- Department of Orthopedic Surgery, Wenzhou Hospital of Integrated Traditional Chinese and Western Medicine, Wenzhou, Zhejiang, China
Background: While increasing concerns arise about the health effects of environmental pollutants, the relationship between blood manganese (Mn) and sarcopenia has yet to be fully explored in the general population.
Objective: This study aims to investigate the association between blood manganese (Mn) levels and sarcopenia in adults.
Methods: In our study, we evaluated 8,135 individuals aged 18–59 years, utilizing data from the National Health and Nutrition Examination Survey (NHANES) spanning 2011 to 2018. We employed generalized additive model (GAM) to discern potential non-linear relationships and utilized the two-piecewise linear regression model to probe the association between blood Mn levels and sarcopenia.
Results: After adjusting for potential confounders, we identified non-linear association between blood Mn levels and sarcopenia, with an inflection point at 13.45 μg/L. The effect sizes and the confidence intervals on the left and right sides of the inflection point were 1.006 (0.996 to 1.048) and 1.082 (1.043 to 1.122), respectively. Subgroup analysis showed that the effect sizes of blood Mn on sarcopenia have significant differences in gender and different BMI groups.
Conclusion: Our results showed that a reverse U-shaped curve between blood Mn levels and sarcopenia, with an identified the inflection point at blood Mn level of 13.45 μg/L.
Introduction
Sarcopenia, characterized by an age-related decrease in skeletal muscle mass and function, is a recognized risk factor for significant adverse health outcomes such as frailty, disability, institutionalization, and mortality (1–3). Starting at age 30, there is a yearly loss of 0.5–1% in skeletal muscle mass, and this decline becomes markedly faster after the age of 65 (4). Sarcopenia affects over 40% of individuals aged 70 and above, equating to nearly 50 million people globally. Given the recent rise in life expectancy, this figure is projected to multiply tenfold by 2050. It is worth noting that while sarcopenia is predominantly diagnosed in the older person, it can manifest in various clinical scenarios (5).
Manganese (Mn) is an essential trace element that is involved in numerous physiological activities, including protein synthesis, signal transduction, and metabolism (6). The typical serum levels range from 4 to 15 μg/L in adults (7). It is a crucial component of enzymes that defend cells from oxidative stress, especially vital for the protection of brain and muscle cells that underpin neuromotor function (8, 9). However, consuming amounts that exceed the body’s homeostatic capacity can lead to toxic effects (8, 10). The main source of exposure to the general population is through the diet, with this element being present in many foods, including nuts, legumes, seeds, tea, whole grains, and leafy green vegetables (11). Dietary intake alone can surpass the body’s homeostatic capacity for Mn, it is generally uncommon for diet alone to result in Mn toxicity. However, certain conditions and lifestyles can disrupt this balance, potentially leading to excess accumulation. Exposure to Mn-containing dust or fumes in occupations such as mining, steel manufacturing, or welding can contribute to this imbalance (11). Additionally, the overuse of certain psychotropic drugs, such as ephedra, can also lead to excess Mn accumulation (10). Notably, Mn toxicity has been associated with neurological disorders (12), but its impact on musculoskeletal health, particularly sarcopenia, is not well understood.
However, no study has yet investigated potential muscle deficits in relation to blood Mn in the general adult population. As such, our study draws on data from participants aged 18–59 across the National Health and Nutrition Examination Survey (NHANES) 2011–2018 in order to evaluate the relationship between the concentration of blood Mn and sarcopenia.
Methods
Study design
Continuous variables were expressed as mean ± standard deviation or median, and categorical variables were expressed in frequency or as a percentage. The One-Way ANOVA, Kruskal-Wallis H test, and chi-square tests were utilized to determine statistical differences in the means and proportions across groups. A univariate linear regression model assessed the associations between blood Mn levels and sarcopenia. Both non-adjusted and multivariate-adjusted models were reported. Following the STROBE statement guidelines, we concurrently presented results from unadjusted, minimally adjusted, and fully adjusted analyses Covariance adjustments were made based on a principle that inclusion in the model altered the matched odds ratio by at least 10%. Furthermore, we employed a generalized additive model (GAM) to detect non-linear relationships. Upon observing a non-linear correlation, a two-piecewise linear regression model was implemented to estimate the threshold effect of blood Mn concentration on sarcopenia, illustrated by the smoothing plot. Subgroup analyses were conducted using stratified linear regression models, with subgroup modifications and interactions being examined via the likelihood ratio test.
Study population
The National Health and Nutrition Examination Survey (NHANES) is a population-based cross-sectional survey administered by the National Center for Health Statistics (NCHS) with the objective of evaluating the health and nutrition status of both adults and children in the United States. Employing a sophisticated multistage sampling design, the NHANES collects data from a representative subset of the population. Since its inception in the 1960s, the NHANES has been conducted on a regular basis, furnishing invaluable insights into the health and nutrition of the American population. Data from the official NHANES website, spanning the period from 2011 to March 2018, were accessed. Additional details can be found therein.
Definition of sarcopenia and assessment methodology
Sarcopenia, defined as an age-related decline in skeletal muscle mass and function, poses significant risks for adverse health outcomes like frailty and disability. In our study, sarcopenia was assessed using Dual-Energy X-ray Absorptiometry (DXA) whole body evaluations, performed with Hologic Discovery Model A densitometers. These evaluations primarily focused on measuring the appendicular skeletal muscle mass to accurately quantify muscle content. The DXA scan exclusions included pregnancy, a weight surpassing 300 pounds (136 kg) due to the scanner’s weight capacity, height exceeding 6′5″ given the DXA table’s limitations, recent barium-based radiographic contrast exposure within the past 7 days, or engagement in nuclear medicine studies in the prior 3 days. All scans were orchestrated using the Hologic software (V.8.26: a3), with DXA being instrumental in gauging the appendicular skeletal muscle mass (13). The sarcopenia index, used to diagnose sarcopenia, was calculated by dividing the total appendicular skeletal muscle mass (in kilograms) by the body mass index (BMI, kg/m^2). For diagnosing sarcopenia in this study, we employed sex-specific cut-off values for the sarcopenia index, setting them at 0.789 for men and 0.512 for women, as recommended by the National Institutes of Health (FNIH) guidelines (14).
Assessment of blood Mn
Following a basic dilution sample preparation, the Mn concentration in whole blood specimens was determined using mass spectrometry. The Mn exhibited a lower limit of detection (LOD) at an estimated 0.99 μg/L. Values measured beneath the detection limit were denoted as the LOD over the square root of 2. For an in-depth overview, refer to the NHANES website (15).
Covariates
Demographic and lifestyle factors are assessed using questionnaires. The baseline data include age (younger than 40, 40 and older), gender (male, female), educational levels (less than high school education, some high school, high school graduate/GED, some college or associate’s degree, college graduate or higher), alcohol intake (non-drinker, 1 to less than 5 drinks per month, 5 to less than 10 drinks per month, or 10 or more drinks per month), smoking status (current, former, or never smoker), physical exercises (none, moderate, vigorous), and poverty level index (PLI). Diabetes is defined as having a fasting glucose level of 126 mg/dL or higher or reporting a previous diagnosis. Hypertension is defined as having persistent resting blood pressure (BP) at or above 140/90 mmHg or reporting a previous diagnosis. Cardiovascular disease encompass self-reported medical diagnoses, including congestive heart failure, angina, myocardial infarction, and stroke.
Statistical analysis
Statistical evaluations were executed using the R statistical software1 and Empower Stats (X&Y Solutions, Inc., Boston, MA).2 Variables adhering to a normal distribution were articulated as mean ± standard deviation (SD). A two-sided p-value of <0.01 was deemed indicative of statistical significance.
Results
Baseline characteristics of the study participants
Of the 8,135 participants in the 2011–2018 NHANES study, 667 were diagnosed with sarcopenia. Among the participants diagnosed with sarcopenia, which constituted 8.2% of our study population, the average age was 43.05 ± 12.23 years. Approximately 62.82% of the participants were aged 40 years or above. In contrast, individuals younger than 40 years comprised 37.18% of the study population. Specifically, younger participants (under 40) exhibited an average Mn level of 10.37 μg/L, while their older counterparts exhibited an average level of 10.15 μg/L. Table 1 compares the baseline demographic, clinical, and biochemical characteristics of individuals by tertiles of the Mn concentration groups. No significant differences were observed in age, diabetes prevalence, cardiovascular disease incidence, and the poverty index across the various blood Mn concentration groups. The mean age of the cohort was 37.354 ± 12.369 years, and 49.81% of the participants were male. Compared to subjects in the lowest tertile of the blood Mn concentration group, those in the highest tertile exhibited the following characteristics: younger age, higher proportion of females, increased waist circumference, higher proportion of 10+ drinks per month, higher proportion of current smokers, lower proportion individuals diagnosed with hypertension proportion of individuals with a college higher, discernible decline in engagement in vigorous physical activities, and lower proportion of individuals with BMI <25.
Univariate analysis
The results of the univariate analysis are presented in Table 2. The results showed that age, BMI, waist circumference, hypertension, diabetes, smoking status, alcohol consumption, family PIR, Mn, and cardiovascular disease were positively correlated with sarcopenia. We also found that gender and physical activity were not associated with sarcopenia.
The results of the relationship between blood Mn and sarcopenia
We applied a univariate linear regression model to assess the relationship between blood Mn levels and sarcopenia. Detailed results of the unadjusted and adjusted analyses are presented in Table 3. In the crude model, blood Mn exhibited positive correlation with sarcopenia (β = 1.039, 95% CI: 1.020 to 1.058, p < 0.001). In the model minimally adjusted for age and gender, the findings remained largely unaltered (β = 1.042, 95%CI: 1.024 to 1.061, p < 0.001). Likewise, in the model fully adjusted for additional covariates, the outcomes persisted in their consistency (β = 1.039, 95%CI: 1.019 to 1.060, p < 0.001). For the purpose of sensitivity analysis, we also handled blood Mn Categorical variable (Quartile), and found that the same trend was observed as well (p for trend was <0.001).
The results of relationship between non-linear relationship
Since blood Mn levels are a continuous variable, analyzing for non-linear relationships is essential (16). In our study, depicted in Figure 1, we identified a non-linear correlation between blood Mn levels and sarcopenia after controlling for factors including age, gender, waist circumference, alcohol use, smoking status, diabetes, hypertension, cardiovascular conditions, educational attainment, BMI, and poverty index. Through the application of a two-piecewise linear regression model, we determined an inflection point to be 13.45 μg/L. To the right of the inflection point, the effect size was 1.006 with a 95% confidence interval (CI) of 0.996 to 1.048 and p-value of 0.769. Notably, a positive association between blood Mn levels and sarcopenia was evident on the left side of the inflection point, with an effect size of 1.082, a 95% CI of 1.043 to 1.122, and p-value of <0.001 (Table 4).
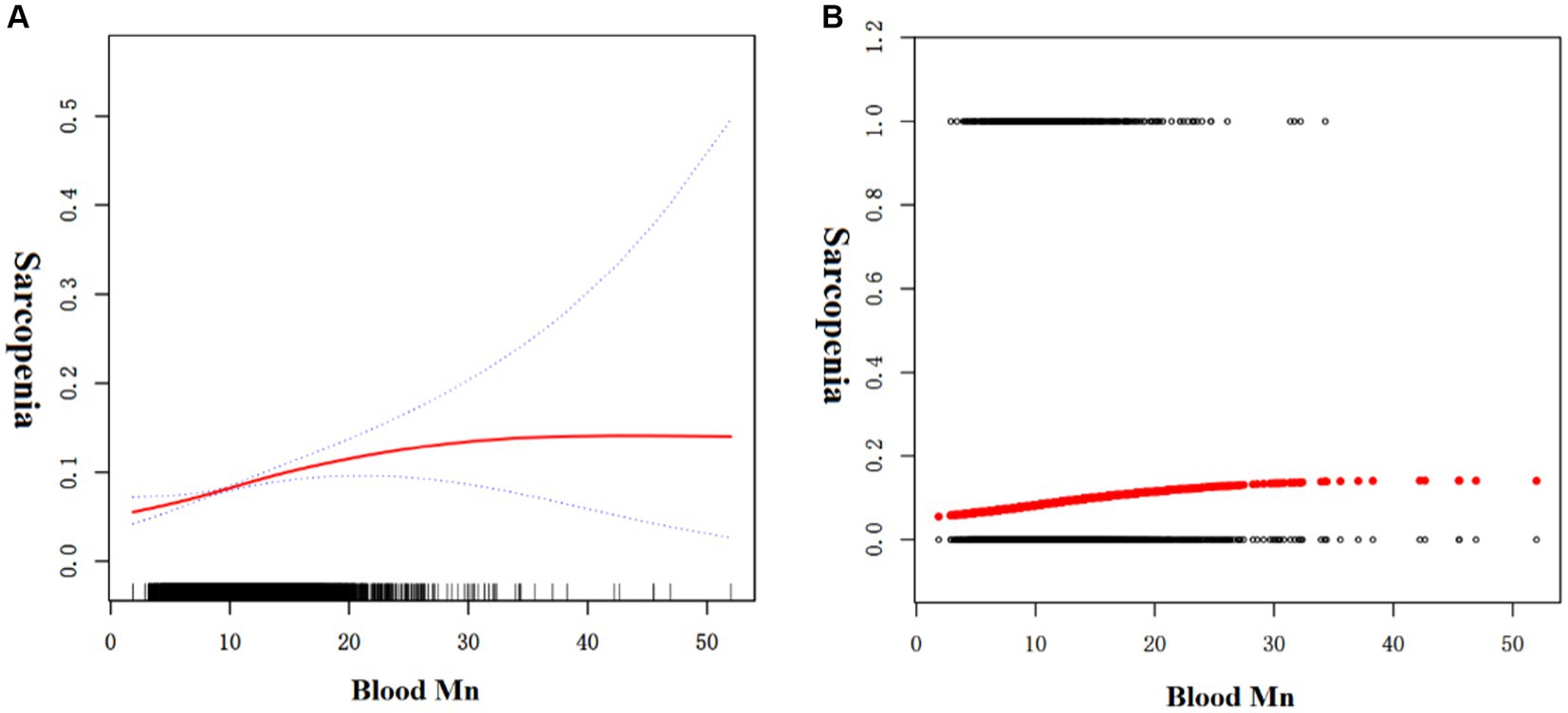
Figure 1. The association between blood Mn and sarcopenia. (A) Solid red line represents the smooth curve fit between variables. Blue bands represent the 95% of confidence interval from the fit. (B) Each black point represents a sample.
The results of subgroup analyses
As shown in Table 5, significant interactions were observed for gender and BMI (P for interaction <0.05). Conversely, for age, alcohol consumption, smoking status, physical activity levels, hypertension, and diabetes, the interactions were not statistically significant, with p-values above 0.05. Notably, the impact of blood Mn levels on sarcopenia differed significantly between genders and across various BMI groups. Blood Mn was positively associated with sarcopenia in male subjects [β = 1.075, 95%CI (1.041, 1.111)] and female subjects [β = 1.027, 95%CI (1.001, 1.1053)]. Blood Mn was positively associated with sarcopenia in different BMI groups [β = 1.093, 95%CI (1.049, 1.139); β = 1.067, 95%CI (1.027, 1.109); β = 1.021, 95%CI (0.995, 1.048), p = 0.021].
Discussion
In this study, we conducted an investigation into the potential relationship between blood Mn levels and the occurrence of sarcopenia in adults aged between 18 and 59 years, drawing on data from the National Health and Nutrition Examination Survey (NHANES) from 2011 to 2018. To the best of our knowledge, this represents the first population-based study that highlights a reverse U-shaped curve between blood Mn levels and sarcopenia, with an identified the inflection point at a blood Mn level of 13.45 μg/L. Our observations revealed disparate correlations between blood Mn levels and the presence of sarcopenia on the two sides of the inflection point (defined as blood Mn level = 13.45 μg/L). Our findings indicate a positive association between blood Mn levels and sarcopenia to the left of the inflection point, whereas to the right, the relationship did not reach statistical significance.
It is important to emphasize that the age range of participants in our study differs from that utilized in the pioneering work of Studenski et al. (14). Among the participants diagnosed with sarcopenia, comprising 8.2% of our study population, the average age is 43.05 ± 12.23 years. A significant 62.82% of the participants are aged 40 years or above. In contrast, individuals younger than 40 years comprise 37.18% of the study population. Landi et al. (17) suggest that the precursors of sarcopenia, including muscle loss and declining muscle function, can begin earlier in life. By extending our analysis to include adults under 40, we aim to explore these early indicators and potentially extend the understanding of sarcopenia’s onset and progression. The division at 40 years serves as a pragmatic threshold to investigate differential impacts of Mn exposure on muscle mass across varying life stages. Hughes et al. (18) suggest that significant changes in body composition, particularly in muscle mass and strength, may begin to manifest around this age, marking the onset of gradual sarcopenia development.
The association between blood Mn levels and sarcopenia is inherently complex due to manganese’s dual role as an essential nutrient and a potential toxic substance, with the degree of exposure playing a pivotal role in this complexity. Likewise, our research consistently points to the association between increased levels of Mn exposure and an elevated risk of sarcopenia, a pattern that was observed across all stratified subgroups, with the exception of gender and BMI. Sarcopenia, impacting almost one-third of the older person, represents a substantial factor in the development of negative health outcomes among geriatric patients (19, 20). The pathogenesis of sarcopenia encompasses a multitude of intricate cellular and molecular mechanisms, including neuromuscular junction dysfunction (21), a reduction in satellite cell number and function (22), a decline in the count of motor units (23), infiltration of intramuscular adipose tissue (24), proinflammatory processes (25), insulin resistance (25, 26)]. Moreover, mitochondrial dysfunction (27) and oxidative stress (28) are emerging as central elements in the pathogenesis of sarcopenia (27).
The underlying mechanisms of Mn toxicity are not fully determined, but mitochondrial dysfunction is a suspected contributor, as Mn accumulates in mitochondria and impairs their function, according to both in vivo and in vitro research (29, 30). Moreover, oxidative stress is linked to Mn toxicity, exacerbated when Mn undergoes a valence transition, amplifying its prooxidant capacity (31). Elevated levels of Mn within the mitochondria disrupt oxidative respiration, resulting in an overproduction of reactive oxygen species (ROS) and subsequent mitochondrial dysfunction (32). Given that Mn3+ possesses a more pronounced pro-oxidant potential compared to Mn2+, its formation within the mitochondria could exacerbate oxidative harm (33). This could impede the mitochondrial electron transfer chain, resulting in reduced ATP synthesis, increased electron escape, and elevated O2 production (34). Research by Remmen et al. (19) has shed light on the potential mechanisms underlying skeletal muscle weakness linked to oxidative stress during aging, specifically sarcopenia, through studies on mice deficient in CuZnSOD (Sod1KO), a cytoplasmic superoxide scavenger. These Sod1KO mice exhibit several phenotypes resembling characteristics of sarcopenia observed in aged wild-type (WT) mice, such as loss of innervation, mitochondrial dysfunction, and heightened production of mitochondrial ROS (mtROS) (10, 35, 36).
Mn accumulation could influence metabolic processes, particularly in relation to type 2 diabetes mellitus (T2M), which may subsequently contribute to the development and progression of sarcopenia. As a transition metal, manganese itself is an oxidant at high concentrations, and it appears to be involved in oxidative damage and mitochondrial dysfunction, which have been implicated in the development of T2D (37). Furthermore, T2DM has been identified as a significant risk factor for sarcopenia, likely due to increased inflammation, oxidative stress, and mitochondrial dysfunction associated with the disease (38, 39). Consequently, it is conceivable that Mn exposure could indirectly influence the development and progression of sarcopenia through its potential impact on metabolic status and the presence of T2DM.
Subgroup analysis plays a pivotal role in scientific research. In our study, we stratified data based on age, gender, BMI, diabetes, hypertension, smoking status, alcohol consumption, and physical activity. Notably, gender and BMI emerged as significant factors. Prior longitudinal studies (40, 41) have indicated that the decrease in height-adjusted muscle mass is less marked in women compared to men, potentially clarifying the gender-differentiated association between blood Mn levels and sarcopenia. However, the relationship between blood Mn levels and sarcopenia across various BMI groups remains unexplained, as it has not been reported in previous studies.
A significant strength of this study is its large and representative sample of the general, non-institutionalized population. We applied rigorous methods tailored for complex survey data, ensuring that our conclusions are applicable to the broader U.S. population. Our analysis was thorough, with adjustments for numerous confounders to solidify the reliability of our results. However, several limitations should be considered in our study. Firstly, the study’s cross-sectional design means exposures and outcomes were measured simultaneously, making it challenging to determine their temporal sequence. Thus, we cannot infer a cause-and-effect relationship, and the possibility of reverse causality remains. Furthermore, we used blood as the biomarker for manganese exposure, though alternative biological matrices, like hair, have been suggested as potentially more precise indicators. While we controlled for a wide array of potential confounders, there may be unaccounted factors such as occupational demands, family health history, and medication usage that could influence the findings, particularly when extrapolating to different populations. Additionally, it is pertinent to note that our study’s age range was also influenced by the inclusion criteria for Dual-energy X-ray Absorptiometry (DXA) measurements available in the NHANES database. The NHANES database restricts DXA measurements to individuals between the ages of 18 and 59 years. This exclusion could prevent the findings from being fully applicable to individuals beyond the age of 59, who might experience different health outcomes or levels of exposure to the studied factors. Finally, our focus was on the influence of individual metals on sarcopenia, without exploring the interactive effects that may result from exposure to multiple metals.
Conclusion
Our study revealed a reverse U-shaped curve between blood Mn levels and sarcopenia, with an identified the inflection point at a blood Mn level of 13.45 μg/L. Additional studies are warranted to confirm our findings in prospective cohorts and to elucidate the potential mechanisms underlying the relationship between Mn and sarcopenia.
Code availability
All analyses were performed with R software, V.4.1.3 [R: a language and statistical computing environment (program), Vienna, Austria: R Foundation for Statistical Computing, 2016], and EmpowerStats (http://www.empowerstats.com).
Data availability statement
The original contributions presented in the study are included in the article/supplementary material, further inquiries can be directed to the corresponding author.
Ethics statement
The studies involving humans were approved by the Institutional Review Board (or Ethics Committee) of the institutional review board of the National Center for Health Statistics, CDC (protocol #2005-06, #2011-17, #2018-01). The studies were conducted in accordance with the local legislation and institutional requirements. The participants provided their written informed consent to participate in this study.
Author contributions
BX: Formal analysis, Funding acquisition, Supervision, Validation, Visualization, Writing – review & editing. Z-xC: Data curation, Investigation, Methodology, Software, Writing – original draft. W-jZ: Data curation, Methodology, Software, Supervision, Writing – original draft, Writing – review & editing. JS: Conceptualization, Data curation, Formal analysis, Investigation, Project administration, Resources, Software, Supervision, Visualization, Writing – original draft, Writing – review & editing. QZ: Conceptualization, Data curation, Formal analysis, Funding acquisition, Investigation, Methodology, Project administration, Resources, Software, Supervision, Validation, Visualization, Writing – original draft, Writing – review & editing.
Funding
The author(s) declare financial support was received for the research, authorship, and/or publication of this article. This research was supported by the Zhejiang Provincial Health Science and Technology Plan Project in China (2022KY1209), Wenzhou Basic scientific research projects in China (Y20210049), Zhejiang Province Traditional Chinese Medicine Science and Technology Plan Project in China (2024ZL998).
Conflict of interest
The authors declare that the research was conducted in the absence of any commercial or financial relationships that could be construed as a potential conflict of interest.
Publisher’s note
All claims expressed in this article are solely those of the authors and do not necessarily represent those of their affiliated organizations, or those of the publisher, the editors and the reviewers. Any product that may be evaluated in this article, or claim that may be made by its manufacturer, is not guaranteed or endorsed by the publisher.
Footnotes
References
1. Morley, JE, Baumgartner, RN, Roubenoff, R, Mayer, J, and Nair, KS. Sarcopenia. J Lab Clin Med. (2001) 137:231–43. doi: 10.1067/mlc.2001.113504
2. Cruz-Jentoft, A. J., Baeyens, J. P., Bauer, J. M., Boirie, Y., Cederholm, T., Landi, F., et al. (2010). Sarcopenia: European consensus on definition and diagnosis report of the European working group on sarcopenia in older people. Age and Ageing.
3. Kyle, U, Genton, L, Hans, D, Karsegard, L, Slosman, D, and Pichard, C. Age-related differences in fat-free mass, skeletal muscle, body cell mass and fat mass between 18 and 94 years. Hum Nutr Appl Nutr. (2001) 55:663–72. doi: 10.1038/sj.ejcn.1601198
4. McLean, RR, Shardell, MD, Alley, DE, Cawthon, PM, Fragala, MS, Harris, TB, et al. Criteria for clinically relevant weakness and low lean mass and their longitudinal association with incident mobility impairment and mortality: the Foundation for the National Institutes of Health (FNIH) sarcopenia project. J Gerontol A Biol Sci Med Sci. (2014) 69:576–83. doi: 10.1093/gerona/glu012
5. Hida, T, Harada, A, Imagama, S, and Ishiguro, N. Managing sarcopenia and its related-fractures to improve quality of life in geriatric populations. Aging Dis. (2014) 5:226–37. doi: 10.14336/AD.2014.0500226
6. Li, LY, and Yang, X. The essential element manganese, oxidative stress, and metabolic diseases: links and interactions. Oxidative Med Cell Longev. (2018) 2018:1–11. doi: 10.1155/2018/7580707
7. Williams, M., Todd, G. D., Roney, N., Crawford, J., Coles, C., McClure, P. R., et al. Toxicological profile for manganese. (2013).
8. Aschner, JL, and Aschner, M. Nutritional aspects of manganese homeostasis. Mol Asp Med. (2005) 26:353–62. doi: 10.1016/j.mam.2005.07.003
9. Fridovich, I. Superoxide radical and superoxide dismutases. Annu Rev Biochem. (1995) 64:97–112. doi: 10.1146/annurev.bi.64.070195.000525
10. Guilarte, TR. Manganese and Parkinson’s disease: a critical review and new findings. Environ Health Perspect. (2010) 118:1071–80. doi: 10.1289/ehp.0901748
11. Erikson, KM, and Aschner, M. Manganese: its role in disease and health. Met Ions Life Sci. (2019) 19:253–66. doi: 10.1515/9783110527872-010
12. Wexler, NS, Lorimer, J, Porter, J, Gomez, F, Moskowitz, C, Shackell, E, et al. Venezuelan kindreds reveal that genetic and environmental factors modulate Huntington’s disease age of onset. Proc Natl Acad Sci USA. (2004) 101:3498–503. doi: 10.1073/pnas.0308679101
13. Heymsfield, SB, Smith, R, Aulet, M, Bensen, B, Lichtman, S, Wang, J, et al. Appendicular skeletal muscle mass: measurement by dual-photon absorptiometry. Am J Clin Nutr. (1990) 52:214–8. doi: 10.1093/ajcn/52.2.214
14. Studenski, SA, Peters, KW, Alley, DE, Cawthon, PM, Mclean, RR, Harris, TB, et al. The FNIH sarcopenia project: rationale, study description, conference recommendations, and final estimates. J Gerontol A Biol Sci Med Sci. (2014) 69:547–58. doi: 10.1093/gerona/glu010
15. Laboratory Procedure Manual. In: Organic Analytical Toxicology Branch. Atlanta, GA, USA: Division of Laboratory Sciences, National Center for Environmental Health. (2013).
16. Chen, C, and Dai, J-L. Triglyceride to high-density lipoprotein cholesterol (HDL-C) ratio and arterial stiffness in Japanese population: a secondary analysis based on a cross-sectional study. Lipids Health Dis. (2018) 17:1–9. doi: 10.1186/s12944-018-0776-7
17. Landi, F, Calvani, R, Cesari, M, Tosato, M, Martone, AM, Bernabei, R, et al. Sarcopenia as the biological substrate of physical frailty. Clin Geriatr Med. (2015) 31:367–74. doi: 10.1016/j.cger.2015.04.005
18. Hughes, VA, Frontera, WR, Roubenoff, R, Evans, WJ, and Singh, MAF. Longitudinal changes in body composition in older men and women: role of body weight change and physical activity. Am J Clin Nutr. (2002) 76:473–81. doi: 10.1093/ajcn/76.2.473
19. Ahn, B, Ranjit, R, Premkumar, P, Pharaoh, G, Piekarz, KM, Matsuzaki, S, et al. Mitochondrial oxidative stress impairs contractile function but paradoxically increases muscle mass via fibre branching. J Cachexia Sarcopenia Muscle. (2019) 10:411–28. doi: 10.1002/jcsm.12375
20. Zaaria, M, Pasleau, F, Reginster, J-Y, and Bruyère, O. Health outcomes of sarcopenia: a systematic review and meta-analysis. J Frailty. (2017) 6:25.
21. Padilla, CJ, Harrigan, ME, Harris, H, Schwab, JM, and Arnold, WD. Profiling age-related muscle weakness and wasting: neuromuscular junction transmission as a driver of age-related physical decline. Gero Science. (2021) 43:1–17. doi: 10.1007/s11357-021-00369-3
22. Yeves, AM, Burgos, JI, Medina, AJ, Villa-Abrille, MC, and Ennis, IL. The countermeasure for cancer cachexia related muscle wasting does not need to be muscle hyperplasia. Acta Physiol. (2018) 224. doi: 10.1111/apha.13092
23. Liu, W, Klose, A, Forman, S, Paris, ND, and Chakkalakal, JV. Loss of adult skeletal muscle stem cells drives age-related neuromuscular junction degeneration. Elife. (2017) 6:e26464. doi: 10.7554/eLife.26464
24. Yoon, CH, Sungeun, K, Woo, PJ, Seok, LN, Young, HS, Young, HJ, et al. Implication of circulating Irisin levels with Brown adipose tissue and sarcopenia in humans. J Clin Endocrinol Metabolism. (2014) 99:2278–85. doi: 10.1210/jc.2014-1195
25. Lambernd, S, Taube, A, Schober, A, Platzbecker, B, Görgens, SW, Schlich, R, et al. Contractile activity of human skeletal muscle cells prevents insulin resistance by inhibiting pro-inflammatory signalling pathways. Diabetologia. (2012) 55:1128–39. doi: 10.1007/s00125-012-2454-z
26. Lopez-Legarrea, P, de la Iglesia, R, Crujeiras, AB, Pardo, M, Casanueva, FF, Zulet, MA, et al. Higher baseline irisin concentrations are associated with greater reductions in glycemia and insulinemia after weight loss in obese subjects. Nutr Diabetes. (2014) 4:e110. doi: 10.1038/nutd.2014.7
27. Marzetti, E, Calvani, R, Cesari, M, Buford, TW, Lorenzi, M, Behnke, BJ, et al. Mitochondrial dysfunction and sarcopenia of aging: from signaling pathways to clinical trials. Int J Biochem Cell Biol. (2013) 45:2288–301. doi: 10.1016/j.biocel.2013.06.024
28. Gonzalez-Freire, M, Cabo, RD, Studenski, SA, and Ferrucci, L. The neuromuscular junction: aging at the crossroad between nerves and muscle. Front Aging Neurosci. (2014) 6:208. doi: 10.3389/fnagi.2014.00208
29. Aschner, M, Erikson, KM, Hernández, EH, and Tjalkens, R. Manganese and its role in Parkinson’s disease: from transport to neuropathology. Neuro Molecular Med. (2009) 11:252–66. doi: 10.1007/s12017-009-8083-0
30. Gavin, C, Gunter, K, and Gunter, T. Manganese and calcium transport in mitochondria: implications for manganese toxicity. Neurotoxicology. (1999) 20:445–53.
32. Gunter, TE, Gavin, CE, Aschner, M, and Gunter, KK. Speciation of manganese in cells and mitochondria: a search for the proximal cause of manganese neurotoxicity. Neurotoxicology. (2006) 27:765–76. doi: 10.1016/j.neuro.2006.05.002
33. Segura-Aguilar, J, and Lind, C. On the mechanism of the Mn3+−induced neurotoxicity of dopamine: prevention of quinone-derived oxygen toxicity by DT diaphorase and superoxide dismutase. Chem Biol Interact. (1989) 72:309–24. doi: 10.1016/0009-2797(89)90006-9
34. Hamai, D, Campbell, A, and Bondy, SC. Modulation of oxidative events by multivalent manganese complexes in brain tissue. Free Radic Biol Med. (2001) 31:763–8. doi: 10.1016/S0891-5849(01)00639-6
35. Sakellariou, GK, Davis, CS, Shi, Y, Ivannikov, MV, Zhang, Y, Vasilaki, A, et al. Neuron-specific expression of CuZnSOD prevents the loss of muscle mass and function that occurs in homozygous CuZnSOD-knockout mice. FASEB J. (2014) 28:1666. doi: 10.1096/fj.13-240390
36. Zhang, Y, Davis, C, Sakellariou, GK, Shi, Y, Kayani, AC, Pulliam, D, et al. CuZnSOD gene deletion targeted to skeletal muscle leads to loss of contractile force but does not cause muscle atrophy in adult mice. FASEB J. (2013) 27:3536. doi: 10.1096/fj.13-228130
37. Maechler, P, and Wollheim, CB. Mitochondrial function in normal and diabetic β-cells. Nature. (2001) 414:807–12. doi: 10.1038/414807a
38. Mesinovic, J, Zengin, A, De Courten, B, Ebeling, PR, and Scott, D. Sarcopenia and type 2 diabetes mellitus: a bidirectional relationship. Diabetes Metab Syndrome Obes. (2019):1057–72. doi: 10.2147/DMSO.S186600
39. Sinclair, AJ, Abdelhafiz, AH, and Rodríguez-Mañas, L. Frailty and sarcopenia-newly emerging and high impact complications of diabetes. J Diabetes Complicat. (2017) 31:1465–73. doi: 10.1016/j.jdiacomp.2017.05.003
40. Ning, HT, Du, Y, Zhao, LJ, Tian, Q, Feng, H, and Deng, HW. Racial and gender differences in the relationship between sarcopenia and bone mineral density among older adults. Osteoporos Int. (2021) 32:841–51. doi: 10.1007/s00198-020-05744-y
Keywords: blood manganese, sarcopenia, adolescents, cross-sectional studies, National Health and Nutrition Examination Survey
Citation: Xu B, Chen Z-x, Zhou W-j, Su J and Zhou Q (2024) Associations between blood manganese levels and sarcopenia in adults: insights from the National Health and Nutrition Examination Survey. Front. Public Health. 12:1351479. doi: 10.3389/fpubh.2024.1351479
Edited by:
Giuseppe Caminiti, Università Telematica San Raffaele, ItalyReviewed by:
Eduardo Abreu, University of Missouri–Kansas City, United StatesMara Carsote, Carol Davila University of Medicine and Pharmacy, Romania
Copyright © 2024 Xu, Chen, Zhou, Su and Zhou. This is an open-access article distributed under the terms of the Creative Commons Attribution License (CC BY). The use, distribution or reproduction in other forums is permitted, provided the original author(s) and the copyright owner(s) are credited and that the original publication in this journal is cited, in accordance with accepted academic practice. No use, distribution or reproduction is permitted which does not comply with these terms.
*Correspondence: Qiang Zhou, zhou_qiang30@sina.com