- Department of Pharmacology, Institute of Neuroscience and Physiology, The Sahlgrenska Academy at the University of Gothenburg, Gothenburg, Sweden
Alcohol use disorder (AUD) is often described as repeated phases of binge drinking, compulsive alcohol-taking, craving for alcohol during withdrawal, and drinking with an aim to a reduce the negative consequences. Although multifaceted, alcohol-induced reward is one aspect influencing the former three of these. The neurobiological mechanisms regulating AUD processes are complex and one of these systems is the gut-brain peptide ghrelin. The vast physiological properties of ghrelin are mediated via growth hormone secretagogue receptor (GHSR, ghrelin receptor). Ghrelin is well known for its ability to control feeding, hunger, and metabolism. Moreover, ghrelin signaling appears central for alcohol-mediated responses; findings reviewed herein. In male rodents GHSR antagonism reduces alcohol consumption, prevents relapse drinking, and attenuates the motivation to consume alcohol. On the other hand, ghrelin increases the consumption of alcohol. This ghrelin-alcohol interaction is also verified to some extent in humans with high alcohol consumption. In addition, either pharmacological or genetic suppression of GHSR decreases several alcohol-related effects (behavioral or neurochemical). Indeed, this suppression blocks the alcohol-induced hyperlocomotion and dopamine release in nucleus accumbens as well as ablates the alcohol reward in the conditioned place preference model. Although not fully elucidated, this interaction appears to involve areas central for reward, such as the ventral tegmental area (VTA) and brain nodes targeted by VTA projections. As reviewed briefly, the ghrelin pathway does not only modulate alcohol-mediated effects, it regulates reward-related behaviors induced by addictive drugs. Although personality traits like impulsivity and risk-taking behaviors are common in patients with AUD, the role of the ghrelin pathway thereof is unknown and remains to be studied. In summary, the ghrelin pathway regulates addiction processes like AUD and therefore the possibility that GHSR antagonism reduces alcohol or drug-taking should be explored in randomized clinical trials.
1. Introduction
In society harmful alcohol consumption is a global health problem, where alcohol use disorder (AUD) is a top pathology associated with this risky consumption (1). The health problems and socioeconomic burden of AUD for individuals and society in large are major concerns (2, 3). AUD is often referred to a cycle of different phases that are repeated over time [for review see Koob (4)]. Alcohol-induced reward is one central aspect influencing phases like the initial binge phase, the latter compulsive alcohol-taking and craving for alcohol during withdrawal. Besides these, drinking with an aim to a reduce the negative consequences is another important phase. An AUD diagnosis is defined according to a set classification system which includes parameters such as loss of intake control, persistent desire, craving and tolerance. Although not a part of the AUD diagnosis, both risk taking and impulsivity are common characteristics observed in patients with AUD (5). A complex disease like AUD involves a vast number of underlying pathways and neurobiological substrates which are being identified by means of both preclinical and clinical research. During latter years gut-brain peptides appear to be important players for the AUD process, possibly due to an interference with alcohol-induced reward [for review see Jerlhag (6)]. To date, multiple gut-brain peptides exist and ghrelin is one of these.
The stomach-derived hormone ghrelin (acyl-ghrelin) exerts its physiological effects via activation of growth hormone secretagogue receptor (GHSR), a receptor known for its intrinsic activity and ability to heterodimer with other receptors [for review see Cornejo et al. (7)]. The receptor is today often referred to the ghrelin receptor, and earned its initial name from the growth hormone releasing ability after activation of the receptor (8). Ghrelin is to date the only identified orexigenic (appetite promoting) gut-brain peptide (8). Notably, the feeding aspects influenced by ghrelin include both the hedonic and homeostatic (7, 9). The homeostatic properties of ghrelin are further evident as it promotes hunger, appetite and body weight gain [for review see Cornejo et al. (7)]. Ghrelin also has a myriad of other physiological properties as it for instance regulates secretion of gastric acid and gastric motility [for review see Cornejo et al. (7)]. This review summarizes the available research on if and how the ghrelin pathway modulates alcohol-related effects (behaviors and neurochemistry) in animals. It should be noted that there are no clinically available GHSR ligands, but for research purposes antagonists like JMV2959 and [D-Lys3]-GHRP-6 and the inverse agonist PF-5190457 are used. The name of these have been omitted throughout the review to increase the readability of the review. First, the ability of ghrelin signaling to modulate different aspects of alcohol drinking is introduced. Then the review will cover the effect of genetic or pharmacological suppression of its receptor on the rewarding aspects of alcohol. Further, this reward reduction is suggested as a tentative explanation to why the alcohol consumption is reduced after GHSR suppression. Thereafter, brain regions and neurocircuits central for the ghrelin-alcohol interaction will be introduced. The review will then briefly cover the role of ghrelin pathway in drug taking. Moreover, confounding factors are described. On a final note, future directions are discussed and the clinical trial testing the effect of GHSR antagonists/inverse agonists on alcohol drinking in AUD patients is suggested as a concluding remark.
The reviewed articles presented herein were selected by means of keywords and the included articles were quality checked before inclusion (Table 1). As this is a review rather than systematic overview, the presented articles were thus not selected using PICOS, PRISM, Cochrane, or JBI. A systematic overview provides a higher reliability, and the lack of such design should be considered as a limitation. It should also be noted that previous reviews cover aspects of this research field. In these, several gut-rain peptides are introduced, the clinical aspects/data or alcohol-associated liver disease are introduced in detail [examples of other reviews within the field (10–14)]. The novelty of this review is the detail insight into available preclinical studies, where the link between ghrelin-pathway and alcohol-related effects is described in detail. Moreover, novel future directions are discussed.
2. The role of the ghrelin pathway on alcohol-related effects in animals
2.1. Inhibition of the ghrelin pathway reduces various alcohol drinking behaviors in rodents
The ability of GHSR antagonists to reduce alcohol drinking has been shown in numerous preclinical studies (Figure 1). It was initially found that acute administration of a GHSR antagonist lowers alcohol intake in male mice (15), high alcohol preferring male rats (16) or male rats consuming alcohol for long periods of time (17). Notably, a dose-dependent reduction was evident after repeated injections of a GHSR antagonist (17). The findings that the decline in alcohol drinking is greater at seven compared to three months of alcohol exposure (17) are interesting from clinical perspective. Indeed, it is plausible that a GHSR antagonist would reduce alcohol drinking more in patients with severe AUD compared to those with a mild AUD. The lack of tolerance toward the GHSR antagonist in drinking experiments (17) is further clinically beneficial, as it indicates that there would not be a dose adjustment during treatment. Although these initial findings report that GHSR antagonists are effective in rodents consuming alcohol for extensive periods of time prior to treatment, the ability of an antagonist to reduce drinking is also evident in animals consuming alcohol for shorter periods of time. On this note, male mice/rats/prairie voles exposed to alcohol shortly before treatment display a decline in alcohol consumption after acute or repeated treatment with a GHSR antagonist (18–22). The ability of GHSR to regulate alcohol consumption is further evident as alcohol drinking is lower in GHSR knockout male mice (15) or rats (23) compared to wild-type littermates. From a mechanistic perspective, vagal afferents appear to be involved in the ability of a GHSR antagonist to reduce alcohol intake in male rats (24).
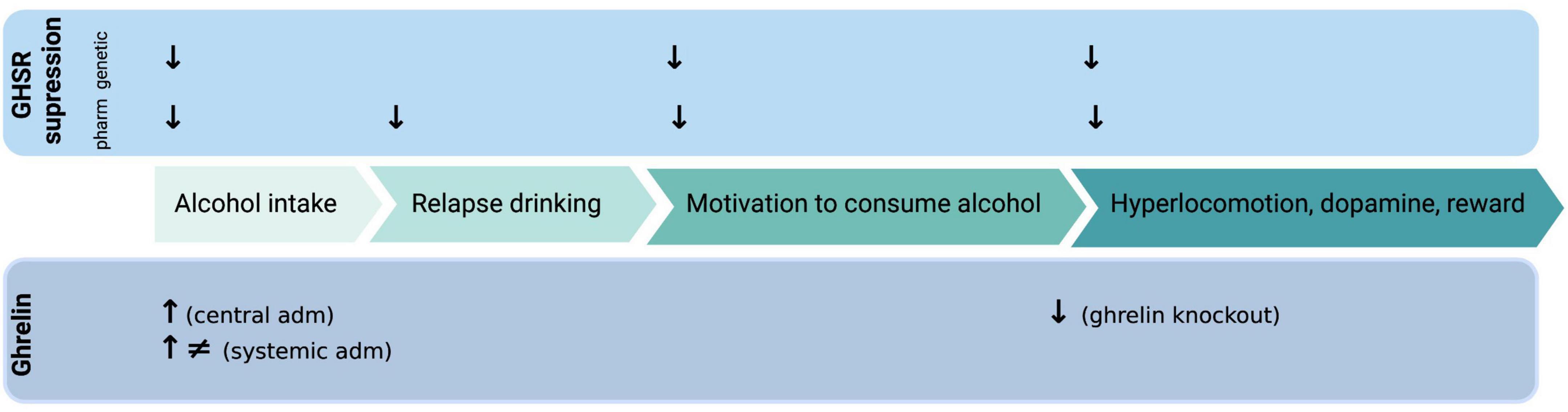
Figure 1. Illustrative summary of available preclinical studies on how ghrelin administration (adm) or genetic/pharmacologic (pharm) GHSR (growth hormone receptor, ghrelin receptor) suppression influences various alcohol-related responses. ↓, decreases; ↑, increases; ≠, no effect. Created with BioRender.com.
In support for these initial findings where a decline in alcohol drinking after GHSR inhibition is reported, are additional studies demonstrating a beneficial role of GHSR antagonists on other alcohol drinking behaviors. One of these is relapse drinking after abstinence, a trait blocked by a GHSR antagonist in male mice (15) and male rats (17). These findings may be intriguing from a clinical perspective as a declined relapse drinking may reflect a reduction in alcohol craving, which is observed in humans during relapse. Moreover, pharmacological (16, 19) or genetic (23) suppression of the GHSR attenuates the motivation to consume alcohol in the operant self-administration paradigm in male rats. As for regular alcohol intake, the ability of a GHSR antagonist to reduce self-administration is persistent in animals exposed to alcohol for long or short periods of time before treatment (16, 19, 23). Moreover, this reduction is also observed in female rats treated with a GHSR antagonist into the nucleus accumbens (NAc) (25). Together these findings indicate that GHSR regulate aspects of the AUD process such as escalated intake, craving for alcohol and motivation to consume alcohol. A clinical relevance is provided as polymorphisms of the GHSR gene is associated with high alcohol intake or AUD diagnosis (26–28). Moreover, in patients with AUD an inverse GHSR-1A agonist reduces self-reported alcohol craving (29) as well as decreases hangover symptoms after intravenous self-administration of alcohol (30). Collectively, these studies imply that GHSR are required for various alcohol drinking behaviors. However, individuals drink alcohol due to various reasons where impulsivity and risk-taking are important features. Upcoming studies should therefore explore the role of the ghrelin pathway for such characteristics in patients with AUD.
Opposed to the clear outcome on alcohol drinking by the GHSR antagonists administered into the brain or systemically, the role of central versus peripheral ghrelin is unclear. An initial study reveals that male mice with an overall ghrelin knockout drink less alcohol compared to their wild-type littermates (18). Moreover, ghrelin administration into the ventricles of the brain increases alcohol intake in male mice consuming alcohol for months before the ghrelin infusion (15). An effect also observed after a systemic administration in one study of male rats (31), but not in another study of male mice (32) exposed to alcohol for a short-period of time. Moreover, neutralization of circulating ghrelin does not influence alcohol intake in male rats drinking alcohol for months prior to treatment (33). The reasons for these diverging results remain to be determined, but may be due to parameters such as different drinking models, time of alcohol exposure prior to test or animals/strain used. The role of circulating ghrelin for AUD processes is further discussed as circulating levels of ghrelin are reduced by acute alcohol in male rats (34, 35) and enhanced by alcohol drinking (36). Whereas another study reveals no differences in plasma ghrelin between low and high alcohol-preferring rats (16). The association between ghrelin and alcohol in humans is extensively reviewed elsewhere (6). In brief, in patients with AUD the high plasma levels of ghrelin is associated with the craving for alcohol (37–41), possibly involving the ventral striatum (41). An area also central for ghrelin-alcohol interaction in animals (25). On the same note, in patients with AUD intravenous infusion of a high dose of ghrelin declines the latency to first alcohol containing drink (42) and elevates alcohol craving in patients with AUD (43). Besides, polymorphisms in the pre-pro-ghrelin gene are associated with aspects of the AUD diagnosis (28, 44, 45).
Collectively, these findings raise the discussion whether circulating or central ghrelin signaling is required for a modulation of the alcohol-related effects, an aspect that should be addressed in upcoming studies. It should also be noted that the divergence between central and peripheral signaling exist when it comes to another gut-brain peptide; glucagon-like peptide-1 (GLP-1). Indeed, central, but not peripheral GLP-1 receptors appear to be important for the ability of a GLP-1 receptor agonist to attenuate alcohol-related responses in male mice (46).
2.2. Suppression of the ghrelin pathway attenuates the alcohol-related responses (behavioral or neurochemical) in rodents
The decline in alcohol drinking by GHSR antagonists in animals exposed to long or short-term alcohol may be due to a reduction in alcohol’s rewarding experience as this also is reduced by GHSR antagonism (Figure 1). A feature essential for the several phase of the addiction cycle (4) and associated to the development of AUD later in life (47). In animal models alcohol reward can be measured by its ability to activate the mesolimbic dopamine system, consisting of dopamine projections from the ventral tegmental area (VTA) to the NAc shell [for review see Jayaram-Lindström et al. (48)]. Specifically, alcohol causes a locomotor stimulation, dopamine release in NAc shell and causes a conditioned place preference (CPP) in rodents that are alcohol naïve prior to test [for review see Jayaram-Lindstroöm et al. (48) and Sanchis-Segura and Spanagel (49)]. Importantly, the elevated dopamine in NAc shell is translated into human studies, where the dopamine release is positively correlated to the reward of alcohol [for review see Jayaram-Lindström et al. (48)]. It should however be noted that this correlation is influenced by factors such as sex (50), possibly due to alcohol’s ability to affect multiple neurotransmitters. When it comes to ghrelin pathway and alcohol reward, preclinical studies of male mice reveal that the ability of alcohol to cause a locomotor stimulation, dopamine release in NAc or CPP is blocked both by pharmacological or genetic suppression of the GHSR (15). A similar finding also observed after sub-chronic administration of a GHSR antagonist (51). On a similar note, these behavioral and neurochemical responses to alcohol are ablated in male mice with an overall ghrelin knockout (52). A finding later replicated as ghrelin knockout male mice do not display a CPP to alcohol (18). In mice using an overall ghrelin knockout the role of central versus peripheral ghrelin cannot be elucidated. In attempts to explore this, a study explored the alcohol responses after neutralization of circulating ghrelin (33). Intriguingly, neutralization of circulating ghrelin did not affect the alcohol-induced locomotor stimulation, dopamine release in NAc and CPP (33). As reviewed extensively elsewhere (10, 11, 13, 14), in humans (and rodents to some extent) associations between alcohol responses and plasma ghrelin have been found. Briefly, a high subjective intensity of alcohol is associated to circulating ghrelin levels (53). On a similar note, in a study with individuals self-administrating alcohol intravenously an intense subjective alcohol experience is positively associated with higher fasting ghrelin levels (54). Taken together, these studies imply that GHSR are required for alcohol to elicit reward and that the role of central versus peripheral ghrelin should be explored in detail.
2.3. Brain areas participating in the interaction between ghrelin and alcohol in rodents
Areas and circuits responsible for the ghrelin-alcohol interaction remain to be fully elucidated, but may involve areas central for reward (Figure 2). First of all, these brain regions express GHSR (55–57), indicating that these areas mediate ghrelin effects. When it comes to NAc, high alcohol intake elevates the GHSR expression in this area (17). Moreover, local infusion of a GHSR antagonist into the NAc blocks the operant self-administration of alcohol in female rats (25). A finding also apparent in humans, as the ability of ghrelin to cause craving for alcohol involves the ventral striatum (corresponding to NAc in rodents) (41). Furthermore, preclinical studies imply that GHSR within the VTA, an area interconnected with the NAc, may be central for the interaction of ghrelin pathway and alcohol. Initial studies demonstrate that local infusion of ghrelin into the VTA increases alcohol intake in male mice (15), and causes reward per se (51, 58–62). Similarly, male rats with short exposure to alcohol prior to treatment display elevated alcohol intake after ghrelin into the VTA (63). This interaction is further evident as high-alcohol consuming rats display higher expression levels of the GHSR in the VTA than low-alcohol consuming rats (17). However, this association was not replicated in a human study (64). Direct application of ghrelin into the VTA of male rodents increases the activity dopamine cells (65) and activates the mesolimbic dopamine system (59, 60, 65–68). Similarly, GHSR on dopamine neurons in the VTA controls brain stimulation reward induced by optogenetic stimulation (69). Within the VTA, a local dopamine release controlled by the GHSR possibly located on dopamine neurons (65) appears central for either ghrelin or alcohol to activate this reward pathway (68). On this note, the ability of GHSR to form a heterodimer with dopamine receptors regulate the activity of dopamine neurons (70–72). These findings collectively suggest that ghrelin and dopamine signaling interact within the VTA to control the ability of stimuli like alcohol to activate the mesolimbic dopamine system. NAc and VTA are bidirectionally interconnected and opposed to VTA-NAc dopamine projection the GABA projection from NAc to VTA does not regulate brain stimulation reward (69).
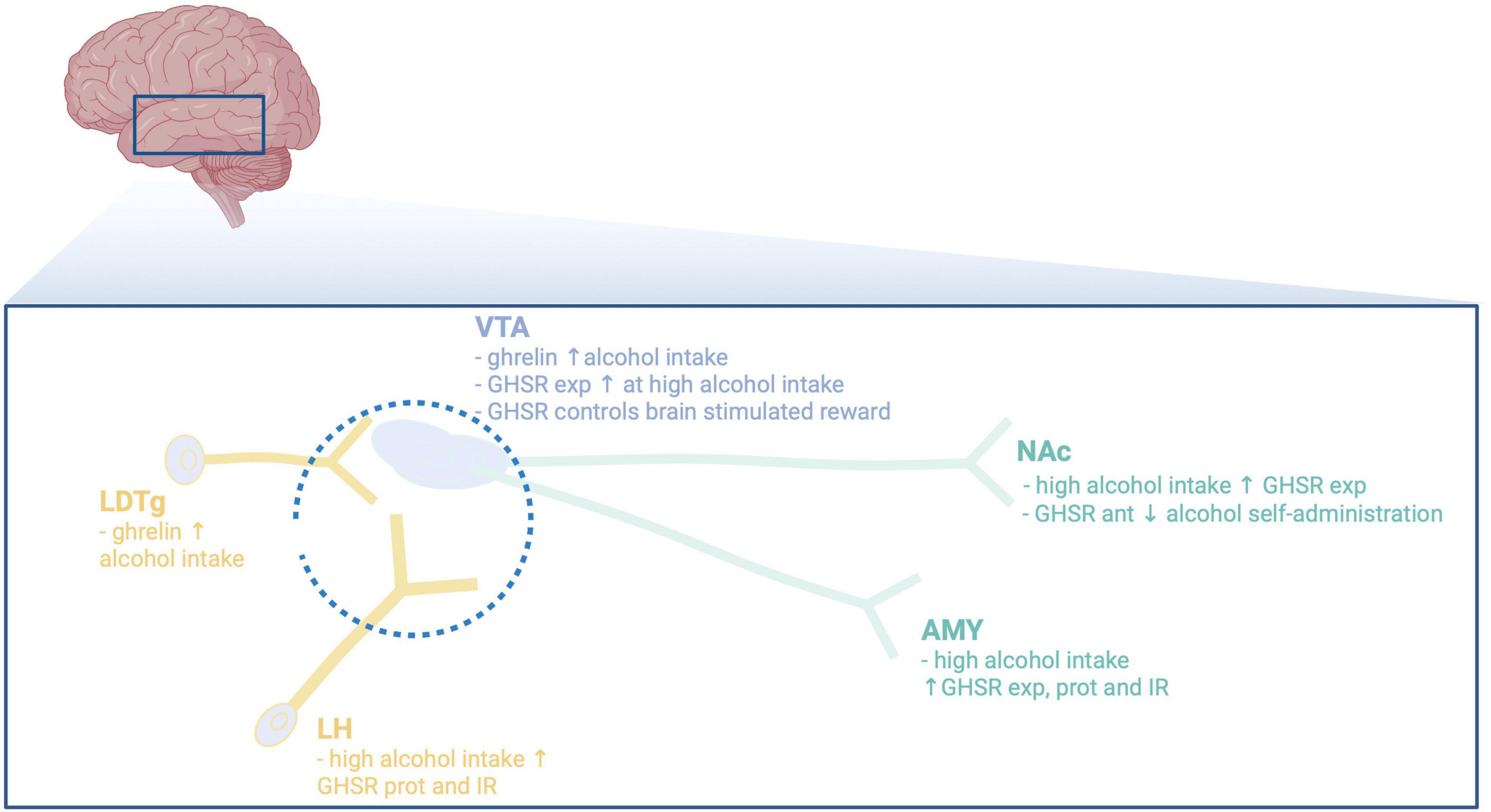
Figure 2. Illustrative summary of available preclinical studies on the main brain regions participating in the interaction between alcohol and the ghrelin pathway. VTA, ventral tegmental area; NAc, nucleus accumbens; LDTg, laterodorsal tegmental area; LH, lateral hypothalamus; AMY, amygdala; exp, expression; ant, antagonist; prot, protein; IR, immunoreactivity; ↓, decreases; ↑, increases. Created with BioRender.com.
Besides NAc, the VTA projects to reward-related areas like amygdala [for review see Jayaram-Lindström et al. (48)], or to Edinger–Westphal nucleus (73). When it comes to the role of GHSR in the amygdala (56, 74), both preclinical and clinical studies reveal that GHSR within this area modulate the alcohol response. Specifically, ghrelin elevates the amygdala levels of GABA in alcohol dependent or naïve male rats (34, 74). Moreover, in this area the protein levels of GHSR and the immunoreactive GHSR cells are elevated by high alcohol intake (34), as are the GHSR expression (17). In humans, ghrelin enhances the ability of alcohol-related cues to activate amygdala an effect involving inflammation markers (42, 75). Although studied to less extent, a GHSR antagonist prevents the ability of alcohol to activate the perioculomotor urocortin-containing neurons of the Edinger–Westphal nucleus (20). The notion that the activity of dopaminergic neurons of the VTA is modulated by afferents from the laterodorsal tegmental area (LDTg) or lateral hypothalamus [for review see Larsson and Engel (76)], raises the possibility that these areas could participate in the ghrelin-alcohol link. Indeed, local infusion of ghrelin into the LDTg activates the dopaminergic neurons of the VTA and enhances alcohol drinking in male mice (15, 58). Additionally, alcohol elevates ghrelin and dopamine within the lateral hypothalamus in male rats with short or long-term alcohol exposure (34). On a similar note, high alcohol consumption increases the protein levels of GHSR and immunoreactive GHSR cells in lateral hypothalamus (34). As the expression of GHSR in the brain is wide spread (55–57), additional area and circuits most likely are involved and should be defined in more detail.
2.4. Antagonism of the ghrelin pathways decreases drug-related behaviors in rodents
In addition to alcohol-mediated responses, the ghrelin pathway appears to influence behaviors induced by addictive drugs such as the psychostimulants. Indeed, the locomotor stimulatory properties (77) and CPP reward (78, 79) of cocaine are enhanced by ghrelin in male rodents. An interaction plausibly involving the ghrelin pathway in the VTA (80, 81) and NAc (82). In contrast to ghrelin, systemic administration of a GHSR antagonist attenuates the cocaine-induced hyperlocomotion, NAc dopamine release and CPP in male mice (83–86). On a similar note, GHSR inhibition reduces the reinstatement of cocaine-seeking in rats with a history of cocaine taking (81). This is further supported as GHSR inhibition reduces the ability of cocaine to cause a locomotor sensitization (84, 85). These cocaine responses appear to be mediated via central rather than peripheral ghrelin (86). Although cocaine does not alter the plasma levels of ghrelin in humans (87), enhanced circulating ghrelin is associated with cocaine seeking and cocaine taking in male rats (88, 89). Likewise, in male rodents a GHSR antagonist reduces relapse and drug-seeking for methamphetamine (90) and decreases the amphetamine-induced hyperlocomotion, NAc-dopamine and CPP (51, 68, 83). On a similar note, GHSR antagonism prevents nicotine (91–94) to activate the mesolimbic dopamine system in male rodents. Moreover, ghrelin potentiates the nicotine-induced dopamine release in striatal rat brain slices (92). These interactions between addictive drugs and ghrelin pathway in rodents are supported by few human genetic studies, where polymorphism of the GHSR genes is associated with either amphetamine or nicotine dependence (28, 95). Although the associations between ghrelin and smoking in humans are contradictory (96–102), craving for nicotine during abstinence appear positively associated with plasma ghrelin (101, 103). A finding in line with the association between ghrelin and alcohol craving (37–41).
The opioid crisis is a world-wide health problem, and novel treatment options is thus needed for opioid addictions. Intriguingly, GHSR could be a potential target as antagonists suppress the reward aspects and the drug taking of various opioid including morphine and fentanyl in male rodents (104–108). Another opioid interacting with the ghrelin pathway is oxycodone. Indeed, in the operant self-administration model systemic administration of a GHSR antagonist reduces the taking and breakpoint for oxycodone in male rats (81). The importance of ghrelin pathway for addictive drugs is also transferred to other addictive drugs, like cannabinoids. Indeed, GHSR antagonism attenuates whereas ghrelin enhances cannabinoid self-administration, seeking, and reward (109, 110). Collectively, these findings report that the ghrelin pathway modulate drug-taking and reward associated with additive drugs in male rodents.
3. Confounding factors
Although the above-mentioned articles provide support for that alcohol effects (drinking, behaviors, and neurochemistry) are enhanced by ghrelin and reduced by GHSR suppression, there are confounding factors that may influence the interpretation of these data. One such may be caloric intake and consummatory behaviors as these are well-established functions of ghrelin [for review see Espinoza Garcia et al. (111)]. This appears less likely as GHSR antagonism prevents alcohol responses like hyperlocomotion, dopamine release in NAc and CPP that are less controlled by such factors [for review see Shevchouk et al. (12)]. Yet another support for that reward, rather than consummatory behaviors and calories, are the findings that the ghrelin pathway controls drug responses which are driven by reward rather than consumption/calories. On a final note, in a choice situation GHSR antagonism decreases the most rewarding food, suggesting that reward rather than general consumption is affected. When using GHSR antagonists like [D-Lys3]-GHRP-6 receptor unselectively may be a concern. However, this appears less likely to influence the interpretation as different antagonists display similar results and opposite results to ghrelin itself. The possibility that enhanced alcohol metabolism rather than reduced alcohol reward should be taken into consideration as another confounding factor. Although not studied for the ghrelin pathway, gut-brain peptides like GLP-1 and amylin do not display such effect (112, 113). Stress is a factor that could influence the ability of the ghrelin pathway to control alcohol effects as ghrelin effects the hypothalamus-pituitary-adrenal axis (114, 115). When it comes to GLP-1, nausea/aversion is a common side effect that might influence the obtained data. This may also a possibility when it comes to ghrelin pathway as ghrelin decreases nausea (116). However, this may be less likely as GHSR antagonists do not (i) reduce water intake, (ii) alter CPP alone, or (iii) changes locomotion or NAC-dopamine per se.
4. Future directions and concluding remarks
Although these preclinical studies show that different GHSR antagonists have a similar ability to decline alcohol drinking, all but one study (25) are conducted in male animals. Besides, the neurobiological underpinnings of AUD may diverge between sexes [for review see Becker et al. (117)], and a sex-divergent difference is also when it comes to ghrelin in plasma from AUD patients (38). Upcoming studies should thus evaluate the influence of the ghrelin pathway on alcohol-mediated behaviors in female subjects. Although the above reviewed studies imply that reward-related areas are central for the alcohol-ghrelin interaction, additional studies are warranted in an attempt to define brain nodes, circuits and neurobiological substrate central for this interaction. One of the mechanisms that should be explored in detail is the ghrelin-dopamine-VTA interaction that has been implied in separate studies (7, 68, 70–72). Moreover, reward has been suggested as a central mechanism contributing to the ability of the ghrelin pathway to control alcohol drinking, but other tentative biological mechanisms should be evaluated for this interaction. Therefore future studies should explore how ghrelin affects impulsivity and risk-taking, behaviors central for AUD diagnosis. Although human and rodent studies reveal similar expression of GHSR (55–57, 64), differences are evident when it comes to effects and regions involved (17, 64). Therefore, studies should explore if similar or diverging mechanisms contribute to the ghrelin-alcohol association in humans as in rodents. Importantly, such studies should control for factors like time of alcohol exposure, withdrawal or not, gender and feeding status. Although available research indicates that GHSR are required for several steps of the AUD cycle, its role for drinking to avoid negative consequences are unknown and a subject for upcoming studies. When it comes to feeding and body weight, the combination of gut-brain peptides synergistic-like decline these parameters (118). Therefore, upcoming studies should test the possibility that the combination of a GHSR antagonist with other gut-brain peptides synergistically or additively reduces on alcohol drinking.
As reviewed above, ghrelin and its receptor appear central for alcohol responses. However, other substrates of the ghrelin pathway exist and their role in addiction processes is unknown. The ghrelin gene is translated into ghrelin-obestatin, which is converted to pro-ghrelin which after acylation by GOAT and conversion forms the active form of ghrelin; acyl-ghrelin (here denominated ghrelin) [for review see Muller et al. (119)]. Ghrelin is then formed into des-acyl-ghrelin (DAG) (120), which previously was considered inactive. However, recent advances show that DAG affects behaviors as it regulates feeding (121) and block physiological properties of ghrelin (121–123). Although, plasma levels of DAG are not associated with alcohol craving in humans (40, 41) its association with various aspects of the AUD process should be elucidated in detail. Another substrate of the ghrelin pathway is LEAP2, an endogenous inverse GHSR agonist that appears to reduce ghrelin-induced behaviors and impair dopaminergic signaling (72, 124–126). As the role of GOAT, DAG, or LEAP2 in addiction processes is unknown, future studies should explore this in detail; both in humans and animals. Besides, LEAP2 has not been explored in relation to alcohol craving or other aspects of the AUD cycle, a tentative focus on warranted plasma-association studies. Speculatively, these studies could be used to identify individuals with elevated alcohol craving that tentatively could benefit from agents targeting the ghrelin pathway. Another aspect that needs further attention is the role of central versus peripheral ghrelin signaling in addiction processes.
In conclusion, the vast number of preclinical studies reveal that the ghrelin pathway is central for reward, reinforcement and addiction processes. Besides, findings from these preclinical studies are accompanied with supporting studies in humans [for review see Morris et al. (127)]. When identifying mechanisms like the ghrelin pathway which is central for AUD, indirect insight into impulsivity and risk-taking are provided. Collectively these studies indicate that pharmacological approaches to dampen the GHSR should be evaluated in patients with AUD.
Author contributions
EJ conducted the literature search for the present review article, summarized the work conducted within the field, and wrote the review.
Funding
This work was supported by grants from the Swedish Research Council (2019–01676) and the Swedish Brain Foundation.
Conflict of interest
The author declares that the research was conducted in the absence of any commercial or financial relationships that could be construed as a potential conflict of interest.
Publisher’s note
All claims expressed in this article are solely those of the authors and do not necessarily represent those of their affiliated organizations, or those of the publisher, the editors and the reviewers. Any product that may be evaluated in this article, or claim that may be made by its manufacturer, is not guaranteed or endorsed by the publisher.
References
1. Carvalho A, Heilig M, Perez A, Probst C, Rehm J. Alcohol use disorders. Lancet. (2019) 394:781–92.
2. Rehm J, Shield K. Global burden of disease and the impact of mental and addictive disorders. Curr Psychiatry Rep. (2019) 21:10.
4. Koob G. Neurocircuitry of alcohol addiction: synthesis from animal models. Handb Clin Neurol. (2014) 125:33–54.
5. Herman A, Duka T. The role of impulsivity facets on the incidence and development of alcohol use disorders. Curr Top Behav Neurosci. (2020) 47:197–221.
6. Jerlhag E. Gut-brain axis and addictive disorders: a review with focus on alcohol and drugs of abuse. Pharmacol Ther. (2019) 196:1–14. doi: 10.1016/j.pharmthera.2018.11.005
7. Cornejo M, Mustafa E, Barrile F, Cassano D, De Francesco P, Raingo J, et al. The intriguing ligand-dependent and ligand-independent actions of the growth hormone secretagogue receptor on reward-related behaviors. Neurosci Biobehav Rev. (2021) 120:401–16. doi: 10.1016/j.neubiorev.2020.10.017
8. Kojima M, Hosoda H, Date Y, Nakazato M, Matsuo H, Kangawa K. Ghrelin is a growth-hormone-releasing acylated peptide from stomach. Nature. (1999) 402:656–60.
10. Farokhnia M, Faulkner M, Piacentino D, Lee M, Leggio L. Ghrelin: From a gut hormone to a potential therapeutic target for alcohol use disorder. Physiol Behav. (2019) 204:49–57.
11. Kharbanda K, Farokhnia M, Deschaine S, Bhargava R, Rodriguez-Flores M, Casey C, et al. Role of the ghrelin system in alcohol use disorder and alcohol-associated liver disease: a narrative review. Alcohol Clin Exp Res. (2022) 46:2149–59. doi: 10.1111/acer.14967
12. Shevchouk O, Tufvesson-Alm M, Jerlhag E. An overview of appetite-regulatory peptides in addiction processes; from bench to bed side. Front Neurosci. (2021) 15:774050. doi: 10.3389/fnins.2021.774050
13. Deschaine S, Leggio L. From “hunger hormone” to “it’s complicated”: ghrelin beyond feeding control. Physiology (Bethesda). (2022) 37:5–15. doi: 10.1152/physiol.00024.2021
14. Gupta S, Mukhopadhyay S, Mitra A. Therapeutic potential of GHSR-1A antagonism in alcohol dependence, a review. Life Sci. (2022) 291:120316. doi: 10.1016/j.lfs.2022.120316
15. Jerlhag E, Egecioglu E, Landgren S, Salome N, Heilig M, Moechars D, et al. Requirement of central ghrelin signaling for alcohol reward. Proc Natl Acad Sci USA. (2009) 106:11318–23.
16. Landgren S, Simms J, Hyytia P, Engel J, Bartlett S, Jerlhag E. Ghrelin receptor (GHS-R1A) antagonism suppresses both operant alcohol self-administration and high alcohol consumption in rats. Addict Biol. (2012) 17:86–94.
17. Suchankova P, Steensland P, Fredriksson I, Engel J, Jerlhag E. Ghrelin receptor (GHS-R1A) antagonism suppresses both alcohol consumption and the alcohol deprivation effect in rats following long-term voluntary alcohol consumption. PLoS One. (2013) 8:e71284. doi: 10.1371/journal.pone.0071284
18. Bahi A, Tolle V, Fehrentz J, Brunel L, Martinez J, Tomasetto C, et al. Ghrelin knockout mice show decreased voluntary alcohol consumption and reduced ethanol-induced conditioned place preference. Peptides. (2013) 43:48–55. doi: 10.1016/j.peptides.2013.02.008
19. Gomez J, Cunningham C, Finn D, Young E, Helpenstell L, Schuette L, et al. Differential effects of ghrelin antagonists on alcohol drinking and reinforcement in mouse and rat models of alcohol dependence. Neuropharmacology. (2015) 97:182–93.
20. Kaur S, Ryabinin A. Ghrelin receptor antagonism decreases alcohol consumption and activation of perioculomotor urocortin-containing neurons. Alcohol Clin Exp Res. (2010) 34:1525–34. doi: 10.1111/j.1530-0277.2010.01237.x
21. Gomez J, Ryabinin A. The effects of ghrelin antagonists [D-Lys(3)]-GHRP-6 or JMV2959 on ethanol, water, and food intake in C57BL/6J mice. Alcohol Clin Exp Res. (2014) 38:2436–44.
22. Stevenson J, Buirkle J, Buckley L, Young K, Albertini K, Bohidar AEGHS-. R1A antagonism reduces alcohol but not sucrose preference in prairie voles. Physiol Behav. (2015) 147:23–9.
23. Zallar L, Beurmann S, Tunstall B, Fraser C, Koob G, Vendruscolo L, et al. Ghrelin receptor deletion reduces binge-like alcohol drinking in rats. J Neuroendocrinol. (2019) 31:e12663. doi: 10.1111/jne.12663
24. Godlewski G, Cinar R, Coffey N, Liu J, Jourdan T, Mukhopadhyay B, et al. Targeting peripheral cb1 receptors reduces ethanol intake via a gut-brain axis. Cell Metab. (2019) 29:1320–33.e8. doi: 10.1016/j.cmet.2019.04.012
25. Abtahi S, Howell E, Currie P. Accumbal ghrelin and glucagon-like peptide 1 signaling in alcohol reward in female rats. Neuroreport. (2018) 29:1046–53. doi: 10.1097/WNR.0000000000001071
26. Landgren S, Jerlhag E, Zetterberg H, Gonzalez-Quintela A, Campos J, Olofsson U, et al. Association of pro-ghrelin and GHS-R1A gene polymorphisms and haplotypes with heavy alcohol use and body mass. Alcohol Clin Exp Res. (2008) 32:2054–61. doi: 10.1111/j.1530-0277.2008.00793.x
27. Suchankova P, Nilsson S, von der Pahlen B, Santtila P, Sandnabba K, Jern P, et al. Genetic variation of the growth hormone secretagogue receptor gene is associated with alcohol use disorders identification test scores and smoking. Addict Biol. (2016) 21:481–8. doi: 10.1111/adb.12277
28. Landgren S, Jerlhag E, Hallman J, Oreland L, Lissner L, Strandhagen E, et al. Genetic variation of the ghrelin signaling system in females with severe alcohol dependence. Alcohol Clin Exp Res. (2010) 34:1519–24.
29. Lee M, Tapocik J, Ghareeb M, Schwandt M, Dias A, Le A, et al. The novel ghrelin receptor inverse agonist PF-5190457 administered with alcohol: preclinical safety experiments and a phase 1b human laboratory study. Mol Psychiatry. (2020) 25:461–75. doi: 10.1038/s41380-018-0064-y
30. Farokhnia M, Lee M, Farinelli L, Ramchandani V, Akhlaghi F, Leggio L. Pharmacological manipulation of the ghrelin system and alcohol hangover symptoms in heavy drinking individuals: Is there a link? Pharmacol Biochem Behav. (2018) 172:39–49. doi: 10.1016/j.pbb.2018.07.004
31. Cepko L, Selva J, Merfeld E, Fimmel A, Goldberg S, Currie P. Ghrelin alters the stimulatory effect of cocaine on ethanol intake following mesolimbic or systemic administration. Neuropharmacology. (2014) 85:224–31.
32. Lyons A, Lowery E, Sparta D, Thiele T. Effects of food availability and administration of orexigenic and anorectic agents on elevated ethanol drinking associated with drinking in the dark procedures. Alcohol Clin Exp Res. (2008) 32:1962–8. doi: 10.1111/j.1530-0277.2008.00784.x
33. Jerlhag E, Ivanoff L, Vater A, Engel J. Peripherally circulating ghrelin does not mediate alcohol-induced reward and alcohol intake in rodents. ACER. (2013) 38:959–68.
34. Yoshimoto K, Nagao M, Watanabe Y, Yamaguchi T, Ueda S, Kitamura Y, et al. Enhanced alcohol-drinking behavior associated with active ghrelinergic and serotoninergic neurons in the lateral hypothalamus and amygdala. Pharmacol Biochem Behav. (2017) 153:1–11. doi: 10.1016/j.pbb.2016.12.001
35. Szulc M, Mikolajczak P, Geppert B, Wachowiak R, Dyr W, Bobkiewicz-Kozlowska T. Ethanol affects acylated and total ghrelin levels in peripheral blood of alcohol-dependent rats. Addict Biol. (2013) 18:689–701. doi: 10.1111/adb.12025
36. Rasineni K, Thomes P, Kubik J, Harris E, Kharbanda K, Casey C. Chronic alcohol exposure alters circulating insulin and ghrelin levels: role of ghrelin in hepatic steatosis. Am J Physiol Gastrointest Liver Physiol. (2019) 316:G453–61. doi: 10.1152/ajpgi.00334.2018
37. Addolorato G, Capristo E, Leggio L, Ferrulli A, Abenavoli L, Malandrino N, et al. Relationship between ghrelin levels, alcohol craving, and nutritional status in current alcoholic patients. Alcohol Clin Exp Res. (2006) 30:1933–7. doi: 10.1111/j.1530-0277.2006.00238.x
38. Wurst F, Graf I, Ehrenthal H, Klein S, Backhaus J, Blank S, et al. Gender differences for ghrelin levels in alcohol-dependent patients and differences between alcoholics and healthy controls. Alcohol Clin Exp Res. (2007) 31:2006–11. doi: 10.1111/j.1530-0277.2007.00527.x
39. Leggio L, Ferrulli A, Cardone S, Nesci A, Miceli A, Malandrino N, et al. Ghrelin system in alcohol-dependent subjects: role of plasma ghrelin levels in alcohol drinking and craving. Addict Biol. (2012) 17:452–64. doi: 10.1111/j.1369-1600.2010.00308.x
40. Koopmann A, von der Goltz C, Grosshans M, Dinter C, Vitale M, Wiedemann K, et al. The association of the appetitive peptide acetylated ghrelin with alcohol craving in early abstinent alcohol dependent individuals. Psychoneuroendocrinology. (2012) 37:980–6. doi: 10.1016/j.psyneuen.2011.11.005
41. Koopmann A, Bach P, Schuster R, Bumb J, Vollstadt-Klein S, Reinhard I, et al. Ghrelin modulates mesolimbic reactivity to alcohol cues in alcohol-addicted subjects: a functional imaging study. Addict Biol. (2019) 24:1066–76. doi: 10.1111/adb.12651
42. Farokhnia M, Grodin E, Lee M, Oot E, Blackburn A, Stangl B, et al. Exogenous ghrelin administration increases alcohol self-administration and modulates brain functional activity in heavy-drinking alcohol-dependent individuals. Mol Psychiatry. (2017) 23:2029–38. doi: 10.1038/mp.2017.226
43. Leggio L, Zywiak W, Fricchione S, Edwards S, de la Monte S, Swift R, et al. Intravenous ghrelin administration increases alcohol craving in alcohol-dependent heavy drinkers: a preliminary investigation. Biol Psychiatry. (2014) 76:734–41. doi: 10.1016/j.biopsych.2014.03.019
44. Suchankova P, Yan J, Schwandt M, Stangl B, Jerlhag E, Engel J, et al. The Leu72Met polymorphism of the prepro-ghrelin gene is associated with alcohol consumption and subjective responses to alcohol: preliminary findings. Alcohol Alcohol. (2017) 52:425–30. doi: 10.1093/alcalc/agx021
45. Landgren S, Berglund K, Jerlhag E, Fahlke C, Balldin J, Berggren U, et al. Reward-related genes and personality traits in alcohol-dependent individuals: a pilot case control study. Neuropsychobiology. (2011) 64:38–46. doi: 10.1159/000324992
46. Sirohi S, Schurdak J, Seeley R, Benoit S, Davis J. Central & peripheral glucagon-like peptide-1 receptor signaling differentially regulate addictive behaviors. Physiol Behav. (2016) 161:140–4.
47. King A, Vena A, Hasin D, deWit H, O’Connor S, Cao D. Subjective responses to alcohol in the development and maintenance of alcohol use disorder. Am J Psychiatry. (2021) 178:560–71.
48. Jayaram-Lindström N, Ericson M, Steensland P, Jerlhag E. Dopamine and alcohol dependence: from bench to clinic. In: Meil W editor. Recent Advances in Drug Addiction Research and Clinical Applications. London: IntechOpen (2016). p. 81–114.
49. Sanchis-Segura C, Spanagel R. Behavioural assessment of drug reinforcement and addictive features in rodents: an overview. Addict Biol. (2006) 11:2–38.
50. Urban N, Kegeles L, Slifstein M, Xu X, Martinez D, Sakr E, et al. Sex differences in striatal dopamine release in young adults after oral alcohol challenge: a positron emission tomography imaging study with [(1)(1)C]raclopride. Biol Psychiatry. (2010) 68:689–96.
51. Suchankova P, Engel J, Jerlhag E. Sub-chronic ghrelin receptor blockade attenuates alcohol- and amphetamine-induced locomotor stimulation in mice. Alcohol Alcohol. (2016) 51:121–7. doi: 10.1093/alcalc/agv100
52. Jerlhag E, Landgren S, Egecioglu E, Dickson S, Engel J. The alcohol-induced locomotor stimulation and accumbal dopamine release is suppressed in ghrelin knockout mice. Alcohol. (2011) 45:341–7.
53. Ralevski E, Horvath T, Shanabrough M, Hayden R, Newcomb J, Petrakis I. Ghrelin is supressed by intravenous alcohol and is related to stimulant and sedative effects of alcohol. Alcohol Alcohol. (2017) 52:431–8. doi: 10.1093/alcalc/agx022
54. Ralevski E, Horvath T, Shanabrough M, Newcomb J, Pisani E, Petrakis I. Ghrelin predicts stimulant and sedative effects of alcohol in heavy drinkers. Alcohol Alcohol. (2022) 58:100–6. doi: 10.1093/alcalc/agac058
55. Guan X, Yu H, Palyha O, McKee K, Feighner S, Sirinathsinghji D, et al. Distribution of mRNA encoding the growth hormone secretagogue receptor in brain and peripheral tissues. Mol Brain Res. (1997) 48:23–9.
56. Landgren S, Engel J, Hyytia P, Zetterberg H, Blennow K, Jerlhag E. Expression of the gene encoding the ghrelin receptor in rats selected for differential alcohol preference. Behav Brain Res. (2011) 221:182–8. doi: 10.1016/j.bbr.2011.03.003
57. Zigman J, Jones J, Lee C, Saper C, Elmquist J. Expression of ghrelin receptor mRNA in the rat and the mouse brain. J Comp Neurology. (2006) 494:528–48.
58. Jerlhag E, Egecioglu E, Dickson S, Douhan A, Svensson L, Engel J. Ghrelin administration into tegmental areas stimulates locomotor activity and increases extracellular concentration of dopamine in the nucleus accumbens. Addict Biol. (2007) 12:6–16. doi: 10.1111/j.1369-1600.2006.00041.x
59. Jerlhag E, Egecioglu E, Dickson S, Engel J. Glutamatergic regulation of ghrelin-induced activation of the mesolimbic dopamine system. Addict Biol. (2011) 16:82–91. doi: 10.1111/j.1369-1600.2010.00231.x
60. Jerlhag E, Egecioglu E, Dickson S, Andersson M, Svensson L, Engel J. Ghrelin stimulates locomotor activity and accumbal dopamine-overflow via central cholinergic systems in mice: implications for its involvement in brain reward. Addict Biol. (2006) 11:45–54. doi: 10.1111/j.1369-1600.2006.00002.x
61. Jensen M, Ratner C, Rudenko O, Christiansen S, Skov L, Hundahl C, et al. Anxiolytic-like effects of increased ghrelin receptor signaling in the amygdala. Int J Neuropsychopharmacol. (2016) 19:yv123. doi: 10.1093/ijnp/pyv123
62. Cornejo M, Barrile F, De Francesco P, Portiansky E, Reynaldo M, Perello M. Ghrelin recruits specific subsets of dopamine and gaba neurons of different ventral tegmental area sub-nuclei. Neuroscience. (2018) 392:107–20. doi: 10.1016/j.neuroscience.2018.09.027
63. Colvin K, Killen H, Kanter M, Halperin M, Engel L, Dickinson M, et al. Differential effects of intra-ventral tegmental area ghrelin and glucagon-like peptide-1 on the stimulatory action of d-amphetamine and cocaine-induced ethanol intake in male sprague dawley rats. Behav Brain Res. (2022) 421:113726. doi: 10.1016/j.bbr.2021.113726
64. Deschaine S, Farokhnia M, Gregory-Flores A, Zallar L, You Z, Sun H, et al. A closer look at alcohol-induced changes in the ghrelin system: novel insights from preclinical and clinical data. Addict Biol. (2021) 27:e13033. doi: 10.1111/adb.13033
65. Abizaid A, Liu Z, Andrews Z, Shanabrough M, Borok E, Elsworth J, et al. Ghrelin modulates the activity and synaptic input organization of midbrain dopamine neurons while promoting appetite. J Clin Invest. (2006) 116:3229–39. doi: 10.1172/JCI29867
66. Jerlhag E. Systemic administration of ghrelin induces conditioned place preference and stimulates accumbal dopamine. Addict Biol. (2008) 13:358–63. doi: 10.1111/j.1369-1600.2008.00125.x
67. Quarta D, Di Francesco C, Melotto S, Mangiarini L, Heidbreder C, Hedou G. Systemic administration of ghrelin increases extracellular dopamine in the shell but not the core subdivision of the nucleus accumbens. Neurochem Int. (2009) 54:89–94.
68. Edvardsson C, Vestlund J, Jerlhag E. A ghrelin receptor antagonist reduces the ability of ghrelin, alcohol or amphetamine to induce a dopamine release in the ventral tegmental area and in nucleus accumbens shell in rats. Eur J Pharmacol. (2021) 899:174039.
69. You Z, Gardner E, Galaj E, Moore A, Buck T, Jordan C, et al. Involvement of the ghrelin system in the maintenance of oxycodone self-administration: converging evidence from endocrine, pharmacologic and transgenic approaches. Mol Psychiatry. (2022) 27:2171–81. doi: 10.1038/s41380-022-01438-5
70. Kern A, Albarran-Zeckler R, Walsh H, Smith R. Apo-ghrelin receptor forms heteromers with DRD2 in hypothalamic neurons and is essential for anorexigenic effects of DRD2 agonism. Neuron. (2012) 73:317–32. doi: 10.1016/j.neuron.2011.10.038
71. Kern A, Mavrikaki M, Ullrich C, Albarran-Zeckler R, Brantley A, Smith R. Hippocampal dopamine/DRD1 signaling dependent on the ghrelin receptor. Cell. (2015) 163:1176–90. doi: 10.1016/j.cell.2015.10.062
72. Mustafa E, Cordisco Gonzalez S, Damian M, Cantel S, Denoyelle S, Wagner R, et al. LEAP2 impairs the capability of the growth hormone secretagogue receptor to regulate the dopamine 2 receptor signaling. Front Pharmacol. (2021) 12:712437. doi: 10.3389/fphar.2021.712437
73. Ryabinin A, Cocking D, Kaur S. Inhibition of VTA neurons activates the centrally projecting edinger-westphal nucleus: evidence of a stress-reward link? J Chem Neuroanat. (2013) 54:57–61. doi: 10.1016/j.jchemneu.2013.05.004
74. Cruz M, Herman M, Cote D, Ryabinin A, Roberto M. Ghrelin increases GABAergic transmission and interacts with ethanol actions in the rat central nucleus of the amygdala. Neuropsychopharmacology. (2013) 38:364–75. doi: 10.1038/npp.2012.190
75. Farokhnia M, Portelli J, Lee M, McDiarmid G, Munjal V, Abshire K, et al. Effects of exogenous ghrelin administration and ghrelin receptor blockade, in combination with alcohol, on peripheral inflammatory markers in heavy-drinking individuals: results from two human laboratory studies. Brain Res. (2020) 1740:146851. doi: 10.1016/j.brainres.2020.146851
76. Larsson A, Engel J. Neurochemical and behavioral studies on ethanol and nicotine interactions. Neurosci Biobehav Rev. (2004) 27:713–20.
77. Wellman P, Davis K, Nation J. Augmentation of cocaine hyperactivity in rats by systemic ghrelin. Regul Pept. (2005) 125:151–4. doi: 10.1016/j.regpep.2004.08.013
78. Davis K, Wellman P, Clifford P. Augmented cocaine conditioned place preference in rats pretreated with systemic ghrelin. Regul Pept. (2007) 140:148–52.
79. Dunn D, Bastacky J, Gray C, Abtahi S, Currie P. Role of mesolimbic ghrelin in the acquisition of cocaine reward. Neurosci Lett. (2019) 709:134367. doi: 10.1016/j.neulet.2019.134367
80. Schuette L, Gray C, Currie P. Microinjection of ghrelin into the ventral tegmental area potentiates cocaine-induced conditioned place preference. J Behav Brain Sci. (2013) 3:276–580. doi: 10.4236/jbbs.2013.38060
81. You Z, Galaj E, Alen F, Wang B, Bi G, Moore A, et al. Involvement of the ghrelin system in the maintenance and reinstatement of cocaine-motivated behaviors: a role of adrenergic action at peripheral beta1 receptors. Neuropsychopharmacology. (2022) 47:1449–60. doi: 10.1038/s41386-021-01249-2
82. Jang J, Kim W, Cho B, Lee J, Kim J. Microinjection of ghrelin in the nucleus accumbens core enhances locomotor activity induced by cocaine. Behav Brain Res. (2013) 248:7–11. doi: 10.1016/j.bbr.2013.03.049
83. Jerlhag E, Egecioglu E, Dickson S, Engel J. Ghrelin receptor antagonism attenuates cocaine- and amphetamine-induced locomotor stimulation, accumbal dopamine release, and conditioned place preference. Psychopharmacology (Berl). (2010) 211:415–22.
84. Clifford P, Rodriguez J, Schul D, Hughes S, Kniffin T, Hart N, et al. Attenuation of cocaine-induced locomotor sensitization in rats sustaining genetic or pharmacologic antagonism of ghrelin receptors. Addict Biol. (2012) 17:956–63.
85. Abizaid A, Mineur Y, Roth R, Elsworth J, Sleeman M, Picciotto M, et al. Reduced locomotor responses to cocaine in ghrelin-deficient mice. Neuroscience. (2011) 192:500–6. doi: 10.1016/j.neuroscience.2011.06.001
86. Wenthur C, Gautam R, Zhou B, Vendruscolo L, Leggio L, Janda K. Ghrelin receptor influence on cocaine reward is not directly dependent on peripheral acyl-ghrelin. Sci Rep. (2019) 9:1841. doi: 10.1038/s41598-019-38549-z
87. Bouhlal S, Ellefsen K, Sheskier M, Singley E, Pirard S, Gorelick D, et al. Acute effects of intravenous cocaine administration on serum concentrations of ghrelin, amylin, glucagon-like peptide-1, insulin, leptin and peptide YY and relationships with cardiorespiratory and subjective responses. Drug Alcohol Depend. (2017) 180:68–75. doi: 10.1016/j.drugalcdep.2017.07.033
88. Tessari M, Catalano A, Pellitteri M, Di Francesco C, Marini F, Gerrard P, et al. Correlation between serum ghrelin levels and cocaine-seeking behaviour triggered by cocaine-associated conditioned stimuli in rats. Addict Biol. (2007) 12:22–9. doi: 10.1111/j.1369-1600.2007.00052.x
89. You Z, Wang B, Gardner E, Wise R. Cocaine and cocaine expectancy increase growth hormone, ghrelin, GLP-1, IGF-1, adiponectin, and corticosterone while decreasing leptin, insulin, GIP, and prolactin. Pharmacol Biochem Behav. (2019) 176:53–6. doi: 10.1016/j.pbb.2018.11.001
90. Havlickova T, Charalambous C, Lapka M, Puskina N, Jerabek P, Sustkova-Fiserova M. Ghrelin receptor antagonism of methamphetamine-induced conditioned place preference and intravenous self-administration in rats. Int J Mol Sci. (2018) 19:2925. doi: 10.3390/ijms19102925
91. Wellman P, Clifford P, Rodriguez J, Hughes S, Eitan S, Brunel L, et al. Pharmacologic antagonism of ghrelin receptors attenuates development of nicotine induced locomotor sensitization in rats. Regul Pept. (2011) 172:77–80.
92. Palotai M, Bagosi Z, Jaszberenyi M, Csabafi K, Dochnal R, Manczinger M, et al. Ghrelin amplifies the nicotine-induced dopamine release in the rat striatum. Neurochem Int. (2013) 63:239–43. doi: 10.1016/j.neuint.2013.06.014
93. Jerlhag E, Engel J. Ghrelin receptor antagonism attenuates nicotine-induced locomotor stimulation, accumbal dopamine release and conditioned place preference in mice. Drug Alcohol Depend. (2011) 117:126–31. doi: 10.1016/j.drugalcdep.2011.01.010
94. Wellman P, Clifford P, Rodriguez J. Ghrelin and ghrelin receptor modulation of psychostimulant action. Front Neurosci. (2013) 7:171. doi: 10.3389/fnins.2013.00171
95. Suchankova P, Jerlhag E, Jayaram-Lindstrom N, Nilsson S, Toren K, Rosengren A, et al. Genetic variation of the ghrelin signalling system in individuals with amphetamine dependence. PLoS One. (2013) 8:e61242. doi: 10.1371/journal.pone.0061242
96. Kokkinos A, Tentolouris N, Kyriakaki E, Argyrakopoulou G, Doupis J, Psallas M, et al. Differentiation in the short- and long-term effects of smoking on plasma total ghrelin concentrations between male nonsmokers and habitual smokers. Metabolism. (2007) 56:523–7. doi: 10.1016/j.metabol.2006.11.012
97. Koopmann A, Bez J, Lemenager T, Hermann D, Dinter C, Reinhard I, et al. Effects of cigarette smoking on plasma concentration of the appetite-regulating peptide ghrelin. Ann Nutr Metab. (2015) 66:155–61. doi: 10.1159/000381834
98. Pilhatsch M, Scheuing H, Kroemer N, Kobiella A, Bidlingmaier M, Farger G, et al. Nicotine administration in healthy non-smokers reduces appetite but does not alter plasma ghrelin. Hum Psychopharmacol. (2014) 29:384–7. doi: 10.1002/hup.2405
99. Lee H, Joe K, Kim W, Park J, Lee D, Sung K, et al. Increased leptin and decreased ghrelin level after smoking cessation. Neurosci Lett. (2006) 409:47–51.
100. Lemieux A, al’Absi M. Changes in circulating peptide YY and ghrelin are associated with early smoking relapse. Biol Psychol. (2018) 131:43–8.
101. al’Absi M, Lemieux A, Nakajima M. Peptide YY and ghrelin predict craving and risk for relapse in abstinent smokers. Psychoneuroendocrinology. (2014) 49:253–9.
102. Wittekind D, Kratzsch J, Mergl R, Enzenbach C, Witte V, Villringer A, et al. Higher fasting ghrelin serum levels in active smokers than in former and never-smokers. World J Biol Psychiatry. (2020) 21:748–56. doi: 10.1080/15622975.2019.1671610
103. Potretzke S, Lemieux A, Nakajima M, al’Absi M. Circulating ghrelin changes as a biomarker of the stress response and craving in abstinent smokers. Pharmacol Biochem Behav. (2022) 218:173423. doi: 10.1016/j.pbb.2022.173423
104. Engel J, Nylander I, Jerlhag E. A ghrelin receptor (GHS-R1A) antagonist attenuates the rewarding properties of morphine and increases opioid peptide levels in reward areas in mice. Eur Neuropsychopharmacol. (2015) 25:2364–71. doi: 10.1016/j.euroneuro.2015.10.004
105. Sustkova-Fiserova M, Jerabek P, Havlickova T, Syslova K, Kacer P. Ghrelin and endocannabinoids participation in morphine-induced effects in the rat nucleus accumbens. Psychopharmacology (Berl). (2016) 233:469–84.
106. Sustkova-Fiserova M, Jerabek P, Havlickova T, Kacer P, Krsiak M. Ghrelin receptor antagonism of morphine-induced accumbens dopamine release and behavioral stimulation in rats. Psychopharmacology (Berl). (2014) 231:2899–908. doi: 10.1007/s00213-014-3466-9
107. Jerabek P, Havlickova T, Puskina N, Charalambous C, Lapka M, Kacer P, et al. Ghrelin receptor antagonism of morphine-induced conditioned place preference and behavioral and accumbens dopaminergic sensitization in rats. Neurochem Int. (2017) 110:101–13. doi: 10.1016/j.neuint.2017.09.013
108. Sustkova-Fiserova M, Puskina N, Havlickova T, Lapka M, Syslova K, Pohorala V, et al. Ghrelin receptor antagonism of fentanyl-induced conditioned place preference, intravenous self-administration, and dopamine release in the nucleus accumbens in rats. Addict Biol. (2020) 25:e12845. doi: 10.1111/adb.12845
109. Charalambous C, Havlickova T, Lapka M, Puskina N, Slamberova R, Kuchar M, et al. Cannabinoid-induced conditioned place preference, intravenous self-administration, and behavioral stimulation influenced by ghrelin receptor antagonism in rats. Int J Mol Sci. (2021) 22:2327. doi: 10.3390/ijms22052397
110. Charalambous C, Lapka M, Havlickova T, Syslova K, Sustkova-Fiserova M. Alterations in rat accumbens dopamine, endocannabinoids and GABA content during WIN55,212-2 treatment: the role of ghrelin. Int J Mol Sci. (2020) 22:210. doi: 10.3390/ijms22010210
111. Espinoza Garcia A, Martinez Moreno A, Reyes Castillo Z. The role of ghrelin and leptin in feeding behavior: genetic and molecular evidence. Endocrinol Diabetes Nutr (Engl Ed). (2021) 68:654–63.
112. Kalafateli A, Vallof D, Jerlhag E. Activation of amylin receptors attenuates alcohol-mediated behaviours in rodents. Addict Biol. (2019) 24:388–402.
113. Vallof D, Maccioni P, Colombo G, Mandrapa M, Jornulf J, Egecioglu E, et al. The glucagon-like peptide 1 receptor agonist liraglutide attenuates the reinforcing properties of alcohol in rodents. Addict Biol. (2015) 21:422–37. doi: 10.1111/adb.12295
114. Micioni Di Bonaventura E, Botticelli L, Del Bello F, Giorgioni G, Piergentili A, Quaglia W, et al. Assessing the role of ghrelin and the enzyme ghrelin O-acyltransferase (GOAT) system in food reward, food motivation, and binge eating behavior. Pharmacol Res. (2021) 172:105847. doi: 10.1016/j.phrs.2021.105847
115. Borchers S, Krieger J, Maric I, Carl J, Abraham M, Longo F, et al. From an empty stomach to anxiolysis: molecular and behavioral assessment of sex differences in the ghrelin axis of rats. Front Endocrinol (Lausanne). (2022) 13:901669. doi: 10.3389/fendo.2022.901669
116. Sanger G, Broad J, Callaghan B, Furness J. Ghrelin and motilin control systems in GI physiology and therapeutics. Handb Exp Pharmacol. (2017) 239:379–416. doi: 10.1007/164_2016_104
117. Becker J, McClellan M, Reed B. Sex differences, gender and addiction. J Neurosci Res. (2017) 95:136–47.
118. Liberini C, Koch-Laskowski K, Shaulson E, McGrath L, Lipsky R, Lhamo R, et al. Combined Amylin/GLP-1 pharmacotherapy to promote and sustain long-lasting weight loss. Sci Rep. (2019) 9:8447. doi: 10.1038/s41598-019-44591-8
119. Muller T, Nogueiras R, Andermann M, Andrews Z, Anker S, Argente J, et al. Ghrelin. Mol Metab. (2015) 4:437–60.
120. De Vriese C, Gregoire F, Lema-Kisoka R, Waelbroeck M, Robberecht P, Delporte C. Ghrelin degradation by serum and tissue homogenates: identification of the cleavage sites. Endocrinology. (2004) 145:4997–5005. doi: 10.1210/en.2004-0569
121. Asakawa A, Inui A, Fujimiya M, Sakamaki R, Shinfuku N, Ueta Y, et al. Stomach regulates energy balance via acylated ghrelin and desacyl ghrelin. Gut. (2005) 54:18–24. doi: 10.1136/gut.2004.038737
122. Heppner K, Piechowski C, Muller A, Ottaway N, Sisley S, Smiley D, et al. Both acyl and des-acyl ghrelin regulate adiposity and glucose metabolism via central nervous system ghrelin receptors. Diabetes. (2014) 63:122–31. doi: 10.2337/db13-0414
123. Fernandez G, Cabral A, Cornejo M, De Francesco P, Garcia-Romero G, Reynaldo M, et al. Des-Acyl ghrelin directly targets the arcuate nucleus in a ghrelin-receptor independent manner and impairs the orexigenic effect of ghrelin. J Neuroendocrinol. (2016) 28:12349. doi: 10.1111/jne.12349
124. Ge X, Yang H, Bednarek M, Galon-Tilleman H, Chen P, Chen M, et al. LEAP2 is an endogenous antagonist of the ghrelin receptor. Cell Metab. (2018) 27:461–9.e6.
125. M’Kadmi C, Cabral A, Barrile F, Giribaldi J, Cantel S, Damian M, et al. N-terminal liver-expressed antimicrobial peptide 2 (LEAP2) region exhibits inverse agonist activity toward the ghrelin receptor. J Med Chem. (2019) 62:965–73. doi: 10.1021/acs.jmedchem.8b01644
126. Islam M, Mita Y, Maruyama K, Tanida R, Zhang W, Sakoda H, et al. Liver-expressed antimicrobial peptide 2 antagonizes the effect of ghrelin in rodents. J Endocrinol. (2020) 244:13–23.
Keywords: appetite-regulatory peptides, gut-brain axis, alcohol, addictive drugs, dopamine, reward, addiction, dependence
Citation: Jerlhag E (2023) Animal studies reveal that the ghrelin pathway regulates alcohol-mediated responses. Front. Psychiatry 14:1050973. doi: 10.3389/fpsyt.2023.1050973
Received: 22 September 2022; Accepted: 21 February 2023;
Published: 08 March 2023.
Edited by:
Dirk Alexander Wittekind, University Hospital Leipzig, GermanyReviewed by:
Kabirullah Lutfy, Western University of Health Sciences, United StatesMorgane Thomsen, Region Hovedstad Psychiatry, Denmark
Copyright © 2023 Jerlhag. This is an open-access article distributed under the terms of the Creative Commons Attribution License (CC BY). The use, distribution or reproduction in other forums is permitted, provided the original author(s) and the copyright owner(s) are credited and that the original publication in this journal is cited, in accordance with accepted academic practice. No use, distribution or reproduction is permitted which does not comply with these terms.
*Correspondence: Elisabet Jerlhag, ZWxpc2FiZXQuamVybGhhZ0BwaGFybS5ndS5zZQ==