- 1National Clinical Research Center for Chinese Medicine Cardiology, Xiyuan Hospital of China Academy of Chinese Medical Sciences, Beijing, China
- 2Graduate School of Beijing University of Chinese Medicine, Beijing, China
- 3Wangjing Hospital, China Academy of Chinese Medical Sciences, Beijing, China
Coronary heart disease (CHD), a cardiovascular condition that poses a significant threat to human health and life, has imposed a substantial economic burden on the world. However, in contrast to conventional risk factors, depression emerges as a novel and independent risk factor for CHD. This condition impacts the onset and progression of CHD and elevates the risk of adverse cardiovascular prognostic events in those already affected by CHD. As a result, depression has garnered increasing global attention. Despite this growing awareness, the specific mechanisms through which depression contributes to the development of CHD remain unclear. Existing research suggests that depression primarily influences the inflammatory response, Hypothalamic-pituitary-adrenocortical axis (HPA) and Autonomic Nervous System (ANS) dysfunction, platelet activation, endothelial dysfunction, lipid metabolism disorders, and genetics, all of which play pivotal roles in CHD development. Furthermore, the effectiveness and safety of antidepressant treatment in CHD patients with comorbid depression and its potential impact on the prognosis of CHD patients have become subjects of controversy. Further investigation is warranted to address these unresolved questions.
1 Introduction
Coronary Heart Disease (CHD), a significant health threat, is steadily increasing yearly, often accompanied by varying mood disorders. Depression, currently the second leading cause of death worldwide after CHD itself, has emerged as an independent risk factor apart from traditional CHD risk factors such as hypertension, hyperlipidemia, diabetes, smoking, and obesity (1). The coexistence of CHD and depression significantly adds to the global healthcare burden (2).
Epidemiological surveys indicate that approximately one-fifth of Americans will experience depression at some point (3). Moreover, the prevalence of depression among CHD patients is exceptionally high, ranging from 15% to 30%, significantly surpassing the 10% prevalence in the general population (4). International studies involving 30 countries and regions reveal an overall prevalence, 1-year prevalence, and lifetime prevalence of depression to be 12.9%, 7.2%, and 10.8%, respectively (5).
Existing research on CHD comorbid with depression primarily relies on depressive symptom scales and often exhibits a dose-response pattern (6, 7). This means that higher levels of depressive symptoms are associated with a greater risk of developing CHD. Nevertheless, causal associations between depression and CHD remain controversial. The physiological pathways connecting depression to CHD are notably intricate, making an in-depth understanding of this relationship crucial for developing rational and effective interventions in clinical treatment. Such efforts are essential to enhance patients’ quality of life and improve cardiovascular outcomes.
This article aims to summarize the effects of depression on CHD and evaluate the efficacy of antidepressant therapy, in order to provide valuable insights and a foundation for clinical treatment strategies and the development of new drugs.
2 Depression as a risk factor for CHD
Depression exhibits a high comorbidity with CHD, and its association with CHD is even stronger when compared to traditional risk factors for cardiovascular disease (8). This mood disorder increases the risk of developing CHD (9) and significantly impacts prognosis (10). A 1-year follow-up study involving 1024 CHD patients revealed that depression constituted a significant risk factor for mortality in this group (HR = 3.19, 95% CI 1.32-7.69). Moreover, the severity of the depressive state correlated with a worse prognosis and a higher incidence of adverse cardiovascular outcomes (11).
At the outset of the 21st century, a comprehensive international study by 30 health organizations examined the interrelationship between psychosomatic factors and CHD. It revealed that, even after accounting for traditional risk factors such as hypertension and obesity, the incidence of CHD was three times higher in individuals with depression (12). In a substantial cohort study involving 1.9 million participants, depression significantly elevated the occurrence of stable/unstable angina and myocardial infarction in CHD patients (13). However, conflicting perspectives exist regarding whether depression increases morbidity and mortality in CHD patients. For instance, the ENRICHD study, a well-known clinical trial in the CHD field, reported that depression did not increase morbidity or mortality, precisely due to CHD. Still, it did lead to an overall increase in mortality (14). Moreover, the impact of depression on CHD appears to be influenced by factors such as gender, age, and the duration of the illness. A Meta-analysis indicated that depression did not raise the risk of CHD in patients with more than 15 years of follow-up. However, it did correlate with an increased incidence of CHD in patients with less than 15 years of follow-up (15). Further reinforcing the association, a 3-year follow-up study confirmed that CHD patients with comorbid depression had a significantly higher morbidity and mortality rate than those with CHD alone (16).
Furthermore, depression can undermine treatment adherence in CHD patients, posing an additional challenge to their healthcare. A study utilizing a medication monitor to gather objective data on patient medication adherence discovered that CHD patients with comorbid depression displayed lower adherence to low-dose aspirin, evident from reduced drug concentrations. Gehi et al. (17) assessed medication adherence through self-reporting in a cohort of 940 patients discharged from the hospital with stable CHD. The findings revealed that the nonadherence rate was 5% among CHD patients without depression, 7% in those with moderate depression, and 14% in those with major depression. In summary, depression impacts the morbidity of CHD and exerts an exceptionally negative influence on its treatment and prognosis. Even with the most well-designed secondary prevention program for CHD, depression can still precipitate adverse cardiovascular events in patients with CHD. As a result, clinicians should be vigilant in screening for depression among CHD patients, and timely provision of antidepressant treatment becomes paramount to improving patient outcomes and reducing morbidity and mortality rates.
3 Mechanisms
There are many hypotheses about the possible mechanisms by which depression contributes to the development of CHD, but they are still not fully elucidated. The currently proposed mechanisms of depression causing CHD include socio-behavioral and physiological mechanisms such as over-activation of the inflammatory response, autonomic dysfunction, endothelial dysfunction, abnormal HPA axis function, platelet over-activation, and genetics. The mechanism is shown schematically in Figure 1.
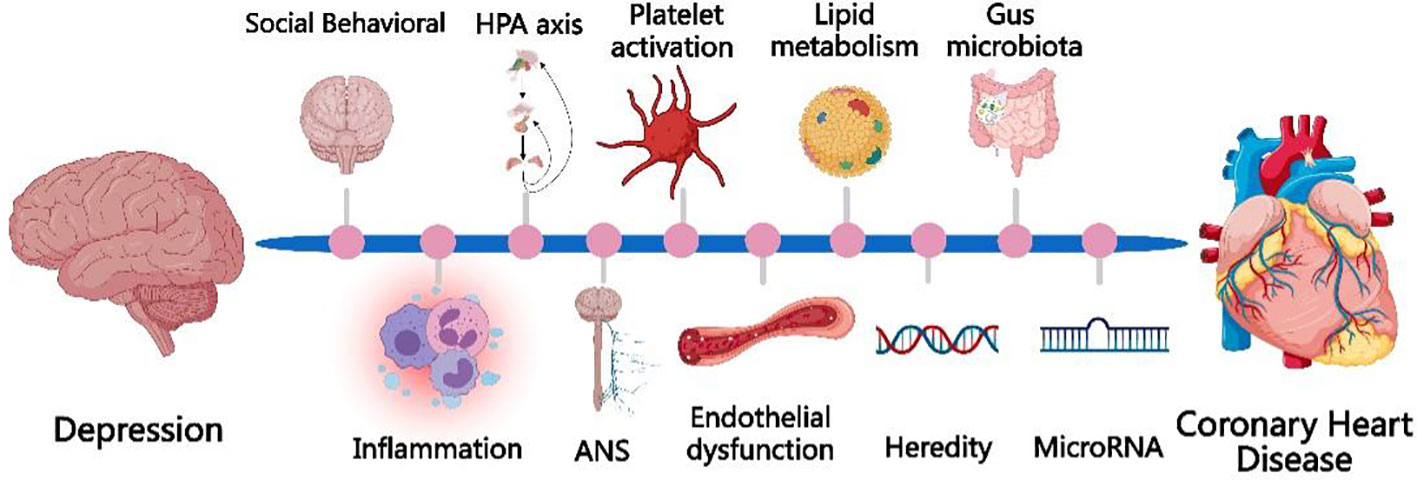
Figure 1 The currently proposed mechanisms of depression causing CHD include socio-behavioral mechanisms and physiological mechanisms, such as over-activation of the inflammatory response, autonomic dysfunction, endothelial dysfunction, abnormal HPA axis function, platelet over-activation, and genetics, microRNA, gut microbiota.
3.1 Social behavioral and type D personality
Behavioral abnormalities in depressed patients may indeed contribute to the development of CHD. In today’s fast-paced lifestyle, characterized by tremendous work, economic, and social stress, the silent toll it takes on human physical and mental health is alarming. Depressed individuals often experience low mood, loss of interest in their surroundings, a significant reduction in physical activity compared to healthier individuals, and may even have suicidal tendencies. A study conducted in 2011 that assessed the depressive status and long-term follow-up of 5,888 older adults discovered that behavioral activities, such as physical activity and depression, were independent predictors of cardiovascular disease mortality. Importantly, the association between depression and cardiovascular disease was strong (18). Chronic stress emerges as a significant risk factor for CHD, leading to an increased susceptibility to cardiovascular events. Chronic stress contributes to this heightened risk by activating the Hypothalamic-Pituitary-Adrenal (HPA) axis, causing autonomic dysfunction and promoting inflammatory responses (19). Studies have consistently demonstrated the link between chronic stress and an increased incidence of depression. Prolonged stress triggers changes in neurotransmitters and neuroendocrine molecules, affecting neuronal activity and potentially contributing to the development of depression through activating the HPA axis and the elevation of pro-inflammatory factors (20).
Type D personality, also known as “sad personality,” refers to an individual’s chronic experience of negative emotions, such as sadness, anxiety, irritability, and anger, as well as a tendency to express negative emotions in social situations. The main personality traits of Type D individuals are negative emotions and social inhibition. CHD patients with a Type D personality are more prone to experiencing depression, and the severity of their Type D personality traits is positively correlated with depression (21). Furthermore, Lin et al. (21) demonstrated that Type D personality is associated with an increased risk of adverse cardiovascular events and death in patients with CHD. The pathophysiological mechanisms underlying this association may involve dysfunction of the cardiac Autonomic Nervous System (ANS) and dysregulation of lipid profiles.
Furthermore, CHD patients who suffer from depression may exhibit decreased treatment adherence, failure to take medications as prescribed, delayed participation in cardiac rehabilitation programs, and some may even become overweight or obese due to side effects of tricyclic antidepressants. Depressed patients are more prone to engaging in adverse lifestyle behaviors such as smoking and alcohol abuse (22). These behavioral abnormalities contribute to an increased incidence of CHD and result in a significant rise in adverse cardiovascular events. Therefore, these behavioral abnormalities play a role in the likelihood of developing CHD and contribute to a significant increase in adverse cardiovascular events.
3.2 Inflammatory response
In the late 1990s, CHD was identified as an inflammatory disorder, and subsequent studies have demonstrated the crucial role of the inflammatory response in the atherosclerotic process (23). Moreover, depression has been linked to a persistent inflammatory state closely associated with the inflammatory response. The inflammatory response is a significant mechanism in the pathogenesis of depression and CHD, and it may be a key factor in how depression contributes to the development of CHD (22). In individuals with depression, the risk of the body being stimulated by bacterial lipopolysaccharide increases, leading to heightened secretion of cytokines by monocytes. This results in the overexpression of inflammatory factors and cytokines like C-reactive protein, tumor necrosis factor, and interleukins. The degree of elevation of these cytokines is positively correlated with the severity of depression (24). These cytokines, individually or in synergy, prompt the conversion of monocytes into pro-inflammatory cells, mediating non-specific inflammatory response and altering immunoreactivity. Additionally, these cytokines can impact the cardiovascular system by influencing the release of neurotransmitters from the central nervous system, thereby mediating sympathetic effects (25). Depression and inflammation exhibit a bidirectional relationship: depression can promote an inflammatory response, and conversely, blocking pro-inflammatory factors (e.g., TNF-α) can partially alleviate depressive symptoms (26). Apart from increased pro-inflammatory factors, a decrease in anti-inflammatory factors has also been associated with depression. For instance, Li et al. (27), in their study on the relationship between depression and inflammatory factors in cancer patients, found a negative correlation between depression severity and plasma levels of the anti-inflammatory factor IL-4. These findings suggest that depression may involve up regulation of pro-inflammatory factors and down regulation of anti-inflammatory factors.
The inflammatory response induced by depression is closely associated with the development of CHD and adverse cardiovascular events. Specifically, CRP, IL-6, and TNF-α can destabilize atherosclerotic plaques, leading to plaque rupture and thrombosis at a later stage. Additionally, they directly affect myocardial contractility, inducing cell apoptosis (28). Experimental studies have shown that chronic stress increases macrophage infiltration and atherosclerotic plaque growth and vulnerability in a mouse model, which is associated with the development of myocardial infarction and injury (29). Clinical studies have found a positive correlation between high levels of hs-CRP and the severity of depression at baseline in patients with myocardial infarction (30). Empana et al. (31), in a prospective epidemiologic study of European patients with myocardial infarction, observed that Welsh Depression Scale scores were associated with elevated levels of IL-6 (OR 1.39), CRP (OR 1.30), intercellular adhesion molecule 1 (OR 1.22), and plasma fibrinogen (OR 1.36) after correcting for other risk factors compared to age-matched controls with angina pectoris or nonfatal myocardial infarction. Chronic inflammation can cause damage to arterial walls and increased activity of T and B lymphocytes, suggesting that depression may contribute to or worsen CHD through the inflammatory response process (32). In conclusion, inflammation and immunity represent significant and promising research areas in studying the pathogenesis of CHD related to depression. In depressed patients, increased pro-inflammatory and decreased anti-inflammatory factors may be associated with CHD’s onset, progression, and prognosis. As more inflammatory markers are used to study CHD and depression, the mechanism of the inflammatory response’s involvement in depression leading to CHD will be further elucidated in depth.
3.3 Dysfunction of the HPA axis
HPA axis dysfunction has been extensively studied in the context of depression. Elevated circulatory cortisol levels, resulting from enhanced HPA axis activity due to depression, may represent another possible biological mechanism that triggers CHD (33). In patients with depression, the HPA axis is activated, and the paraventricular nucleus of the hypothalamus synthesizes and releases corticotropin-releasing hormone (CRH) and arginine vasopressin, which are then transported to the anterior pituitary gland and stimulate the secretion of adrenocorticotropic hormone (ACTH) into the systemic circulation. Subsequently, ACTH acts on the zona fasciculata of the adrenal cortex, promoting cortisol synthesis and release. CRH, in turn, increases heart rate, cardiac output, and mean arterial pressure by stimulating the secretion of norepinephrine and epinephrine. Cortisol influences the cardiovascular system, increasing myocardial contractility, cardiac output, and blood pressure. It also contributes to the redistribution of visceral fat, triggers inflammatory responses, leads to insulin resistance, and results in hypercholesterolemia – all risk factors for CHD. Moreover, cortisol plays a role in inhibiting growth hormone secretion and activity of the gonadal axis (34). Deficiency in growth hormone increases the risk of adverse cardiovascular events, while testosterone deficiency is associated with metabolic abnormalities and poor prognosis in patients with cardiovascular disease (35). On the other hand, estrogen’s protective effects on the cardiovascular system have long been recognized. In summary, HPA axis dysfunction and the subsequent elevation of cortisol levels can contribute to the development of CHD, potentially through various cardiovascular and metabolic effects. Additionally, the interplay of growth, testosterone, and estrogen hormones further influences cardiovascular health and outcomes in patients with CHD and depression.
Indeed, depression can lead to changes in neuroendocrine function, activating both the HPA axis and the sympathetic nervous system. This activation, in turn, promotes the release of angiotensin-II and glucocorticoids, subsequently activating the glucocorticoid receptor, thereby increasing the risk of CHD (36). Studies on rat models of depression have shown significantly elevated levels of glucocorticoids, which were found to reduce the viability of cardiomyocytes cultured in vitro (37). Glucocorticoids can directly impact the pathophysiological mechanisms of the cardiovascular system. They can lead to the over-activation of sympathetic nerves, elevate blood pressure, increase myocardial oxygen consumption, and enhance the sensitivity of the cardiovascular system to catecholamines. These effects can contribute to an increased risk of CHD (37). In summary, depression-induced changes in neuroendocrine function, particularly the activation of the HPA axis and sympathetic nervous system, can elevate glucocorticoid levels, affecting the cardiovascular system and increasing susceptibility to CHD. The intricate interplay of these mechanisms highlights the complex relationship between depression and cardiovascular health.
3.4 Autonomic nervous system dysfunction
The relationship between ANS (Autonomic Nervous System) dysfunction, depression, and CHD has been extensively studied, and impaired ANS function is considered an intermediate risk factor that links depression to an increased risk of CHD. As mentioned earlier, depression can lead to the hyperfunction of the HPA axis, which, in turn, can alter cardiac autonomic function through central nervous system regulation. This alteration results in sympathetic overactivation and parasympathetic hypofunction. Sympathetic overactivation can have various detrimental effects on the cardiovascular system. On one hand, it can lead to the excitation of α-receptors, causing coronary artery spasm and vasoconstriction, resulting in myocardial ischemia and increased blood pressure. On the other hand, sympathetic overactivation can stimulate β-receptors, increasing heart rate, myocardial contractility, and myocardial oxygen consumption. This effect lowers the threshold for ventricular fibrillation, making the heart more susceptible to ventricular tachycardia, ventricular fibrillation, and other malignant cardiac arrhythmias. Additionally, it increases the risk of sudden cardiac death (38).
Heart rate variability (HRV) is an important repeatable quantitative and noninvasive indicator of cardiac autonomic nervous system (ANS) function (39). It refers to the variation in sinus rhythm (the standard deviation of the intervals between two consecutive R-waves in sinus rhythm on the electrocardiogram) over a certain period, typically 24 hours. HRV reflects the ability of the ANS to change heart rate in response to changes in hemodynamics or other physiological disturbances, indicating the interaction and balance between sympathetic and parasympathetic nerves in regulating heart rate. A decrease in HRV suggests dysfunction of the cardiac ANS, which is considered a strong and independent predictor of poor prognosis in CHD. Notably, HRV has also been a focus of biomarker studies in depression. Research has found that HRV is significantly lower in patients with major depressive disorder than in healthy controls and tends to normalize as depressive symptoms improve. Various parameters of HRV, such as the standard deviation of all sinus beat R-R intervals (SDNN), root mean square of the difference between neighboring R-R intervals (rMSSD), and the percentage of R-R intervals with a difference of more than 50 ms to the total number of R-R intervals (PNN50), are reduced in depression (40, 41). Depression can lead to sympathetic excitation, resulting in an increased heart rate in patients, and a higher incidence of various tachyarrhythmias compared to CHD patients without comorbid depression (42). Individuals with CHD and depression exhibit lower HRV, reduced pressure receptor sensitivity, increased QT interval variability, and heart rate oscillations compared to those without depression (43). Animal experiments have also confirmed that depression significantly increases the incidence of ventricular arrhythmias, prolongs the QT interval, increases sympathetic excitability, and enhances post-infarction myocardial remodeling in rats (44). These findings collectively suggest that depression can adversely affect CHD by influencing ANS function and, consequently, impacting HRV and CHD outcomes. However, whether depression affects HRV in all types of patients with CHD remains controversial. Some studies have shown that depression causes a significant decrease in HRV and a significant increase in heart rate in patients with stable angina (45). In contrast, other studies have found that depression has no significant effect on HRV in patients with unstable angina (46). Furthermore, it has been suggested that HRV reduction may be associated only with specific depressive symptoms (47). Nonetheless, some evidence indicates that antidepressants can significantly affect HRV (48). These discrepancies may stem from the complexity of depression and its various interactions with ANS function in different populations of CHD patients.
After excessive activation of sympathetic nerves due to depression, catecholamine levels are elevated, and adrenergic receptor action is enhanced, significantly increasing the risk of adverse outcomes such as the development of CHD and malignant arrhythmias, and even sudden cardiac death. Experimental studies in rats with depression combined with Myocardial Infarction (MI) have shown elevated plasma levels of epinephrine and norepinephrine, along with higher levels of myocardial tyrosine hydroxylase expression compared to the MI-only group. Additionally, these rats exhibited increased left ventricular volume and thinned myocardial walls, suggesting that depression may contribute to the unfavorable prognosis of MI by enhancing sympathetic nerve activity and promoting ventricular remodeling (49). Hence, it is evident that the hyperfunction of the HPA axis induced by depression, coupled with the overactivation of sympathetic nerves, collaborates to reduce myocardial stress tolerance, significantly influencing the pathogenesis and prognosis of CHD.
3.5 Platelet activation and 5-hydroxytryptamine
The primary pathological change in CHD is atherosclerosis, characterized by an inflammatory process that involves impaired endothelial function mediated by platelets and leukocytes. Depression plays a role in promoting CHD by activating platelets through sympathetic overactivation and influencing the 5-HT (serotonin) system, which can lead to the rupture of atherosclerotic plaques and thrombosis.
Ormonde do Carmo et al. (50) conducted a study comparing blood samples from 22 depressed patients without antidepressant treatment to 27 healthy controls. The findings revealed that depressed patients exhibited increased protein carbonylation in platelets, along with an overexpression of phosphodiesterase-5 and significantly heightened platelet aggregation. In another study by Williams et al. (51) involving 300 patients with cardiovascular disease comorbid with depression, it was observed that compared to patients with uncomplicated depression, those with comorbid depression had more serotonin receptors in their blood, and their platelets were more prone to aggregation. Similarly, a Chinese study also confirmed that patients with CHD combined with depression had elevated platelet specific volume and increased platelet activity (52). Therefore, based on these findings, it is plausible to hypothesize that platelet activation and aggregation may represent another essential mechanism through which depression contributes to the development of CHD.
Furthermore, 5-HT (serotonin) acts as a weak platelet activator, stored within platelets, and induces both platelet activation and coronary vasoconstriction. It also triggers other platelet activators, such as adenosine diphosphate, thromboxane A2, thrombin, and catecholamines, leading to increased platelet activity (53). In cases of depression, there is a reduction or abnormality in 5-HT levels, resulting in an up-regulation of 5-HT receptors on the platelet surface (53). This increased receptor responsiveness to 5-HT renders platelets more susceptible to activation. Moreover, depression can elevate the expression of integrin αIIb-β3 and increase plasma levels of platelet activation markers, such as platelet factor-4 (PF-4), β-thromboglobulin (β-TG), and P-selectin, ultimately enhancing platelet activity. A study demonstrated significantly higher levels of platelet activation markers, including PF-4 and β-TG, in patients with both CHD and depression compared to patients with CHD alone and healthy controls (54). Platelet activation not only fosters endothelial damage by interacting with leukocytes but also stimulates the release of related factors, further promoting the development of atherosclerosis. This has adverse implications for the prevention and treatment of CHD and heightens the risk of adverse cardiovascular events. Additionally, it has been suggested that depression-induced platelet aggregation may be related to the elevated levels of inflammatory factors induced by depression. These factors can promote the release of adhesion molecules from coronary endothelial cells (42), thereby exacerbating the progression of atherosclerosis and its detrimental impact on CHD management and cardiovascular outcomes.
3.6 Endothelial dysfunction
Depression’s impact on CHD is likely mediated through endothelial dysfunction. Endothelial dysfunction involves several key characteristics, such as reduced endothelium-dependent vasodilation and barrier function, hemodynamic abnormalities, impaired fibrinolytic capacity, increased expression of growth factors and adhesion molecules, and heightened oxidative stress, among others (55). Vascular endothelial dysfunction is the initiator of atherosclerosis, being a primary cause of diminished vascular reactivity and a significant pathomechanism in CHD. Depression can contribute to the progression of endothelial dysfunction, which, in turn, leads to the development and exacerbation of atherosclerosis. These harmful effects on the vascular endothelium can further compromise vascular health and increase the risk of CHD-related complications. Understanding the relationship between depression and endothelial dysfunction is crucial in comprehending the comprehensive mechanisms underlying the association between depression and CHD.
Flow-mediated dilatation (FMD) is a commonly used to assess vascular endothelial function. Patients with CHD combined with depression were found to have significantly lower absolute and percentage FMD compared to those with CHD alone, providing support for the notion that depression contributes to endothelial dysfunction (56). Furthermore, the correlation between endothelial dysfunction and depression persists even after adjusting for various influences, such as lifestyle factors (57). Nitric oxide (NO) is a critical mediator of vascular endothelial function and is a common vasodilator, which plays a crucial role in maintaining a healthy vascular system. NO promotes vasodilation, inhibits smooth muscle cell contraction, and prevents platelet aggregation and leukocyte adhesion. Reduced levels of endogenous NO can lead to endothelial dysfunction (58). Studies conducted by Greaney et al. (59) revealed that depressed patients experience increased oxidative stress in the vascular endothelium, along with decreased NO synthesis. Similarly, experimental studies on depressed rats have yielded similar findings. These rats exhibited significantly reduced endothelium-dependent vasodilatory responses, and direct administration of the NO donor sodium nitroprusside or the non-NO-dependent opioid agonist failed to induce vasodilation in the depressed rats (60). Severe depression has been associated with increased lipid peroxidation, elevated plasma levels of lipid peroxidation metabolites such as 4methyl-2-NONERAL and asymmetric dimethylarginine, decreased endothelial nitric oxide synthase (NOS) activity, and reduced plasma NO levels. These alterations impact vasoconstriction, neurotransmitter release, and perfusion, and contribute to endothelial damage. This cascade of events ultimately leads to platelet aggregation, an upsurge in inflammatory response, coronary artery constriction, and enhanced intercellular adhesion, significantly increasing CHD and cardiovascular events (25).
3.7 Disorders of lipid metabolism
Depression can lead to disorders of lipid metabolism, contributing to the development of CHD by promoting an increase in abdominal fat and epicardial fat surrounding the coronary arteries. Elevated lipids are well-known risk factors for CHD, particularly serum total cholesterol (TC) and low-density lipoprotein cholesterol (LDL-C). Clinical studies have shown that depression is associated with elevated TC and LDL-C levels, while triglyceride levels tend to be lower. Notably, the correlation between depression and LDL-C is more pronounced (61, 62). Animal studies have provided similar insights, demonstrating that simvastatin, a lipid-lowering drug commonly used in CHD, significantly improved depressive symptoms in mice with depression-like models (63). In addition to the overall lipid levels, oxidized LDL (ox-LDL) plays a crucial role in the pathogenesis of CHD. Maes et al. observed that depressed patients had higher levels of ox-LDL and lower levels of vitamin E compared to controls. These findings indicate that depressed patients have relatively enhanced lipid oxidation and weakened antioxidant protection mechanisms in their bodies, further predisposing them to CHD.
Abnormal metabolism of omega-3 polyunsaturated fatty acids is also a significant intermediate factor that affects the development of both depression and CHD. Omega-3 polyunsaturated fatty acids are important neurotransmitters, influencing depression by regulating 5-HT, dopamine, and brain-derived neurotrophic factor (64, 65). Evidence suggests that reduced levels of omega-3 polyunsaturated fatty acids are associated with the development of depression and other affective disorders in a bidirectional manner. Moreover, decreased levels of omega-3 polyunsaturated fatty acids are closely linked to the development of CHD. Under normal circumstances, these fatty acids exert beneficial effects by acting as anti-inflammatory agents, antioxidants, and regulators of lipid metabolism, thereby reducing the progression of atherosclerosis and stabilizing plaques. Studies have revealed that plasma levels of omega-3 polyunsaturated fatty acids in patients with CHD combined with depression are lower compared to those without comorbid depression. Notably, supplementation with omega-3 polyunsaturated fatty acids has been shown to slow the progression of CHD and decrease the risk of cardiovascular events (66). Hence, it is plausible that depression contributes to the development of CHD by affecting the metabolism of omega-3 polyunsaturated fatty acids. The interplay of these fatty acids in both depression and CHD underscores their significance in the pathogenesis and management of these conditions, emphasizing the importance of exploring their potential therapeutic implications in preventing and treating both depression and CHD.
3.8 Heredity
Depression, like traditional risk factors for CHD, has a hereditary component. However, direct evidence regarding the genetic mechanisms linking depression and CHD remains limited. In the early 21st century, a study involving 2,731 same-sex twins (mean age 41.9 years, SD 2.7) found a significant genetic correlation between depression, hypertension (r = 0.19), and CHD (r = 0.42). These findings suggest that common genetic risk factors may be involved in the association between depression and CHD (67). Subsequently, Lu et al. (68) conducted a Mendelian randomization and mediation analysis to evaluate the associations of depression-related genetic variants with CHD. They concluded that Genetic liability to depression is associated with higher CHD risks. These discoveries have led to the possibility that depression and CHD may represent different phenotypes of the same genes. However, some researchers, such as de Geus (69), argue that genetic pleiotropy does not rule out other possibilities. For instance, a particular gene may cause depression, and subsequently, depression may lead to or worsen CHD. This alternative explanation highlights the complex interplay of genetic and environmental factors in the relationship between depression and CHD. While the genetic basis of the association between depression and CHD warrants further investigation, it is evident that both conditions share common risk factors and biological pathways. The convergence of genetic, environmental, and behavioral factors likely contributes to the intricate relationship between depression and CHD. More comprehensive research is needed to unravel the underlying mechanisms and potential therapeutic implications for managing both depression and CHD effectively.
Nakatani et al. (67) examined the S allele of the 5-hydroxytryptamine transporter gene in 2,509 post-myocardial infarction patients. They found that individuals with the S allele had a higher prevalence of depression (48%) compared to those without it (35%). Furthermore, the presence of the S allele was associated with an increased risk of cardiovascular events (HR 1.69), but this effect became non-significant after adjusting for depressive symptoms. This suggests that depression has the potential to influence the prognosis of CHD. Subsequently, mounting evidence supports the notion that the 5-HT transporter (5-HTT) gene and the 5-HT receptor (5-HTR) gene may play a role in the process of depression contributing to CHD. Specifically, gene polymorphisms in the promoter sequence of the 5-HT transporter gene (5-HTTLPR) are closely associated with depression. The SS genotype of 5-HTTLPR restricts the transcriptional activity of the promoter, leading to a decrease in the level of transcription of the 5-HT transporter protein gene (SLC6A4) and a reduction in 5-HT reuptake, which can contribute to the development of depression (70). Furthermore, the SS genotype is also independently associated with comorbid depression in patients with CHD, making it a susceptibility gene for depression in individuals with CHD. Additionally, the haplotype angiotensin I-converting enzyme (ACE) gene D/D and 5-HTTLPR I/I may be a vulnerability factor for comorbid depressive symptoms in CHD patients (71). Therefore, it is hypothesized that the 5-HTTLPR gene polymorphism may represent one of the genetic mechanisms by which depression contributes to CHD. However, further in-depth research must validate and explore this hypothesis thoroughly.
3.9 Other emerging potential mechanisms
The study of microRNA (miRNA) alterations in CHD and depression is an evolving field, revealing the intricate role of miRNA in both systemic and mental health disorders. Numerous miRNAs exhibit altered expression levels during cardiovascular diseases such as atherosclerosis, myocardial infarction, and heart failure. Furthermore, miRNAs associated with various risk factors have contributed to the development of CHD (72, 73). MicroRNA-34a (miR-34a) is essential in regulating blood lipid, inflammation, cell adhesion molecules, and atherosclerosis; the latter factors are closely involved in coronary heart disease (CHD) etiology. MiR-34a was elevated in CHD patients compared to controls (p < 0.001), and it disclosed an excellent diagnostic value of CHD (74). Approximately 100 miRNAs involved in lipid metabolism have been identified, with miRNA-122 highlighted by Tsai et al. as capable of reducing the expression of genes involved in triglyceride metabolism through the adenosine monophosphate-activated protein kinase pathway (75).
Functional dysregulation of miRNA regulation has also been observed in depression (76, 77). MicroRNA-16, for instance, exerts antidepressant activity by inhibiting the expression of the serotonin transporter, reducing the reuptake of synaptic serotonin, and promoting serotonin signal transduction (78–80). Brain-derived neurotrophic factor (BDNF) plays a crucial role in modulating miRNA expression. BDNF, through the activation of the mitogen-activated protein kinase/extracellular signal-regulated kinase pathway, selectively upregulates the expression miRNA-132 in cultured rat cortical neurons. This upregulation correlates with increased axonal growth and dendritic spine numbers, suggesting that miR-132 may regulate BDNF-mediated neural plasticity (81–83). Considering the significant role of BDNF in the pathogenesis of depression, dysregulation of BDNF-associated miRNAs may also be closely linked to depression.
Research into miRNA changes in CHD and depression is rapidly advancing, underscoring the functional roles and dysfunctions of miRNA in both systemic and mental disorders. CHD and depression appear to share some common pathological mechanisms that depend on miRNA regulation. Although there is currently no evidence supporting the use of miRNA gene chip detection and related methods in patients with comorbid CHD and depression, these studies are in their early stages. Therefore, it is believed that miRNA will directly correlate with the comorbidity of CHD and depression shortly, becoming a valuable research tool and possibly a valuable therapeutic tool.
The gut microbiota is key in regulating physiological processes such as intestinal environmental stability, metabolic regulation, and immune responses. Recent studies have shown that the gut microbiota is closely related to the pathogenesis of coronary heart disease and depression, mainly through the multilevel regulation of the microbiota-gut-brain axis, encompassing inflammatory responses, chronic inflammation, and metabolites (84). Changes in the composition and diversity of the gut microbiota have been associated with the development of chronic inflammatory diseases, including cardiovascular disease. It has been noted that gut microbial disorders can trigger various pathophysiological events, including weight gain, adipose deposition, low-grade inflammatory response, and the association of reduced gut microbial diversity with the prevalence of diabetes mellitus, obesity, and metabolic syndrome (85). In addition, the gut microbiota is directly involved in the development of coronary atherosclerosis and may play a key role in cardiac metabolic homeostasis and cardiovascular disease through multiple pathways (86).
On the other hand, the gut microbiota composition may show differences in patients with CVD. Significant differences in the diversity and composition of the gut microbiota were found between patients with coronary artery disease and healthy controls, including a decrease in the phylum Bacteroidetes and Aspergillus and an increase in the phylum Fusobacterium and Fusobacterium (87, 88). The presence of Aspergillus bacteria in the bloodstream has been strongly correlated with cardiovascular complications and may serve as an independent risk marker for cardiovascular disease (89, 90).
Clinical studies have confirmed that changes in the microbiota characterization are strongly associated with an increased risk of cardiovascular disease. Different abundances of the microbiota may be more important in determining the risk of cardiovascular disease than their relative frequencies. In addition, the composition and relative abundance of the gut microbiota impact neurologic function, suggesting a strong link between cardiovascular and neurologic diseases (91). The gut microbiota has been shown to impact neurologic function significantly.
The gut microbiota influences brain development and function by releasing metabolites or stimulating the production of neuroactive substances by endocrine cells in the gastrointestinal tract (92–94). The brain monitors and regulates the composition of the gut microbiota through neural, immune, and endocrine pathways to maintain a normal species profile or adjust the microbial profile in response to environmental changes (95–97). The beneficial role of probiotics in depressed patients may be related to vagal regulatory pathways (98). However, the “microbiota-gut-brain axis” interactions may also lead to alterations in the microbial environment, which may affect neural activity and function and trigger pathological outcomes such as depression (87). Clinical studies have demonstrated that patients with depression have changes in the composition and relative abundance of gut flora, which are mediated through inflammatory responses, HPA, and other factors that may affect the gut flora’s ability to respond to the environment. Changes induce depression by altering CNS function through inflammatory responses, HPA axis activity, and neurotransmitter signaling (99, 100). Therefore, restoration of physiological homeostasis of the CNS and modulation of inflammatory factors and neurotransmitters by adjusting the composition and function of gut microflora may improve depressive behavior.
4 Interventions
The United States Preventive Services Task Force (USPSTF) once emphasized the increased risk of depression in patients with cardiovascular diseases (101). However, despite the gradual increase in depression screening since 2009, only 3% of outpatients still received depression screening by 2015 (102). Although providing reasonable intervention methods for CHD patients with depression is particularly important, accurate screening and diagnosis are prerequisites. Therefore, developing standardized screening methods for depression in CHD patients provides potential for early recognition and prevention of CHD and depression (103).
Current clinical treatment options for CHD co-morbid with depression encompass medication, psychological intervention therapy, exercise therapy, and acupuncture. Antidepressant treatment, combined with conventional CHD therapy, has improved psychological status and symptoms (8). Selective 5-hydroxytryptamine reuptake inhibitors (SSRIs) are the preferred antidepressants due to their anti-inflammatory, antiplatelet aggregation, and HPA axis activity reduction effects (104). These SSRIs increase the concentration of synaptic 5-HT, which helps inhibit depression-induced CHD with minimal side effects on the cardiovascular system. Sertraline hydrochloride, paroxetine, and citalopram are among the commonly used SSRIs. Notably, sertraline has significantly reduced the recurrence of myocardial infarction (MI) and mortality, making it the first-line drug for treating depression combined with CHD (105). Studies have also suggested that sertraline treatment may improve vascular endothelial function and reduce adverse cardiac events by decreasing platelet activity (106). However, evidence from further studies is required to corroborate this. Other SSRIs, such as citalopram and fluoxetine, researches have demonstrated positive effects on reducing myocardial necrosis and improving prognosis (107, 108). However, animal experiments have yielded varying conclusions regarding the effect of antidepressant treatment on CHD, warranting further investigation. It’s essential to be cautious about potential drug interactions when prescribing SSRIs to patients concurrently taking other CHD medications, as some SSRIs are metabolized by the same hepatic enzyme (P450).
Unlike Western medicine treatment, traditional Chinese medicine (TCM) for treating depression with CHD is based on syndrome differentiation and treatment, with the advantages of multi-target, multi-pathway, overall treatment, and security good (109). Chen et al. (110) found through meta-analysis that, a well-known Chinese patent drug, Xinkeshu tablets (XKS), might benefit CHD patients experiencing depression after PCI by helping to improve their depression symptoms, TC and TG blood lipid levels. A meta-analysis and systematic review by Wang et al. (109) included 32 studies, which results showed that compared with the blank control groups, TCM was more beneficial in treating depression in patients with CHD [depression: OR = 3.27, 95% CI (1.67, 6.40), p = 0.0005, I2 = 0%], and the efficacy of TCM was not inferior to that of Western medicine [depression: OR = 1.97, 95%CI (0.73, 5.28), p = 0.18, I2 = 33%],. In addition, TCM also showed a significant advantage in improving the symptoms of angina pectoris in CHD patients with depression. And the most closely and simultaneously related to the pathological targets of CHD and depression, the most active compounds of TCM, such as quercetin, kaempferol, puerarin, baicalein, tanshinone IIa, mainly exert their effects through anti-inflammatory, antioxidant stress, and anti-damage/apoptosis underlying pathways. On the other hand, experimental studies have found that acupuncture, as one of the important therapies of traditional Chinese medicine, can significantly increase the serum levels of Glutathione and Catalase, the expression of Beclin-1 and LC3B proteins in the hippocampus of rats with depression and CHD. This therapeutic effect may be closely related to mitochondrial autophagy and oxidative stress (111, 112). From the perspective of combining Western medicine and traditional Chinese medicine in the future, providing antidepressant treatment for CHD patients with depression can be an effective and safe approach. Table 1 presents the medication details of antidepressant treatment for CHD with depression.
Psychological interventions, such as cognitive-behavioral therapy, interpersonal psychotherapy, and problem-solving therapy, have shown promise in reducing depressive symptoms, improving treatment compliance, and preventing relapse when combined with medication (113). These interventions have led to gradual improvements in the condition of CHD patients (114). However, while behavioral cognitive therapy has been associated with improved psychological status and quality of life, it does not significantly impact cardiac-related mortality (115). Additionally, psychological interventions have shown benefits in reducing cardiac deaths and alleviating psychological symptoms compared to conventional treatment, but they do not reduce overall mortality (111). Despite the lack of reduced endpoint events, antidepressant treatment has been shown to alleviate depressed mood and improve the quality of life in patients, making it a valuable therapeutic approach for CHD patients with comorbid depression. Exercise therapy, tailored to individual cardiac function and exercise endurance, can also play a role in reducing depression and CHD-related symptoms (115). This treatment modulates inflammatory factors and the parasympathetic nervous system, effectively reducing prevalence and mortality in co-morbid patients (115). Encouraging lifestyle changes can further support this approach. A comprehensive treatment approach that combines medication, psychological interventions, and exercise therapy should be actively administered to CHD patients with comorbid depression.
The relationship between depression and CHD is a significant concern, and it is crucial to determine whether combining antidepressant therapy with standard CHD treatment can improve symptoms and prognosis. Studies have shown that antidepressant treatment for patients with CHD and depression leads to substantial improvements in chest pain symptoms, depressive symptoms, and various cardiac parameters (109, 116, 117). Furthermore, effective antidepressant treatment in depressed patients after acute myocardial infarction (AMI) has been associated with reduced adverse cardiovascular events and improved survival during a 29-month follow-up (118). Interventions to address depressive symptoms in patients with acute coronary syndrome (ACS) have also proven effective in reducing the number of ACS-related re-hospitalizations. However, this benefit may not be sustained after treatment discontinuation (119). However, conflicting results have been reported, with some studies indicating that the benefits of antidepressant treatment for CHD are not significant (120–122). This suggests that although cognitive behavior therapy can alleviate depressive symptoms in CHD patients, it is only during short-term follow-up, and its impact on the long-term prognosis is not definite (120). Meanwhile, for patients with MI and depression, antidepressant treatment does not seem to reduce long-term major adverse cardiovascular events (MACE) or all-cause mortality (121). Similarly, Tully et al. concluded that while antidepressant treatment may lower the incidence of short-term MACE in CHD patients with depression, its impact on medium- and long-term MACE rates appears insignificant (123). Given the mixed findings, more research is needed to ascertain the true efficacy of antidepressant therapy in improving the prognosis of CHD patients with comorbid depression. Future studies should consider larger sample sizes, longer follow-up periods, and potentially tailored treatment approaches based on individual patient characteristics to determine the most effective course of action. Additionally, understanding the potential factors contributing to the varying results can help develop targeted interventions for specific subgroups of patients with CHD and depression.
Researchers have increasingly recognized the combination of multiple interventions for patients with comorbid CHD and depression. Studies have shown that screening CHD patients for depression and providing self-management instructions for depressive symptoms can improve clinical outcomes (122). Guidelines recommend various interventions for CHD patients with depression, including stress management, individual or group counseling, self-care practices, and supportive pharmacotherapy (22). For patients with depression and significant symptoms or psychosocial factors, a combination of interventions from different channels is often more effective, such as psychotherapy, pharmacotherapy, and collaborative care. Cardiovascular specialists need to be vigilant about screening for depressive symptoms in CHD patients to enhance clinical care and improve their quality of life. When administering antidepressant therapy to depressed patients with comorbid CHD, psychologists should comprehensively assess the potential effects of antidepressant medications on cardiovascular disease. In cases where a more holistic approach is necessary, a multidisciplinary approach can be adopted to develop the best treatment plan for the patients. By incorporating various interventions and considering each patient’s unique needs, healthcare professionals can effectively address both the cardiovascular and mental health aspects of comorbid CHD and depression, leading to better overall patient outcomes.
The interrelationship between depression and CHD has garnered significant global. However, there remains a lack of expert consensus and authoritative guidelines on clinical interventions for patients with these co-morbidities. Several reasons contribute to this situation:
1. Contradictory Conclusions: There are still divergent findings regarding the relationship between depression and CHD, and an international consensus on the precise pathogenesis of depression’s impact on CHD is yet to be established. This lack of clarity makes it challenging to develop targeted interventions based on the underlying mechanisms.
2. Complex Diagnosis and Treatment: Assessing psychiatric and psychological factors in clinical diagnosis and treatment requires specialized expertise, which can be intricate. This complexity presents challenges in comprehensive interventions for patients with comorbid depression and CHD.
3. Unclear Impact of Antidepressant Treatment: The effect of antidepressant treatment on CHD is not fully understood, and there are no definitive treatment guidelines to guide clinicians in managing patients with both conditions. Addressing these issues will necessitate further research and exploration in the future. Efforts should be made to understand the relationship between depression and CHD better, develop effective treatment guidelines, and improve the clinical management of patients with these co-morbidities. With continued investigation and collaboration, we can work towards providing more comprehensive and targeted care for individuals affected by both depression and CHD.
5 Conclusions
Currently, most of the existing literature on CHD co-morbid with depression focuses on observational studies, with limited research on the physiological mechanisms that underlie the impact of depression on CHD and potential therapeutic interventions. The identified physiological mechanisms linking depression and CHD primarily revolve around inflammatory response, HPA axis dysfunction, autonomic dysfunction, endothelial dysfunction, and platelet activation. However, there is still much to explore concerning mechanisms like D-type personality and genetics. Although it is generally agreed upon that combining pharmacological and non-pharmacological treatments offers the best approach for managing CHD and depression, further comprehensive experimental and clinical studies are essential to validate its efficacy and safety. Additionally, developing treatment guidelines for managing these co-morbidities requires greater attention. In addition to the existing knowledge, it is important to comment on future research and clinical practice prospects. Moving forward, a more integrated and interdisciplinary approach is needed to unravel the complex interplay between depression and CHD. This could involve exploring novel mechanisms such as the role of epigenetic factors, immune dysregulation, and the gut-brain axis. Additionally, leveraging technological advancements, such as wearable devices and mobile health applications, could aid in monitoring and managing the co-morbidities more effectively. Furthermore, identifying specific subgroups of patients who are more vulnerable to the detrimental effects of depression on CHD could help tailor interventions and improve patient outcomes. To advance our understanding and treatment of CHD combined with depression, it is crucial to conduct more in-depth investigations to identify the primary mechanisms and their interactions. This will enable more effective and safe interventions, providing patients with a broader range of treatment options and ultimately enhancing their overall quality of life. By dedicating efforts to research further, we can improve care for patients with CHD and depression.
Author contributions
LX: Conceptualization, Writing – original draft. XZ: Formal analysis, Writing – review & editing. DS: Conceptualization, Writing – original draft. YZ: Methodology, Writing – review & editing.
Funding
The author(s) declare financial support was received for the research, authorship, and/or publication of this article. This work was supported by the Basic Scientific Research Business Expenses of Central Public Welfare Scientific Research Institutes (No. ZZ13-YQ008) and Major Research Project of Scientific and Technological Innovation Project of Chinese Academy of Traditional Chinese Medicine (No. CI2021A03115).
Conflict of interest
The authors declare that the research was conducted in the absence of any commercial or financial relationships that could be construed as a potential conflict of interest.
Publisher’s note
All claims expressed in this article are solely those of the authors and do not necessarily represent those of their affiliated organizations, or those of the publisher, the editors and the reviewers. Any product that may be evaluated in this article, or claim that may be made by its manufacturer, is not guaranteed or endorsed by the publisher.
References
1. Lichtman J, Froelicher E, Blumenthal J, Carney R, Doering L, Frasure-Smith N, et al. Depression as a risk factor for poor prognosis among patients with acute coronary syndrome: systematic review and recommendations: a scientific statement from the American Heart Association. Circulation (2014) 129(12):1350–69. doi: 10.1161/CIR.0000000000000019
2. Jha MK, Qamar A, Vaduganathan M, Charney DS, Murrough JW. Screening and management of depression in patients with cardiovascular disease. J Am Coll Cardiol (2019) 73(14):1827–45. doi: 10.1016/j.jacc.2019.01.041
3. Hasin DS, Sarvet AL, Meyers JL, Saha TD, Ruan WJ, Stohl M, et al. Epidemiology of adult DSM-5 major depressive disorder and its specifiers in the United States. JAMA Psychiatry (2018) 75(4):336–46. doi: 10.1001/jamapsychiatry.2017.4602
4. Penninx BW. Depression and cardiovascular disease: Epidemiological evidence on their linking mechanisms. Neurosci Biobehav Rev (2017) 74(Pt B):277–86. doi: 10.1016/j.neubiorev.2016.07.003
5. Lim GY, Tam WW, Lu Y, Ho CS, Zhang MW, Ho RC. Prevalence of depression in the community from 30 countries between 1994 and 2014. Sci Rep (2018) 8(1):2861–70. doi: 10.1038/s41598-018-21243-x
6. Jha MK, Minhajuddin A, South C, Rush AJ, Trivedi MH. Worsening anxiety, irritability, insomnia, or panic predicts poorer antidepressant treatment outcomes: clinical utility and validation of the concise associated symptom tracking (CAST) scale. Int J Neuropsychopharmacol (2018) 21:325–32. doi: 10.1093/ijnp/pyx097
7. Redelmeier DA, Kraus NC. Patterns in patient access and utilization of online medical records: analysis of myChart. J Med Internet Res (2018) 20:e43. doi: 10.2196/jmir.8372
8. De Hert M, Detraux J, Vancampfort D. The intriguing relationship between coronary heart disease and mental disorders. Dialogues Clin Neurosci (2018) 20(1):31–40. doi: 10.31887/DCNS.2018.20.1/mdehert
9. Carney RM, Freedland KE, Steinmeyer BC, Rubin EH, Rich MW. Residual symptoms after treatment for depression in patients with coronary heart disease. Psychosom Med (2018) 80(4):385–92. doi: 10.1097/PSY.0000000000000570
10. Hagström E, Norlund F, Stebbins A, Armstrong PW, Chiswell K, Granger CB, et al. Psychosocial stress and major cardiovascular events in patients with stable coronary heart disease. J Intern Med (2018) 283(1):83–92. doi: 10.1111/joim.12692
11. Kuhlmann SL, Arolt V, Haverkamp W, Martus P, Ströhle A, Waltenberger J, et al. Prevalence, 12-month prognosis, and clinical management need of depression in coronary heart disease patients: A prospective cohort study. Psychother Psychosom (2019) 88(5):300–11. doi: 10.1159/000501502
12. Gomez-Caminero A, Blumentals WA, Russo LJ, Brown RR, Castilla-Puentes R. Does panic disorder increase the risk of coronary heart disease? A cohort study of a national managed care database. Psychosom Med (2005) 67(5):688–91. doi: 10.1097/01.psy.0000174169.14227.1f
13. Daskalopoulou M, George J, Walters K, Osborn DP, Batty GD, Stogiannis D, et al. Depression as a risk factor for the initial presentation of twelve cardiac, cerebrovascular, and peripheral arterial diseases: data linkage study of 1.9 million women and men. PloS One (2016) 11:e0153838. doi: 10.1371/journal.pone.0153838
14. Berkman LF, Blumenthal J, Burg M, Carney RM, Catellier D, Cowan MJ, et al. Effects of treating depression and low perceived social support on clinical events after myocardial infarction: the Enhancing Recovery in Coronary Heart Disease Patients (ENRICHD) Randomized Trial. JAMA (2003) 289:3106–16. doi: 10.1001/jama.289.23.3106
15. Gan Y, Gong Y, Tong X, Sun H, Cong Y, Dong X, et al. Depression and the risk of coronary heart disease: a meta-analysis of prospective cohort studies. BMC Psychiatry (2014) 14:371. doi: 10.1186/s12888-014-0371-z
16. Gehi A, Haas D, Pipkin S, Whooley MA. Association of anxiety and depression with all-cause mortality in individuals with coronary heart disease. J Am Heart Assoc (2013) 2(2):e000068. doi: 10.1161/JAHA.112.000068
17. Gehi A, Haas D, Pipkin S, Whooley MA. Depression and medication adherence in outpatients with coronary heart disease: Findings from the heart and soul Study. Arch Intern Med (2005) 165:2508–13. doi: 10.1001/archinte.165.21.2508
18. Win S, Parakh K, Eze-Nliam CM, Gottdiener JS, Kop WJ, Ziegelstein RC. Depressive symptoms, physical inactivity and risk of cardiovascular mortality in older adults: The Cardiovascular Health Study. Heart (2011) 97(6):500–5. doi: 10.1136/hrt.2010.209767
19. Zhu CF, Zhang M. Research progress on chronic stress and coronary heart disease. Adv Cardiovasc Dis (2018) 39(4):611–4.
20. Hassamal S. Chronic stress, neuroinflammation, and depression: an overview of pathophysiological mechanisms and emerging anti-inflammatories. Front Psychiatry (2023) 14:1130989. doi: 10.3389/fpsyt.2023.1130989
21. Lin IM, Wang SY, Chu IH, Lu YH, Lee CS, Lin TH, et al. The association of type D personality with heart rate variability and lipid profiles among patients with coronary artery disease. Int J Behav Med (2017) 24(1):101–9. doi: 10.1007/s12529-016-9571-x
22. Vaccarino V, Badimon L, Bremner JD, Cenko E, Cubedo J, Dorobantu M, et al. Depression and coronary heart disease: 2018 position paper of the ESC working group on coronary pathophysiology and microcirculation. Eur Heart J (2020) 41(17):1687–96. doi: 10.1093/eurheartj/ehy913
23. Fioranelli M, Bottaccioli AG, Bottaccioli F, Bianchi M, Rovesti M, Roccia MG. Stress and inflammation in coronary artery disease: a review psychoneuro endocrine immunology-based. Front Immunol (2018) 9:2. doi: 10.3389/fimmu.2018.02031
24. Renna ME, O'Toole MS, Spaeth PE, Lekander M, Mennin DS. The association between anxiety, traumatic stress, and obsessive - compulsive disorders and chronic inflammation: A systematic review and meta - analysis. Depress Anxiety (2018) 35(11):1081–94. doi: 10.1002/da.22790
25. Kalkman HO. The association between vascular inflammation and depressive disorder. Causality, biomarkers and targeted treatment. Pharm (Basel) (2020) 13(5):92.
26. Zhang XL, Zhao YF, Ren YC, Wu ZH, Wang YY, Hu XT. The relationship between anxiety and depression, inflammatory response, and endothelial function in patients with coronary heart disease. Chin J Evidence Based Cardiovasc Med (2018) 10(2):199–202. doi: 10.3969/j.issn.1674-4055.2018.02.20
27. Li M, Kouzmina E, McCusker M, Rodin D, Boutros PC, Paige CJ, et al. Pro- and anti- inflammatory cytokine associations with major depression in cancer patients. Psychooncology (2017) 26(12):2149–56. doi: 10.1002/pon.4316
28. Yang N, Sun S, Duan G, Lv K, Liang C, Zhang L, et al. Advances of endothelial progenitor cells in the development of depression. Front Cell Neurosci (2021) 15:608656. doi: 10.3389/fncel.2021.608656
29. Wang M, Pan W, Xu Y, Zhang J, Wan J, Jiang H. Microglia-mediated neuroinflammation: A potential target for the treatment of cardiovascular diseases. J Inflammation Res (2022) 15:3083–94. doi: 10.2147/JIR.S350109
30. Sforzini L, Pariante CM, Palacios JE, Tylee A, Carvalho LA, Viganò CA, et al. Inflammation associated with coronary heart disease predicts onset of depression in a three-year prospective follow-up: A preliminary study. Brain Behav Immun (2019) 81:659–64. doi: 10.1016/j.bbi.2019.07.023
31. Empana JP, Sykes DH, Luc G, Juhan-Vague I, Arveiler D, Ferrieres J, et al. Contributions of depressive mood and circulation inflammatory markers to coronary heart disease in healthy European men: The prospective epidemiological study of myocardial infarction (PRIME). Circulation (2005) 111:2299–305. doi: 10.1161/01.CIR.0000164203.54111.AE
32. Tully PJ, Ang SY, Lee EJ, Bendig E, Bauereiß N, Bengel J, et al. Psychological and pharmacological interventions for depression in patients with coronary artery disease. Cochrane Database Syst Rev (2021) 12(12):CD008012. doi: 10.1002/14651858.CD008012.pub4
33. Khandaker GM, Zuber V, Rees JMB, Carvalho L, Mason AM, Foley CN, et al. Shared mechanisms between coronary heart disease and depression: Findings from a large UK general population-based cohort. Mol Psychiatry (2020) 25(7):1477–86. doi: 10.1038/s41380-019-0395-3
34. Masafi S, Saadat SH, Tehranchi K, Olya R, Heidari M, Malihialzackerini S, et al. Effect of stress, depression and type D personality on immune system in the incidence of coronary artery disease. Open Access Maced J Med Sci (2018) 6(8):1533–44. doi: 10.3889/oamjms.2018.217
35. Grande D, Terlizzese P, Gioia MI, Parisi G, Giagulli VA, Triggiani V, et al. New frontiers in the therapeutic approach of patients with cardiovascular and endocrine diseases. Endocr Metab Immune Disord Drug Targets (2019) 19(5):605–21. doi: 10.2174/1871530319666190101151542
36. Mohd Azmi NAS, Juliana N, Azmani S, Mohd Effendy N, Abu IF, Mohd Fahmi Teng NI, et al. Cortisol on circadian rhythm and its effect on cardiovascular system. Int J Environ Res Public Health (2021) 18(2):676. doi: 10.3390/ijerph18020676
37. Xinxing W, Wei L, Lei W, Rui Z, Baoying J, Lingjia Q. A neuroendocrine mechanism of co-morbidity of depression-like behavior and myocardial injury in rats. PloS One (2014) 9(2):e88427. doi: 10.1371/journal.pone.0088427
38. Pinter A, Szatmari S Jr, Horvath T, Penzlin AI, Barlinn K, Siepmann M, et al. Cardiac dysautonomia in depression - heart rate variability biofeedback as a potential add-on therapy. Neuropsychiatr Dis Treat (2019) 15:1287–310. doi: 10.2147/NDT.S200360
39. Siepmann M, Weidner K, Petrowski K, Siepmann T. Heart rate variability: A measure of cardiovascular health and possible therapeutic target in dysautonomic mental and neurological disorders. Appl Psychophysiol Biofeedback (2022) 47(4):273–87. doi: 10.1007/s10484-022-09572-0
40. Hartmann R, Schmidt FM, Sander C, Hegerl U. Heart rate variability as indicator of clinical state in depression. Front Psychiatry (2019) 9:735. doi: 10.3389/fpsyt.2018.00735
41. Blase K, Vermetten E, Lehrer P, Gevirtz R. Neurophysiological approach by self-control of your stress-related autonomic nervous system with depression, stress and anxiety patients. Int J Environ Res Public Health (2021) 18(7):3329. doi: 10.3390/ijerph18073329
42. Nikkheslat N, Zunszain PA, Horowitz MA, Barbosa IG, Parker JA, Myint AM, et al. Insufficient glucocorticoid signaling and elevated inflammation in coronary heart disease patients with comorbid depression. Brain Behav Immun (2015) 48:8–18. doi: 10.1016/j.bbi.2015.02.002
43. Čukić M, Savić D, Sidorova J. When heart beats differently in depression: review of nonlinear heart rate variability measures. JMIR Ment Health (2023) 10:e40342.
44. Theodosis-Nobelos P, Asimakopoulou E, Madianos M. [Pathophysiological mechanisms of major mental disorders related to cardiovascular disease]. Psychiatriki (2022) 33(2):113–23.
45. Carney RM, Freedland KE, Steinmeyer BC, Rubin EH, Stein PK, Rich MW. Nighttime heart rate predicts response to depression treatment in patients with coronary heart disease. J Affect Disord (2016) 200:165–71. doi: 10.1016/j.jad.2016.04.051
46. Luo Y, Zhang S, Zheng R, Xu L, Wu J. Effects of depression on heart rate variability in elderly patients with stable coronary artery disease. J Evid Based Med (2018) 11(4):242–5. doi: 10.1111/jebm.12310
47. Borrione L, Brunoni AR, Sampaio-junior B, Aparicio LM, Kemp AH, Benseñor I, et al. Associations between symptoms of depression and heart rate variability: An exploratory study. Psychiatry Res (2018) 262:482–7. doi: 10.1016/j.psychres.2017.09.028
48. Kircanski K, Williams LM, Gotlib IH. Heart rate variability as a biomarker of anxious depression response to antidepressant medication. Depress Anxiety (2019) 36(1):63–71. doi: 10.1002/da.22843
49. Shi S, Liang J, Liu T, Yuan X, Ruan B, Sun L, et al. Depression increases sympathetic activity and exacerbates myocardial remodeling after myocardial infarction: Evidence from an animal experiment. PloS One (2014) 9(7):e101734. doi: 10.1371/journal.pone.0101734
50. Ormonde do Carmo MB, Mendes-Ribeiro AC, Matsuura C, Pinto VL, Mury WV, Pinto NO, et al. Major depression induces oxidative stress and platelet hyperaggregability. J Psychiatr Res (2015) 61:19–24. doi: 10.1016/j.jpsychires.2014.12.009
51. Williams MS, Ziegelstein RC, Mccann UD, Gould NF, Ashvetiya T, Vaidya D. Platelet serotonin signaling in patients with cardiovascular disease and comorbid depression. Psychosom Med (2019) 81(4):352–62. doi: 10.1097/PSY.0000000000000689
52. Peng M, Pu WD, Yu S, Zhao WF, Qiu XY, Wang HZ, et al. Association of BDNF gene polymorphisms with co-morbid depression in coronary heart disease and its mechanism of platelet activity. Chin J Clin Psychol (2018) 26(2):230–3. doi: 10.16128/j.cnki.1005-3611.2018.02.005
53. Rieder M, Gauchel N, Bode C, Duerschmied D. Serotonin: a platelet hormone modulating cardiovascular disease. J Thromb Thrombolysis (2021) 52(1):42–7. doi: 10.1007/s11239-020-02331-0
54. Delle Chiaie R, Capra E, Salviati M, Trabucchi G, Pancheri C, Corrado A, et al. Persistence of subsyndromal residual symptoms after remission of major depression in patients without cardiovascular disease may condition maintenance of elevated platelet factor 4 and beta-thromboglobulin plasma levels. J Affect Disord (2013) 150(2):664–7. doi: 10.1016/j.jad.2013.03.017
55. Zhang J. Biomarkers of endothelial activation and dysfunction in cardiovascular diseases. Rev Cardiovasc Med (2022) 23(2):73. doi: 10.31083/j.rcm2302073
56. Aydin Sunbul E, Sunbul M, Gulec H. The impact of major depression on heart rate variability and endothelial dysfunction in patients with stable coronary artery disease. Gen Hosp Psychiatry (2017) 44:4–9. doi: 10.1016/j.genhosppsych.2016.10.006
57. Van Dooren FE, Schram MT, Schalkwijk CG, Stehouwer CD, Henry RM, Dagnelie PC, et al. Associations of low grade inflammation and endothelial dysfunction with depression - The Maastricht Study. Brain Behav Immun (2016) 56:390–6. doi: 10.1016/j.bbi.2016.03.004
58. Janaszak-Jasiecka A, Siekierzycka A, Płoska A, Dobrucki IT, Kalinowski L. Endothelial dysfunction driven by hypoxia-the influence of oxygen deficiency on NO bioavailability. Biomolecules (2021) 11(7):982. doi: 10.3390/biom11070982
59. Greaney JL, Saunders EFH, Santhanam L, Alexander LM. Oxidative stress contributes to microvascular endothelial dysfunction in men and women with major depressive disorder. Circ Res (2019) 124(4):564–74. doi: 10.1161/CIRCRESAHA.118.313764
60. Gacar G, Gocmez SS, Halbutoğulları ZS, Kılıç KC, Kaya A, Yazir Y, et al. Resveratrol improves vascular endothelial dysfunction in the unpredictable chronic mild stress model of depression in rats by reducing inflammation. Behav Brain Res (2023) 438:114186. doi: 10.1016/j.bbr.2022.114186
61. Husain MI, Chaudhry IB, Khoso AB, Kiran T, Khan N, Ahmad F, et al. Effect of adjunctive simvastatin on depressive symptoms among adults with treatment-resistant depression: A randomized clinical trial. JAMA Netw Open (2023) 6(2):e230147. doi: 10.1001/jamanetworkopen.2023.0147
62. Pinto B, Conde T, Domingues I, Domingues MR. Adaptation of lipid profiling in depression disease and treatment: A critical review. Int J Mol Sci (2022) 23(4):2032. doi: 10.3390/ijms23042032
63. Yu XB, Zhang HN, Dai Y, Zhou ZY, Xu RA, Hu LF, et al. Simvastatin prevents and ameliorates depressive behaviors via neuroinflammatory regulation in mice. J Affect Disord (2019) 245:939–49. doi: 10.1016/j.jad.2018.11.086
64. Paduchová Z, Katrenčíková B, Vaváková M, Laubertová L, Nagyová Z, Garaiova I, et al. The effect of omega-3 fatty acids on thromboxane, brain-derived neurotrophic factor, homocysteine, and vitamin D in depressive children and adolescents: randomized controlled trial. Nutrients (2021) 13(4):1095. doi: 10.3390/nu13041095
65. Carney RM, Freedland KE, Rubin EH, Rich MW, Steinmeyer BC, Harris WS. A randomized placebo-controlled trial of omega-3 and sertraline in depressed patients with or at risk for coronary heart disease. J Clin Psychiatry (2019) 80(4):19m12742. doi: 10.4088/JCP.19m12742
66. Mori TA. Reprint of: Marine OMEGA-3 fatty acids in the prevention of cardiovascular disease. Fitoterapia (2018) 126:8–15. doi: 10.1016/j.fitote.2018.04.003
67. Zhang LJ, Zeng XT, Zhao MJ, He DF, Liu JY, Liu MY. The important effect of 5-HTTLPR polymorphism on the risk of depression in patients with coronary heart disease: a meta-analysis. BMC Cardiovasc Disord (2020) 20(1):141. doi: 10.1186/s12872-020-01424-1
68. Lu Y, Wang Z, Georgakis MK, Lin H, Zheng L. Genetic liability to depression and risk of coronary artery disease, myocardial infarction, and other cardiovascular outcomes. J Am Heart Assoc (2021) 10(1):e017986. doi: 10.1161/JAHA.120.017986
69. deGeus EJ. Genetic pleiotropy in depression and coronary artery disease. Psychosom Med (2006) 68:185–6. doi: 10.1097/01.psy.0000208628.90274.bc
70. Lam D, Ancelin ML, Ritchie K, Freak-Poli R, Saffery R, Ryan J. Genotype-dependent associations between serotonin transporter gene (SLC6A4) DNA methylation and late-life depression. BMC Psychiatry (2018) 18(1):282. doi: 10.1186/s12888-018-1850-4
71. Meyer T, Rothe I, Staab J, Deter HC, Fangauf SV, Hamacher S, et al. Length polymorphisms in the angiotensin I-converting enzyme gene and the serotonin-transporter-linked polymorphic region constitute a risk haplotype for depression in patients with coronary artery disease. Biochem Genet (2020) 58(4):631–48. doi: 10.1007/s10528-020-09967-w
72. Das S, Halushka MK. Extracellular vesicle microRNA transfer in cardiovascular disease. Cardiovasc Pathol (2015) 24(4):199–206. doi: 10.1016/j.carpath.2015.04.007
73. Pfeifer P, Werner N, Jansen F. Role and function of microRNAs in extracellular vesicles in cardiovascular biology. BioMed Res Int (2015) 2015:161393. doi: 10.1155/2015/161393
74. Li H, Chen M, Feng Q, Zhu L, Bai Z, Wang B, et al. MicroRNA-34a in coronary heart disease: Correlation with disease risk, blood lipid, stenosis degree, inflammatory cytokines, and cell adhesion molecules. J Clin Lab Anal (2022) 36(1):e24138. doi: 10.1002/jcla.24138
75. Tsai WC, Hsu SD, Hsu CS, Lai TC, Chen SJ, Shen R, et al. MicroRNA-122 plays a critical role in liver homeostasis and hepatocarcinogenesis[J]. J Clin Invest (2012) 122(8):2884–97. doi: 10.1172/JCI63455
76. Mouillet-Richard S, Baudry A, Launay JM, Kellermann O. MicroRNAs and depression. Neurobiol Dis (2012) 46(2):272–8. doi: 10.1016/j.nbd.2011.12.035
77. Gururajan A, Naughton ME, Scott KA, O'Connor RM, Moloney G, Clarke G, et al. MicroRNAs as biomarkers for major depression: a role for let-7b and let-7c. Trans Psychiatry Nat Publishing Group (2016) 6(8):e862–2. doi: 10.1038/tp.2016.131
78. Bai M, Zhu X, Zhang Y, Zhang S, Zhang L, Xue L, et al. Abnormal Hippocampal BDNF and miR-16 Expression Is Associated with Depression-Like Behaviors Induced by Stress during Early Life. PloS ONE Public Library Sci (2012) 7(10):e46921. doi: 10.1371/journal.pone.0046921
79. Baudry A, Mouillet-Richard S, Schneider B, Launay JM, Kellermann O. MiR-16 targets the serotonin transporter: A new facet for adaptive responses to antidepressants. Science Am Assoc Advancement Sci (2010) 329(5998):1537–41. doi: 10.1126/science.1193692
80. Moya PR, Wendland JR, Salemme J, Fried RL, Murphy DL. miR-15a and miR-16 regulate serotonin transporter expression in human placental and rat brain raphe cells. Int J Neuropsychopharmacol (2013) 16(3):621–9. doi: 10.1017/S1461145712000454
81. Kawashima H, Numakawa T, Kumamaru E, Adachi N, Mizuno H, Ninomiya M, et al. Glucocorticoid attenuates brain-derived neurotrophic factor-dependent upregulation of glutamate receptors via the suppression of microRNA-132 expression. Neuroscience (2010) 165(4):1301–11. doi: 10.1016/j.neuroscience.2009.11.057
82. Su M, Hong J, Zhao Y, Liu S, Xue X. MeCP2 controls hippocampal brain-derived neurotrophic factor expression via homeostatic interactions with microRNA-132 in rats with depression. Mol Med Reports Spandidos Publications (2015) 12(4):5399–406. doi: 10.3892/mmr.2015.4104
83. Yi LT, Li J, Liu BB, Luo L, Liu Q, Geng D. BDNF–ERK–CREB signalling mediates the role of miR-132 in the regulation of the effects of oleanolic acid in male mice. J Psychiatry Neuroscience J Psychiatry Neurosci (2014) 39(5):348–59. doi: 10.1503/jpn.130169
84. Chen X, Zhang H, Ren S, Ding Y, Remex NS, Bhuiyan MS, et al. Gut microbiota and microbiota-derived metabolites in cardiovascular diseases. Chin Med J (2023) 136(19):2269–84. doi: 10.1097/CM9.0000000000002206
85. Lau K, Srivatsav V, Rizwan A, Nashed A, Liu R, Shen R, et al. Bridging the gap between gut microbial dysbiosis and cardiovascular diseases. Nutrients (2017) 9(8):859–9. doi: 10.3390/nu9080859
86. Bogiatzi C, Gloor G, Allen-Vercoe E, Reid G, Wong RG, Urquhart BL, et al. Metabolic products of the intestinal microbiome and extremes of atherosclerosis. Atherosclerosis (2018), 27391–97. doi: 10.1016/j.atherosclerosis.2018.04.015
87. Cui L, Zhao T, Hu H, Zhang W, Hua X. Association study of gut flora in coronary heart disease through high-throughput sequencing. BioMed Res Int (2017) 2017:3796359. doi: 10.1155/2017/3796359
88. Feng Q, Liu Z, Zhong S, Li R, Xia H, Jie Z, et al. Integrated metabolomics and metagenomics analysis of plasma and urine identified microbial metabolites associated with coronary heart disease. Sci Rep (2016) 6(1):22525. doi: 10.1038/srep22525
89. Amar J, Lange C, Payros G, Garret C, Chabo C, Lantieri O, et al. Blood microbiota dysbiosis is associated with the onset of cardiovascular events in a large general population: the D.E.S.I.R. study. PloS One (2017) 8(1):e54461. doi: 10.1371/journal.pone.0054461
90. Ahmad Adila F, Ward NC, Girish D. The gut microbiome and heart failure. Curr Opin Cardiol (2019) 34(2):225–32. doi: 10.1097/HCO.0000000000000598
91. Bravo JA, Forsythe P, Chew MV, Escaravage E, Savignac HM, Dinan TG, et al. Ingestion of Lactobacillus strain regulates emotional behavior and central GABA receptor expression in a mouse via the vagus nerve. Proc Natl Acad Sci United States America (2011) 108(38):16050–5. doi: 10.1073/pnas.1102999108
92. Grice EA, Segre JA. The human microbiome: our second genome. Annu Rev Genomics Hum Genet (2012) 13(1):151–70. doi: 10.1146/annurev-genom-090711-163814
93. Rothschild D, Weissbrod O, Barkan E, Kurilshikov A, Korem T, Zeevi D, et al. Environment dominates over host genetics in shaping human gut microbiota. Nature (2018) 555(7695):210–5. doi: 10.1038/nature25973
94. Zhu B, Wang X, Li L. Human gut microbiome: the second genome of human body. Protein Cell (2010) 1(8):718–25. doi: 10.1007/s13238-010-0093-z
95. Mohajeri MH, La Fata G, Steinert RE, Weber P. Relationship between the gut microbiome and brain function. Nutr Rev (2018) 76(7):481–96. doi: 10.1093/nutrit/nuy009
96. Pirbaglou M, Katz J, de Souza RJ, Stearns JC, Motamed M, Ritvo P. Probiotic supplementation can positively affect anxiety and depressive symptoms: a systematic review of randomized controlled trials. Nutr Res (2016) 36(9):889–98. doi: 10.1016/j.nutres.2016.06.009
97. Wallace CJK, Miley R. Erratum to: The effects of probiotics on depressive symptoms in humans: a systematic review. Ann Gen Psychiatry (2017) 16(1):18. doi: 10.1186/s12991-017-0141-7
98. Lin P, Ding B, Feng C, Yin S, Zhang T, Qi X, et al. Prevotella and Klebsiella proportions in fecal microbial communities are potential characteristic parameters for patients with major depressive disorder. J Affect Disord (2017), 207300–304. doi: 10.1016/j.jad.2016.09.051
99. Jiang H, Ling Z, Zhang Y, Mao H, Ma Z, Yin Y, et al. Altered fecal microbiota composition in patients with major depressive disorder. Brain behavior Immun (2015), 48186–94. doi: 10.1016/j.bbi.2015.03.016
100. Zheng P, Zeng B, Zhou C, Liu M, Fang Z, Xu X, et al. Gut microbiome remodeling induces depressive-like behaviors through a pathway mediated by the host's metabolism. Mol Psychiatry (2016) 21(6):786–96. doi: 10.1038/mp.2016.44
101. Siu AL, the USPSTF. Screening for depression in adults: Us preventive services task force recommendation statement. JAMA (2016) 315:380–7. doi: 10.1001/jama.2015.18392
102. Bhattacharjee S, Goldstone L, Vadiei N, Lee JK, Burke WJ. Depression screening patterns, predictors, and trends among adults without a depression diagnosis in ambulatory settings in the United States. Psychiatr Serv (2018) 69(10):1098–100. doi: 10.1176/appi.ps.201700439
103. Kim JM, Stewart R, Lee YS, Lee HJ, Kim MC, Kim JW, et al. Effect of escitalopram vs placebo treatment for depression on long-term cardiac outcomes in patients with acute coronary syndrome: A randomized clinical trial. JAMA (2018) 320:350–8. doi: 10.1001/jama.2018.9422
104. Ronaldson A, Carvalho LA, Kostich K, Lazzarino AI, Urbanova L, Steptoe A. The effects of six-day SSRI administration on diurnal cortisol secretion in healthy volunteers. Psychopharmacol (Berl) (2018) 235(12):3415–22. doi: 10.1007/s00213-018-5050-1
105. Pina IL, Di Palo KE, Ventura HO. Psychopharmacology and cardiovascular disease. J Am Coll Cardiol (2018) 71:2346–59. doi: 10.1016/j.jacc.2018.03.458
106. Gutlapalli SD, Prakash K, Swarnakari KM, Bai M, Manoharan MP, Raja R, et al. The risk of fatal arrhythmias associated with sertraline in patients with post-myocardial infarction depression. Cureus (2022) 14(9):e28946. doi: 10.7759/cureus.28946
107. Croatto G, Vancampfort D, Miola A, Olivola M, Fiedorowicz JG, Firth J, et al. The impact of pharmacological and non-pharmacological interventions on physical health outcomes in people with mood disorders across the lifespan: An umbrella review of the evidence from randomized controlled trials. Mol Psychiatry (2023) 28(1):369–90. doi: 10.1038/s41380-022-01770-w
108. Guo S, Chen L, Cheng S, Xu H. Comparative cardiovascular safety of selective serotonin reuptake inhibitors (SSRIs) among Chinese senile depression patients: A network meta-analysis of randomized controlled trials. Med (Baltimore) (2019) 98(22):e15786. doi: 10.1097/MD.0000000000015786
109. Wang B, Teng Y, Li Y, Lai S, Wu Y, Chen S, et al. Evidence and characteristics of traditional chinese medicine for coronary heart disease patients with anxiety or depression: A meta-analysis and systematic review. Front Pharmacol (2022) 13:854292. doi: 10.3389/fphar.2022.854292
110. Chen M, Liu M, Guo X, Zhou J, Yang H, Zhong G, et al. Effects of Xinkeshu tablets on coronary heart disease patients combined with anxiety and depression symptoms after percutaneous coronary intervention: A meta-analysis. Phytomedicine (2022) 104:154243. doi: 10.1016/j.phymed.2022.154243
111. Ren ZK. A study on the regulation of GSH and Beclin-1 by electroacupuncture in depression combined with coronary heart disease model rats. Shenyang, China: Liaoning University of Traditional Chinese Medicine (2022).
112. Bai S. The effect of electroacupuncture on the expression of CAT and LC3B proteins in depression combined with coronary heart disease model rats. Shenyang, China: Liaoning University of Traditional Chinese Medicine (2022).
113. Westermair AL, Schaich A, Willenborg B, Willenborg C, Nitsche S, Schunkert H, et al. Utilization of mental health care, treatment patterns, and course of psychosocial functioning in Northern German coronary artery disease patients with depressive and/or anxiety disorders. Front Psychiatry (2018) 9:75. doi: 10.3389/fpsyt.2018.00075
114. Reavell J, Hopkinson M, Clarkesmith D, Lane DA. Effectiveness of cognitive behavioral therapy for depression and anxiety in patients with cardiovascular disease: A systematic review and meta-analysis. Psychosom Med (2018) 80(8):742–53. doi: 10.1097/PSY.0000000000000626
115. Wang L, Sun Y, Zhan J, Wu Z, Zhang P, Wen X, et al. Effects of exercise therapy on anxiety and depression in patients with coronary heart disease: A meta-analysis of a randomized controlled study. Front Cardiovasc Med (2021) 8:730155. doi: 10.3389/fcvm.2021.730155
116. Li X, Yang S, Wang Y, Yang B, Zhang J. Effects of a transtheoretical model - based intervention and motivational interviewing on the management of depression in hospitalized patients with coronary heart disease: a randomized controlled trial. BMC Public Health (2020) 20(1):420. doi: 10.1186/s12889-020-08568-x
117. Chen M, Men L, Ou L, Li T, Li M, Zhong X, et al. Effectiveness and safety of modified 'Huoxue Shugan' formulas on coronary heart disease combined with depression: protocol for a systematic review. BMJ Open (2018) 8(11):e022868. doi: 10.1136/bmjopen-2018-022868
118. Banankhah SK, Friedmann E, Thomas S. Effective treatment of depression improves post-myocardial infarction survival. World J Cardiol (2015) 7:215–23. doi: 10.4330/wjc.v7.i4.215
119. Kim JM, Stewart R, Kang HJ, Kim SY, Kim JW, Lee HJ, et al. Long-term cardiac outcomes of depression screening, diagnosis and treatment in patients with acute coronary syndrome: the DEPACS study. Psychol Med (2021) 51(6):964–74. doi: 10.1017/S003329171900388X
120. Nuraeni A, Suryani S, Trisyani Y, Sofiatin Y. Efficacy of cognitive behavior therapy in reducing depression among patients with coronary heart disease: an updated systematic review and meta-analysis of RCTs. Healthcare (Basel) (2023) 11(7):943. doi: 10.3390/healthcare11070943
121. Chaitman BR, Alexander KP, Cyr DD, Berger JS, Reynolds HR, Bangalore S, et al. Myocardial infarction in the ISCHEMIA trial: impact of different definitions on incidence, prognosis, and treatment comparisons. Circulation (2021) 143(8):790–804. doi: 10.1161/CIRCULATIONAHA.120.047987
122. Lowe B, Blankenberg S, Wegscheider K, König HH, Walter D, Murray AM, et al. Depression screening with patient-targeted feedback in cardiology: DEPSCREEN-INFO randomized clinical trial. Br J Psychiatry (2018) 210(2):132–9. doi: 10.1192/bjp.bp.116.184168
Keywords: coronary heart disease, depression, bipolar heart disease, mechanisms, antidepressant treatment
Citation: Xu L, Zhai X, Shi D and Zhang Y (2024) Depression and coronary heart disease: mechanisms, interventions, and treatments. Front. Psychiatry 15:1328048. doi: 10.3389/fpsyt.2024.1328048
Received: 27 October 2023; Accepted: 24 January 2024;
Published: 09 February 2024.
Edited by:
Pei Jiang, Jining First People’s Hospital, ChinaReviewed by:
Angelos Halaris, Loyola University Chicago, United StatesPao-Huan Chen, Taipei Medical University, Taiwan
Copyright © 2024 Xu, Zhai, Shi and Zhang. This is an open-access article distributed under the terms of the Creative Commons Attribution License (CC BY). The use, distribution or reproduction in other forums is permitted, provided the original author(s) and the copyright owner(s) are credited and that the original publication in this journal is cited, in accordance with accepted academic practice. No use, distribution or reproduction is permitted which does not comply with these terms.
*Correspondence: Dazhuo Shi, c2hpZGF6aHVvQGNhY21zLmNu; Ying Zhang, ZWNobzk5MzI3MkBzaW5hLmNvbQ==
†These authors share first authorship