- 1Department of Neurology, Children's Hospital of Fudan University, Shanghai, China
- 2Shanghai YangZhi Rehabilitation Hospital, School of Medicine, Tongji University, Shanghia, China
Objective: This study investigated the mental development of children with Angelman syndrome (AS) in China and evaluated the relationship between neurodevelopment and molecular subtype, age, epilepsy, and sex using the Chinese version of the Griffith Mental Development Scale (GMDS-C) to provide detailed baseline data regarding neurodevelopment with AS in China.
Methods: Participants were recruited from the AS Natural History Study. The GMDS-C was used to evaluate all participants' mental age and developmental quotients. The general quotient (GQ) and quotients of five subscales (sports, personal-social, auditory language, eye-hand coordination, and comprehensive performance) were calculated.
Results: A total of 119 children (average age: 42.12 months; range, 7.5–95.5 months) with a genetic diagnosis of AS were enrolled. The median GQ score of the GMDS was 29.6 points (95% confidence interval, 28.6–33.25). The children had relatively good locomotor and personal-social skills but poor language skills. Overall, 89% (106/119) had mental ages younger than 24 months for all five subscales. The non-deletion group (i.e., without deletion in chromosome 15q11–13) had higher GQs and locomotor, personal-social, and performance subscale quotients. The GQ was significantly different among the three age subgroups and significantly correlated with age. Compared with the non-epilepsy group, the epilepsy group had lower GQs and lower quotients for the locomotor, personal-social, speech, language, and eye-hand coordination subscales.
Conclusion: Children with AS in China experience severe neurodevelopmental deterioration. In addition to age, molecular subtypes and the onset of seizures may also correlate with these patients' intellectual development. The GMDS-C is an accurate tool that can assess the clinical characteristics of AS. The data of this study can be used as baseline data for clinical trials performed to evaluate drug development or other AS treatment development.
Introduction
Angelman syndrome (AS; OMIM: 105830) is a rare neurogenetic disorder prevalent in approximately 1 in 24,000 to 1 in 52,000 of the population (1, 2). AS is characterized by developmental delay, epilepsy, loss of language, and the tendency to laugh (3). Although the clinical features and neurodevelopment vary, all patients require lifelong care, thereby imposing a huge burden on the family and society (4, 5). Etiologically, AS involves decreased expression of maternal ubiquitin ligase protein 3a (UBE3A), which has maternal-specific expression in neurons by genomic imprinting (6, 7). The four molecular subtypes of AS have different mechanisms: maternal deletion in 15q11–q13, which involves the critical region (68–75%); mutation in UBE3A (Mut) (8–11%); paternal uniparental disomy (UPD) (2–7%); and imprinted gene defect (ID) (2–5%) (8, 9).
Children with AS usually have a considerable intellectual disability, especially language delay, and the few patients who can express themselves have limited vocabulary (10). Additionally, patients with AS have movement incongruity, dystonia, and other conditions that limit motor development (11). These characteristic developmental delays and imbalances can be used to diagnose AS.
The molecular subtype affects the phenotype, and individuals with deletions in 15q11–13 often have a more severe presentation than those without deletions (12, 13). A study conducted in 2020 found that compared with the non-deletion type, children with AS of deletion type had lower scores at baseline and lower acquisition of skills using the Bayley-III. This study also simultaneously describes the developmental trajectories of individuals of all molecular subtypes (14). Epilepsy may also affect the development of AS. Epilepsy occurs in approximately 80% of children with AS, and the first epileptic attack usually occurs early, before age 3 years (15). However, although there have been studies on the mechanism of and treatment for epilepsy in children with AS (6, 16, 17), the relationship between epilepsy and intellectual development has not been clarified. One small study found that epileptic activity is not associated with developmental milestones in patients with AS (18), while another study suggested that early-onset epilepsy (<2 years of age) may cause severe development delay (19). Given that the current conclusions are still controversial, we also took this factor into account in this study. Otherwise, the effect of sex on neurodevelopment has not been studied.
To the best of our knowledge, despite the increasing number of AS cases in China (20, 21), no studies have examined their neurodevelopment objectively. Additionally, although natural history research has become increasingly important to targeted therapy development, the neurodevelopment of patients with AS is often difficult to evaluate and compare because of the severe intellectual disability and dispersion (22). Furthermore, current intelligence screening methods have many limitations. The Wechsler Intelligence Scale (23) could accurately identify children with intellectual development disorder, but it lacks discrimination for diseases involving severe intellectual disability. Bayley-III, which is a scale designed for children younger than 42 months (24, 25), has limited potential for evaluating developmental skills; therefore, it may fail to appreciate the skills of children with advanced development. Although Bayley-III has been used to assess the development of patients with AS, there is still no consensus regarding the appropriate threshold for neurodevelopmental delay (26, 27). Because of the lack of standardized guidelines for the use of Bayley-III in China, the long evaluation time, and the complicated evaluation and analysis, it is not considered well-suited for assessing children with AS. The Gesell Developmental Scale, which is suitable for children younger than 6 years, has similar limitations and has not been revised in the Chinese context for more than 20 years (28).
The Griffiths Mental Development Scale Chinese version (GMDS-C) is an accurate and objective tool used to assess the mental development of children from birth to 8 years, especially those with developmental disorders (29). It is widely used in many countries and has excellent psychometric performance (30, 31). The GMDS-C was improved and updated in the Chinese context in 2016. An across-context analysis also confirmed that the GMDS is applicable for the assessment of children from birth to age 8 years in China (32). Furthermore, language skills do not have much influence on the evaluation of other scales, thereby allowing children with AS to perform more tasks despite the lack of language.
This study aimed to investigate the mental development of children with AS in China and evaluate the correlation between neurodevelopment and the molecular subtype, age, epilepsy, and sex using the GMDS-C. We collected the clinical and genetic characteristics of 119 children with genetically confirmed AS and evaluated their locomotor, personal-social, speech, language, and eye-hand coordination abilities using the GMDS-C. Furthermore, the age at the time of diagnosis of AS was collected, and the mental age, subscale quotient, and general quotient (GQ) were calculated as statistical indicators for analysis (33, 34) to provide detailed neurodevelopmental baseline data for AS natural history research in China and provide evidence for follow-up intervention research.
Methods
Study Design and Participants
This cross-sectional study was approved by the Ethical Institutional Review Committee of Children's Hospital of Fudan University and was conducted according to the tenets of the Declaration of Helsinki. Written informed consent was obtained from the legal guardian of each participant.
Participants in the AS Natural History study (ClinicalTrial.gov identifier: NCT03358823) were studied from January 2015 to December 2020. At least two pediatric neurologists at the Children's Hospital of Fudan University in China that were familiar with AS, conducted interviews and physical examinations of all patients. The inclusion criteria were as follows: age 6 months to 8 years; molecularly confirmed diagnosis of AS that can be classified as one of the four molecular subtypes (maternal deletion in the key region of 15q11.2–q13; paternal UPD; imprint center defect [ID]; and UBE3A mutation); clinical characteristics corresponding to the updated consensus for the diagnostic criteria of AS in 2005 (3); and no other comorbidities that might obscure the AS phenotype. Participants were excluded if they had the mosaic genotype or frequent epileptic attacks that may affect the evaluation process.
Participants with a deletion in chromosome 15q11–13 were classified as the deletion group, whereas participants with the other three molecular subtypes were classified as the non-deletion group. The deletion group was further divided into three subgroups by age as follows: group 1, age 6–36 months; group 2, age 36–60 months; and group 3, age 60–96 months. To analyze the correlation between neurodevelopment and epilepsy, age-matched patients with and without epilepsy were categorized as the epilepsy group and non-epilepsy group; all of those patients with and without epilepsy were in the deletion group.
Neurodevelopmental Assessment
General characteristics, including age, sex, mutation type, age at the time of the first evaluation, age at the time of diagnosis, and seizure status (according to the International League Against Epilepsy Classification of the Epilepsies in 2017) (30) were collected using a self-designed general survey form. Neurodevelopment was assessed using the GMDS-C. Briefly, the GMDS-C includes six subscales (locomotor, personal-social interaction, hearing and language, eye-hand coordination, performance, and practical reasoning); only five of those subscales (locomotor, personal-social interaction, hearing and language, eye-hand coordination, performance) are needed to evaluate children younger than 2 years. The development quotient (DQ) was expressed as the ratio of the DQ as follows: DQ = (mental age/chronological age) × 100. We calculated subscale quotients for each subscale and one GQ for general. The mean GQ was 100 in normal children (standard deviation [SD] = 15), and a quotient <70 for each subscale indicated severe neurodevelopmental delay (23, 24).
Statistical Analysis
The results were analyzed according to different molecular subtypes, presence of epilepsy, and age at the time of assessment. The Kolmogorov-Smirnov test was used to analyze data normality. Continuous variables are described as mean ± SD or the 50th percentile (median) with the 25th percentile and 75th percentile; categorical variables are presented as the frequency and percentage. A t-test or non-parametric test was used to compare differences between two groups; a one-way analysis of variance was applied to compare more groups. The association of age with the subscale quotients and GQs was investigated using a linear analysis. All statistical analyses were performed using SPSS Statistics (version 20.0; IBM Corp., Armonk, NY, USA). The significance threshold was set at P < 0.05.
Results
Neurodevelopment
A total of 119 children (75 boys and 44 girls) with a mean age of 42.12 months (SD = 21.0; range, 7.5–95.5 months) were enrolled. The median GQ was 29.6 points (95% confidence interval = 28.6–33.25 points). None of the participants had measurable scores for practical reasoning, poor language skills, and limited developmental levels. In addition, there was a significant difference in the five subscale quotients (P < 0.0001). Table 1 shows the subscale quotients and GQs of the participants. A comparison of the subscale quotients with GQs showed that the quotient of the locomotor subscale was significantly higher than the GQ (P = 0.0061), whereas the quotient of the language subscale was significantly lower than the GQ (P < 0.0001).
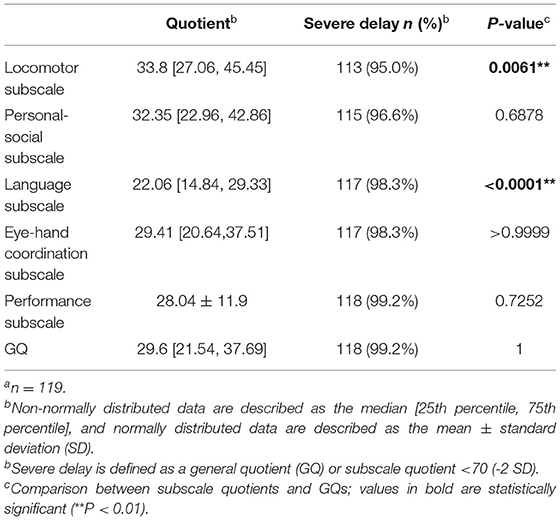
Table 1. Results of GMDS-C assessmenta.
Regarding mental age, 89.1% (106/119) of the participants had mental ages younger than 24 months for all five subscales, and the other 11.9% (13/119) had mental ages older than 24 months for at least one subscale (seven patients were in the deletion group and six patients were in the non-deletion group). The distribution of mental ages for the five subscales differed and ranged from 2 months to 47.5 months for the locomotor subscale; from 5 months to 39 months for the personal-social subscale; from 2.5 months to 24.5 months for the speech and language subscale; from 2 months to 36 months for the eye-hand coordination subscale; and from 1.5 months to 40 months for the performance subscale. The specific distributions are shown in Figure 1.
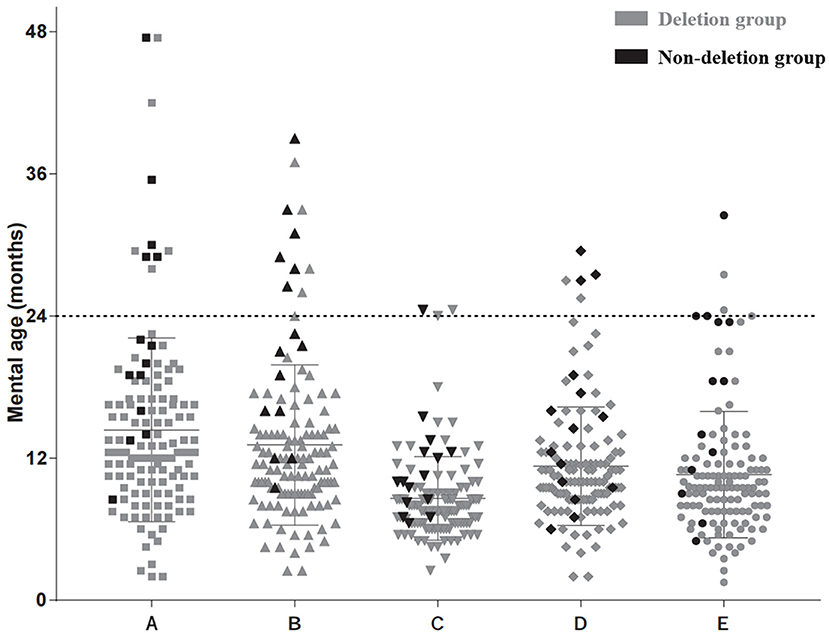
Figure 1. Mental age distribution according to five subscales. (A) Locomotor subscale. (B) Personal-social subscale. (C) Speech and language subscale. (D) Eye-hand coordination subscale. (E) Performance subscale. Each black dot represents one result of the deletion type in this area. Each gray dot represents one result of the non-deletion type.
Distribution of the Subscale Quotients by Age
Table 2 shows the distribution of GQs and subscale quotients of the participants in the following age groups: 6–36 months (48 observations); 36–60 months (34 observations); and 60–96 months (21 observations). There were significant differences in GQs and all subscale quotients among the three age subgroups (P < 0.001). A correlation analysis of different age subgroups found significant negative correlations between the chronological age during assessment and the development quotients for the five subscales and GQs (r < 0; P < 0.001). The SDs of the mean are shown by the error bars in Figure 2.
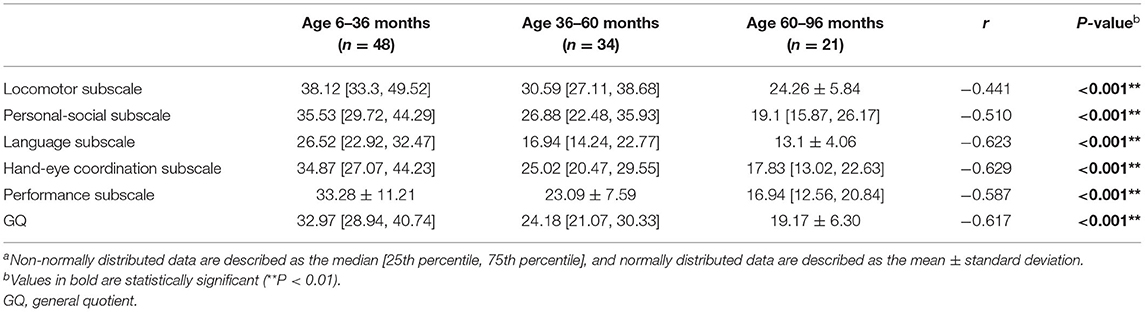
Table 2. Distribution of the subscale quotients for the GMDS-C by age groupa.
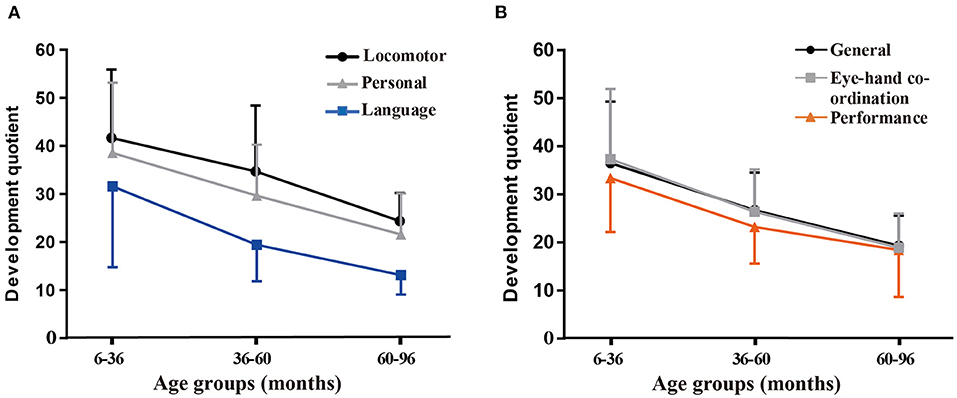
Figure 2. Variations in the mean general quotients (GQs) and quotients for the five subscales according to the age group. (A) Variations in the mean quotients for the locomotor, personal-social, and language subscales. (B) Variations in the mean GQs and quotients for hand-eye coordination and performance subscales.
Correlation Between Neurodevelopment and Molecular Subtypes
Among all the participants, 88.2% (105/119) had a chromosome 15q11–q13 deletion, 5.04% (6/119) had UPD, 7.56% (9/119) had a UBE3A variant, and 0.8% (1/119) had ID. Table 3 shows the distribution of GQs and the subscale quotients by molecular subgroup. The non-deletion group had higher GQs and quotients for the locomotor, personal-social, and performance subscales (all P < 0.05); however, there was no significant difference in the quotients for the language and eye-hand coordination subscales (all P > 0.05).
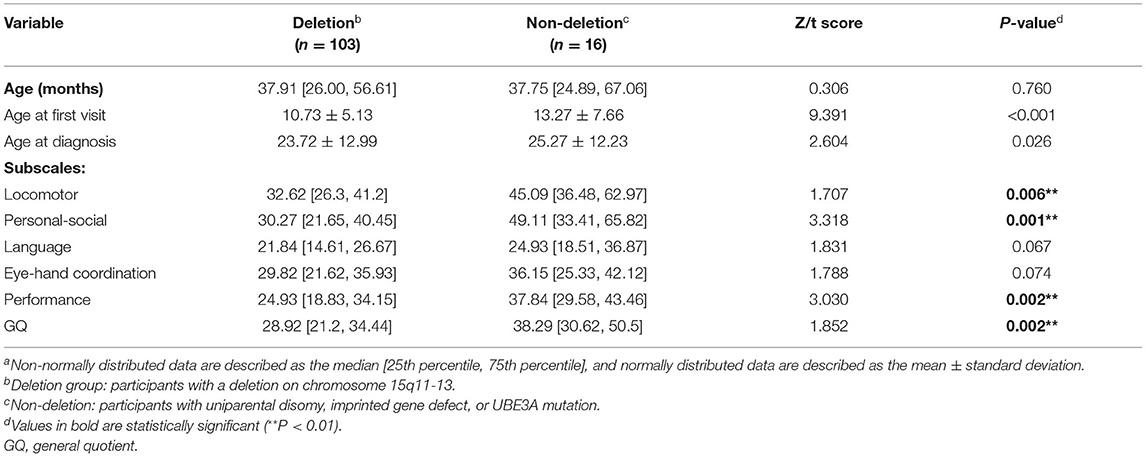
Table 3. Correlation between neurodevelopment and molecular subtypesa.
Correlation Between Neurodevelopment and Epilepsy
During this study, 79.8% (95/119) of patients reported clinical seizures. We selected 30 age-matched patients without epilepsy. As shown in Table 4, there were differences in DQs among four subscales (i.e., locomotor scale, personal-social scale, speech and language, and eye-hand coordination) and GQs (all P < 0.05). There was no significant difference in the quotients for the performance subscale. The parents of six children reported developmental regression after the seizure; this regression was reflected in the movement of five children.
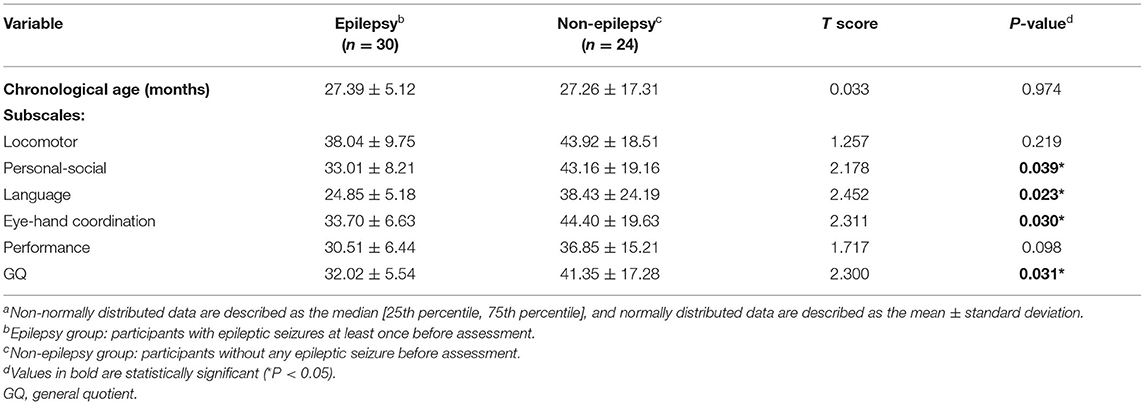
Table 4. Correlation between neurodevelopment and epilepsya.
Correlation Between Neurodevelopment and Sex
The correlation between neurodevelopment and sex for participants in the deletion group was analyzed to rule out the influence of the molecular type. As shown in Table 5, there was no significant difference in neurodevelopment between the two sex groups.
Discussion
The neurodevelopment of patients with AS is often difficult to evaluate; therefore, its characteristics and influencing factors have not been completely elucidated. The results of this study indicated that children with AS in China experience severe neurodevelopmental deterioration. In addition to age, molecular subtypes and the onset of seizures also correlate with the intellectual development of these patients. To the best of our knowledge, this is the largest cross-sectional study to use the GMDS-C to assess the development of children with AS; furthermore, it is also the first to collect objective developmental data of AS patients in China. Our data showed that 99.2% of the participants had GMDS-C GQs <70, thus indicating severe neurodevelopmental delay. Furthermore, the 95% confidence interval of the GMDS-C GQ ranged from 28.6 to 33.25 points. Such low values generally do not appear with developmental delays caused by exogenous factors, such as premature birth, infection, trauma, and extreme malnutrition (35, 36). Additionally, similar to the findings of other studies (5, 37), there was an imbalance in cognitive development; the participants had relatively strong locomotor and personal-social skills but weak language skills. These findings indicate that clinicians should be alerted to consider a diagnosis of AS if the patient has a declining GQ of ~29.6 points according to the GMDS-C, has good locomotor and personal-social skills, and has poor language skills.
Regarding the developmental age, 88.4% of the participants had a developmental age within 24 months, whereas the other 11.6% had a developmental age older than 24 months for at least one subscale; the highest developmental age was 47.5 months (observed using the locomotor scale). This age is older than that observed during a 2001 study that also used the GMDS to assess 20 children with AS (age 2–14 years) (38) and reported that all the participants had developmental ages younger than 24 months. This difference may reflect an improvement in the understanding of AS; previous diagnoses and rehabilitation may increase the upper limit of the developmental level, which needs to be confirmed by further research. Moreover, the results may vary by the tool used for the evaluation, the population, and the culture (31). A 2021 study that used Bayley-III showed that children with AS demonstrated skills when their developmental age was approximately 14 to 27 months, but their actual age was 6 years (14). Language skills are not a major factor in the GMDS-C assessment, thus allowing children with AS to perform more tasks despite their lack of language skills. The clinical symptoms of children with AS are mainly caused by the UBE3A gene loss of function, which has been well-confirmed in mouse models (39). The pathogenesis of AS is unclear, while diffusion tensor imaging of the brain has indicated defects in language pathways (40, 41), and motor impairments may be associated with cerebellar dysfunction and defects in motor cortex and nigrostriatal pathways (42, 43).
A linear analysis indicated an overall reduction in the GQ and quotient for all subscales with increasing age, indicating the progressive neurodevelopmental deterioration of children with AS, consistent with clinical observations (43, 44). The reduction was more obvious in the younger group and was decreased in the older group. Although we did not include patients older than 8 years, the downtrend also indicates changing trends, thus helping to predict the subsequent development of AS. It seemed that children with AS continued to develop higher skill levels over time, but at a slower rate. A 2021 study showed that the developmental skills of children with AS continued to improve until at least 12 years of age (14).
We verified that individuals with deletions in the 15q11–13 chromosome experience worse development, consistent with the results of prior studies (45). A non-parametric test showed lower GQs and quotients for sports, personal-social, and performance subscales in the deletion group than in the non-deletion group. This difference may be attributable to the deficiency of GABAA receptor subunit genes in the 15q11–13 region other than UBE3A, which has been associated with epilepsy and developmental delay (46). Interestingly, the two molecular subgroups did not show significant differences in language and eye-hand coordination. However, we believe that if the sample size had been sufficiently large, then the scores for all subscales would have been significantly different between the two groups. Nevertheless, it still suggested that the scores of these two subscales should not be used as criteria to distinguish between the two molecular subgroups. A study of 22 individuals with mosaic AS showed that the most prominent characteristic is the preservation of the ability to express language (47), indicating that UBE3A may be crucial to the development of brain regions that control this ability.
In addition to the molecular subtype, epilepsy, which is a common symptom of AS, also influences the cognition of patients with AS. The t-test results showed that the epilepsy group had worse GQ and quotients for the locomotor, personal-society, eye-hand coordination, and performance subscales. Additionally, five of our participants developed significant regression of development milestones after epileptic seizures; however, their abilities gradually improved after the seizures were controlled. This is intuitive evidence that epilepsy may affect the development of children with AS. Although there is currently no radical treatment for AS, therapies focused on reducing the severity of seizures and minimizing their frequency could be beneficial for the neurodevelopment of children with AS (16, 48). A 2008 study showed that one-fourth of patients with epilepsy have ID, and that one-fifth of patients with ID have epilepsy (49). Therefore, further research is needed to clarify whether worse development itself is a high-risk factor for seizures occurring in patients with AS.
Although AS was first described in 1965, the few descriptive studies performed in China include small samples (20, 50). The interval between diagnosis and the first evaluation was almost 18 months during this study, which is unfavorable for early rehabilitation and family genetic counseling. Many studies have explored different methods of gene therapy for curing AS (51, 52). Regarding assessments, the GMDS-C is more suitable because it can be applied to a broader age range, provides a detailed assessment of different aspects of development, has low reliance on language, and can be adapted to the Chinese culture. Therefore, the findings of this study provide basic evidence for the use of the GMDS-C to establish the baseline natural history of AS.
This study had some limitations. The use of a cross-sectional design made it difficult to represent the development trends of the same groups according to age. Additionally, controlled trials and a longitudinal cohort study are needed to provide clinical guidance. Furthermore, the number of participants in the non-deletion group was limited, thus making it difficult to compare different subtypes (UBE3A mutation, UPD, and ID) in this group; however, this will be an important focus of our research in the future.
In conclusion, our study uses the GMD-S scale, which is well-established in China, to fill the gap in the assessment of the baseline level of the natural developmental history of AS. It was confirmed that molecular subtypes and age influence the intellectual development of children with AS, and our results further indicated that epileptic seizures cause secondary developmental regression; therefore, epilepsy control is important for development. These results may provide useful endpoints for clinical trials that can be performed to evaluate the development of drugs and other treatments for AS.
Data Availability Statement
The original contributions presented in the study are included in the article/supplementary material, further inquiries can be directed to the corresponding author/s.
Ethics Statement
The studies involving human participants were reviewed and approved by Ethical Institutional Review Committee of Children's Hospital of Fudan University. Written informed consent to participate in this study was provided by the participants' legal guardian/next of kin.
Author Contributions
SL, XD, and YW contributed to developing the concept of the manuscript. SL wrote the first draft of the manuscript. SL, XD, and HJ participated in the Griffith assessments. YM and TW participated in the collection and analysis of data. All authors contributed to manuscript revision, read, and approved the submitted version.
Funding
This work was supported by the Shanghai Municipal Science and Technology Major Project (Grant No. 2017SHZDZX01), Shanghai Key Laboratory of Birth Defects, Shanghai, 201102, China (Grant No. 13DZ2260600), Shanghai Municipal Science and Technology Major Project (Grant No. 2018SHZDZX03), and Omics-based precision medicine of epilepsy being entrusted by Key Research Project of the Ministry of Science and Technology of China (Grant No. 2016YFC0904400).
Conflict of Interest
The authors declare that the research was conducted in the absence of any commercial or financial relationships that could be construed as a potential conflict of interest.
Publisher's Note
All claims expressed in this article are solely those of the authors and do not necessarily represent those of their affiliated organizations, or those of the publisher, the editors and the reviewers. Any product that may be evaluated in this article, or claim that may be made by its manufacturer, is not guaranteed or endorsed by the publisher.
Acknowledgments
We sincerely thank all the patients and their parents who participated in the study, and the clinicians involved in their recruitment and evaluation efforts. Thank to Xue Zhang and the Chinese Angelman Syndrome Organization for their assistance in recruiting and organizing patients. We would like to thank Editage (www.editage.cn) for English language editing.
References
1. Mertz LGB, Christensen R, Vogel I, Hertz JM, Nielsen KB, Grønskov K, et al. Angelman syndrome in Denmark. birth incidence, genetic findings, and age at diagnosis. Am J Med Genet A. (2013) 161A:2197–203. doi: 10.1002/ajmg.a.36058
2. Yakoreva M, Kahre T, Žordania R, Reinson K, Teek R, Tillmann V, et al. A retrospective analysis of the prevalence of imprinting disorders in Estonia from 1998 to 2016. Eur J Hum Genet. (2019) 27:1649–58. doi: 10.1038/s41431-019-0446-x
3. Williams CA, Beaudet AL, Clayton-Smith J, Knoll JH, Kyllerman M, Laan LA, et al. Angelman syndrome 2005: updated consensus for diagnostic criteria. Am J Med Genet A. (2006) 140:413–8. doi: 10.1002/ajmg.a.31074
4. Frohlich J, Miller MT, Bird LM, Garces P, Purtell H, Hoener MC, et al. Electrophysiological phenotype in angelman syndrome differs between genotypes. Biol Psychiatry. (2019) 85:752–9. doi: 10.1016/j.biopsych.2019.01.008
5. Bird LM. Angelman syndrome: review of clinical and molecular aspects. Appl Clin Genet. (2014) 7:93–104. doi: 10.2147/TACG.S57386
6. Gu B, Carstens KE, Judson MC, Dalton KA, Rougié M, Clark EP, et al. Ube3a reinstatement mitigates epileptogenesis in Angelman syndrome model mice. J Clin Invest. (2019) 129:163–8. doi: 10.1172/JCI120816
7. Lopez SJ, Segal DJ, LaSalle JM. UBE3A: An E3 Ubiquitin ligase with genome-wide impact in neurodevelopmental disease. Front Mol Neurosci. (2018) 11:476. doi: 10.3389/fnmol.2018.00476
8. Buiting K, Clayton-Smith J, Driscoll DJ, Gillessen-Kaesbach G, Kanber D, Schwinger E, et al. \Clinical utility gene card for: Angelman syndrome. Eur J Hum Genet. (2015) 23:93. doi: 10.1038/ejhg.2014.93
9. Keute M, Miller MT, Krishnan ML, Sadhwani A, Chamberlain S, Thibert RL, et al. Angelman syndrome genotypes manifest varying degrees of clinical severity and developmental impairment. Mol Psychiatry. 26:3625–33. doi: 10.1038/s41380-020-0858-6
10. Gentile JK, Tan W-H, Horowitz LT, Bacino CA, Skinner SA, Barbieri-Welge R, et al. A neurodevelopmental survey of Angelman syndrome with genotype-phenotype correlations. J Dev Behav Pediatr. (2010) 31:592–601. doi: 10.1097/DBP.0b013e3181ee408e
11. Micheletti S, Palestra F, Martelli P, Accorsi P, Galli J, Giordano L, et al. Neurodevelopmental profile in Angelman syndrome: more than low intelligence quotient. Ital J Pediatr. (2016) 42:91. doi: 10.1186/s13052-016-0301-4
12. Tan W-H, Bacino CA, Skinner SA, Anselm I, Barbieri-Welge R, Bauer-Carlin A, et al. Angelman syndrome: mutations influence features in early childhood. Am J Med Genetics Part A. (2011) 155:81–90. doi: 10.1002/ajmg.a.33775
13. Varela MC, Kok F, Otto PA, Koiffmann CP. Phenotypic variability in Angelman syndrome: comparison among different deletion classes and between deletion and UPD subjects. Eur J Hum Genet. (2004) 12:987–92. doi: 10.1038/sj.ejhg.5201264
14. Sadhwani A, Wheeler A, Gwaltney A, Peters SU, Barbieri-Welge RL, Horowitz LT, et al. Developmental skills of individuals with angelman syndrome assessed using the Bayley-III. J Autism Dev Disord. (2021) 30:1–18. doi: 10.1007/s10803-020-04861-1
15. Thibert RL, Conant KD, Braun EK, Bruno P, Said RR, Nespeca MP, et al. Epilepsy in Angelman syndrome: a questionnaire-based assessment of the natural history and current treatment options. Epilepsia. (2009) 50:2369–76. doi: 10.1111/j.1528-1167.2009.02108.x
16. Samanta D. Epilepsy in Angelman syndrome: a scoping review. Brain Dev. (2021) 43:32–44. doi: 10.1016/j.braindev.2020.08.014
17. Sun AX, Yuan Q, Fukuda M, Yu W, Yan H, Lim GGY, et al. Potassium channel dysfunction in human neuronal models of Angelman syndrome. Science. (2019) 366:1486–92. doi: 10.1126/science.aav5386
18. Ohtsuka Y, Kobayashi K, Yoshinaga H, Ogino T, Ohmori I, Ogawa K, et al. Relationship between severity of epilepsy and developmental outcome in Angelman syndrome. Brain Dev. (2005) 27:95–100. doi: 10.1016/j.braindev.2003.09.015
19. Bindels-de Heus KGCB, Mous SE, Ten Hooven-Radstaake M, van Iperen-Kolk BM, Navis C, Rietman AB, et al. An overview of health issues and development in a large clinical cohort of children with Angelman syndrome. Am J Med Genet A. (2020) 182:53–63. doi: 10.1002/ajmg.a.61382
20. Luk HM, Lo IFM. Angelman syndrome in Hong Kong Chinese: a 20 years' experience. Eur J Med Genet. (2016) 59:315–9. doi: 10.1016/j.ejmg.2016.05.003
21. Liu C, Zhang X, Wang J, Zhang Y, Wang A, Lu J, et al. Genetic testing for Prader-Willi syndrome and Angelman syndrome in the clinical practice of Guangdong Province, China. Mol Cytogenet. (2019) 12:7. doi: 10.1186/s13039-019-0420-x
22. Elgersma Y, Sonzogni M. UBE3A reinstatement as a disease-modifying therapy for Angelman syndrome. Dev Med Child Neurol. (2021) 63:802–7. doi: 10.1111/dmcn.14831
23. Na SD, Burns TG. Wechsler intelligence scale for children-V: test review. Appl Neuropsychol Child. (2016) 5:156–60. doi: 10.1080/21622965.2015.1015337
24. Janssen AJWM, Oostendorp RAB, Akkermans RP, Steiner K, Kollée LAA. Nijhuis-van der Sanden MWG. High variability of individual longitudinal motor performance over 5 years in very preterm infants. Res Dev Disabil. (2016) 59:306–17. doi: 10.1016/j.ridd.2016.09.017
25. Otake Y, Nakajima S, Uno A, Kato S, Sasaki S, Yoshioka E, et al. Association between maternal antenatal depression and infant development: a hospital-based prospective cohort study. Environ Health Prev Med. (2014) 19:30–45. doi: 10.1007/s12199-013-0353-7
26. Johnson S, Moore T, Marlow N. Using the Bayley-III to assess neurodevelopmental delay: which cut-off should be used? Pediatr Res. (2014) 75:670–4. doi: 10.1038/pr.2014.10
27. Velikos K, Soubasi V, Michalettou I, Sarafidis K, Nakas C, Papadopoulou V, et al. Bayley-III scales at 12 months of corrected age in preterm infants: patterns of developmental performance and correlations to environmental and biological influences. Res Dev Disabil. (2015) 45–46:110–9. doi: 10.1016/j.ridd.2015.07.014
28. Li HH, Wang CX, Feng JY, Wang B, Li CL, Jia FY, et al. Developmental profile of children with autism spectrum disorder in china using the griffiths mental development scales. Front Psychol. (2020) 11:570923. doi: 10.3389/fpsyg.2020.570923
29. Cirelli I, Bickle Graz M, Tolsa J-F. Comparison of Griffiths-II and Bayley-II tests for the developmental assessment of high-risk infants. Infant Behav Dev. (2015) 41:17–25. doi: 10.1016/j.infbeh.2015.06.004
30. Luiz DM, Foxcroft CD, Stewart R. The construct validity of the Griffiths scales of mental development. Child Care Health Dev. (2001) 27:73–83. doi: 10.1046/j.1365-2214.2001.00158.x
31. Tso WWY, Wong VCN, Xia X, Faragher B, Li M, Xu X, et al. The Griffiths development scales-Chinese (GDS-C): a cross-cultural comparison of developmental trajectories between Chinese and British children. Child Care Health Dev. (2018) 44:378–83. doi: 10.1111/cch.12548
32. Li PY, Fu NN, Li QY, Wang GF, Gao L, Zhang X. The Griffiths Development Scales-Chinese (GDS-C): a reliable and valid neurodevelopmental assessment tool in children with ASD aged 3–8 years old in Tianjin, China. Asian J Psychiatr. (2020) 52:102144. doi: 10.1016/j.ajp.2020.102144
33. Dykens EM, Hodapp RM, Evans DW. Profiles and development of adaptive behavior in children with Down syndrome. Am J Ment Retard. (1994) 98:580–7.
34. Roberts JE, Mankowski JB, Sideris J, Goldman BD, Hatton DD, Mirrett PL, et al. Trajectories and predictors of the development of very young boys with fragile X syndrome. J Pediatr Psychol. (2009) 34:827–36. doi: 10.1093/jpepsy/jsn129
35. Vissers LELM, Gilissen C, Veltman JA. Genetic studies in intellectual disability and related disorders. Nat Rev Genet. (2016) 17:9–18. doi: 10.1038/nrg3999
36. Mithyantha R, Kneen R, McCann E, Gladstone M. Current evidence-based recommendations on investigating children with global developmental delay. Arch Dis Child. (2017) 102:1071–6. doi: 10.1136/archdischild-2016-311271
37. Mertz LGB, Thaulov P, Trillingsgaard A, Christensen R, Vogel I, Hertz JM, et al. Neurodevelopmental outcome in Angelman syndrome: genotype-phenotype correlations. Res Dev Disabil. (2014) 35:1742–7. doi: 10.1016/j.ridd.2014.02.018
38. Andersen WH, Rasmussen RK, Strømme P. Levels of cognitive and linguistic development in Angelman syndrome: a study of 20 children. Logoped Phoniatr Vocol. (2001) 26:2–9. doi: 10.1080/14015430117324
39. Bakke KA, Howlin P, Retterstøl L, Kanavin ØJ, Heiberg A, Nærland T. Effect of epilepsy on autism symptoms in Angelman syndrome. Mol Autism. Available online at: https://www.ncbi.nlm.nih.gov/pmc/articles/PMC5759870/ doi: 10.1186/s13229-017-0185-1 (accessed 2019 25, March).
40. Buiting K, Williams C, Horsthemke B. Angelman syndrome - insights into a rare neurogenetic disorder. Nat Rev Neurol. (2016) 12:584–93. doi: 10.1038/nrneurol.2016.133
41. Mabb AM, Judson MC, Zylka MJ, Philpot BD. Angelman syndrome: insights into genomic imprinting and neurodevelopmental phenotypes. Trends Neurosci. (2011) 34:293–303. doi: 10.1016/j.tins.2011.04.001
42. Wilson BJ, Sundaram SK, Huq AHM, Jeong JW, Halverson SR, Behen ME, et al. Abnormal language pathway in children with Angelman syndrome. Pediatr Neurol. (2011) 44:350–6. doi: 10.1016/j.pediatrneurol.2010.12.002
43. Castro-Gago M, Gómez-Lado C, Eirís-Puñal J, Rodríguez-Mugico VM. Abnormal myelination in Angelman syndrome. Eur J Paediatr Neurol. (2010) 14:292. doi: 10.1016/j.ejpn.2009.07.007
44. Bruinsma CF, Schonewille M, Gao Z, Aronica EMA, Judson MC, Philpot BD, et al. Dissociation of locomotor and cerebellar deficits in a murine Angelman syndrome model. J Clin Invest. (2015) 125:4305–15. doi: 10.1172/JCI83541
45. Sahoo T, Peters SU, Madduri NS, Glaze DG, German JR, Bird LM, et al. Microarray based comparative genomic hybridization testing in deletion bearing patients with Angelman syndrome: genotype-phenotype correlations. J Med Genet. (2006) 43:512–6. doi: 10.1136/jmg.2005.036913
46. Hernandez CC, Macdonald RL. A structural look at GABAA receptor mutations linked to epilepsy syndromes. Brain Res. (2019) 1714:234–47. doi: 10.1016/j.brainres.2019.03.004
47. Carson RP, Bird L, Childers AK, Wheeler F, Duis J. Preserved expressive language as a phenotypic determinant of Mosaic Angelman syndrome. Mol Genet Genomic Med. (2019) 7:e837. doi: 10.1002/mgg3.837
48. Doran Z, Shankar R, Keezer MR, Dale C, McLean B, Kerr MP, et al. Managing anti-epileptic drug treatment in adult patients with intellectual disability: a serious conundrum. Eur J Neurol. (2016) 23:1152–7. doi: 10.1111/ene.13016
49. Shankar R, Rowe C, Van Hoorn A, Henley W, Laugharne R, Cox D, et al. Under representation of people with epilepsy and intellectual disability in research. PLoS ONE. (2018) 13:e0198261. doi: 10.1371/journal.pone.0198261
50. Bai JL, Qu YJ, Jin YW, Wang H, Yang YL, Jiang YW, et al. Molecular and clinical characterization of Angelman syndrome in Chinese patients. Clin Genet. (2014) 85:273–7. doi: 10.1111/cge.12155
51. Rotaru DC, Mientjes EJ, Elgersma Y. Angelman syndrome: from mouse models to therapy. Neuroscience. (2020) 445:172–89. doi: 10.1016/j.neuroscience.2020.02.017
Keywords: Angelman syndrome, neurodevelopment, GMDS-C, children, epilepsy
Citation: Li S, Ma Y, Wang T, Jin H, Du X and Wang Y (2022) Epilepsy and Molecular Phenotype Affect the Neurodevelopment of Pediatric Angelman Syndrome Patients in China. Front. Psychiatry 13:886028. doi: 10.3389/fpsyt.2022.886028
Received: 28 February 2022; Accepted: 08 April 2022;
Published: 28 April 2022.
Edited by:
Roberto Canitano, Siena University Hospital, ItalyReviewed by:
Roberto Palumbi, University of Bari Aldo Moro, ItalyHong-Hua Li, Jilin University, China
Copyright © 2022 Li, Ma, Wang, Jin, Du and Wang. This is an open-access article distributed under the terms of the Creative Commons Attribution License (CC BY). The use, distribution or reproduction in other forums is permitted, provided the original author(s) and the copyright owner(s) are credited and that the original publication in this journal is cited, in accordance with accepted academic practice. No use, distribution or reproduction is permitted which does not comply with these terms.
*Correspondence: Xiaonan Du, ZHJlbWVkdUAxMjYuY29t; Yi Wang, eWl3YW5nQHNobXUuZWR1LmNu