recipient. While most research in microbial transplants has focused on the lower digestive tract, as fecal microbial transplants are successfully used to treat antibiotic-resistant Clostridium difficile infections (84), this technology is currently being developed in the mouth (61). Oral microbial transplants (OMTs) may be able to serve as a first line of defense against caries or be used as a treatment of periodontal disease, but this has not been tested in humans (85). In the future, applications for OMTs should be explored for their use in alleviating symptoms of systemic diseases, such as NPDs. Nevertheless, OMT therapies should account for evolutionary, environmental, and sociocultural factors which may affect transplant efficacy and utilize oral microbiota that have not been linked to NPDs.
Oral hygiene products, like toothpastes and mouthwashes, manage oral microbial communities by limiting growth of certain species and may be a way to promote colonization of desired oral microbes. For example, current toothpastes are formulated with chemicals with antimicrobial properties, such as fluoride, which has been shown to decrease overall microbial load and diversity (86) in addition to promoting healthy enamel. Further, many mouthwashes contain alcohol to kill microorganisms. While these are tools used in daily hygiene practices to prevent and reduce oral disease, they may also be tools to help modulate microbes that are linked to NPDs. New research is examining additional chemical compounds that may maintain oral hygiene without disrupting the commensal balance in microbiota (87). These compounds could also be examined in the context of treating or reducing NPDs symptoms in the future.
An important component to consider in researching preventative measures and interventions for oral disease are the biofilms created by microbiota. Biofilms are the extracellular matrices formed by colonies of oral microbes on the hard surfaces of dentition (88). These biofilms adhere to the tooth surface and encapsulate microbiota within a protective layer of secreted polymers and allow microbes to resist environmental changes (88). Microbial communities are also able to alter biofilm phenotypes in response to change via gene expression patterns (88). Because of this, oral microbial biofilms are notoriously capable of resisting removal and antibiotic or antimicrobial treatment efforts (88, 89). Thus, biofilms are likely to play a significant role in transplant success or antimicrobial product efficacy, and could potentially play a role in NPD development and treatment response.
Lastly, oral microbiomes can also be modulated by shifting one’s lifeway or diet, as discussed above, or potentially by changing one’s environmental exposures. Research is increasingly linking human health and the microorganism in the body to the environments they live in, both through exposure to pollutants and chemicals, but also diverse sets of environmental microbes. A One Health perspective is useful in these contexts, which posits that our health is reliant on the external health of our environments and ecosystems. Using a One Health approach could be valuable to inform health policies aimed at targeting oral and mental health and examining how exposure to environmental microbes may influence oral health. There is precedence of the introduction of environmental microorganisms into the mouth, both via dietary food and water sources (90), although limited research exists in this area. Nevertheless, environmental exposures may play a role in how we think about modulating oral microbiota to address systemic health concerns. For example, city planning for urban areas to include greater diversity of soil microbes through incorporation of natural “green spaces” could help to increase population exposure to beneficial microbes (91), as exposure to these environmental microbes may play key roles in the treatment of NPDs. Specifically, exposure to the soil bacterium Mycobacterium vaccae has been shown to have an anxiolytic effect on the host, as the host’s immune response releases anti-inflammatory cytokines that have positive effects on reducing inflammation in the body and brain—a major component in anxiety and depression (92, 93). However, mechanisms that are facilitated via the oral microbiome have not yet been identified. Nevertheless, implementing social policies that provide health, environmental exposures (e.g., requiring that children have time to engage with these spaces daily during school), could further serve to ensure that people are able to consistently access and benefit places with diverse environmental microbes (91).
Microbiome research on NPDs is still in its infancy, but what has been published regarding the OMBA suggests that it may play a critical role in mental and physical health. Already, there are efforts to apply these novel findings in creating treatments for a range of NPDs and NCDs. However, there are still a large number of unknown factors which contribute to the OMBA in healthy and diseased states. As such, when it comes to regulatory policies regarding new oral products, a stance based on precaution would be advisable until further research is able to establish what risks to consumer health they may pose.
Conclusion
Oral microbiota likely play key roles in the development, progression, and symptoms of NPDs via the OMBA. An interdisciplinary approach to studying the OMBA can help identify unique factors that influence the oral microbiome and potentially mental health outcomes at individual, community, and population levels. Such research can connect the OMBA to real-world outcomes, revealing specific patterns occurring in the microbiome in responses to changing environments and identify populations that may be more vulnerable to NPDs. Including multidisciplinary approaches that address the OMBA may also lead to better identification, prevention, and treatment of NPDs. By acknowledging and addressing the OMBA and the sociocultural and behavioral factors that shape oral microbiota, we can create solutions and implement policies that better target and prevent oral disease and NPDs.
Author Contributions
GB researched and wrote the review. LW edited the manuscript. Both authors contributed to the article and approved the submitted version.
Conflict of Interest
The authors declare that the research was conducted in the absence of any commercial or financial relationships that could be construed as a potential conflict of interest.
Publisher’s Note
All claims expressed in this article are solely those of the authors and do not necessarily represent those of their affiliated organizations, or those of the publisher, the editors and the reviewers. Any product that may be evaluated in this article, or claim that may be made by its manufacturer, is not guaranteed or endorsed by the publisher.
References
1. Liang S, Wu X, Jin F. Gut-brain psychology: rethinking psychology from the microbiota–gut–brain axis. Front Integr Neurosci. (2018) 12:33. doi: 10.3389/fnint.2018.00033
2. Stilling RM, Dinan TG, Cryan JF. Microbial genes, brain & behaviour – epigenetic regulation of the gut–brain axis. Genes Brain Behav. (2014) 13:69–86. doi: 10.1111/gbb.12109
3. Lam YY, Ha CWY, Hoffmann JMA, Oscarsson J, Dinudom A, Mather TJ, et al. Effects of dietary fat profile on gut permeability and microbiota and their relationships with metabolic changes in mice. Obesity (Silver Spring). (2015) 23:1429–39. doi: 10.1002/oby.21122
4. Benahmed AG, Gasmi A, Doşa A, Chirumbolo S, Mujawdiya PK, Aaseth J, et al. Association between the gut and oral microbiome with obesity. Anaerobe. (2020) 70:102248. doi: 10.1016/j.anaerobe.2020.102248
5. Grice EA, Segre JA. The human microbiome: our second genome. Annu Rev Genom Hum Genet. (2012) 13:151–70. doi: 10.1146/annurev-genom-090711-163814
6. Carabotti M, Scirocco A, Maselli MA, Severi C. The gut-brain axis: interactions between enteric microbiota, central and enteric nervous systems. Ann Gastroenterol. (2015) 28:203–9.
7. Arneth BM. Gut-brain axis biochemical signalling from the gastrointestinal tract to the central nervous system: gut dysbiosis and altered brain function. Postgrad Med J. (2018) 94:446–52. doi: 10.1136/postgradmedj-2017-135424
8. Arneth BM. Gut–brain axis biochemical signalling from the gastrointestinal tract to the central nervous system: gut dysbiosis and altered brain function. Postgrad Med J. (2018) 94:446–52. doi: 10.1136/postgradmedj-2017-135424
9. Lyte M. Microbial endocrinology in the microbiome-gut-brain axis: how bacterial production and utilization of neurochemicals influence behavior. PLoS Pathog. (2013) 9:e1003726. doi: 10.1371/journal.ppat.1003726
10. Logan AC, Jacka FN. Nutritional psychiatry research: an emerging discipline and its intersection with global urbanization, environmental challenges and the evolutionary mismatch. J Physiol Anthropol. (2014) 33:22. doi: 10.1186/1880-6805-33-22
11. Neuman H, Debelius JW, Knight R, Koren O. Microbial endocrinology: the interplay between the microbiota and the endocrine system. FEMS Microbiol Rev. (2015) 39:509–21. doi: 10.1093/femsre/fuu010
12. Fuqua WC, Winans SC, Greenberg EP. Quorum sensing in bacteria: the LuxR-LuxI family of cell density-responsive transcriptional regulators. J Bacteriol. (1994) 176):269–75. doi: 10.1128/jb.176.2.269-275.1994
13. Karavolos MH, Winzer K, Williams P, Ajam Kahn CM. Pathogen espionage: multiple bacterial adrenergic sensors eavesdrop on host communication systems. Mol Microbiol. (2013) 87:455–65. doi: 10.1111/mmi.12110
14. Iyer LM, Aravind L, Coon SL, Klein DC, Koonin EV. Evolution of cell–cell signaling in animals: did late horizontal gene transfer from bacteria have a role? Trends Genet. (2004) 20:292–9. doi: 10.1016/j.tig.2004.05.007
15. Daniel H, Gholami AM, Berry D, Desmarchelier C, Hahne H, Loh G, et al. High-fat diet alters gut microbiota physiology in mice. ISME J. (2014) 8:295–308. doi: 10.1038/ismej.2013.155
16. Lloyd-Price J, Abu-Ali G, Huttenhower C. The healthy human microbiome. Genome Med. (2016) 8):51. doi: 10.1186/s13073-016-0307-y
17. Dominguez-Bello MG, Godoy-Vitorino F, Knight R, Blaser MJ. Role of the microbiome in human development. Gut. (2019) 68:1108–14. doi: 10.1136/gutjnl-2018-317503
18. Gur TL, Worly BL, Bailey MT. Stress and the commensal microbiota: importance in parturition and infant neurodevelopment. Front Psychiatry. (2015) 6:5. doi: 10.3389/fpsyt.2015.00005
19. Mudd AT, Berding K, Wang M, Donovan SM, Dilger RN. Serum cortisol mediates the relationship between fecal Ruminococcus and brain N-acetylaspartate in the young pig. Gut Microbes. (2017) 8:589–600. doi: 10.1080/19490976.2017.1353849
20. Lyte M. Microbial endocrinology in the microbiome-gut-brain axis: how bacterial production and utilization of neurochemicals influence behavior. PLOS Pathog. (2013) 9:e1003726. doi: 10.1371/journal.ppat.1003726
21. Shafquat A, Joice R, Simmons SL, Huttenhower C. Functional and phylogenetic assembly of microbial communities in the human microbiome. Trends Microbiol. (2014) 22:261–6. doi: 10.1016/j.tim.2014.01.011
22. Baker JL, Bor B, Agnello M, Shi W, He X. Ecology of the oral microbiome: beyond bacteria. Trends Microbiol. (2017) 25:362–74. doi: 10.1016/j.tim.2016.12.012
23. Siqueira JF, Rôças IN. The oral microbiota: general overview, taxonomy, and nucleic acid techniques. In: GJ Seymour, MP Cullinan, NCK Heng editors. Oral Biology: Molecular Techniques and Applications. Totowa, NJ: Humana Press (2010). p. 55–69. doi: 10.1007/978-1-60761-820-1_5
24. Knight R, Callewaert C, Marotz C, Hyde ER, Debelius JW, McDonald D, et al. The microbiome and human biology. Annu Rev Genom Hum Genet. (2017) 18:65–86. doi: 10.1146/annurev-genom-083115-022438
25. Xue L, Zou X, Yang X-Q, Peng F, Yu D-K, Du J-R. Chronic periodontitis induces microbiota-gut-brain axis disorders and cognitive impairment in mice. Exp Neurol. (2020) 326:113176. doi: 10.1016/j.expneurol.2020.113176
26. Lin D, Hutchison KE, Portillo S, Vegara V, Ellingson JM, Liu J, et al. Association between the oral microbiome and brain resting state connectivity in smokers. Neuroimage. (2019) 200:121–31. doi: 10.1016/j.neuroimage.2019.06.023
27. Yang I, Arthur RA, Zhao L, Clark J, Hu Y, Corwin EJ, et al. The oral microbiome and inflammation in mild cognitive impairment. Exp Gerontol. (2021) 147:111273. doi: 10.1016/j.exger.2021.111273
28. Cunha FA, Cota LOM, Cortelli SC, Miranda TB, Neves FS, Cortelli JR, et al. Periodontal condition and levels of bacteria associated with periodontitis in individuals with bipolar affective disorders: a case-control study. J Periodontal Res. (2019) 54:63–72. doi: 10.1111/jre.12605
29. Deo PN, Deshmukh R. Oral microbiome: unveiling the fundamentals. J Oral Maxillofac Pathol. (2019) 23:122–8. doi: 10.4103/jomfp.JOMFP_304_18
30. Duran-Pinedo AE, Solbiati J, Frias-Lopez J. The effect of the stress hormone cortisol on the metatranscriptome of the oral microbiome. NPJ Biofilms Microbiomes. (2018) 4:25. doi: 10.1038/s41522-018-0068-z
31. Li X, Kolltveit KM, Tronstad L, Olsen I. Systemic diseases caused by oral infection. Clin Microbiol Rev. (2000) 13:547–58. doi: 10.1128/CMR.13.4.547
32. Contaldo M, Itro A, Lajolo C, Gioco G, Inchingolo F, Serpico R. Overview on osteoporosis, periodontitis and oral dysbiosis: the emerging role of oral microbiota. Appl Sci. (2020) 10:6000. doi: 10.3390/app10176000
33. Vieira AT, Castelo PM, Ribeiro DA, Ferreira CM. Influence of oral and gut microbiota in the health of menopausal women. Front Microbiol. (2017) 8:1884. doi: 10.3389/fmicb.2017.01884
34. Do LG, Ha DH, Bell LK, Devenish G, Golley RK, Leary SD, et al. Study of mothers’ and infants’ life events affecting oral health (SMILE) birth cohort study: cohort profile. BMJ Open. (2020) 10:e041185. doi: 10.1136/bmjopen-2020-041185
35. Craig SJC, Blankenberg D, Parodi ACL, Paul IM, Birch LL, Savage JS, et al. Child weight gain trajectories linked to oral microbiota composition. Sci Rep. (2018) 8:14030. doi: 10.1038/s41598-018-31866-9
36. Jensen ED, Selway CA, Allen G, Bednarz J, Weyrich LS, Gue S, et al. Early markers of periodontal disease and altered oral microbiota are associated with glycemic control in children with type 1 diabetes. Pediatr Diabetes. (2021) 22:474–81. doi: 10.1111/pedi.13170
37. Willis JR, Gabaldón T. The human oral microbiome in health and disease: from sequences to ecosystems. Microorganisms. (2020) 8:308. doi: 10.3390/microorganisms8020308
38. Xiao J, Fiscella KA, Gill SR. Oral microbiome: possible harbinger for children’s health. Int J Oral Sci. (2020) 12:12. doi: 10.1038/s41368-020-0082-x
39. Bulgart HR, Neczypor EW, Wold LE, Mackos AR. Microbial involvement in Alzheimer disease development and progression. Mol Neurodegen. (2020) 15:42. doi: 10.1186/s13024-020-00378-4
40. Watanabe I, Kuriyama N, Miyatani F, Nomura R, Naka S, Nakano K, et al. Oral Cnm—positive Streptococcus mutans expressing collagen binding activity is a risk factor for cerebral microbleeds and cognitive impairment. Sci Rep. (2016) 6:38561. doi: 10.1038/srep38561
41. Lassalle F, Spagnoletti M, Fumagalli M, Shaw L, Dyble M, Walker C, et al. Oral microbiomes from hunter-gatherers and traditional farmers reveal shifts in commensal balance and pathogen load linked to diet. Mol Ecol. (2018) 27:182–95. doi: 10.1111/mec.14435
42. Dominy SS, Lynch C, Ermini F, Benedyk M, Marczyk A, Konradi A, et al. Porphyromonas gingivalis in Alzheimer’s disease brains: evidence for disease causation and treatment with small-molecule inhibitors. Sci Adv. (2019) 5:eaau3333. doi: 10.1126/sciadv.aau3333
43. Xia M-X, Ding X, Qi J, Gu J, Hu G, Sun X-L. Inhaled budesonide protects against chronic asthma-induced neuroinflammation in mouse brain. J Neuroimmunol. (2014) 273:53–7. doi: 10.1016/j.jneuroim.2014.06.005
45. Ding Y, Ren J, Yu H, Yu W, Zhou Y. Porphyromonas gingivalis, a periodontitis causing bacterium, induces memory impairment and age-dependent neuroinflammation in mice. Immun Ageing (2018) 15:6. doi: 10.1186/s12979-017-0110-7
46. Karr JE, Graham RB, Hofer SM, Muniz-Terrera G. When does cognitive decline begin? A systematic review of change point studies on accelerated decline in cognitive and neurological outcomes preceding mild cognitive impairment, dementia, and death. Psychol Aging. (2018) 33:195–218. doi: 10.1037/pag0000236
47. Papageorgiou SN, Hagner M, Nogueira AVB, Franke A, Jäger A, Deschner J. Inflammatory bowel disease and oral health: systematic review and a meta-analysis. J Clin Periodontol. (2017) 44:382–93. doi: 10.1111/jcpe.12698
48. Bernstein CN, Hitchon CA, Walld R, Bolton JM, Sareen J, Walker JR, et al. Increased burden of psychiatric disorders in inflammatory bowel disease. Inflamm Bowel Dis. (2019) 25:360–8. doi: 10.1093/ibd/izy235
49. van den Heuvel MP, Hulshoff Pol HE. Exploring the brain network: a review on resting-state fMRI functional connectivity. Eur Neuropsychopharmacol. (2010) 20:519–34. doi: 10.1016/j.euroneuro.2010.03.008
50. Olsen I, Hicks SD. Oral microbiota and autism spectrum disorder (ASD). J Oral Microbiol. (2019) 12:1702806. doi: 10.1080/20002297.2019.1702806
51. Bonaz B, Bazin T, Pellissier S. The vagus nerve at the interface of the microbiota-gut-brain axis. Front Neurosci. (2018) 12:49. doi: 10.3389/fnins.2018.00049
52. Goellner E, Rocha CE. Anatomy of trigeminal neuromodulation targets: from periphery to the brain. Prog Neurol Surg. (2020) 35:18–34. doi: 10.1159/000511257
53. Tsigos C, Chrousos GP. Hypothalamic-pituitary-adrenal axis, neuroendocrine factors and stress. J Psychosom Res. (2002) 53:865–71. doi: 10.1016/s0022-3999(02)00429-4
54. Roberts A, Matthews JB, Socransky SS, Freestone PPE, Williams PH, Chapple ILC. Stress and the periodontal diseases: effects of catecholamines on the growth of periodontal bacteria in vitro. Oral Microbiol Immunol. (2002) 17:296–303. doi: 10.1034/j.1399-302X.2002.170506.x
55. Akcalı A, Huck O, Tenenbaum H, Davideau J-L, Buduneli N. Periodontal diseases and stress: a brief review. J Oral Rehabil. (2012) 40:60–8. doi: 10.1111/j.1365-2842.2012.02341.x
56. Jentsch HFR, März D, Krüger M. The effects of stress hormones on growth of selected periodontitis related bacteria. Anaerobe. (2013) 24:49–54. doi: 10.1016/j.anaerobe.2013.09.001
57. García-Peña C, Álvarez-Cisneros T, Quiroz-Baez R, Friedland RP. Microbiota and aging. A review and commentary. Arch Med Res. (2017) 48:681–9. doi: 10.1016/j.arcmed.2017.11.005
58. Selway CA, Mills JG, Weinstein P, Skelly C, Yadav S, Lowe A, et al. Transfer of environmental microbes to the skin and respiratory tract of humans after urban green space exposure. Environ Int. (2020) 145:106084. doi: 10.1016/j.envint.2020.106084
59. Cutler C, Kiernan M, Willis JR, Gallardo-Alfaro L, Casas-Agustench P, White D, et al. Post-exercise hypotension and skeletal muscle oxygenation is regulated by nitrate-reducing activity of oral bacteria. Free Rad Biol Med. (2019) 143:252–59. doi: 10.1016/j.freeradbiomed.2019.07.035
60. Li J, Quinque D, Horz H-P, Li M, Rzhetskaya M, Raff JA, et al. Comparative analysis of the human saliva microbiome from different climate zones: Alaska, Germany, and Africa. BMC Microbiol. (2014) 14:316. doi: 10.1186/s12866-014-0316-1
61. Nath S, Zilm P, Jamieson L, Kapellas K, Goswami N, Ketagoda K, et al. Development and characterization of an oral microbiome transplant among Australians for the treatment of dental caries and periodontal disease: a study protocol. PLoS One. (2021) 16:e0260433. doi: 10.1371/journal.pone.0260433
62. Henrich J, Heine SJ, Norenzayan A. The weirdest people in the world? Behav Brain Sci. (2010) 33:61–83. doi: 10.1017/S0140525X0999152X
63. Kohrt BA, Mendenhall E, Brown PJ. How anthropological theory and methods can advance global mental health. Lancet Psychiatry. (2016) 3:396–8. doi: 10.1016/S2215-0366(16)00046-8
64. Kaiser BN, Kohrt BA. Why psychiatry needs the anthropologist: a reflection on 80 years of culture in mental health. Psychiatry. (2019) 82:205–15. doi: 10.1080/00332747.2019.1653142
65. Lozupone CA, Stombaugh JI, Gordon JI, Jansson JK, Knight R. Diversity, stability and resilience of the human gut microbiota. Nature. (2012) 489:220–30. doi: 10.1038/nature11550
66. Agwu E, Ihongbe JC, Ezeonwumelu JO, Lodhi MM. Baseline burden and antimicrobial susceptibility of pathogenic bacteria recovered from oral lesions of patients with HIV/AIDS in South-Western Uganda. Oral Sci Int. (2015) 12:59–66. doi: 10.1016/S1348-8643(15)00018-X
67. Abdul-Aziz M, Barreiro L, Perry G, Weyrich L. (2022). Chapter 4: Industrialisation Dramatically Impacts Oral Microbiota on a Multi-continental Scale [PhD, University of Adelaide]. Available online at: https://www.google.com/search?q=Industrialisation+dramatically+impacts+oral+microbiota+on+a+multi-continental+scale&oq=Industrialisation+dramatically+impacts+oral+microbiota+on+a+multi-continental+scale&aqs=chrome..69i57.421j0j4&sourceid=chrome&ie=UTF-8
68. Ozga AT, Sankaranarayanan K, Tito RY, Obregon-Tito AJ, Foster MW, Tallbull G, et al. Oral microbiome diversity among Cheyenne and Arapaho individuals from Oklahoma. Am J Phys Anthropol. (2016) 161:321–7. doi: 10.1002/ajpa.23033
69. Handsley-Davis M., Jamieson L., Kapellas K., Hedges J., Weyrich L. S. (2020). The role of the oral microbiota in chronic non-communicable disease and its relevance to the Indigenous health gap in Australia. BMC Oral Health 20:327. doi: 10.1186/s12903-020-01308-y
70. Kort R, Caspers M, van de Graaf A, van Egmond W, Keijser B, Roeselers G. Shaping the oral microbiota through intimate kissing. Microbiome. (2014) 2:41. doi: 10.1186/2049-2618-2-41
71. Monteiro MF, Altabtbaei K, Kumar PS, Casati MZ, Ruiz KGS, Sallum EA, et al. Parents with periodontitis impact the subgingival colonization of their offspring. Sci Rep. (2021) 11:1357. doi: 10.1038/s41598-020-80372-4
72. Raju SC, Lagström S, Ellonen P, de Vos WM, Eriksson JG, Weiderpass E, et al. Gender-specific associations between saliva microbiota and body size. Front Microbiol. (2019) 10:767. doi: 10.3389/fmicb.2019.00767
73. Zhao J, Zhou Y-H, Zhao Y-Q, Feng Y, Yan F, Gao Z-R, et al. Gender variations in the oral microbiomes of elderly patients with initial periodontitis. J Immunol Res. (2021) 2021:7403042. doi: 10.1155/2021/7403042
74. Biskup E, Martinkova J, Ferretti MT. Chapter 27 – Gender medicine: towards a gender-specific treatment of neuropsychiatric disorders. In: R Lanzenberger, GS Kranz, I Savic editors. Handbook of Clinical Neurology. (Vol. 175), Amsterdam: Elsevier (2020). p. 437–48. doi: 10.1016/B978-0-444-64123-6.00029-1
76. Skelly E, Kapellas K, Cooper A, Weyrich LS. Consequences of colonialism: a microbial perspective to contemporary indigenous health. Am J Phys Anthropol. (2018) 167:423–37. doi: 10.1002/ajpa.23637
77. Schuch HS, Haag DG, Kapellas K, Arantes R, Peres MA, Thomson WM, et al. The magnitude of Indigenous and non-Indigenous oral health inequalities in Brazil, New Zealand and Australia. Community Dent Oral Epidemiol. (2017) 45:434–41. doi: 10.1111/cdoe.12307
78. Parker EJ, Jamieson LM, Broughton J, Albino J, Lawrence HP, Roberts-Thomson K. The oral health of Indigenous children: a review of four nations. J Paediatr Child Health. (2010) 46:483–6. doi: 10.1111/j.1440-1754.2010.01847.x
79. Duran-Pinedo AE, Solbiati J, Frias-Lopez J. (2018). The effect of the stress hormone cortisol on the metatranscriptome of the oral microbiome. NPJ Biofilms Microbiomes 4:25. doi: 10.1038/s41522-018-0068-z
80. Sanz M, Beighton D, Curtis MA, Cury JA, Dige I, Dommisch H, et al. Role of microbial biofilms in the maintenance of oral health and in the development of dental caries and periodontal diseases. Consensus report of group 1 of the Joint EFP/ORCA workshop on the boundaries between caries and periodontal disease. J Clin Periodontol. (2017) 44:S5–11. doi: 10.1111/jcpe.12682
82. Meurman JH. Probiotics: do they have a role in oral medicine and dentistry? Eur J Oral Sci. (2005) 113:188–96. doi: 10.1111/j.1600-0722.2005.00191.x
83. Allaker RP, Stephen AS. Use of probiotics and oral health. Curr Oral Health Rep. (2017) 4:309–18. doi: 10.1007/s40496-017-0159-6
84. Burke KE, Lamont JT. Fecal transplantation for recurrent clostridium difficile infection in older adults: a review. J Am Geriatr Soc. (2013) 61:1394–8. doi: 10.1111/jgs.12378
85. Nascimento MM. Oral microbiota transplant: a potential new therapy for oral diseases. J Calif Dent Assoc. (2017) 45:565–8.
86. Haraszthy VI, Raylae CC, Sreenivasan PK. Antimicrobial effects of a stannous fluoride toothpaste in distinct oral microenvironments. J Am Dental Assoc. (2019) 150:S14–24. doi: 10.1016/j.adaj.2019.01.007
87. Al-Mutairi AS, Edris S, Sabbagh H, Al-Quwaie D, Alsubhi NH, Abulfaraj A, et al. Alteration of oral microbiome in children after using miswak (Salvadora persica l.) made from arak as a natural toothpaste. Appl Ecol Environ Res. (2020) 18:7089–138. doi: 10.15666/aeer/1805_70897138
88. Hall-Stoodley L, Stoodley P. Developmental regulation of microbial biofilms. Curr Opin Biotechnol. (2002) 13:228–33. doi: 10.1016/S0958-1669(02)00318-X
89. Berger D, Rakhamimova A, Pollack A, Loewy Z. Oral biofilms: development, control, and analysis. High Throughput. (2018) 7:24. doi: 10.3390/ht7030024
90. Handsley-Davis M, Kapellas KM, Jamieson L, Hedges J, Skelly E, Kaidonis J, et al. Heritage-specific oral microbiota in Indigenous Australian dental calculus. Evol Med Public Health (2021) Submitted for review.
91. Mills JG, Weinstein P, Gellie NJC, Weyrich LS, Lowe AJ, Breed MF. Urban habitat restoration provides a human health benefit through microbiome rewilding: the microbiome rewilding hypothesis. Restor Ecol. (2017) 25:866–72. doi: 10.1111/rec.12610
92. Reber SO, Siebler PH, Donner NC, Morton JT, Smith DG, Kopelman JM, et al. Immunization with a heat-killed preparation of the environmental bacterium Mycobacterium vaccae promotes stress resilience in mice. Proc Natl Acad Sci USA. (2016) 113:E3130–9. doi: 10.1073/pnas.1600324113
93. Liddicoat C, Sydnor H, Cando-Dumancela C, Dresken R, Liu J, Gellie NJC, et al. Naturally-diverse airborne environmental microbial exposures modulate the gut microbiome and may provide anxiolytic benefits in mice. Sci Total Environ. (2020) 701:134684. doi: 10.1016/j.scitotenv.2019.134684
Keywords: oral microbiota, oral microbiome, dentistry, psychiatric disease, mental health
Citation: Bowland GB and Weyrich LS (2022) The Oral-Microbiome-Brain Axis and Neuropsychiatric Disorders: An Anthropological Perspective. Front. Psychiatry 13:810008. doi: 10.3389/fpsyt.2022.810008
Received: 05 November 2021; Accepted: 14 February 2022;
Published: 30 March 2022.
Edited by:
Teodor T. Postolache, University of Maryland, Baltimore, United StatesReviewed by:
Padhmanand Sudhakar, KU Leuven, BelgiumJasenka Zubcevic, University of Toledo, United States
Copyright © 2022 Bowland and Weyrich. This is an open-access article distributed under the terms of the Creative Commons Attribution License (CC BY). The use, distribution or reproduction in other forums is permitted, provided the original author(s) and the copyright owner(s) are credited and that the original publication in this journal is cited, in accordance with accepted academic practice. No use, distribution or reproduction is permitted which does not comply with these terms.
*Correspondence: Grace B. Bowland, Z2JiNTIxNkBwc3UuZWR1