- 1Department of Neurology, University of Alabama at Birmingham, Birmingham, AL, United States
- 2Department of Neurosurgery, University of Alabama at Birmingham, Birmingham, AL, United States
- 3Department of Pathology, University of Alabama at Birmingham, Birmingham, AL, United States
- 4Department of Psychology, Samford University, Birmingham, AL, United States
- 5Department of Biostatistics, University of Alabama at Birmingham, Birmingham, AL, United States
- 6UAB Center for Exercise Medicine, University of Alabama at Birmingham, Birmingham, AL, United States
- 7Florida Institute for Human and Machine Cognition, Pensacola, FL, United States
- 8Department of Neurology, Emory University, Atlanta, GA, United States
Background: In a randomized, controlled trial, we showed that high-intensity rehabilitation, combining resistance training and body-weight interval training, improves sleep efficiency in Parkinson's disease (PD). Quantitative sleep EEG (sleep qEEG) features, including sleep spindles, are altered in aging and in neurodegenerative disease.
Objective: The objective of this post-hoc analysis was to determine the effects of exercise, in comparison to a sleep hygiene, no-exercise control group, on the quantitative characteristics of sleep spindle morphology in PD.
Methods: We conducted an exploratory post-hoc analysis of 24 PD participants who were randomized to exercise (supervised 3 times/week for 16 weeks) versus 26 PD participants who were assigned to a sleep hygiene, no-exercise control group. At baseline and post-intervention, all participants completed memory testing and underwent polysomnography (PSG). PSG-derived sleep EEG central leads (C3 and C4) were manually inspected, with rejection of movement and electrical artifacts. Sleep spindle events were detected based on the following parameters: (1) frequency filter = 11–16 Hz, (2) event duration = 0.5–3 s, and (3) amplitude threshold 75% percentile. We then calculated spindle morphological features, including density and amplitude. These characteristics were computed and averaged over non-rapid eye movement (NREM) sleep stages N2 and N3 for the full night and separately for the first and second halves of the recording. Intervention effects on these features were analyzed using general linear models with group x time interaction. Significant interaction effects were evaluated for correlations with changes in performance in the memory domain.
Results: A significant group x time interaction effect was observed for changes in sleep spindle density due to exercise compared to sleep hygiene control during N2 and N3 during the first half of the night, with a moderate effect size. This change in spindle density was positively correlated with changes in performance on memory testing in the exercise group.
Conclusions: This study is the first to demonstrate that high-intensity exercise rehabilitation has a potential role in improving sleep spindle density in PD and leading to better cognitive performance in the memory domain. These findings represent a promising advance in the search for non-pharmacological treatments for this common and debilitating non-motor symptom.
Introduction
Neurophysiological rhythms in the brain underlie the fundamental biological process of sleep (1). Sleep spindles are one such crucial oscillatory rhythm characteristic of non-rapid eye movement (NREM) sleep (2). This oscillation encompasses frequencies ranging from 11 to 16 Hz and originates in the thalamo-cortical loop (3). Sleep spindles are essential for declarative memory and sleep-related memory consolidation (3).
Although Parkinson's disease (PD) has historically been viewed primarily as a neurodegenerative motor disorder, we now have a clearer picture of non-motor manifestations of the disease (4–6). In fact, sleep disorders are frequently seen among individuals with PD and affect 74%–98% of patients (7, 8). Unfortunately, despite the profound impact of sleep dysfunction on PD, few pharmacological therapies have been shown to improve these symptoms, and the available treatments can have adverse side effects (9). In the absence of a biomarker, it has been difficult to find a treatment for cognitive dysfunction. However, a recent study by Latreille and colleagues found that sleep spindles are associated with the risk of cognitive decline in PD (10). Indeed, at the physiological level, spindles play an important role in the consolidation of memory (11).
The benefits of exercise on non-motor symptoms of PD are widely recognized (12, 13) as an exciting paradigm for nonpharmacological treatment. Indeed, a recent meta-analysis showed that exercise provided significant improvements in subjective sleep quality (14). Furthermore, our previous work and the only study in the literature to evaluate the effects of exercise on objective sleep outcomes showed an increase in sleep efficiency as measured by polysomnography (PSG) (15). However, to the best of our knowledge, no study has examined the effects of exercise on quantitative sleep EEG (qEEG) in PD (16).
With this objective in mind, this post hoc analysis leverages data collected in our recent clinical trial investigating the influence of exercise on objective sleep outcomes in PD (15) to address whether exercise also affects the microarchitecture of sleep spindles. This is a critical area of study due to the importance of spindles for memory consolidation, the high prevalence of both sleep and cognitive dysfunction in PD, and the limited treatment options available for these non-motor symptoms. We therefore sought to examine the effects of exercise on sleep spindle density and amplitude in a comprehensive manner. We hypothesized that exercise would increase spindle density and amplitude compared to a no-exercise control group and that changes in sleep spindles due to exercise would correlate with exercise-induced changes in memory performance.
Methods
Participants
This study represents a post hoc analysis performed on polysomnography-acquired EEG collected during a randomized controlled trial (clinicaltrials.gov: NCT02593955). The primary objective of the parent trial was to investigate the impact of high-intensity exercise rehabilitation (EX), compared to a sleep hygiene no-exercise control (SH-C), on objective sleep outcomes in Parkinson's disease (15). The CONSORT flow diagram [Figure 1, adapted from (15)] shows the enrollment, group allocation, and follow-up of participants in the parent study. The analysis for the current study excluded five additional participants (N = 3 EX and N = 2 SH-C) because the sleep study file either at baseline or post-intervention were not usable for the qEEG analysis. Therefore, 50 participants (N = 24 EX and N = 26 SH-C) were included in the current analysis. Participants in this study were recruited from the Movement Disorders Center at the University of Alabama at Birmingham (UAB). Detailed eligibility criteria are previously reported (15); briefly, inclusion required: (1) diagnosis of PD based on the Movement Disorders Society's clinical criteria (17), (2) age >45 years, (3) on stable medications for at least four weeks prior to study entry, (4) Hoehn and Yahr stages 2–3, and (5) Montreal Cognitive Assessment (MoCA) ≥18. Exclusion criteria were: (1) atypical or secondary parkinsonism, (2) untreated sleep apnea, (3) inability to walk without a cane, (4) deep brain stimulator, or (5) meeting or exceeding the U.S. Health and Human Services physical activity guidelines (≥150 min/ week of moderate-intensity aerobic activity or 75 min/week of vigorous-intensity activity and muscle strengthening activities involving all muscle groups 2 or more days/week). Subjective sleep quality was not one of the entry criteria. The Institutional Review Board of UAB approved the study. Prior to participation, all participants signed a written informed consent.
Intervention
Exercise group
Participants randomized to the EX group trained three times per week for 16 weeks at the UAB Center for Exercise Medicine under the supervision of experienced trainers certified by the American College of Sports Medicine. Sessions included resistance training (RT) and bodyweight functional mobility exercises to improve endurance, strength, power, and balance (15, 18), as previously described. Participants completed all sessions before 2 PM at the time they felt their PD medications were most effective. The intervention protocol was adapted from our previous dose-response optimization study in older adults (19, 20). In brief, the first four sessions consisted of a familiarization phase followed by a ramp-up phase, during which resistance training volume and intensity increased. Following ramp-up, RT intensity targeted 10-repetition maximum (10RM) in sessions 1 and 3 each week, while session 2 reduced resistance loads by 30% and maximized speed of movement during the concentric phase. The RT sessions included strength-building exercises (leg press, knee extension, chest press, overhead press, pull down); trunk exercises to improve postural stability (trunk extension/flexion); and body-weight exercises to improve balance and power (step-up, squat, jump squat, lunge, side lunge, push-up, assisted pull-up, assisted dip). In this manner, different muscle groups were targeted by alternating RT and bodyweight exercises. Heart rate (HR) was recorded with a Polar HR monitor throughout the sessions to determine short rest intervals between sets. Adherence was emphasized and the mean adherence to exercise was 92.2 ± 12.5% of sessions.
Sleep hygiene group
Participants randomized to the SH-C group received sleep hygiene counseling from a board-certified sleep medicine physician (AWA). During the counseling session, participants were given the opportunity to discuss their specific sleep complaints and were provided recommendations for improving sleep hygiene. Additionally, participants received written materials describing strategies for sleep relaxation and ways to improve insomnia. To enhance study engagement, SH-C participants were contacted every 4 weeks by telephone to address any questions related to sleep hygiene.
Assessments
Polysomnography
Laboratory-based, supervised polysomnography (PSG) was performed at baseline and following the 16-week intervention. PSG was recorded with a 32-channel Natus Sandman Elite™ (Middleton, WI, USA), and included EEG, electrooculogram, submental and bilateral anterior tibial and extensor digitorum communis electromyograms, chest and abdominal polyvinylidene fluoride belts, airflow monitoring with thermocouple and nasal pressure, pulse oximetry and video. The EEG signals were digitized at a sampling rate of 512 Hz. EEG included frontal (F3, F4), central (C3, C4), and occipital (O1, O2) leads, all referenced to the contralateral mastoid. A certified sleep technician and a board-certified sleep physician (AWA) staged sleep in 30-second epochs according to the American Academy of Sleep Medicine's (AASM's) Manual for the Scoring of Sleep and Associated Events (21). Additionally, REM without atonia was scored according to the AASM scoring criteria (21). PSGs were labeled with a study code to allow blinding of group allocation during PSG interpretation.
Additional assessments
Participants were assessed for disease severity using the Movement Disorders Society-Unified Parkinson's Disease Rating Scale (MDS-UPDRS) (17). In addition, cognitive performance was evaluated in the memory domain. The tests in the memory domain included: (1) Hopkins Verbal Learning Test-Revised (HVLT-R) total recall and delayed recall; and (2) 10–36 Spatial Recall Test (10–36) immediate recall and delayed recall. Using the raw scores for the HVLT-R and 10–36, a normalized (z-) score was calculated based on normative values, controlling for age, race, and years of education, as appropriate for each test. Lastly, the individual test z-scores were averaged for each participant to determine the memory domain score.
Quantitative sleep spindle morphological analysis
Preprocessing involved converting sleep EEG data into European Data Format (EDF); importing EDF into MATLAB (version R2020b); and inspecting each 30-s epoch for artifacts. The EEG evaluator (AAM) was blinded to the participant's group allocation. Because sleep spindle activity tends to be predominantly found in central leads during non-rapid eye movement sleep (NREM) (3), sleep spindle activity was analyzed in central leads and averaged over NREM stage 2 (N2) and stage 3 (N3). We visually examined the C3 channel for the entire PSG recording and detected electrical and movement artifacts. Whenever the C3 lead had continuous artifacts, C4 channels were used. Total artifact rejections pre-intervention included 3.9% of N2 and 1.0% of N3 for the EX group and 2.6% of N2 and 0.7% of N3 for the SH-C group. Post-intervention included: 2.8% of N2 and 0.8% of N3 for the EX group and 3.5% of N2 and 0.8% of N3 for the SH-C group.
A custom-made MATLAB script was used to detect sleep spindle events in the postprocessing step (3, 22). The following parameters were applied to the most artifact-free central channel: (1) frequency filter = 11–16 Hz, (2) amplitude threshold = 75th % percentile, (3) duration = 0.5–3 s. After that, the following sleep spindle characteristics were calculated and averaged over all N2 and N3 epochs of the whole night of PSG: (1) density (events/minute); and (2) amplitude (peak to peak, expressed in µV). In addition, we examined the spindle's characteristics over the first and second halves of the night because sleep spindle density has been shown to change over the course of the night in relation to elapsed sleep time (23).
Statistical analysis
Statistical analysis was conducted using JMP Statistical Discovery Pro version 16.0. In the descriptive statistics, the Shapiro-Wilk test was used to identify the normality of all variables. We compared the demographic and polysomnographic characteristics between the EX and SH-C groups using Fischer's exact test for categorical variables and the independent sample two-tailed t-test for continuous variables. The main statistical methods for analyzing the intervention effects were general linear models with group x time interaction. Effect size was evaluated with Cohen's d. The significance level was set at p < 0.05. Due to the exploratory nature of our study, we did not correct for multiple comparisons and opted to tolerate possible Type I error rather than reject potential associations due to correction-induced Type II errors.
Results
Demographics, clinical and polysomnographic characteristics
In Table 1, the demographics and disease characteristics are summarized. There were no significant differences between the groups regarding age, sex, race, use of medications that impact sleep, disease duration, levodopa equivalent dose, or MDS UPDRS Parts I, II, III, or IV. In addition, there were no significant differences in baseline sleep architecture for the full night, first half, or second half of the night between the EX and the SH-C groups including sleep efficiency, total sleep time, sleep latency, wake after sleep onset, time and percent of each sleep stage, number of participants with REM sleep behavior disorder, apnea hypopnea index, or periodic limb movement index (all p > 0.05) (data not shown).
NREM quantitative sleep spindle analysis
There were no significant differences between changes in sleep spindle density or amplitude from baseline to post-intervention between the groups when evaluated for the entire night (Figures 2A, 3A). However, when analyzed separately for the first and second halves of the night, there was a significant decrease in spindle density in the SH-C group (F = 6.40, p = 0.0181) in the first half of the night and exercise attenuated this decrease in spindle density experienced by the SH-C group (i.e., a significant group x time interaction; F = 4.19, p = 0.046; d = 0.58) (Figure 2B). No significant differences were observed in spindle density or amplitude for the second half of the night (Figures 2C, 3C).
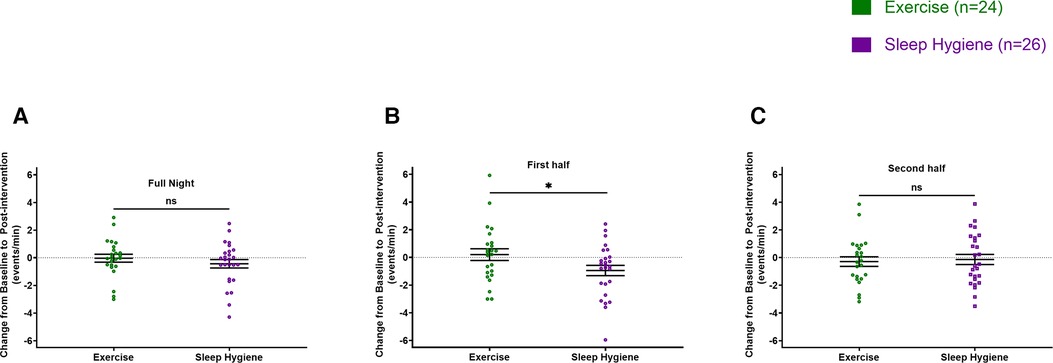
Figure 2. Exercise prevented the decrease in spindle density experienced during the first half of sleep by the sleep hygiene group (B). No significant changes in spindle density for the full night (A) and the second half of the sleep (C). In all panels, the figures are scatter plots, and error bars represent the mean and standard error of mean. ns: not significant.
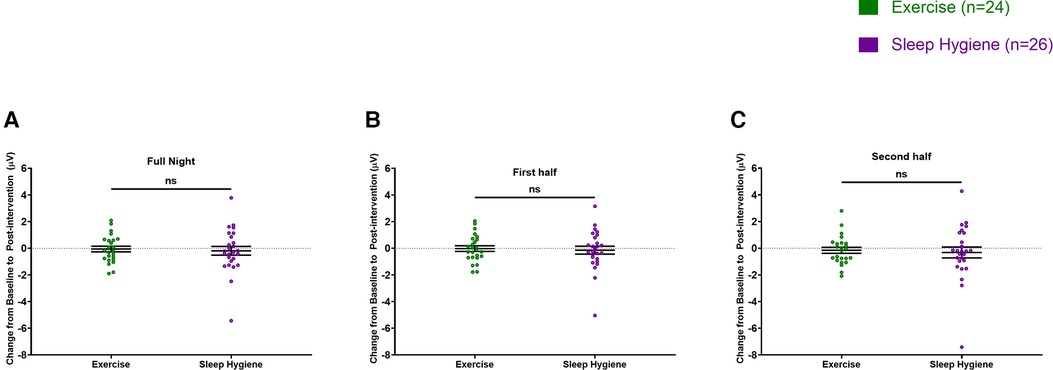
Figure 3. Spindle amplitude was not different between the two groups throughout the night (A), the first half of the sleep (B), or the second half of the sleep (C). In all panels, the figures are scatter plots, and error bars represent the mean and standard error of mean. ns: not significant.
Memory performance and spindle analysis
Next, we evaluated whether the beneficial effects of exercise in preventing decrease in spindle density were associated with better memory performance. The change in sleep spindle density in the EX-group was correlated with the change in the memory domain due to exercise (r = 0.42, p = 0.0413). A detailed analysis revealed that this effect was primarily driven by changes during the first half of the night (Figure 4). There was no significant correlation between the change in sleep density score in the SH-C group and the change in the memory domain. There was no significant correlation between the change scores in spindle amplitude and the change scores in the memory domain for either group (data not shown).
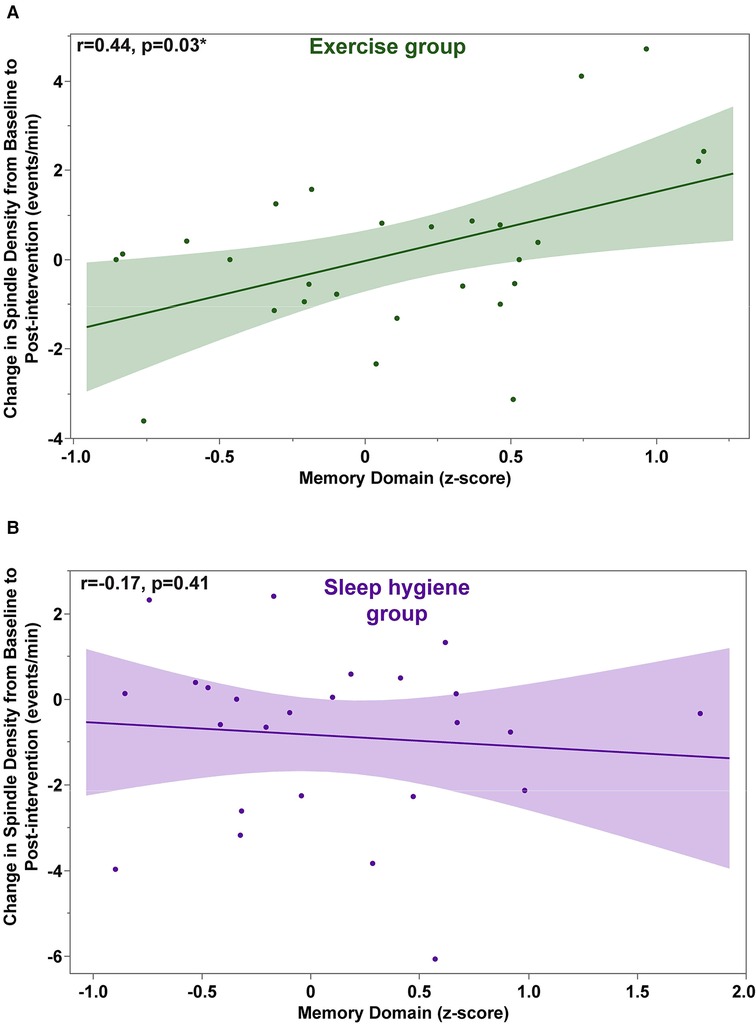
Figure 4. Exercise-induced changes in spindle density during the first half of the night are significantly correlated with changes in the memory domain scores (A). No significant correlations were found in the sleep hygiene group (B).
Discussion
In this post hoc analysis of polysomnography-derived EEG from a randomized controlled clinical trial, exercise rehabilitation counteracted the decline in spindle density during NREM in the first half of the night when compared with a no-exercise control group with a moderate effect size (d = 0.58). In addition, exercise-induced changes in spindle density were positively correlated with better cognitive performance in the memory domain. Although this investigation is exploratory, the findings inform directions for future larger studies with investigation of exercise-induced changes in sleep spindles as the primary outcome. Because available pharmacological treatments for sleep and cognitive dysfunction are ineffective or have intolerable side effects (24), the current study represents a potential step towards identifying mechanisms of nonpharmacological interventions on sleep and cognition in PD.
Exercise has been shown to significantly improve subjective sleep quality in people with PD (14). The only study in the literature that evaluated the effects of exercise on objective sleep outcomes demonstrated an increase in sleep efficiency as measured by PSG (15). However, there was no change in the amount of N2 sleep. We posited that one possible reason for the lack of exercise-induced changes in stage N2 might be the semi-quantitative nature of the sleep-stage scoring system. In fact, for more than 50 years, since 1968, sleep has been evaluated by applying standardized scoring criteria to electroencephalograms and electromyography recordings developed by Rechtshanffen and Kales (25) and later modified by the American Academy of Sleep Medicine. The limitation of qualitative sleep staging is that stage N2 is scored only if one or more trains of K-complexes or sleep spindles can be observed during a 30-second epoch. Moreover, qualitative staging does not quantify the number of spindles, which have been shown to correlate with markers of cognitive performance (23). As PD involves the thalamocortical circuits in which spindles are generated (3), and spindle density predicts the development of PD dementia (10), we investigated the effects of exercise on spindle density. We found that exercise significantly prevented the decrease in spindle density experienced by the SH-C group during the first half of sleep. The rationale behind evaluating NREM separately during the first and second halves of the night is that physiologically, NREM sleep dominates during the first half of the night, whereas REM sleep dominates during the latter half (26). In addition, the first half of the night appears to have a differential effect on spindle activity, based on previous studies. For example, in a study by Dang-vu and colleagues, predictive relationships between spindle density and stress were only observed during the early stages of NREM sleep (27). Furthermore, Lopez and colleagues found that teenagers who were depressed had lower spindle density during the third and fourth stages of their NREM sleep cycle than those who were not depressed (28). It may be possible that in PD the beneficial effects of exercise are modulating NREM during the first half of the night. Also, the reason for the decrease in spindle density in the no-exercise group could represent natural progression of disease or may be related to other causes. However, the expected longitudinal change in spindle density is not well established in PD, so will need additional study. To our knowledge, no prior study has investigated the effects of exercise on sleep qEEG in neurodegenerative disease (16).
There are many proposed mechanisms to explain ways in which exercise may affect sleep, including exercise-induced decreases in inflammation, changes in core body temperature, changes in neurotransmitters that affect sleep, alterations in growth hormone or brain-derived neurotrophic factor (BDNF), changes in heart rate variability, and changes in autonomic function (29–32). In the parent clinical trial, sleep efficiency was improved in the exercise group, which may have resulted in part from increased spindle density since spindles play a crucial role in maintaining and sustaining sleep (2, 3). The mechanism underlying the exercise-induced alterations in sleep spindles cannot be determined from the current study but should be explored in future studies.
The contribution of sleep spindles to cognitive function is well established in physiological aging, mild cognitive impairment and neurodegenerative diseases (10, 33–36). Furthermore, a longitudinal study by Latreille and colleagues has demonstrated that baseline spindle density is also predictive of the development of dementia in patients with PD (10). Therefore, we evaluated the relationship between exercise-induced changes in spindles and changes in cognition. Change scores in spindle density were positively correlated with improved performance in the memory domain in the exercise group but not in the sleep hygiene group. Even though these findings are exploratory, given the prevalence of cognitive decline in PD, and the limited available treatment options, this is an exciting new development that should be further examined in future research.
Sleep spindle amplitude represents synaptic strength and synchronization of excitatory postsynaptic potentials and prior work has shown that PD patients have reduced spindle amplitude compared to controls (10). In this cohort, exercise did not significantly change sleep spindle amplitude. Therefore, it is not surprising that neither EX or SH-C showed correlations between changes in spindle amplitude and memory performance. It is necessary to conduct additional studies to confirm these findings; however, it may be that exercise-induced changes are more sensitive to spindle density than to amplitude.
There are several strengths to this study, including the utilization of polysomnography-derived EEG collected during a randomized, controlled clinical trial and the use of automated qEEG analysis methods. There are also several important limitations, including the post hoc, exploratory nature of the analysis. Additionally, there was no correction for multiple comparisons because one purpose of this analysis was to generate hypotheses for future research. However, it is important to be aware of this when interpreting the findings.
In conclusion, to our knowledge, the present study is the first to examine the effects of exercise on quantitative sleep spindle morphology in PD. Exercise rehabilitation may have potential benefits in preventing a decline in spindle density during the first half of the night compared to a no-exercise control group. Further, the effects of exercise on spindle density were positively correlated with better memory performance. Due to the exploratory nature of this study, future studies should examine the potential beneficial role of rehabilitation-induced changes in spindle density and cognition, given the high prevalence of this nonmotor manifestation and the limited treatment options available. These findings have important therapeutic implications and represent an exciting advance in the search for non-pharmacological treatments for this common and debilitating non-motor symptom.
Data availability statement
The original contributions presented in the study are included in the article/Suplementary Material, further inquiries can be directed to the corresponding author/s.
Ethics statement
The studies involving human participants were reviewed and approved by University of Alabama at Birmingham Institutional Review Board. The patients/participants provided their written informed consent to participate in this study.
Author contributions
AAM, ZI, MB, SM, and AWA were involved in conceptualization of the research project; AAM, CC, JLP, RAM, AJ, KHW, MB, and AWA were involved in organization of the research project; AAM, CC, ZI, RAM, AJ, KHW, MG, and AWA were involved in execution of the research project; AAM, KHW, GC, and AWA were involved in execution of the statistical analysis; AAM, CC, ZI, RAM, AJ, AHW, GC, MB, SM, and AWA were involved in review and critique of statistical analysis; AAM wrote the first draft, AAM, CC, ZI, JLP, RAM, AJ, KHW, GC, MB, SM, and AWA were involved in review and critique of the manuscript. All authors contributed to the article and approved the submitted version.
Funding
AM received funding from NINDS R25NS079188 Training Fellowship; KW received funding from NIH T32 HD071866 Training Fellowship; AWA received funding from NIH (K23NS080912 and R01HD100670). SM received funding from NIH (K23NS097576).
Acknowledgments
We are grateful to the research participants, without whom this study would not have been possible.
Conflict of interest
The authors declare that the research was conducted in the absence of any commercial or financial relationships that could be construed as a potential conflict of interest.
Publisher's note
All claims expressed in this article are solely those of the authors and do not necessarily represent those of their affiliated organizations, or those of the publisher, the editors and the reviewers. Any product that may be evaluated in this article, or claim that may be made by its manufacturer, is not guaranteed or endorsed by the publisher.
References
1. Weber F, Dan Y. Circuit-based interrogation of sleep control. Nature. (2016) 538(7623):51–9. doi: 10.1038/nature19773
2. Weiner OM, Dang-Vu TT. Spindle oscillations in sleep disorders: a systematic review. Neural Plast. (2016) 2016:7328725. doi: 10.1155/2016/7328725
3. Fernandez LMJ, Lüthi A. Sleep spindles: mechanisms and functions. Physiol Rev. (2020) 100(2):805–68. doi: 10.1152/physrev.00042.2018
4. Dijk DJ, Beersma DG, van den Hoofdakker RH. All night spectral analysis of EEG sleep in young adult and middle-aged male subjects. Neurobiol Aging. (1989) 10(6):677–82. doi: 10.1016/0197-4580(89)90004-3
5. Rukavina K, Batzu L, Boogers A, Abundes-Corona A, Bruno V, Chaudhuri KR. Non-motor complications in late stage Parkinson's disease: recognition, management and unmet needs. Expert Rev Neurother. (2021) 21(3):335–52. doi: 10.1080/14737175.2021.1883428
6. Simuni T, Caspell-Garcia C, Coffey CS, Weintraub D, Mollenhauer B, Lasch S, et al. Baseline prevalence and longitudinal evolution of non-motor symptoms in early Parkinson’s disease: the PPMI cohort. J Neurol Neurosurg Psychiatry. (2018) 89(1):78–88. doi: 10.1136/jnnp-2017-316213
7. Lees AJ, Blackburn NA, Campbell VL. The nighttime problems of Parkinson's disease. Clin Neuropharmacol. (1988) 11(6):512–9. doi: 10.1097/00002826-198812000-00004
8. Nausieda PA, Weiner WJ, Kaplan LR, Weber S, Klawans HL. Sleep disruption in the course of chronic levodopa therapy: an early feature of the levodopa psychosis. Clin Neuropharmacol. (1982) 5(2):183–94. doi: 10.1097/00002826-198205020-00003
9. Amara AW, Chahine LM, Videnovic A. Treatment of sleep dysfunction in Parkinson's disease. Curr Treat Options Neurol. (2017) 19(7):26. doi: 10.1007/s11940-017-0461-6
10. Latreille V, Carrier J, Lafortune M, Postuma RB, Bertrand J-A, Panisset M, et al. Sleep spindles in Parkinson's disease may predict the development of dementia. Neurobiol Aging. (2015) 36(2):1083–90. doi: 10.1016/j.neurobiolaging.2014.09.009
11. Wei Y, Krishnan GP, Komarov M, Bazhenov M. Differential roles of sleep spindles and sleep slow oscillations in memory consolidation. PLoS Comput Biol. (2018) 14(7):e1006322. doi: 10.1371/journal.pcbi.1006322
12. Reynolds GO, Otto MW, Ellis TD, Cronin-Golomb A. The therapeutic potential of exercise to improve mood, cognition, and sleep in Parkinson’s disease. Mov Disord. (2016) 31(1):23–38. doi: 10.1002/mds.26484
13. Amara AW, Memon AA. Effects of exercise on non-motor symptoms in Parkinson's disease. Clin Ther. (2018) 40(1):8–15. doi: 10.1016/j.clinthera.2017.11.004
14. Cristini J, Weiss M, De Las Heras B, Medina-Rincón A, Dagher A, Postuma RB, et al. The effects of exercise on sleep quality in persons with Parkinson's disease: a systematic review with meta-analysis. Sleep Med Rev. (2021) 55:101384. doi: 10.1016/j.smrv.2020.101384
15. Amara AW, Wood KH, Joop A, Memon RA, Pilkington J, Tuggle SC, et al. Randomized, controlled trial of exercise on objective and subjective sleep in Parkinson's disease. Mov Disord. (2020) 35(6):947–58. doi: 10.1002/mds.28009
16. Memon AA, Coleman JJ, Amara AW. Effects of exercise on sleep in neurodegenerative disease. Neurobiol Dis. (2020) 140:104859. doi: 10.1016/j.nbd.2020.104859
17. Postuma RB, Berg D, Stern M, Poewe W, Olanow CW, Oertel W, et al. MDS Clinical diagnostic criteria for Parkinson's disease. Mov Disord. (2015) 30(12):1591–601. doi: 10.1002/mds.26424
18. Kelly NA, Ford MP, Standaert DG, Watts RL, Bickel CS, Moellering DR, et al. Novel, high-intensity exercise prescription improves muscle mass, mitochondrial function, and physical capacity in individuals with Parkinson's disease. J Appl Physiol. (2014) 116(5):582–92. doi: 10.1152/japplphysiol.01277.2013
19. Walton RG, Dungan CM, Long DE, Tuggle SC, Kosmac K, Peck BD, et al. Metformin blunts muscle hypertrophy in response to progressive resistance exercise training in older adults: a randomized, double-blind, placebo-controlled, multicenter trial: the MASTERS trial. Aging Cell. (2019) 18(6):e13039. doi: 10.1111/acel.13039
20. Stec MJ, Thalacker-Mercer A, Mayhew DL, Kelly NA, Tuggle SC, Merritt EK, et al. Randomized, four-arm, dose-response clinical trial to optimize resistance exercise training for older adults with age-related muscle atrophy. Exp Gerontol. (2017) 99:98–109. doi: 10.1016/j.exger.2017.09.018
21. Berry RB, Albeertario CL, Harding SM, Uoyd RM, Plante DT, Quan SF, et al. The AASM manual for the scoring of sleep and associated events: Rules, terminology and technical specifications. Darien, IL: American Academy of Sleep Medicine (2018).
22. Helfrich RF, Mander BA, Jagust WJ, Knight RT, Walker MP. Old brains come uncoupled in sleep: slow wave-spindle synchrony, brain atrophy, and forgetting. Neuron. (2018) 97(1):221–230.e4. doi: 10.1016/j.neuron.2017.11.020
23. Purcell SM, Manoach DS, Demanuele C, Cade BE, Mariani S, Cox R, et al. Characterizing sleep spindles in 11,630 individuals from the National Sleep Research Resource. Nat Commun. (2017) 8:15930. doi: 10.1038/ncomms15930
24. Seppi K, Ray Chaudhuri K, Coelho M, Fox SH, Katzenschlager R, Perez Lloret S, et al. Update on treatments for nonmotor symptoms of Parkinson's disease-an evidence-based medicine review. Mov Disord. (2019) 34(2):180–98. doi: 10.1002/mds.27602
25. Rechtschaffen A, Kales A. A manual of standardized terminology, techniques and scoring system for sleep stages of human subjects. Washington, DC: Public Health Service, US Government Printing Office (1968).
26. Carley DW, Farabi SS. Physiology of sleep. Diabetes Spectr. (2016) 29(1):5–9. doi: 10.2337/diaspect.29.1.5
27. Dang-Vu TT, Salimi A, Boucetta S, Wenzel K, O’Byrne J, Brandewinder M, et al. Sleep spindles predict stress-related increases in sleep disturbances. Front Hum Neurosci. (2015) 9:68. 3389/fnhum.2015.00068 25713529
28. Lopez J, Hoffmann R, Armitage R. Reduced sleep spindle activity in early-onset and elevated risk for depression. J Am Acad Child Adolesc Psychiatry. (2010) 49(9):934–43. doi: 10.1016/j.jaac.2010.05.014
29. Kredlow MA, Capozzoli MC, Hearon BA, Calkins AW, Otto MW. The effects of physical activity on sleep: a meta-analytic review. J Behav Med. (2015) 38(3):427–49. doi: 10.1007/s10865-015-9617-6
30. Uchida S, Shioda K, Morita Y, Kubota C, Ganeko M, Takeda N. Exercise and sleep - review and future directions. JPFSM. (2012) 1(2):317–24. doi: 10.7600/jpfsm.1.317
31. Szuhany KL, Bugatti M, Otto MW. A meta-analytic review of the effects of exercise on brain-derived neurotrophic factor. J Psychiatr Res. (2015) 60:56–64. doi: 10.1016/j.jpsychires.2014.10.003
32. Sellami M, Bragazzi NL, Slimani M, Hayes L, Jabbour G, De Giorgio A, et al. The effect of exercise on glucoregulatory hormones: a countermeasure to human aging: insights from a comprehensive review of the literature. Int J Environ Res Public Health. (2019) 16(10):1709–25. doi: 10.3390/ijerph16101709
33. Westerberg CE, Mander BA, Florczak SM, Weintraub S, Mesulam M-M, Zee PC, et al. Concurrent impairments in sleep and memory in amnestic mild cognitive impairment. J Int Neuropsychol Soc. (2012) 18(3):490–500. doi: 10.1017/S135561771200001X
34. Fogel S, Martin N, Lafortune M, Barakat M, Debas K, Laventure S, et al. NREM sleep oscillations and brain plasticity in aging. Front Neurol. (2012) 3:176. doi: 10.3389/fneur.2012.00176
35. Lafortune M, Gagnon J-F, Martin N, Latreille V, Dubé J, Bouchard M, et al. Sleep spindles and rapid eye movement sleep as predictors of next morning cognitive performance in healthy middle-aged and older participants. J Sleep Res. (2014) 23(2):159–67. doi: 10.1111/jsr.12108
Keywords: Parkinson’s disease, sleep spindles, exercise, sleep, cognition
Citation: Memon AA, Catiul C, Irwin Z, Pilkington J, Memon RA, Joop A, Wood KH, Cutter G, Bamman M, Miocinovic S and Amara AW (2022) Effects of exercise on sleep spindles in Parkinson's disease. Front. Rehabilit. Sci. 3:952289. doi: 10.3389/fresc.2022.952289
Received: 24 May 2022; Accepted: 18 July 2022;
Published: 11 August 2022.
Edited by:
Javier Güeita-Rodriguez, Rey Juan Carlos University, SpainReviewed by:
Lorenzo Appendini, Ospedale Civile di Saluzzo, ItalyJack Jiaqi Zhang, Hong Kong Polytechnic University, Hong Kong, China
© 2022 Memon, Catiul, Irwin, Pilkington, Memon, Joop, Wood, Cutter, Bamman, Miocinovic and Amara. This is an open-access article distributed under the terms of the Creative Commons Attribution License (CC BY). The use, distribution or reproduction in other forums is permitted, provided the original author(s) and the copyright owner(s) are credited and that the original publication in this journal is cited, in accordance with accepted academic practice. No use, distribution or reproduction is permitted which does not comply with these terms.
*Correspondence: Amy W. Amara YWFtYXJhQHVhYm1jLmVkdQ==
Specialty Section: This article was submitted to Interventions for Rehabilitation, a section of the journal Frontiers in Rehabilitation Sciences