- 1TMS Clinical and Research Program, Neuromodulation Division, Semel Institute for Neuroscience and Human Behavior at UCLA, Los Angeles, CA, United States
- 2Department of Psychiatry and Biobehavioral Sciences, David Geffen School of Medicine at UCLA, Los Angeles, CA, United States
- 3Department of Psychology, University of California, Los Angeles, Los Angeles, CA, United States
- 4David Geffen School of Medicine at UCLA, Los Angeles, CA, United States
- 5Division of the Humanities and Social Sciences, California Institute of Technology, Pasadena, CA, United States
Background: Specific phobias represent the largest category of anxiety disorders. Previous work demonstrated that stimulating the ventromedial prefrontal cortex (vmPFC) with repetitive Transcranial Magnetic Stimulation (rTMS) may improve response to exposure therapy for acrophobia.
Objective: To examine feasibility of accelerating extinction learning in subjects with spider phobia using intermittent Theta Burst Stimulation (iTBS) rTMS of vmPFC.
Methods: In total, 17 subjects with spider phobia determined by spider phobia questionnaires [Spider Phobia Questionnaire (SPQ) and Fear of Spiders questionnaire (FSQ)] underwent ratings of fear of spiders as well as behavioral and skin conductance data during a behavioral avoidance test (BAT). Subjects then received a sequential protocol of in vivo spider exposure followed by iTBS for three sessions administered to either active or control treatment sites (vmPFC [n = 8] or vertex [n = 9], respectively), followed 1 week later by repetition of questionnaires and BAT.
Results: All subjects improved significantly regardless of group across both questionnaires (FSQ η2 = 0.43, p = 0.004; SPQ η2 = 0.39, p = 0.008) and skin conductance levels during BAT (Wald χ2 = 30.9, p < 0.001). Subjects in the vmPFC group tolerated lower treatment intensity than in the control group, and there was a significant correlation between treatment intensity, BAT subjective distress improvement, and physiologic measures (all ρ > 0.5).
Conclusion: This proof-of-concept study provides preliminary evidence that a sequential exposure and iTBS over vmPFC is feasible and may have rTMS intensity-dependent effects on treatment outcomes, providing evidence for future areas of study in the use of rTMS for phobias.
Introduction
Specific phobias represent the largest category of anxiety disorders in the world with an estimated lifetime prevalence of 7.4–15% (1–3). The core diagnostic criterion is persistent fear, anxiety, or avoidance of a specific stimulus that results in a clinically significant impairment in daily functioning (3, 4).
Exposure therapy (ET) is currently considered the gold-standard treatment for specific phobia, with an estimated 75% of patients seeing clinical benefit compared with placebo (5) ET extinguishes conditional fear through repeated, unreinforced exposure to the feared stimulus repeatedly over time (6, 7). 25–30% of patients will not respond to ET, however, and many phobic patients avoid or refuse treatment or discontinue treatment before completion (4, 5, 8, 9).
Repetitive Transcranial Magnetic Stimulation (rTMS) is a novel treatment utilized for Major Depressive Disorder (MDD) (10–12), obsessive-compulsive disorder (OCD) (13, 14), substance dependence (15–17), and other neuropsychiatric disorders (18–20). rTMS uses low-intensity electromagnetic energy to stimulate critical hubs of brain networks and “reset” function (21). rTMS stimulation of a particular target is believed to alter connectivity between the target and other brain regions in the same network (22–25). Studies suggest that rTMS may be efficacious in the treatment of anxiety disorders (14, 26–29), consistent with evidence showing that stimulation of the medial prefrontal cortex modulates discrimination of learned safety and threat cues (30) and may ameliorate symptoms in specific phobia (26, 30–33).
The ventromedial prefrontal cortex (vmPFC) is a promising rTMS treatment target (34, 35) that is thought to play a central role in the etiology, maintenance, and treatment of anxiety disorders due to its functional and structural connectivity with subcortical regions involved in fear learning and recall, such as the amygdala (36–41) and hippocampus (41–44). One study found that individuals with acrophobia who received rTMS to the vmPFC along with ET demonstrated a greater improvement compared to those receiving ET and sham stimulation (35).
In this proof-of-concept pilot study, we aimed to examine the feasibility and potential benefit of using rTMS stimulation of vmPFC to augment the efficacy of ET for the treatment of spider phobia. Previous work examining the use of rTMS in spider phobia has examined the immediate effects of a single session of rhythmic rTMS (32, 33). We utilized multiple sessions of intermittent Theta-Burst Stimulation (iTBS, which has recently been shown to have efficacy similar to rhythmic rTMS while reducing treatment time by as much as 90%) and examined durability of effects 1 week after treatment (45–48). The protocol in this study is similar to later work in acrophobia that demonstrated positive results (35). We hypothesized that iTBS stimulation of vmPFC would be well tolerated in subjects with spider phobia and that those receiving iTBS vmPFC stimulation prior to a behavioral avoidance test (BAT) would exhibit greater willingness to approach a novel spider, lower self-reported distress during the BAT, and greater reductions in skin conductance level (SCL, a physiologic measure commonly used in studies of phobias, including spider phobia) (33, 49–51) compared to those receiving control stimulation. Additional exploratory analyses assessed the extent to which stimulation intensity to the vmPFC was associated with these same dependent variables.
Materials and Methods
Design
Twenty-two rTMS-naïve subjects meeting criteria for spider phobia were recruited and randomized 1:1 to treatment with iTBS stimulation of vmPFC (active) or Cz (control) following each of three repeated exposures to live spiders.
Subjects and research staff conducting the behavioral assessments and exposures were blinded to rTMS treatment condition. After initial screening, subjects completed online screening for eligibility and gave written informed consent in accordance with the Declaration of Helsinki (52). The ClinicalTrials.gov Identifier is NCT04019054. After informed consent, subjects completed baseline Spider Phobia Questionnaire (SPQ) and Fear of Spiders questionnaire (FSQ), and a behavioral avoidance test (BAT), followed by an exposure session and iTBS administered to either an active or control stimulation site based on group assignment (randomly assigned by non-rater study staff on enrollment prior to iTBS treatment). Two subsequent exposure plus iTBS treatments were administered at 24–48 h intervals (49, 51, 53, 54). At a final visit 1 week later, the SPQ and FSQ were repeated followed by an identical BAT to that administered at the initial visit. Both subjects and raters then completed a blinding questionnaire asking them to state whether they were assigned to active or control group. Subjects were debriefed by the principal investigator on completion of their participation, and all subjects completed participation within 2 weeks. Full timeline also outlined in Figure 1.
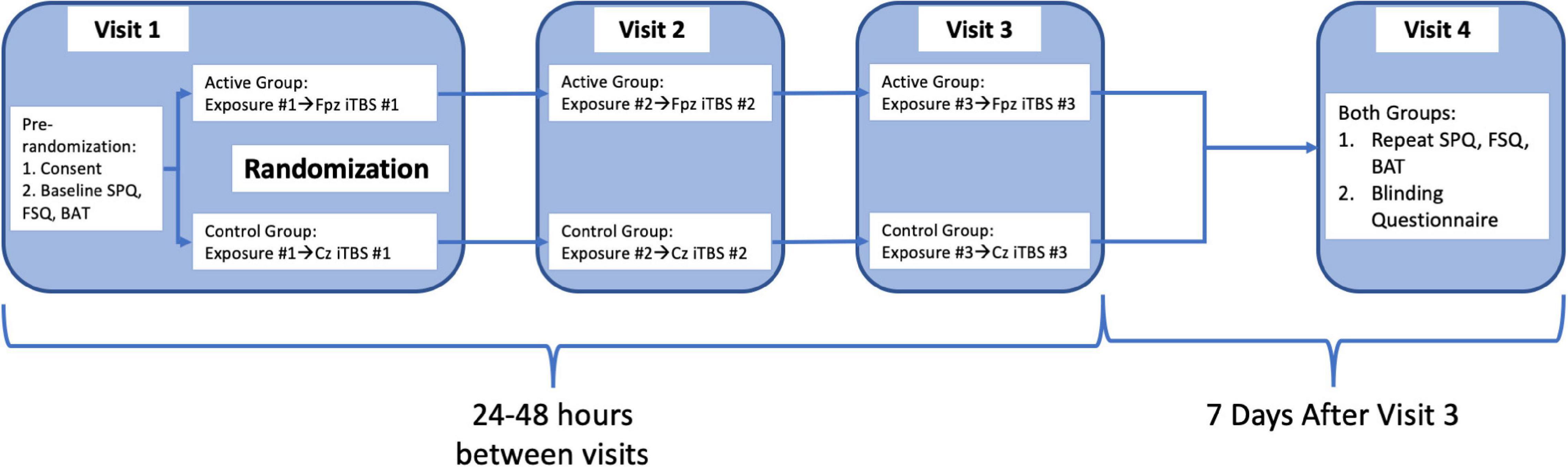
Figure 1. Graphical representation of procedural flow of study steps as detailed in the section “Procedures.”
Subjects
Inclusion criteria were: at least 18 years of age, English-speaking, and a pre-screening SPQ score of at least 18. Exclusion criteria were: inability to provide informed consent, presence of suicidal ideation, history of a diagnosed mood, psychotic, or anxiety disorder (other than specific phobia), active prescriptions for medications known to affect seizure threshold, elevated anxiety and depression screening tool scores (PHQ9 > 10, GAD7 > 10, HAMD > 8 or suicide item score >2), history of neuromodulation treatments for any condition (rTMS, direct current stimulation, electroconvulsive therapy, or vagus nerve stimulation), history of a condition affecting the central nervous system (stroke, seizure, neurocognitive disorders, and intracranial implants), current pregnancy, increased seizure risk due to medication or family history, and known bee or insect allergies.
Procedures
Behavioral Avoidance Testing
A behavioral avoidance testing (BAT) with nine standardized steps was utilized as previously described (administered by research staff blinded to subject group assignment) (49, 51). Prior to initiation of the BAT, baseline skin conductance level (SCL) was recorded for 2 min from two electrodes attached to the second and third fingers of the subjects’ non-dominant hand. SCL was continuously measured with BIOPAC MP150 hardware and AcqKnowledge version 4.2 software (BIOPAC Systems, Inc., Goleta, CA, United states). Subjects were exposed to one of two Chilean Rose Tarantulas (Grammostola rosea) throughout the BAT. Subjects were instructed to approach the spider as closely as possible according to a series of nine standardized sequential steps lasting 30 s each. The first step involved standing 5 feet away from the spider, and the last step involved the subject touching the spider’s back leg continuously with the tip of their index finger. When subjects failed to complete a step in the sequence (such as withdrawing during or before completion of a step), the BAT was terminated and the number of steps fully completed was recorded. Prior to the BAT, subjects rated their overall confidence and distress about completing all nine steps. During the BAT, they rated their confidence and anticipatory distress prior to each step, and maximum distress after each step, on a scale from 0 to 100.
In vivo Exposures
In vivo exposure was conducted with a different Chilean Rose Tarantula from the one used in the BAT immediately prior to each of the three iTBS treatments. Exposures consisted of 10 identical exposure trials (involving subjects hovering their ungloved hand 3 in. above the tarantula in its terrarium) of 30-s duration with a 30-s pause between trials, for a total task duration of 10 min.
Transcranial Magnetic Stimulation
All rTMS treatments were performed with either the Magstim Rapid 2 stimulator using a 70 mm butterfly coil (Magstim, Whitland, NSW, United Kingdom) or the Magventure MagPro R30 stimulator using a 75 mm butterfly coil (MagVenture, Farum, Denmark). Resting motor threshold (RMT) was determined for all subjects as the minimum stimulus intensity necessary to elicit a motor response in the right abductor pollicis brevis or first dorsal interosseus muscles for ≥50% of stimuli applied to the motor cortex (55). RMT, a stable intra-subject parameter, was performed before the repeated exposures on the first day to minimize time delay from repeated exposures to TMS (56–58). Each participant used only one coil type (i.e., their MT was measured on one device and they were treated with that device for all three sessions), and coil assignment was based on device availability in the UCLA TMS Clinical and Research Service. All subjects, regardless of group assignment, then were instructed to hold an ice pack on their forehead (over the vmPFC stimulation site) for 5–7 min before beginning rTMS. This was done primarily to minimize discomfort for the active group, though it was done for all subjects in order to maintain blinding for subjects and raters. To maintain blinding, raters did not observe rTMS treatments, and subjects were not informed of the difference between groups during their participation in the study.
Subjects then underwent the iTBS protocol. For the active group, the TMS coil was positioned over the subject’s vmPFC (as determined using position Fpz, or the nasion, of the international 10–20 EEG electrode system) as seen in Figure 2 using a modified version of the Beam F3 method (30, 31, 35, 59–61). Stimulation was delivered at 100% of the subject’s RMT (using a ramp-up protocol starting from 80% RMT and advancing as tolerated) in bursts of three pulses at a frequency of 50 Hz every 200 ms on top of a 5 Hz carrier wave. Pulse delivery occurred over 2 s and was repeated every 10 s, 20 times in succession, for a total of 600 pulses delivered in 192 s (31, 35, 45, 60).
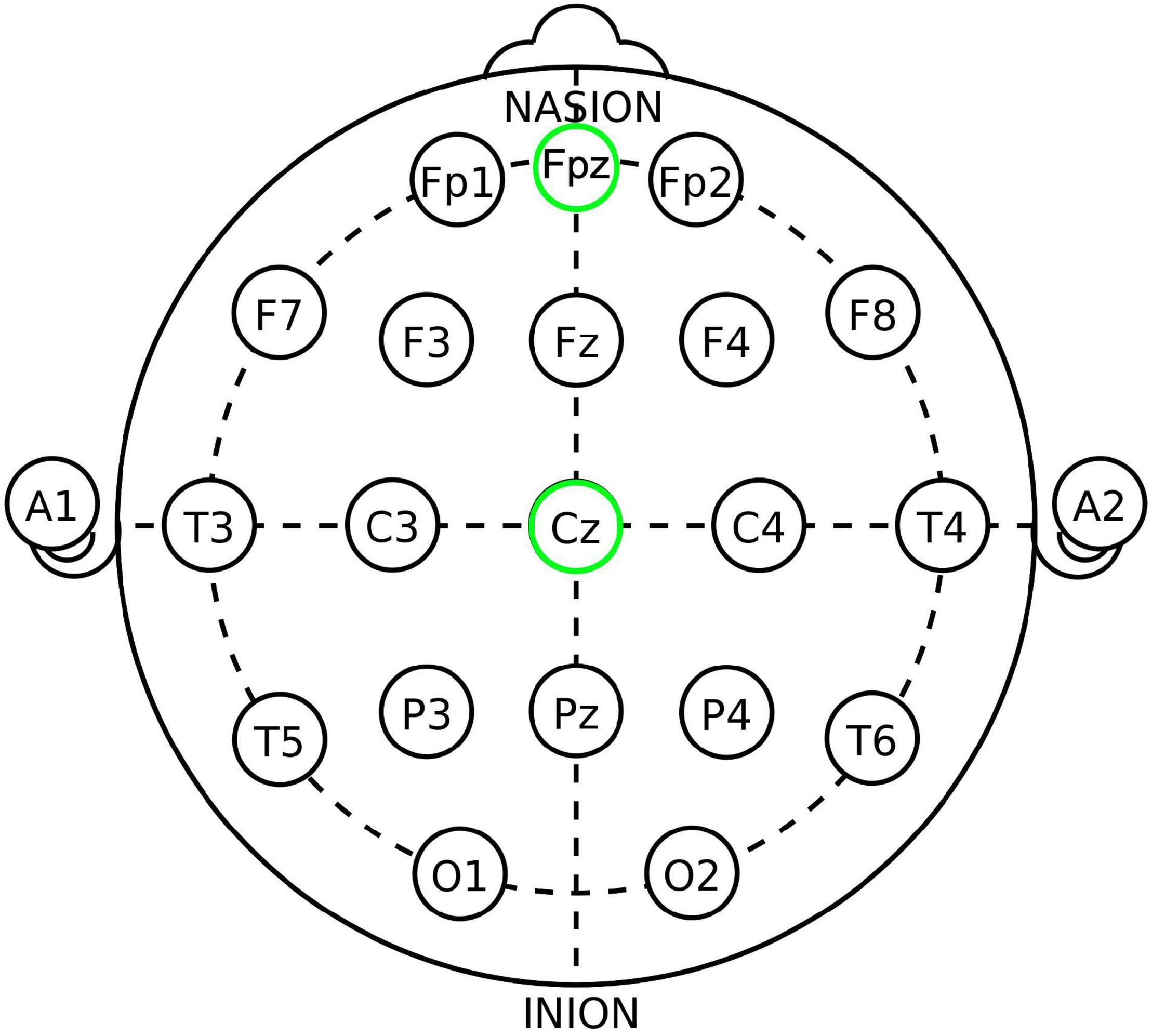
Figure 2. The 10–20 International system of EEG electrode placement, from Wikipedia.org “10–20 system (EEG)” and modified. Fpz (placement utilized in this study for active/vmPFC placement) and Cz (placement utilized for control/Cz placement) locations circled in green.
Intermittent theta burst stimulation in the control group consisted of the same stimulation parameters applied to the vertex (as determined using position Cz, of the International 10–20 EEG Electrode System) in place of vmPFC. The Cz placement was chosen for control because: (1) it is a commonly used control site in TMS studies and has a similar risk profile to other TMS sites (23, 62–67), (2) its position over the vertex minimizes the amount of cortex stimulated (67), (3) the stimulated area is not known to be associated with the circuitry examined in this experiment (32, 35, 39, 67, 68), and (4) it is generally associated with low rates of scalp discomfort and no clinically observed behavioral or mood effects (64, 66, 67, 69). The difference between the active and control groups was not disclosed to subjects until after completion of their participation.
Measures
The first outcome measures examined were fear of spiders as determined using both the 31-item spider phobia questionnaire (SPQ) and the 18-item fear of spiders questionnaire (FSQ) (53, 54).
During each BAT, skin conductance level (SCL) served as a physiological marker of fearful arousal. Three SCL values were calculated: (1) Baseline SCL, i.e., mean SCL during a 2-min period prior to initiation of each BAT, (2) Anticipatory SCL, i.e., mean SCL during a 1-min anticipation period after reading the subject a description of the BAT but prior to BAT initiation, and (3) mean SCL during each fully completed BAT step (51). Data were filtered using a finite impulse response (FIR) low pass filter with the frequency cutoff fixed at 2 Hz; no significant movement confounds emerged during data extraction.
In addition to SCL, confidence and distress rating were obtained using a visual analog rating scale ranging from 0 to 100 (0 = no distress/no confidence; 100 = severe distress/complete confidence). Anticipatory distress (AntD) and confidence ratings were obtained before beginning the BAT and prior to beginning each step, and maximum distress ratings (MaxD) were obtained after each step. The number of BAT steps fully completed (0–9) served as a behavioral measure of avoidance related to fear of spiders.
Variable experiment parameters recorded included post-rTMS pain scores as rated by a brief McGill questionnaire, minute delay from repeated exposures to initiation of rTMS treatment, maximum rTMS treatment intensity tolerated, and any early treatment terminations due to rTMS tolerability (70). Baseline demographic and psychiatric screening information was gathered but not included in statistical analysis.
Statistical Analysis
The number of statistical tests performed was minimized and multi-level mixed-effects models (MLMs) and non-parametric tests were utilized as appropriate based on the sample size and non-normal distributions of multiple outcome measures. Group assignment, timepoint (pre or post-treatment), and BAT step were examined in association with each measure acquired during the BATs (anticipatory distress, maximum distress, SCL at each step) using MLMs in Stata version 16. All other analyses were performed in SPSS (version 26.0.0.0). Demographics and experimental parameters were analyzed for between-group differences using 2-sided Mann–Whitney U Tests. Changes in self-reported fear of spiders (SPQ and FSQ scores) were analyzed using a repeated-measures MANOVA (Greenhouse Geisser correction was applied in case of non-sphericity). Chi-square analyses were performed to validate blinding of the study using the previously described blinding questionnaires. A p-value of <0.05 was considered statistically significant for all tests.
Results
Participants—Demographics, Baseline, and Experimental Parameters
In total, 85 subjects were screened and 22 enrolled in the study. Gender, age, and screening survey data are shown in Table 1. Two subjects withdrew (one from each group) after the first rTMS session for tolerability reasons as did one additional subject from the active group during the third rTMS session. One subject from the control group with a history of MDD and GAD was excluded by staff prior to completion of the study due to meeting exclusion criteria. One subject from the active group withdrew after the first rTMS session due to safety concerns related to COVID-19 in March of 2020. No subjects had active prescriptions for antidepressant or anxiolytic medications at the time of the study. Subject enrollment and completion is further shown in our CONSORT diagram (Figure 3). Test steps completed by participants in each group at pre- and post-treatment are shown in Table 2.
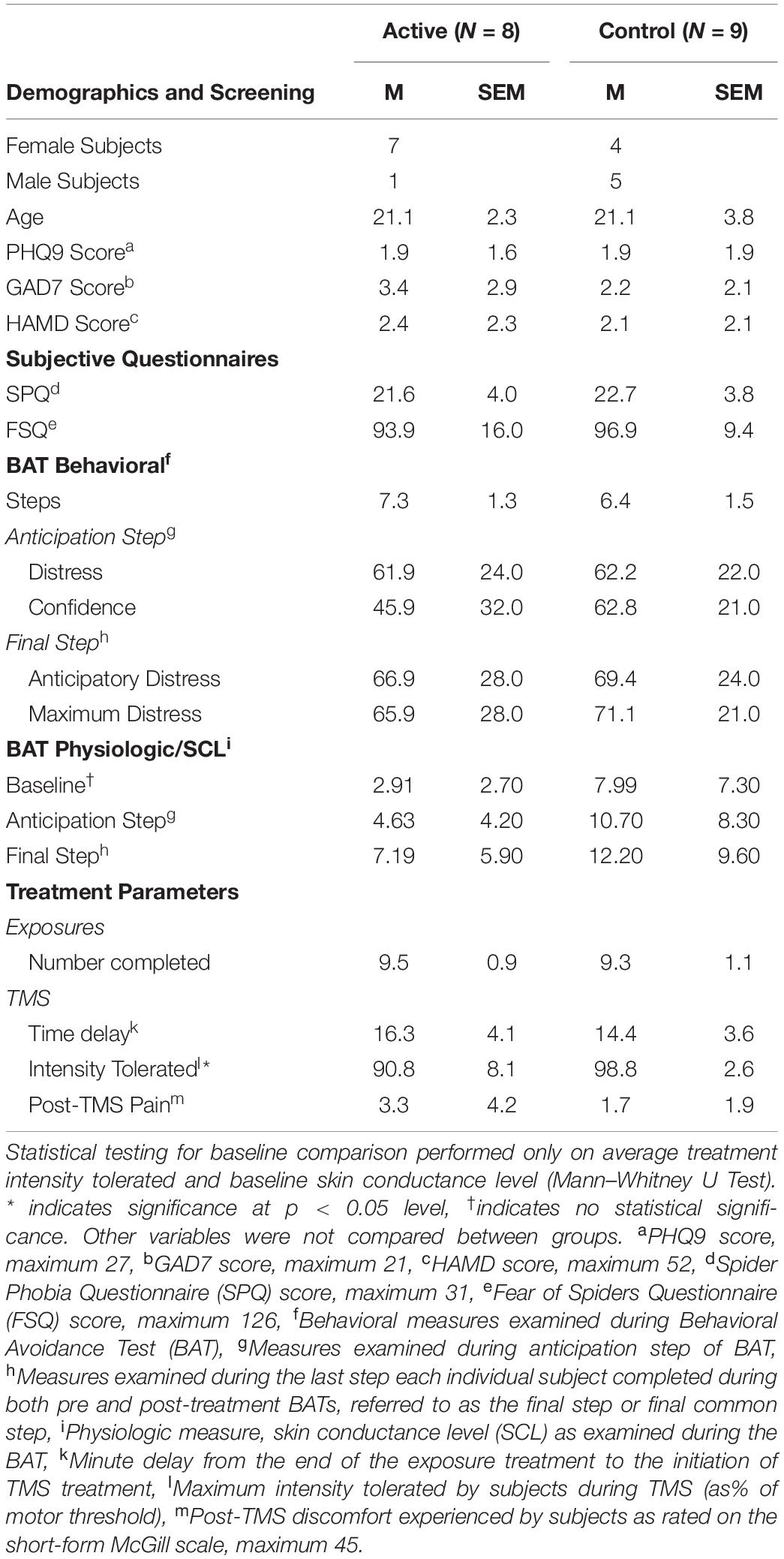
Table 1. Baseline demographic and screening data, as well as pre-treatment baseline measurement of outcome variables and experimental parameters.
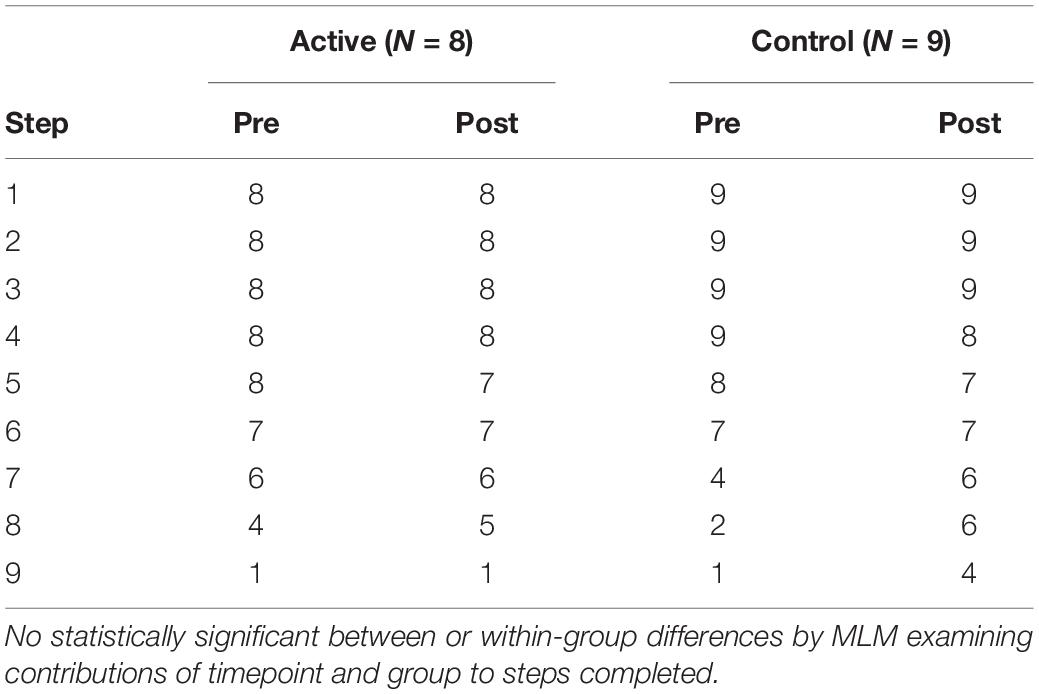
Table 2. Number of participants who completed each BAT step before and after treatment in each group.
The active treatment group tolerated significantly less intense iTBS stimulation than the control group (U = 13.5, p < 0.05) (Table 1). The majority of subjects in the control group tolerated 100% treatment intensity for the entire duration of treatment, whereas less than 40% of the active group was able to do so (Figure 4).
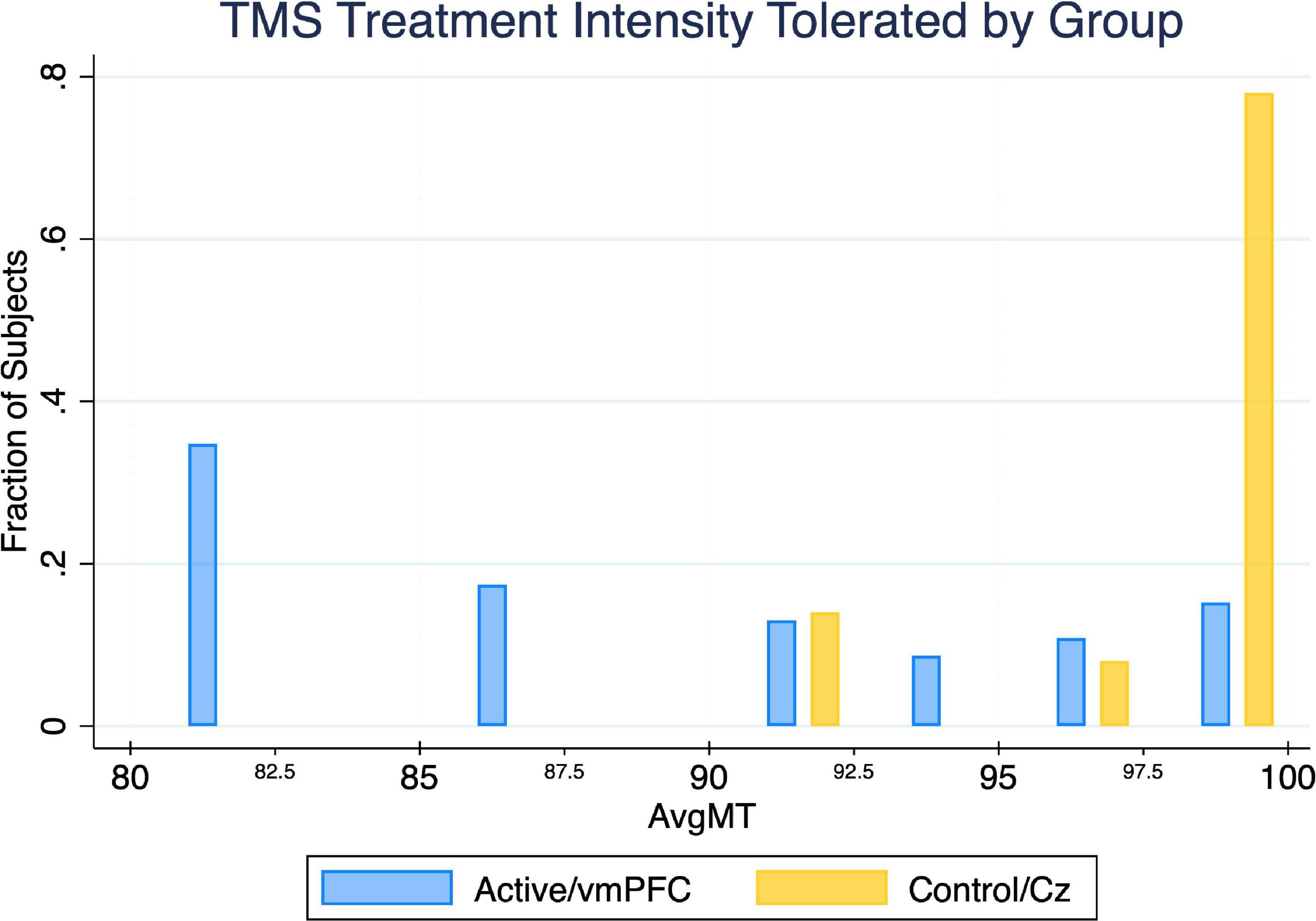
Figure 4. Between-group comparison of average TMS treatment intensity tolerated (noted as a percent of the resting motor threshold). Utilizing a 2-sided Mann–Whitney U Test, we found the maximum TMS intensity tolerated by the active treatment group is significantly lower (p = 0.027) than the control treatment group by an average of 8.0%.
Self-Reported Survey Outcomes
There were significant decreases in subjective distress as indicated by both the FSQ and SPQ for both groups over time with no significant effect of treatment group (FSQ η2 = 0.43, p = 0.004; SPQ η2 = 0.39, p = 0.008) (Figures 5A,B).
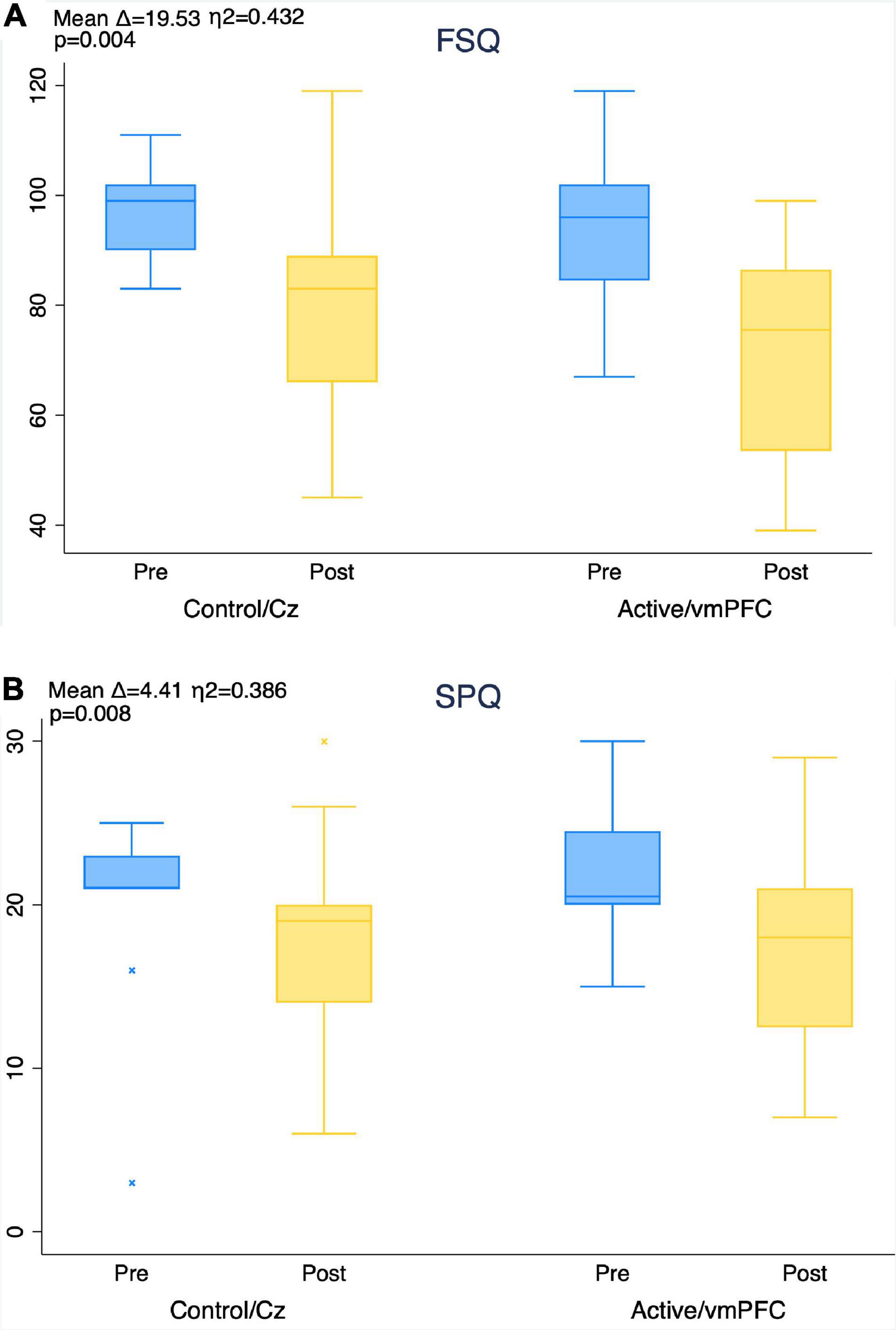
Figure 5. Improvement in fear of spiders questionnaire (FSQ, A) and spider phobia questionnaire (SPQ, B) scores from pre- to post-treatment by group. Mean score improvement (mean delta), MANOVA level of significance, and effect size (eta-squared) of aggregate subject pool provided for timepoint comparison in each figure. No between-group effects found using MANOVA.
Multi-Level Models, Physiological, and Observed Behavioral Outcomes
Three-way effect models showed significant effects for group, BAT step, and time (Wald χ2: χ2 SCL = 51.9, χ2 AntD = 167.8, χ2 MaxD = 105.3; all p < 0.001) with no significant interactions for any of the outcome measures (SCL: β = −0.002, SEM = 0.06, p = 0.97; AntD: β = −0.15, SEM = 0.13, p = 0.25; MaxD: β = −0.001, SEM = 0.14, p = 1.0).
Examining two-way group and timepoint effect models of SCL, we found the overall model to be significant (χ2 = 47.9, p < 0.001) in addition to a significant group-x-timepoint interaction (β = 0.50, SEM = 0.108, p < 0.01). Simple effects indicated this was due to group differences during the pre-treatment BAT, not due to differences with treatment, and therefore not further examined. Similarly, examining the two-way timepoint and step models, we found the overall model to be significant (χ2 = 30.90, p < 0.001) in addition to a significant timepoint-x-step interaction (β = 0.06, SEM = 0.027, p = 0.029). Mean SCL decreased with active treatment up to and including step 4 of the BAT (Figure 6 and Table 3). There appeared to be a crossover effect at step 7 (Figure 4), although examination of this possible effect is complicated by the diminished number of subjects who completed more than 6 steps before and after treatment.
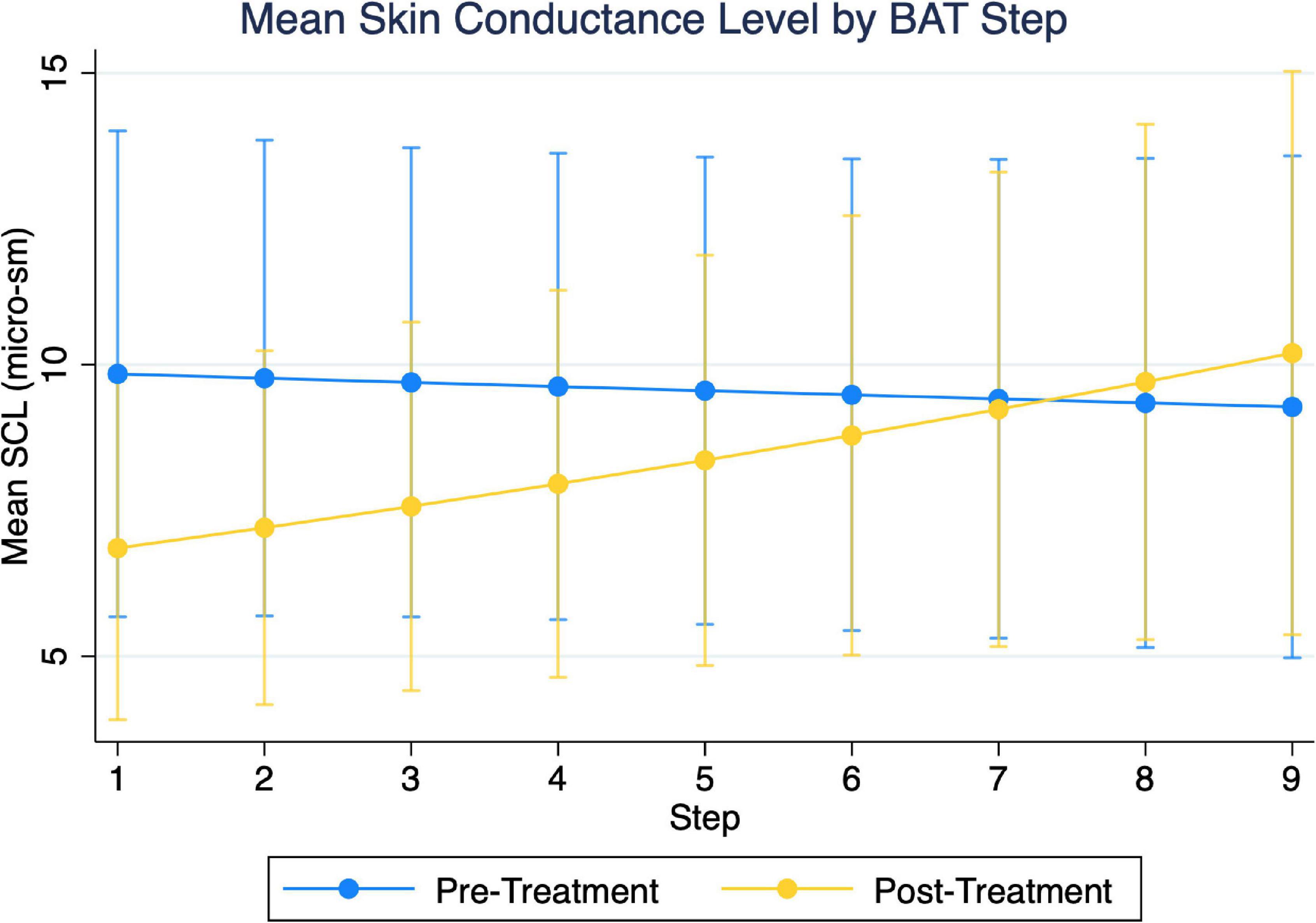
Figure 6. Depiction of two-variable multi-level models of skin conductance level (SCL), as a function of step and timepoint. Initial three-variable model including group were found not to have significant three-way interaction.
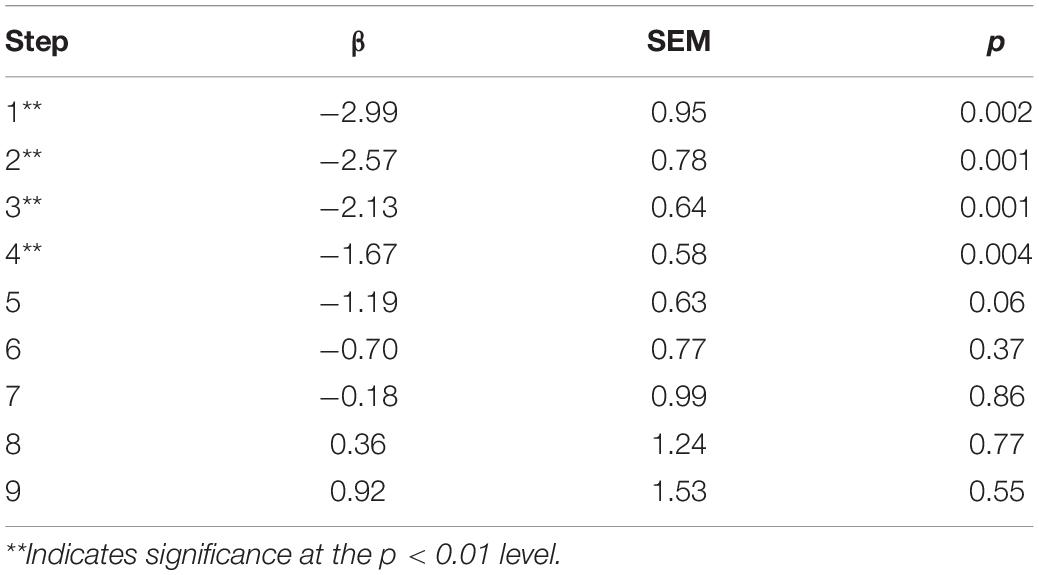
Table 3. Coefficient, standard error of the mean, and level of significance of timepoint difference for skin conductance multi-level model.
Examining AntD, we again found the overall model with regard to group and timepoint to be significant (χ2 = 158.7, p < 0.001), as well as a significant group-x-timepoint interaction (β = 1.27, SEM = 0.26, p < 0.001) as well as the model of group and step and their interaction effects (p < 0.001, χ2 = 121.8; β = −0.15, SEM = 0.075, p = 0.047). Simple effects again indicated these were due to group differences during the pre-treatment BAT, not due to differences with treatment, and therefore not further examined. We additionally found that the model of step and timepoint was significant (χ2 = 114.0, p < 0.001) with no significant step-x-timepoint interaction (β = −0.03, SEM = 0.06, p = 0.63).
For MaxD, the model of group and timepoint was significant (χ2 = 96.9, p < 0.001) with a significant group-x-timepoint interaction (β = 1.31, SEM = 0.30, p < 0.001). Similarly, the model of group and step and their interaction effects were found to be significant (χ2 = 78.44, p < 0.001; β = −0.19, SEM = 0.082, p = 0.024). Simple effects again indicated these were due to group differences during the pre-treatment BAT, not due to differences with treatment, and therefore not further examined.
Post-hoc Exploratory Analyses: Treatment Intensity Relationships
Given the significant differences in treatment intensity by group (Figure 4), a post-hoc analysis was performed to examine the within-group correlation between treatment intensity and (1) other experimental parameters, as well as (2) outcome measures of interest. There was a significant correlation between treatment intensity and length of delay (mean delay = 15.25 min, SD = 3.84 min) from the end of the exposures to the initiation of TMS on average (ρ = 0.75) and for the latter two of the three visits (Visit 1 ρ = 0.23, visit 2 ρ = 0.68, visit 3 ρ = 0.75) (Figure 7). Individuals who tolerated greater treatment intensity also experienced a greater decrease in subjective distress from pre- to post- treatment BATs (AntD ρ = 0.53, MaxD ρ = 0.60; Figure 8). A similar relationship was observed between treatment intensity and changes in mean SCL during the final BAT step each subject completed both before and after treatment (ρ = 0.76; Figure 9).
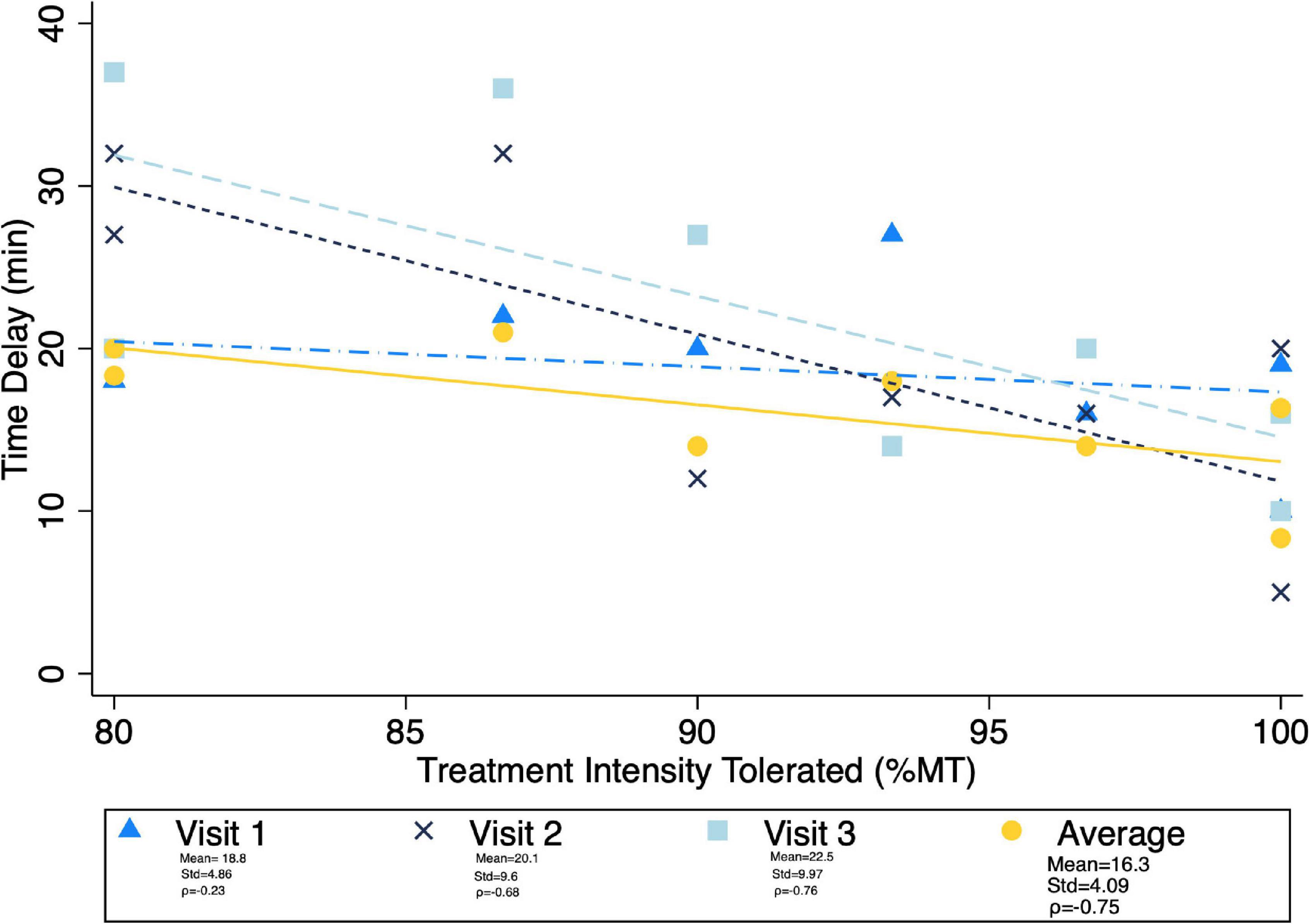
Figure 7. Correlation between average maximum treatment intensity tolerated (noted as a percent of resting motor threshold) and time delay from exposure to TMS. Mean time delay, standard deviation of the delay, and spearman correlation coefficient between treatment intensity and time delay noted for each visit. Pearson correlation was notably lowest at visit 1, and ρ > = 0.7 for all other visits and average delay.
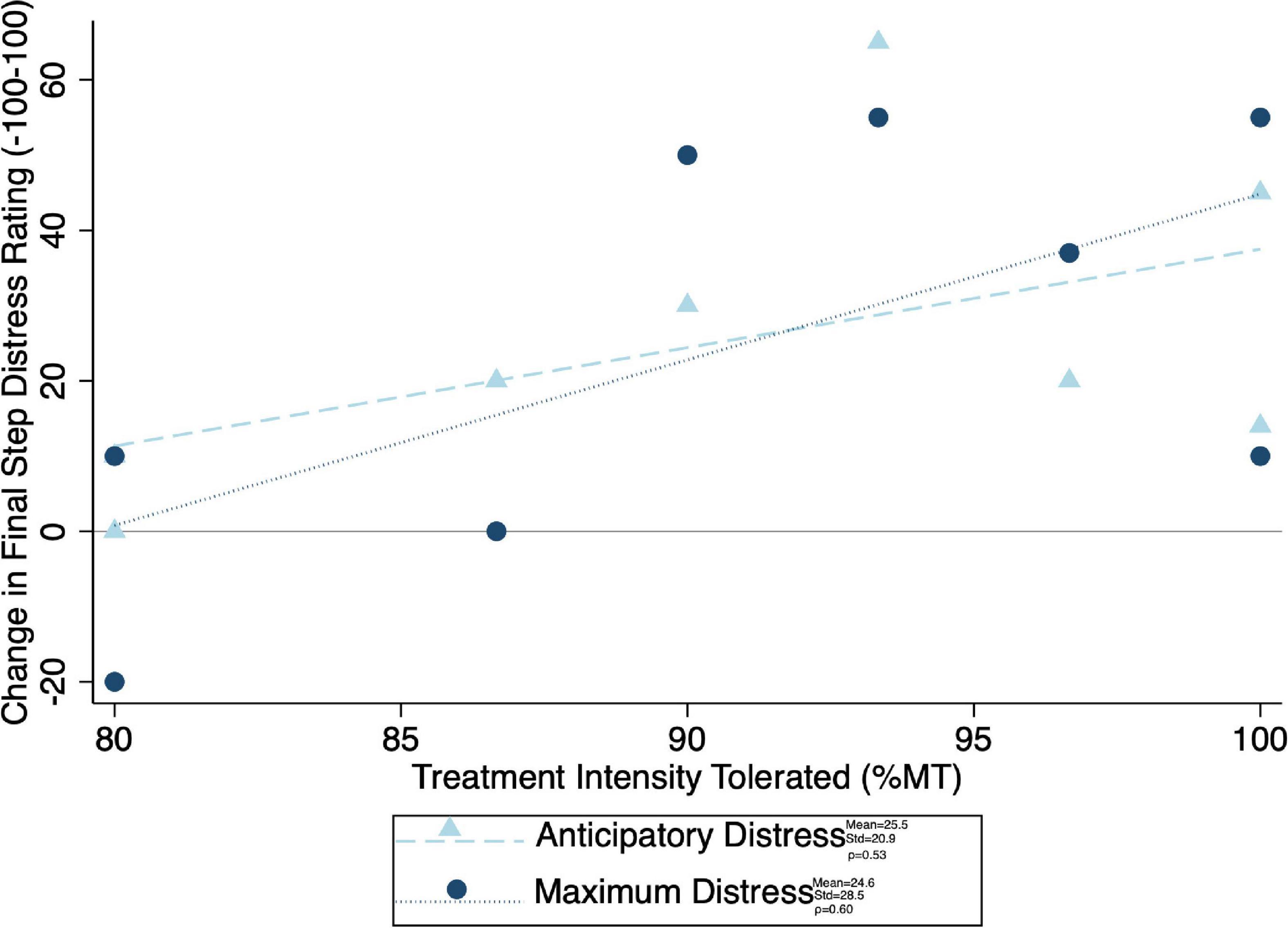
Figure 8. Correlation between maximum treatment intensity tolerated and final common step distress (both anticipatory and maximum experienced distress shown). Change in distress was calculated as pre minus post, with positive values indicating a decrease in distress with treatment. Mean change in distress, standard of deviation of the change in distress, and spearman correlation coefficient (ρ) for both anticipatory and maximum distress shown.
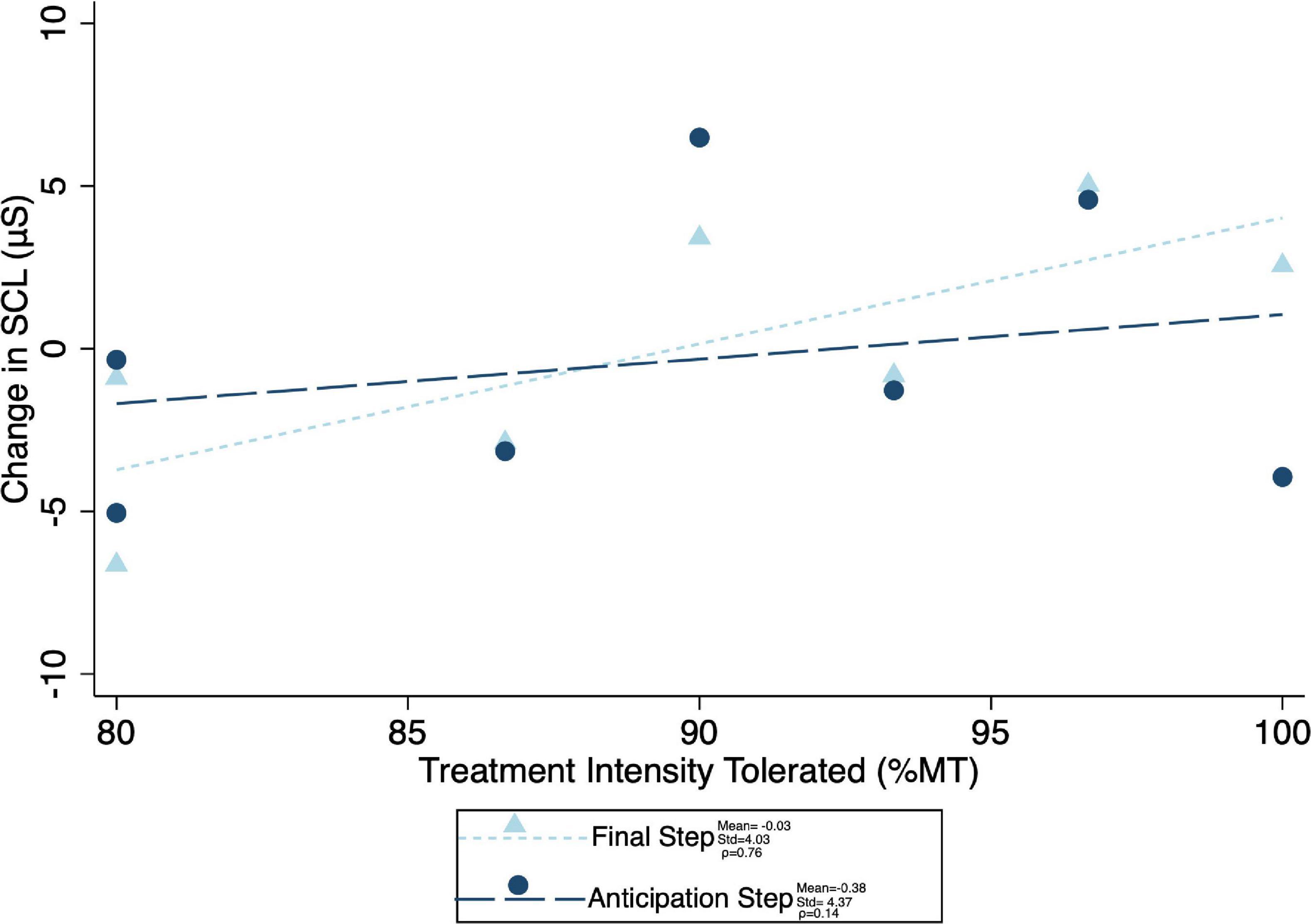
Figure 9. Correlation between maximum treatment intensity tolerated and both anticipation step and mean final common step skin conductance level change (difference in mean skin conductance during the final step that was completed during both the pre- and post-treatment BATs). Change in SCL was calculated as pre minus post, with positive values indicating a decrease in SCL with treatment. Mean change in SCL, standard of deviation of the SCL change, and spearman correlation coefficient (ρ) for both anticipation and final common steps shown.
Validation of Blinding
Blinding validation study utilizing chi-square tests comparing correct and incorrect group assignment guesses by subjects and raters did not significantly deviate from random chance (Table 4).
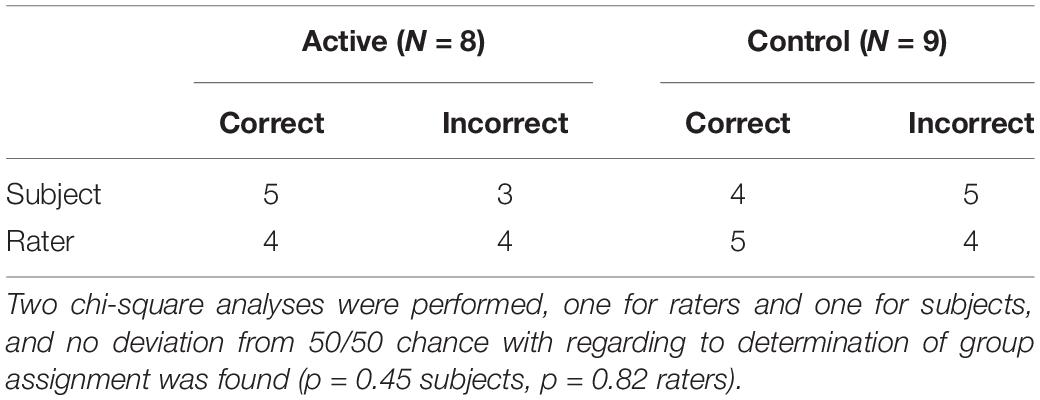
Table 4. Number of subjects and raters correctly guessing group assignment after treatment and repeat BAT.
Discussion
These findings support the feasibility and potential efficacy of rTMS augmentation for the treatment of spider phobia. Most subjects were able to complete the combined iTBS and exposure treatment paradigm, and both groups of subjects experienced a significant reduction in subjective and physiological exposure-related distress. Subjects experienced greater discomfort related to stimulation at the vmPFC site as indicated by the lower average intensity of iTBS administered to this location, raising some concerns regarding the tolerability of treatment. At the same time, there were no differences in drop-out rates and we anticipate subjects would adjust to treatment-associated-discomfort with additional treatment sessions (71, 72). Although there were no significant differences in the benefit of the intervention between treatment groups, post-hoc analyses revealed that those subjects who received more intense active stimulation experienced a greater reduction in both subjective and physiologic distress with treatment. These analyses, though limited in size and scope, indicate the potential presence of intensity-dependent effects of iTBS in the treatment of phobias.
The strong correlation between the treatment intensity tolerated and multiple physiologic and subjective distress measures during exposure suggests that subjects who were able to tolerate greater stimulation intensity were likely to receive the greatest benefit from iTBS treatment. This finding is consistent with the hypothesis that vmPFC is a promising treatment target for specific phobia and supports the need for further research into vmPFC stimulation, including a more prolonged course of treatment (35). Alternatively, is possible that the ability to tolerate a higher treatment intensity reflects an ability to tolerate increased distress from other sources, meaning that the intensity tolerated and the correlated measures of distress simply reflect one’s distress tolerance. This explanation of effects was not tested in the present study and warrants further research.
Prior work theorizes that network-priming effects during exposure therapy are related to enhancement of the response to exposure rather than an independent effect on extinction recall (33, 35, 60). rTMS has demonstrated both some degree of immediate augmentation of the exposure response and its ability to affect (at least transiently) the networks involved with threat processing (the same networks primed by exposures, as performed in this study) (21, 31, 33, 35, 41, 60, 73). However, rTMS is generally performed without any additional stimulus provided during treatment (i.e., rTMS and exposure therapy are generally performed separately). The effects seen with short courses of combined exposure and rTMS therapy have not yet been studied with longer courses of treatment, and the number of treatment sessions needed to achieve maximal benefit should be examined in future work. Although the mechanism of augmentation is not certain, future research should evaluate whether a longer treatment course may yield additive benefits over time and magnify clinical improvement.
The results of the present study should be interpreted in the context of several limitations. In particular, this proof-of-concept study utilized a small sample size. This substantially limits the power of this study, such that conclusions drawn here are preliminary and should be explored in future work. In addition, this study utilized a homogeneous study population (due to recruitment methods), yielding a primarily college-age population, which may limit the generalizability of these results. Future studies should examine these methods in larger, more heterogenous samples with the goal of determining the efficacy of rTMS as a treatment for phobias. This study also utilized very brief treatment compared both to conventional rTMS and exposure treatment standards (48, 74). It is conceivable that differences between the treatment groups could have been amplified by a greater number of exposure practices, possibly resulting in more subjects completing the BAT after treatment completion, as well as a greater number of TMS treatments, which might have resulted in a larger effect. A more prolonged course of treatment would also have the advantage of allowing the subjects greater time to accommodate to the discomfort of stimulation. Future studies also should employ strategies such as ramping up of intensity in order to improve tolerability of stimulation. Furthermore, although we selected our control condition with the goal of imitating the sensation of rTMS without involving the circuitry implicated in the phobic response (as discussed earlier, and as prior work has done), it is possible that our control condition was not inert (23, 62–65). Additionally, this study involved a substantial average delay between exposures and rTMS. This may have limited efficacy of the rTMS, as prior work has utilized shorter time periods between the two (33, 35, 60). Finally, we noted a large (though not statistically significant) difference in pre-treatment baseline SCL between groups. However, our analyses do not presently indicate that this difference limited our ability to detect physiologic differences between groups.
Conclusion
This study demonstrates the feasibility of adjunctive use of rTMS with exposure therapy in a sample of phobic subjects. We found improvement across subjects in active and control conditions, and post-hoc analyses showed that higher vmPFC rTMS intensity correlated with greater improvements in behavioral and physiologic distress. These data, in conjunction with prior work, preliminarily suggest that rTMS may have beneficial effects to enhance exposure therapy. Further work is needed to explore the beneficial nature of rTMS and to further clarify the protocols and parameters to be used for treatment.
Data Availability Statement
The datasets presented in this article are not readily available due to privacy or ethical restrictions. Requests to access the datasets should be directed to ML, bWtsZXVjaHRlckBtZWRuZXQudWNsYS5lZHU=.
Ethics Statement
The studies involving human participants were reviewed and approved by the UCLA Medical IRB #3. The patients/participants provided their written informed consent to participate in this study.
Author Contributions
ML, BR, AL, AM, MC, and MI designed the study with input from DK, NG, JCL, SW, RT, JL, and KM. ML, BR, and GS recruited the subjects for the study. BR, GS, and NW performed the behavioral testing and exposure trials and gathered the corresponding data. AL, DK, NG, JCL, SW, RT, JL, and KM performed the TMS treatments. ML and BR analyzed the study data with input and statistical support from AM, AL, MC, and MI. ML and BR primarily drafted the manuscript. All authors contributed to the data interpretation, assisted in manuscript editing, and approved the final manuscript.
Funding
This project was made possible by the Ryan Family Fund for TMS Research.
Conflict of Interest
ML discloses that he has served as a consultant to Neuroelectrics, Inc., within the past 36 months. AL has served as a consultant to NeoSync, Inc., Ionis Pharmaceuticals, Inc., and ElMindA. AL is Chief Scientific Officer of Brain Biomarker Analytics LLC (BBA), and has equity interest in BBA.
The remaining authors declare that the research was conducted in the absence of any commercial or financial relationships that could be construed as a potential conflict of interest.
Publisher’s Note
All claims expressed in this article are solely those of the authors and do not necessarily represent those of their affiliated organizations, or those of the publisher, the editors and the reviewers. Any product that may be evaluated in this article, or claim that may be made by its manufacturer, is not guaranteed or endorsed by the publisher.
Acknowledgments
We thank the Ryan Family for their generous support of innovative approaches to depression treatment and of groundbreaking TMS technology.
Abbreviations
rTMS, repetitive transcranial magnetic stimulation; vmPFC, ventromedial prefrontal cortex; rTMS, repetitive transcranial magnetic stimulation; iTBS, intermittent theta burst stimulation; SPQ, spider phobia questionnaire; FSQ, fear of spiders questionnaire; BAT, behavioral avoidance test; SCL, skin conductance level; PHQ9, patient health questionnaire-9; GAD-7, generalized anxiety disorder-7; HAMD, Hamilton Depression Rating Scale; ET, exposure therapy; MDD, major depressive disorder; GAD, generalized anxiety disorder; RMT, resting motor threshold; AntD, anticipatory distress; MaxD, maximum distress; MLM, multi-level mixed-effects model.
References
1. Kessler RC, Petukhova M, Sampson NA, Zaslavsky AM, Wittchen H-U. Twelve-month and lifetime prevalence and lifetime morbid risk of anxiety and mood disorders in the United States. Int J Methods Psychiatr Res. (2012) 21:169–84. doi: 10.1002/mpr.1359
2. Wardenaar KJ, Lim CCW, Al-Hamzawi AO, Alonso J, Andrade LH, Benjet C, et al. The cross-national epidemiology of specific phobia in the World Mental Health Surveys. Psychol. Med. 47:1744–60. doi: 10.1017/S0033291717000174
3. Eaton WW, Bienvenu OJ, Miloyan B. Specific phobias. Lancet Psychiatry. (2018) 5:678–86. doi: 10.1016/S2215-0366(18)30169-X
4. Choy Y, Fyer AJ, Lipsitz JD. Treatment of specific phobia in adults. Clin Psychol Rev. (2007) 27:266–86. doi: 10.1016/j.cpr.2006.10.002
5. Wolitzky-Taylor KB, Horowitz JD, Powers MB, Telch MJ. Psychological approaches in the treatment of specific phobias: a meta-analysis. Clin Psychol Rev. (2008) 28:1021–37. doi: 10.1016/j.cpr.2008.02.007
6. Craske MG, Kircanski K, Zelikowsky M, Mystkowski J, Chowdhury N, Baker A. Optimizing inhibitory learning during exposure therapy. Behav Res Ther. (2008) 46:5–27. doi: 10.1016/j.brat.2007.10.003
7. Craske M, Treanor M, Conway C, Zbozinek T, Vervliet B. Maximizing exposure therapy: an inhibitory learning approach. Behav Res Ther. (2014) 58:10–23. doi: 10.1016/j.brat.2014.04.006.Maximizing
8. Issakidis C, Andrews G. Pretreatment attrition and dropout in an outpatient clinic for anxiety disorders. Acta Psychiatr Scand. (2004) 109:426–33. doi: 10.1111/j.1600-0047.2004.00264.x
9. Essau CA, Conradt J, Petermann F. Frequency, comorbidity, and psychosocial impairment of specific phobia in adolescents. J Clin Child Adolesc Psychol. (2000) 29:221–31. doi: 10.1207/S15374424jccp2902_8
10. George MS, Wassermann EM, Williams WA, Callahan A, Ketter TA, Basser P, et al. Daily repetitive transcranial magnetic stimulation (rTMS) improves mood in depression. Neuroreport. (1995) 6:1853–6. doi: 10.1097/00001756-199510020-00008
11. O’Reardon JP, Solvason HB, Janicak PG, Sampson S, Isenberg KE, Nahas Z, et al. Efficacy and safety of transcranial magnetic stimulation in the acute treatment of major depression: a multisite randomized controlled trial. Biol Psychiatry. (2007) 62:1208–16. doi: 10.1016/j.biopsych.2007.01.018
12. Perera T, George MS, Grammer G, Janicak PG, Pascual-Leone A, Wirecki TS. The clinical TMS society consensus review and treatment recommendations for TMS therapy for major depressive disorder. Brain Stimul. (2016) 9:336–46. doi: 10.1016/j.brs.2016.03.010
13. Jaafari N, Rachid F, Rotge JY, Polosan M, El-Hage W, Belin D, et al. Safety and efficacy of repetitive transcranial magnetic stimulation in the treatment of obsessive-compulsive disorder: a review. World J Biol Psychiatry. (2012) 13:164–77. doi: 10.3109/15622975.2011.575177
14. Trevizol AP, Shiozawa P, Cook IA, Sato IA, Kaku CB, Guimarães FB, et al. Transcranial magnetic stimulation for obsessive-compulsive disorder: an updated systematic review and meta-analysis. J ECT. (2016) 32:262–6. doi: 10.1097/YCT.0000000000000335
15. Gorelick DA, Zangen A, George MS. Transcranial magnetic stimulation in the treatment of substance addiction. Ann N Y Acad Sci. (2014) 1327:79–93. doi: 10.1111/nyas.12479
16. Dunlop K, Hanlon CA, Downar J. Noninvasive brain stimulation treatments for addiction and major depression. Ann N Y Acad Sci. (2017) 1394:31–54. doi: 10.1111/nyas.12985
17. Ekhtiari H, Tavakoli H, Addolorato G, Baeken C, Bonci A, Campanella S, et al. Transcranial electrical and magnetic stimulation (tES and TMS) for addiction medicine: a consensus paper on the present state of the science and the road ahead. Neurosci Biobehav Rev. (2019) 104:118–40. doi: 10.1016/j.neubiorev.2019.06.007
18. Wieczorek T, Kobyłko A, Stramecki F, Fila-Witecka K, Beszłej J, Jakubczyk M, et al. Transcranial magnetic stimulation (TMS) in psychiatric disorders treatment - current review. Psychiatr Pol. (2021) 55:565–83. doi: 10.12740/PP/ONLINEFIRST/115556
19. Croarkin PE, Wall CA, Lee J. Applications of transcranial magnetic stimulation (TMS) in child and adolescent psychiatry. Int Rev Psychiatry. (2011) 23:445–53. doi: 10.3109/09540261.2011.623688
20. Kim TD, Hong G, Kim J, Yoon S. Cognitive enhancement in neurological and psychiatric disorders using transcranial magnetic stimulation (TMS): a review of modalities, potential mechanisms and future implications. Exp Neurobiol. (2019) 28:1–16. doi: 10.5607/en.2019.28.1.1
21. Leuchter AF, Hunter AM, Krantz DE, Cook IA. Rhythms and blues: modulation of oscillatory synchrony and the mechanism of action of antidepressant treatments. Ann N Y Acad Sci. (2015) 1344:78–91. doi: 10.1111/nyas.12742
22. Fox MD, Buckner RL, White MP, Greicius MD, Pascual-Leone A. Efficacy of transcranial magnetic stimulation targets for depression is related to intrinsic functional connectivity with the subgenual cingulate. Biol Psychiatry. (2012) 72:595–603. doi: 10.1016/j.biopsych.2012.04.028
23. Tik M, Hoffmann A, Sladky R, Tomova L, Hummer A, Navarro de Lara L, et al. Towards understanding rTMS mechanism of action: stimulation of the DLPFC causes network-specific increase in functional connectivity. Neuroimage. (2017) 162:289–96. doi: 10.1016/j.neuroimage.2017.09.022
24. Philip NS, Barredo J, van ’t Wout-Frank M, Tyrka AR, Price LH, Carpenter LL. Network mechanisms of clinical response to transcranial magnetic stimulation in posttraumatic stress disorder and major depressive disorder. Biol Psychiatry. (2018) 83:263–72. doi: 10.1016/j.biopsych.2017.07.021
25. Taylor R, Galvez V, Loo C. Transcranial magnetic stimulation (TMS) safety: a practical guide for psychiatrists. Australas Psychiatry. (2018) 26:189–92. doi: 10.1177/1039856217748249
26. Paes F. Repetitive transcranial magnetic stimulation (rTMS) to treat social anxiety disorder: case reports and a review of the literature. Clin Pract Epidemiol Ment Health. (2013) 9:180–8. doi: 10.2174/1745017901309010180
27. Machado S, Arias-Carrión O, Paes F, Vieira RT, Caixeta L, Novaes F, et al. Nörolojik ve psikiyatrik bozukluklarda klinik uygulamalar için transkraniyal manyetik uyari{dotless}m: genel bir baki{dotless}ş. Eurasian J Med. (2013) 45:191–206. doi: 10.5152/eajm.2013.39
28. Sagliano L, Atripaldi D, De Vita D, D’Olimpio F, Trojano L. Non-invasive brain stimulation in generalized anxiety disorder: a systematic review. Prog Neuro Psychopharmacol Biol Psychiatry. (2019) 93:31–8. doi: 10.1016/j.pnpbp.2019.03.002
29. Karsen EF, Watts BV, Holtzheimer PE. Review of the effectiveness of transcranial magnetic stimulation for post-traumatic stress disorder. Brain Stimul. (2014) 7:151–7. doi: 10.1016/j.brs.2013.10.006
30. Guhn A, Dresler T, Hahn T, Mühlberger A, Ströhle A, Deckert J, et al. Medial prefrontal cortex activity during the extinction of conditioned fear: an investigation using functional near-infrared spectroscopy. Neuropsychobiology. (2012) 65:173–82. doi: 10.1159/000337002
31. Baeken C, De Raedt R, Van Schuerbeek P, Vanderhasselt MA, De Mey J, Bossuyt A, et al. Right prefrontal HF-rTMS attenuates right amygdala processing of negatively valenced emotional stimuli in healthy females. Behav Brain Res. (2010) 214:450–5. doi: 10.1016/j.bbr.2010.06.029
32. Deppermann S, Notzon S, Kroczek A, Rosenbaum D, Haeussinger FB, Diemer J, et al. Functional co-activation within the prefrontal cortex supports the maintenance of behavioural performance in fear-relevant situations before an iTBS modulated virtual reality challenge in participants with spider phobia. Behav Brain Res. (2016) 307:208–17. doi: 10.1016/j.bbr.2016.03.028
33. Notzon S, Deppermann S, Fallgatter A, Diemer J, Kroczek A, Domschke K, et al. Psychophysiological effects of an iTBS modulated virtual reality challenge including participants with spider phobia. Biol Psychol. (2015) 112:66–76. doi: 10.1016/j.biopsycho.2015.10.003
34. Downar J, Daskalakis ZJ. New targets for rTMS in depression: a review of convergent evidence. Brain Stimul. (2013) 6:231–40. doi: 10.1016/j.brs.2012.08.006
35. Herrmann MJ, Katzorke A, Busch Y, Gromer D, Polak T, Pauli P, et al. Medial prefrontal cortex stimulation accelerates therapy response of exposure therapy in acrophobia. Brain Stimul. (2017) 10:291–7. doi: 10.1016/j.brs.2016.11.007
36. Kim MJ, Whalen PJ. The structural integrity of an amygdala-prefrontal pathway predicts trait anxiety. J Neurosci. (2009) 29:11614–8. doi: 10.1523/JNEUROSCI.2335-09.2009
37. Kim MJ, Loucks RA, Palmer AL, Brown AC, Solomon KM, Marchante AN, et al. The structural and functional connectivity of the amygdala: from normal emotion to pathological anxiety. Behav Brain Res. (2011) 223:403–10. doi: 10.1016/j.bbr.2011.04.025
38. Bukalo O, Pinard CR, Silverstein S, Brehm C, Hartley ND, Whittle N, et al. Prefrontal inputs to the amygdala instruct fear extinction memory formation. Sci Adv. (2015) 1:1–9. doi: 10.1126/sciadv.1500251
39. Gold AL, Morey RA, McCarthy G. Amygdala–prefrontal cortex functional connectivity during threat-induced anxiety and goal distraction. Biol Psychiatry. (2015) 77:394–403. doi: 10.1016/j.biopsych.2014.03.030
40. Motzkin JC, Philippi CL, Wolf RC, Baskaya MK, Koenigs M. Ventromedial prefrontal cortex is critical for the regulation of amygdala activity in humans. Biol Psychiatry. (2015) 77:276–84. doi: 10.1016/j.biopsych.2014.02.014
41. Riedel P, Heil M, Bender S, Dippel G, Korb FM, Smolka MN, et al. Modulating functional connectivity between medial frontopolar cortex and amygdala by inhibitory and excitatory transcranial magnetic stimulation. Hum Brain Mapp. (2019) 40:4301–15. doi: 10.1002/hbm.24703
42. Kalisch R, Korenfeld E, Stephan KE, Weiskopf N, Seymour B, Dolan RJ. Context-dependent human extinction memory is mediated by a ventromedial prefrontal and hippocampal network. J Neurosci. (2006) 26:9503–11. doi: 10.1523/JNEUROSCI.2021-06.2006
43. Corcoran KA, Quirk GJ. Recalling safety: cooperative functions of the ventromedial prefrontal cortex and the hippocampus in extinction. CNS Spectr. (2007) 12:200–6. doi: 10.1017/S1092852900020915
44. Milad MR, Wright CI, Orr SP, Pitman RK, Quirk GJ, Rauch SL. Recall of fear extinction in humans activates the ventromedial prefrontal cortex and hippocampus in concert. Biol Psychiatry. (2007) 62:446–54. doi: 10.1016/j.biopsych.2006.10.011
45. Bulteau S, Sébille V, Fayet G, Thomas-Ollivier V, Deschamps T, Bonnin-Rivalland A, et al. Efficacy of intermittent theta burst stimulation (iTBS) and 10-Hz high-frequency repetitive transcranial magnetic stimulation (rTMS) in treatment-resistant unipolar depression: study protocol for a randomised controlled trial. Trials. (2017) 18:1–10. doi: 10.1186/s13063-016-1764-8
46. Chung SW, Hoy KE, Fitzgerald PB. Theta-burst stimulation: a new form of tms treatment for depression? Depress Anxiety. (2015) 32:182–92. doi: 10.1002/da.22335
47. Mutz J, Edgcumbe DR, Brunoni AR, Fu CHY. Efficacy and acceptability of non-invasive brain stimulation for the treatment of adult unipolar and bipolar depression: a systematic review and meta-analysis of randomised sham-controlled trials. Neurosci Biobehav Rev. (2018) 92:291–303. doi: 10.1016/j.neubiorev.2018.05.015
48. Blumberger DM, Vila-Rodriguez F, Thorpe KE, Feffer K, Noda Y, Giacobbe P, et al. Effectiveness of theta burst versus high-frequency repetitive transcranial magnetic stimulation in patients with depression (THREE-D): a randomised non-inferiority trial. Lancet. (2018) 391:1683–92. doi: 10.1016/S0140-6736(18)30295-2
49. McGlade AL, Craske MG. Optimizing exposure: between-session mental rehearsal as an augmentation strategy. Behav Res Ther. (2021) 139:103827. doi: 10.1016/j.brat.2021.103827
50. Christopoulos GI, Uy MA, Yap WJ. The body and the brain: measuring skin conductance responses to understand the emotional experience. Organ Res Methods. (2016) 22:394–420. doi: 10.1177/1094428116681073
51. Kircanski K, Lieberman MD, Craske MG. Feelings into words: contributions of language to exposure therapy. Psychol Sci. (2012) 23:1086–91. doi: 10.1177/0956797612443830
52. Cook RJ, Dickens BM, Fathalla MF. World medical association declaration of Helsinki: ethical principles for medical research involving human subjects. J Am Coll Dent. (2014) 81:14–8. doi: 10.1093/acprof:oso/9780199241323.003.0025
53. Klorman R, Weerts TC, Hastings JE, Melamed BG, Lang PJ. Psychometric description of some specific-fear questionnaires. Behav Ther. (1974) 5:401–9. doi: 10.1016/S0005-7894(74)80008-0
54. Szymanski J, O’Donohue W. Fear of spiders questionnaire. J Behav Ther Exp Psychiatry. (1995) 26:31–4. doi: 10.1016/0005-7916(94)00072-T
55. Corlier J, Carpenter LL, Wilson AC, Tirrell E, Gobin AP, Kavanaugh B, et al. The relationship between individual alpha peak frequency and clinical outcome with repetitive transcranial magnetic stimulation (rTMS) treatment of major depressive disorder (MDD). Brain Stimul. (2019) 12:1572–8. doi: 10.1016/j.brs.2019.07.018
56. Rossini PM, Burke D, Chen R, Cohen LG, Daskalakis Z, Di Iorio R, et al. Non-invasive electrical and magnetic stimulation of the brain, spinal cord, roots and peripheral nerves: basic principles and procedures for routine clinical and research application. an updated report from an I.F.C.N. Committee. Clin Neurophysiol. (2015) 126:1071–107. doi: 10.1016/j.clinph.2015.02.001
57. Dissanayaka T, Zoghi M, Farrell M, Egan G, Jaberzadeh S. Comparison of Rossini–Rothwell and adaptive threshold-hunting methods on the stability of TMS induced motor evoked potentials amplitudes. J Neurosci Res. (2018) 96:1758–65. doi: 10.1002/jnr.24319
58. Ferland MC, Therrien-Blanchet JM, Lefebvre G, Klees-Themens G, Proulx S, Théoret H. Longitudinal assessment of 1H-MRS (GABA and Glx) and TMS measures of cortical inhibition and facilitation in the sensorimotor cortex. Exp Brain Res. (2019) 237:3461–74. doi: 10.1007/s00221-019-05691-z
59. Beam W, Borckardt JJ, Reeves ST, George MS. An efficient and accurate new method for locating the F3 position for prefrontal TMS applications. Brain Stimul. (2009) 2:50–4. doi: 10.1016/j.brs.2008.09.006
60. Guhn A, Dresler T, Andreatta M, Müller LD, Hahn T, Tupak SV, et al. Medial prefrontal cortex stimulation modulates the processing of conditioned fear. Front Behav Neurosci. (2014) 8:44. doi: 10.3389/fnbeh.2014.00044
61. Suarez-Jimenez B, Bisby JA, Horner AJ, King JA, Pine DS, Burgess N. Linked networks for learning and expressing location-specific threat. Proc Natl Acad Sci USA. (2018) 115:E1032–40. doi: 10.1073/pnas.1714691115
62. Weiss PH, Sparing R, Fink GR, Tomasino B, Dafotakis M. Action verbs and the primary motor cortex: a comparative TMS study of silent reading, frequency judgments, and motor imagery. Neuropsychologia. (2008) 46:1915–26. doi: 10.1016/j.neuropsychologia.2008.01.015
63. Foltys H, Sparing R, Boroojerdi B, Krings T, Meister IG, Mottaghy FM, et al. Motor control in simple bimanual movements: a transcranial magnetic stimulation and reaction time study. Clin Neurophysiol. (2001) 112:265–74. doi: 10.1016/S1388-2457(00)00539-3
64. Duecker F, de Graaf TA, Jacobs C, Sack AT. Time- and task-dependent non-neural effects of real and sham TMS. PLoS One. (2013) 8:e73813. doi: 10.1371/journal.pone.0073813
65. Nyffeler T, Wurtz P, Pflugshaupt T, von Wartburg R, Luthi M, Hess CW, et al. One-Hertz transcranial magnetic stimulation over the frontal eye field induces lasting inhibition of saccade triggering. Neuroreport. (2006) 17:273–5. doi: 10.1097/01.wnr.0000199468.39659.bf
66. Rossi S, Hallett M, Rossini PM, Pascual-Leone A. Safety of TMS consensus group. safety, ethical considerations, and application guidelines for the use of transcranial magnetic stimulation in clinical practice and research. Clin Neurophysiol. (2009) 120:2008–39. doi: 10.1016/j.clinph.2009.08.016
67. Jung J, Bungert A, Bowtell R, Jackson SR. Vertex stimulation as a control site for transcranial magnetic stimulation: a concurrent TMS/fMRI Study. Brain Stimul. (2016) 9:58–64. doi: 10.1016/j.brs.2015.09.008
68. Buckner RL, Andrews-Hanna JR, Schacter DL. The brain’s default network: anatomy, function, and relevance to disease. Ann N Y Acad Sci. (2008) 1124:1–38. doi: 10.1196/annals.1440.011
69. Duecker F, Sack AT. Rethinking the role of sham TMS. Front Psychol. (2015) 6:210. doi: 10.3389/fpsyg.2015.00210
70. Melzack R. The short-form McGill pain questionnaire. Pain. (1987) 30:191–7. doi: 10.1016/0304-3959(87)91074-8
71. Janicak PG, O’Reardon JP, Sampson SM, Husain MM, Lisanby SH, Rado JT, et al. Transcranial magnetic stimulation in the treatment of major depressive disorder: a comprehensive summary of safety experience from acute exposure, extended exposure, and during reintroduction treatment. J Clin Psychiatry. (2008) 69:222–32. doi: 10.4088/JCP.v69n0208
72. Anderson BS, Kavanagh K, Borckardt JJ, Nahas ZH, Kose S, Lisanby SH, et al. Decreasing procedural pain over time of left prefrontal rtms for depression: initial results from the open-label phase of a multisite trial (OPT-TMS). Brain Stimul. (2009) 2:88–92. doi: 10.1016/j.brs.2008.09.001
73. Scharmüller W, Leutgeb V, Schäfer A, Köchel A, Schienle A. Source localization of late electrocortical positivity during symptom provocation in spider phobia: an sLORETA study. Brain Res. (2011) 1397:10–8. doi: 10.1016/j.brainres.2011.04.018
Keywords: intermittent theta-burst stimulation (iTBS), phobia, spider phobia, neuromodulation, transcranial magnetic stimulation (TMS), repetitive transcranial magnetic stimulation (rTMS), behavioral
Citation: Leuchter MK, Rosenberg BM, Schapira G, Wong NR, Leuchter AF, McGlade AL, Krantz DE, Ginder ND, Lee JC, Wilke SA, Tadayonnejad R, Levitt J, Marder KG, Craske MG and Iacoboni M (2022) Treatment of Spider Phobia Using Repeated Exposures and Adjunctive Repetitive Transcranial Magnetic Stimulation: A Proof-of-Concept Study. Front. Psychiatry 13:823158. doi: 10.3389/fpsyt.2022.823158
Received: 26 November 2021; Accepted: 10 February 2022;
Published: 15 March 2022.
Edited by:
Murat Ilhan Atagün, Çanakkale Onsekiz Mart University, TurkeyReviewed by:
Lee Wei Lim, The University of Hong Kong, Hong Kong SAR, ChinaWiebke Struckmann, Uppsala University, Sweden
Copyright © 2022 Leuchter, Rosenberg, Schapira, Wong, Leuchter, McGlade, Krantz, Ginder, Lee, Wilke, Tadayonnejad, Levitt, Marder, Craske and Iacoboni. This is an open-access article distributed under the terms of the Creative Commons Attribution License (CC BY). The use, distribution or reproduction in other forums is permitted, provided the original author(s) and the copyright owner(s) are credited and that the original publication in this journal is cited, in accordance with accepted academic practice. No use, distribution or reproduction is permitted which does not comply with these terms.
*Correspondence: Michael K. Leuchter, bWtsZXVjaHRlckBtZWRuZXQudWNsYS5lZHU=