- 1Department of Rehabilitation Medicine, Kariya Toyota General Hospital, Kariya, Japan
- 2Assistive Robot Center, National Center for Geriatrics and Gerontology, Obu, Japan
- 3Department of Rehabilitation Medicine I, School of Medicine, Fujita Health University, Toyoake, Japan
Wearable devices for the quantification of walking have recently been adopted for gait rehabilitation. To apply this method in subacute rehabilitation settings, this approach must be effective in these populations and implemented as a feasible method in terms of adherence and safety, especially the risk of falling. This study aimed to investigate the feasibility and efficacy of an activity monitoring approach in subacute rehabilitation using a commercially available pedometer validated with slow walking. This randomized controlled study with blinded assessors recruited 29 patients admitted to a rehabilitation ward. The participants were randomly assigned to either the feedback (intervention) or the no-feedback (control) group. Participants in both groups received at least 120 min of therapy sessions every day for 6 or 7 days per week while wearing pedometers on their unaffected ankles from the day they were permitted to walk independently till discharge. Only participants in the feedback group received weekly encouragement and the next goals. The primary outcome was the change in the 6-minute walking distance (Δ6MD). Feasibility (percentage of pedometer data acquisition days in the total observational period and the number of falls) and other efficacy outcomes (step counts, gait speed, 30-seconds chair stand test, Berg Balance Scale, and Timed Up and Go Test) were also evaluated. Regarding feasibility outcomes, the data acquisition rate was 94.1% and the number of falls during the observation period was one in the feedback group. Regarding efficacy outcomes, Δ6MD was not significantly greater in the feedback group [mean (standard deviation): 79.1 (51.7) m] than in the no-feedback group [86.1 (65.4) m] (p = 0.774) and the other five secondary outcomes showed no between-group difference. Considering the large number of steps per day in both groups [6,912 (4,751) and 5,600 (5,108) steps in the feedback and no-feedback group, respectively], the effect of the intended intervention might have been masked by the effect of simply wearing pedometers in the control group. This study revealed that the activity monitoring approach using an ankle-worn pedometer was practical in terms of adherence and safety. Further clinical trials are required to elucidate ways to effectively use wearable devices in subacute rehabilitation.
1. Introduction
Enhancing a patient's walking ability is one of the most common goals in subacute rehabilitation settings, regardless of the underlying disease. As evidence suggests that high-volume, task-specific training leads to greater efficacy (1, 2), it is essential to increase the walking dose in order to improve walking ability. Training sessions provided by physical therapists are often limited in terms of time and amount of training; therefore, increasing walking activity outside of supervised rehabilitation sessions could be a strategy to increase the walking dose.
Recently, activity monitoring approaches using wearable monitors, pedometers, or accelerometers have been applied to promote voluntary walking activity as a part of rehabilitative intervention in various clinical scenarios, such as cardiac rehabilitation (3, 4), pulmonary rehabilitation (5, 6) and stroke rehabilitation (7–10). These devices can quantify walking activity by step counts or the duration of walking bouts, and feedback from this information helps patients and physical therapists discuss specific goals and plans for increasing walking activity. Several systematic reviews have revealed significant increases in physical activity in some populations using pedometers and activity monitors (11–15). Such activity-monitoring approaches are expected to be effective in subacute rehabilitation settings, as well as in other rehabilitative situations. However, to the best of our knowledge, this has not yet been fully elucidated as previous studies have targeted specific disease types, as opposed to a mixed population as would be met in clinical settings. Additionally, considering clinical implementation, the approach should be feasible in terms of adherence and safety. Regarding adherence, the wearability of these devices, such as wearing around the waist with a band (16) or attaching to the front of the mid-thigh (17), are likely to decrease patient compliance when implemented in clinical settings due to discomfort and difficulty in handling. Moreover, there is often a laterality of symptoms due to the presence of affected and unaffected sides resulting from hemiparetic stroke, fracture, or Parkinsonism in subacute rehabilitation patients. It is known that devices are generally most accurate when positioned on the unaffected ankle (18, 19); however, adherence to this technically recommended position is yet to be revealed in these populations. Regarding safety, subacute rehabilitation inpatients have a potentially high risk of falls, and increased physical activity could further raise their risk of falls due to increased exposure to environmental hazards (20, 21). Therefore, the assessment of falls is necessary to examine the safety of the activity monitoring approach.
Another topic of the activity-monitoring approach is that it remains unclear whether the increase in physical activity gained through activity monitoring and feedback results in improved walking ability, which is the ultimate goal of gait rehabilitation, as pointed out in previous reviews (14). For example, in a randomized controlled trial (RCT) that explored the effects of providing activity data obtained by accelerometers to inpatients and clinicians in post-acute geriatric rehabilitation units (22), the time spent walking outside of therapy sessions was significantly longer in the intervention group. However, the effect on walking ability, as measured by gait speed, was not statistically significant. Similarly, an international RCT exploring the effect of providing the walking activity record in inpatients with subacute stroke (8) showed no significant between-group difference in 15-meter walking speed. However, some RCTs that targeted outpatients with chronic obstructive pulmonary disease (5) or chronic stroke (9) revealed the effects of activity monitoring and feedback on 6-meter walking distance. These results may suggest that the activity-monitoring approach affects walking endurance rather than other walking abilities such as walking speed.
Therefore, the present study aimed to investigate the feasibility and efficacy on physical function of an activity monitoring approach that can be implemented in various individuals during the subacute rehabilitation phase, regardless of the disease type. Based on previous studies, we hypothesized that the activity-monitoring approach would generate greater improvements in walking endurance in inpatients undergoing subacute rehabilitation. This pilot study was conducted to estimate the effect size of this type of intervention in a subacute rehabilitation setting.
2. Materials and methods
2.1. Study design and settings
This randomized controlled study was conducted as a parallel, assessors-blinded, 2-group design in a convalescent rehabilitation ward, a system for intensive inpatient rehabilitation for patients during the subacute period, covered by Japanese governmental medical insurance, in a general hospital. The study protocol was approved by the hospital's institutional review board (No.397) and registered in the UMIN Clinical Trials Registry (UMIN000032265). All the participants provided written informed consent.
2.2. Participants
Inpatients admitted to the convalescent rehabilitation ward between May 2018 and March 2020 were recruited. The inclusion criteria were (a) Functional Ambulation Category (23) 3 or more (dependent, supervision) and (b) the Japanese version of the Mini-Mental State Examination (MMSE-J) (24) score of ≥24. Individuals with a walking speed less than 0.4 meters/second (m/sec) in a 10-meter walking test were excluded because the accuracy of the fitness trackers might not be assured in these slow walking populations as shown in previous studies (19, 25). In addition, individuals unable to understand verbal instructions due to aphasia or medical reasons that could restrict the amount of walking ability, such as uncontrolled joint pain, were excluded.
2.3. Randomization
After the eligibility criteria were ascertained by the rehabilitation physicians (EO, KO, KY), an independent investigator who did not engage in enrollment or assessment (AH) assigned the participants using permuted block randomization concealed from others to one of two groups: feedback (intervention) or no-feedback (control).
2.4. Intervention
All participants in both groups wore Fitbit One (Fitbit Inc., San Francisco, CA, United States) all day except bath time from the day of assignment to discharge. The Fitbit One was used as an example of a commercially available physical activity monitor validated in the targeted population and providing instantaneous visual feedback of walking steps on the display. At slower walking speeds typically seen in rehabilitation patients, the ankle-positioned accelerometer has proven to be more accurate (19, 26) as sensors placed on the distal position of the lower limb can detect lower acceleration amplitudes. This device has also been proven to measure walking steps accurately in slow walking populations including cane-users (0.59–0.4 m/sec) when positioned on the ankle (27) with the same accuracy as the StepWatch Activity Monitor, the gold standard for assessing step count in the population undergoing rehabilitation, when positioned on the non-paretic ankle (25). Based on these previous studies, the Fitbit was positioned just above the lateral malleolus on the unaffected side (Figure 1).
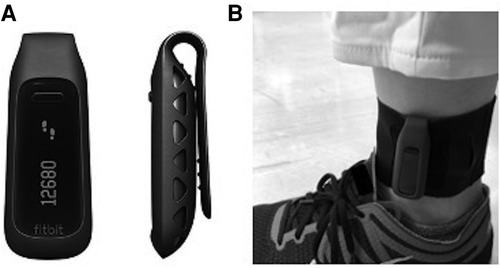
Figure 1. Wearable device for activity monitoring. (A) Front and side views of Fitbit One (Fitbit Inc., San Francisco, CA, United States). A display shows the current step count. (B) Fitbit One worn on the ankle with a soft elastic band.
Participants in the feedback (intervention) group received the activity-monitoring approach from the day they were permitted to walk independently in a ward until the day of discharge. Permission to walk independently was provided by the physicians when they met all the prescribed criteria for assessing their ability to safely walk and perform activities of daily living in the ward. As part of the activity-monitoring approach, they were first instructed to self-record steps per day at the end of the day in a diary, making use of the display of Fitbit One, which can provide instantaneous information on walking steps. This process was established to encourage participants in the feedback group to pay attention to their activities. Moreover, to strengthen the intended intervention of promoting voluntary walking activity, the physicians in charge motivated them weekly by visualizing their weekly walking amounts using graphs and presenting goals for the next week. They were encouraged to increase steps per day by 10% compared with the average step counts in the previous week, as the specific goals and feedback of progress toward goals can increase motivation and self-efficacy and promote practical action according to goal-setting theory (28–30). Corridors of 50 m in the ward were permitted to voluntarily practice walking. This cycle continued until the discharge. In contrast, the no-feedback (control) group did not provide instructions for receiving feedback from the display or weekly feedback from the staff during hospitalization. On discharge home, they were provided with summarized data on their step counts and general advice for maintaining physical activities at home.
As is provided to all the inpatients in the rehabilitation ward, participants in both the feedback (intervention) and no-feedback (control) groups received at least a 60-minute physical therapy session and a 60-minute occupational therapy session, 6 or 7 days per week throughout the study period. The sessions typically included walking retraining on the ground or stairs and non-gait-related activities such as clothing, bathing, and cooking, all of which are needed for discharge home.
2.5. Outcomes
The primary outcome was the change in the 6-minute walking distance (6MD) during the observational period, that is, from the day the participants were permitted to walk independently in a ward until the day before discharge. As secondary efficacy outcomes, blinded assessors performed a 10-meter walking test, a 30-second chair stand test (31) as a measure of lower muscle power, Berg Balance Scale (32) as a measure of balance (0: worst—56: best), and Timed Up and Go Test (33) to assess functional mobility. The number of steps per day recorded by the Fitbit device was also analyzed as a secondary efficacy outcome.
Regarding feasibility outcomes, two kinds of indicators were assessed. First, the pedometer data acquisition rate, which was calculated as the ratio of the number of days that pedometer data was obtained to the days of the whole observational period, was examined as an indicator of adherence. Secondly, the number of falls during the monitoring period was examined as an indicator of safety as increased activity may result in a greater risk of falling for these fall-prone populations.
2.6. Sample size
As there was no prior information to base the sample size for this pilot study, we set the sample size to 12 per group based on the minimum sample size required in a pilot study (34). Expecting some dropouts, we aimed to include 30 participants.
2.7. Blinding
This was a single-blind study. All therapists who served as assessors were unaware of the group allocation. As the objective of the intervention was to make the participants aware of their walking activity through pedometer monitoring, the participants could not be blinded.
2.8. Statistical analyses
Baseline comparisons between the feedback (intervention) and no-feedback (control) groups were performed using the unpaired t-test for numerical variables and Fisher's exact test for categorical variables. All analyses were conducted following the modified intention-to-treat principle, whereby patients with missing outcome data were excluded. The outcome measures were first analyzed using an unpaired t-test and the effect sizes (Cohen's d) were calculated. Statistical analyses were performed using STATA/SE 13.1 (StataCorp., Texas, United States). Any p-values less than 0.05 were considered statistically significant.
3. Results
3.1. Baseline characteristics
A total of 29 participants were randomly assigned to the feedback group (n = 16) or the no-feedback group (n = 13). The participants’ characteristics, including the primary disease, are shown in Table 1. There were no significant differences in the demographic and disease characteristics and baseline assessments between the two groups. Of these, two participants in the feedback group had missing outcome data due to the failure of the monitoring device. Two other participants, one in the feedback group and one in the no-feedback group, refused to continue receiving the allocated intervention. One participant withdrew because of ankle pain on the unaffected side. The flow diagram of the participants is shown in Figure 2.
3.2. Efficacy outcomes
The changes in 6MD were not greater in the feedback group [mean (standard deviation, SD):79.1 (51.7) m] than in the no-feedback group [86.1 (65.4) m; p = 0.774]. There were no significant differences in the other secondary outcomes between the groups (Table 2). The mean (SD) steps per day in the feedback group were 6,912 (4,751) steps, larger than in the no-feedback group [5,600 (5,108) steps], but with no significant difference (p = 0.522) and a small effect size (d = 0.266). Similarly, the mean (SD) values of the total number of steps during the whole monitoring period were 140,246 (124,994) steps in the feedback group and 135,081 (124,950) steps in the no-feedback group, with no significant difference (p = 0.920) and a very small effect size (d = 0.041).
3.3. Feasibility outcomes
Sensor data were obtained on 94.1% of all observational days for the feedback group and 97.9% for the no-feedback group, with no significant difference between groups (p = 0.204). The overall data acquisition rate was 96.0%.
Regarding adverse events, the number of falls during the observational period was zero in the no-feedback group and one in the feedback group, which was calculated as 3.6 per 1,000 person-days fall rate. This fall rate was sufficiently small in comparison with the fall rate in Japanese rehabilitation wards reported previously, ranging from 4.6 (35) to 13.9 (36). No other adverse events were reported.
4. Discussion
This pilot randomized controlled trial aimed to examine whether activity monitoring and feedback intervention could be feasible and enhance the improvement in walking ability among individuals in a subacute rehabilitation ward. The results demonstrated excellent adherence to device use, a sufficiently small fall rate, and a large number of steps, regardless of the experimental group. However, the outcome measures of walking ability and other related outcome measures did not show significant improvement in the intervention group.
In the present study, adherence to device use was as high as 96.0% across all participants, which was remarkably high, despite the fact that the participants wore pedometers by themselves. This tendency is more evident than that found in a previous study, in which the sensors were placed on both ankles in early subacute stroke patients, and sensor data were reported to be obtained for 84.4% of all study days (8). From these results, positioning activity-monitoring devices on the ankle is considered to be an acceptable and continuable way of activity monitoring for these populations under subacute rehabilitation. In addition, the eligibility criteria of the present study included normal MMSE-J scores, because recording steps per day by the participants themselves was required for the intended intervention. This condition might have favorably affected the high utilization rate of the devices in that participants with better cognitive function could sufficiently understand the necessity of wearing the pedometers for their rehabilitation regardless of the experimental group, which is supported by a previous study (37) suggesting a relationship between cognitive function and the number of daily steps in a convalescent rehabilitation ward. The very high adherence to the device use is an advantage of this intervention method in that it can be delivered and practiced as intended without failure. Furthermore, the results showed that the fall rate was very low even though the participants were encouraged to be more active. Subacute rehabilitation patients have a potentially high risk of falls because their physical function and walking ability are in the process of improving and are still inadequate for daily life. The risk of falls generally tends to increase as the level of physical activity is enhanced (21). However, the present study revealed that gaining physical activity can be achieved safely after patients are certified to have sufficient walking ability in a standardized manner.
The intended intervention in the present study did not significantly enhance the change in 6MD compared with the control group. One possible reason might be that there was no between-group difference in the number of steps per day between the two experimental groups, as a greater dose of walking activity is considered necessary to obtain greater improvement in walking ability. In fact, both the intervention group and the control group had a larger number of steps compared with typical steps per day in inpatients undergoing rehabilitation, that is, around or below 5,000 steps per day in inpatients with subacute stroke (1, 7) and cardiac rehabilitation inpatients (4). Furthermore, both experimental groups had comparable or improved 6MD as compared to similar intervention studies (58–61 m) (1). Considering this result, the effect of wearing pedometers on increasing walking activity could have emerged not only in the intervention group but also in the control group. Several systematic reviews have reported that the use of physical activity monitors significantly enhanced the daily number of steps with an average of 2,491 steps per day in adults (11) and 1,297 steps in older adults (12) compared to the control groups without physical activity monitors, regardless of disease or intervention details. These reviews discussed that all participants could be encouraged to increase their physical activity simply due to participation in activity-monitoring studies, with the awareness that they are being measured, whether there are any other interventions, or not. Moreover, the displays on pedometers worn in the control group were not concealed in the present study. Although we did not provide instructions on how to view the display, it cannot be denied that some participants in the control group might have learned how to use them and checked their step counts. Therefore, the effect size of the intended intervention in the present study may have been masked by improvements in the control group, as noted in a previous study (38). These interpretations are reinforced by the fact that a few participants in the control group reported a large average number of steps per day (approximately 15,000 steps per day). To overcome this problem, the method of monitoring physical activity as a study outcome needs to be reconsidered by combining devices or technologies that can measure step counts without participants’ awareness, such as insole sensors (39, 40) in addition to ankle-worn pedometers as an intended intervention. The other possible reason could be that the effect of the intervention was masked by participants with high physical activity or in a good condition at the baseline. This possibility is derived by, as in Table 2, participants in both experimental groups having a very large number of daily steps or having a very short hospital stay. This is partly because the present study dealt with a real-world heterogeneous population as a feature of the study. Some research on physical activity suggests that participants with higher baseline levels of physical activity tend to show less improvement than those with relatively lower levels of physical activity at baseline (9, 41). Thus, in future studies, screening for baseline physical activity or general condition and excluding patients who are active or in good condition before enrollment may be necessary to elucidate the effectiveness and medical indications of this activity-monitoring approach in subacute rehabilitation.
This study was limited by its small sample size, as is the nature of a pilot study. Moreover, participants could not be blinded to the group assignment as the intervention required patients to be aware of their own physical activity; however, therapists who assessed outcomes were blinded to the group assignment. Finally, as the present study was conducted among participants with normal cognitive function (MMSE-J ≥24), the feasibility of this activity-monitoring approach using wearable devices might not be generalized among those with cognitive decline, as the barriers to acceptance and use of assistive technologies among people with cognitive impairment are frequently discussed (42).
In conclusion, the present study revealed that the activity monitoring and feedback approach using an ankle-worn pedometer was practical in terms of adherence and risk of falls. Both the intervention and control groups had a larger number of steps per day than usual rehabilitation inpatients; however, the changes in 6MD and other outcomes were not significantly greater in the intervention group than in the control group. The effect of the intended intervention might have been masked by the effect of simply wearing pedometers in the control group, considering the large number of steps taken and the improvement in 6MD in both experimental groups. Although the present study has revealed little effect, further clinical trials with better designs might elucidate better ways to effectively use wearable activity-monitoring devices in subacute rehabilitation settings.
Data availability statement
The raw data supporting the conclusions of this article will be made available by the authors, without undue reservation.
Ethics statement
The studies involving human participants were reviewed and approved by Kariya Toyota General Hospital institutional review board. The patients/participants provided their written informed consent to participate in this study.
Author contributions
EO and KO determined the study concept and design, collected and analyzed the data, and edited the manuscript. TH, KY, and SM participated in data collection and manuscript editing. AH participated in the assignment of enrolled participants. YO participated in study conceptualization, data analysis, and manuscript revision. All authors contributed to the article and approved the submitted version.
Acknowledgments
The authors thank the members of the Department of Rehabilitation at Kariya Toyota General Hospital for their technical support in this study.
Conflict of interest
The authors declare that the research was conducted in the absence of any commercial or financial relationships that could be construed as a potential conflict of interest.
Publisher's note
All claims expressed in this article are solely those of the authors and do not necessarily represent those of their affiliated organizations, or those of the publisher, the editors and the reviewers. Any product that may be evaluated in this article, or claim that may be made by its manufacturer, is not guaranteed or endorsed by the publisher.
References
1. Klassen TD, Dukelow SP, Bayley MT, Benavente O, Hill MD, Krassioukov A, et al. Higher doses improve walking recovery during stroke inpatient rehabilitation. Stroke. (2020) 51(9):2639–48. doi: 10.1161/strokeaha.120.029245
2. Said CM, McGinley JL, Szoeke C, Workman B, Hill KD, Wittwer JE, et al. Factors associated with improved walking in older people during hospital rehabilitation: secondary analysis of a randomized controlled trial. BMC Geriatr. (2021) 21(1):90. doi: 10.1186/s12877-021-02016-0
3. Furber S, Butler L, Phongsavan P, Mark A, Bauman A. Randomised controlled trial of a pedometer-based telephone intervention to increase physical activity among cardiac patients not attending cardiac rehabilitation. Patient Educ Couns. (2010) 80(2):212–8. doi: 10.1016/j.pec.2009.11.012
4. Izawa KP, Watanabe S, Hiraki K, Morio Y, Kasahara Y, Takeichi N, et al. Determination of the effectiveness of accelerometer use in the promotion of physical activity in cardiac patients: a randomized controlled trial. Arch Phys Med Rehabil. (2012) 93(11):1896–902. doi: 10.1016/j.apmr.2012.06.015
5. Mendoza L, Horta P, Espinoza J, Aguilera M, Balmaceda N, Castro A, et al. Pedometers to enhance physical activity in copd: a randomised controlled trial. Eur Respir J. (2015) 45(2):347–54. doi: 10.1183/09031936.00084514
6. Kawagoshi A, Kiyokawa N, Sugawara K, Takahashi H, Sakata S, Satake M, et al. Effects of low-intensity exercise and home-based pulmonary rehabilitation with pedometer feedback on physical activity in elderly patients with chronic obstructive pulmonary disease. Respir Med. (2015) 109(3):364–71. doi: 10.1016/j.rmed.2015.01.008
7. Mansfield A, Wong JS, Bryce J, Brunton K, Inness EL, Knorr S, et al. Use of accelerometer-based feedback of walking activity for appraising progress with walking-related goals in inpatient stroke rehabilitation: a randomized controlled trial. Neurorehabil Neural Repair. (2015) 29(9):847–57. doi: 10.1177/1545968314567968
8. Dorsch AK, Thomas S, Xu X, Kaiser W, Dobkin BH. Sirract: an international randomized clinical trial of activity feedback during inpatient stroke rehabilitation enabled by wireless sensing. Neurorehabil Neural Repair. (2015) 29(5):407–15. doi: 10.1177/1545968314550369
9. Danks KA, Pohlig R, Reisman DS. Combining fast-walking training and a step activity monitoring program to improve daily walking activity after stroke: a preliminary study. Arch Phys Med Rehabil. (2016) 97(9 Suppl):S185–93. doi: 10.1016/j.apmr.2016.01.039
10. Kanai M, Izawa KP, Kobayashi M, Onishi A, Kubo H, Nozoe M, et al. Effect of accelerometer-based feedback on physical activity in hospitalized patients with ischemic stroke: a randomized controlled trial. Clin Rehabil. (2018) 32(8):1047–56. doi: 10.1177/0269215518755841
11. Bravata DM, Smith-Spangler C, Sundaram V, Gienger AL, Lin N, Lewis R, et al. Using pedometers to increase physical activity and improve health: a systematic review. J Am Med Assoc. (2007) 298(19):2296–304. doi: 10.1001/jama.298.19.2296
12. Larsen RT, Christensen J, Juhl CB, Andersen HB, Langberg H. Physical activity monitors to enhance amount of physical activity in older adults - a systematic review and meta-analysis. Eur Rev Aging Phys Act. (2019) 16:7. doi: 10.1186/s11556-019-0213-6
13. Lynch EA, Jones TM, Simpson DB, Fini NA, Kuys SS, Borschmann K, et al. Activity monitors for increasing physical activity in adult stroke survivors. Cochrane Database Syst Rev. (2018) 7(7):Cd012543. doi: 10.1002/14651858.CD012543.pub2
14. de Leeuwerk ME, Bor P, van der Ploeg HP, de Groot V, van der Schaaf M, van der Leeden M. The effectiveness of physical activity interventions using activity trackers during or after inpatient care: a systematic review and meta-analysis of randomized controlled trials. Int J Behav Nutr Phys Act. (2022) 19(1):59. doi: 10.1186/s12966-022-01261-9
15. Braakhuis HEM, Berger MAM, Bussmann JBJ. Effectiveness of healthcare interventions using objective feedback on physical activity: a systematic review and meta-analysis. J Rehabil Med. (2019) 51(3):151–9. doi: 10.2340/16501977-2522
16. Salih SA, Peel NM. Clinical utility of accelerometry in geriatric rehabilitation. Geriatr Gerontol Int. (2012) 12(4):753–4. doi: 10.1111/j.1447-0594.2012.00844.x
17. Taraldsen K, Askim T, Sletvold O, Einarsen EK, Bjåstad KG, Indredavik B, et al. Evaluation of a body-worn sensor system to measure physical activity in older people with impaired function. Phys Ther. (2011) 91(2):277–85. doi: 10.2522/ptj.20100159
18. Mudge S, Stott NS, Walt SE. Criterion validity of the stepwatch activity monitor as a measure of walking activity in patients after stroke. Arch Phys Med Rehabil. (2007) 88(12):1710–5. doi: 10.1016/j.apmr.2007.07.039
19. Treacy D, Hassett L, Schurr K, Chagpar S, Paul SS, Sherrington C. Validity of different activity monitors to count steps in an inpatient rehabilitation setting. Phys Ther. (2017) 97(5):581–8. doi: 10.1093/ptj/pzx010
20. Rubenstein LZ, Josephson KR. Interventions to reduce the multifactorial risks for falling. In: Masdeu JC, Sudarsky L, Wolfson L, editors. Gait disorders of aging: Falls and therapeutic strategies. Philadelphia: Lippincott-Raven (1997). p. 309–26.
21. Kato Y, Kitamura S, Katoh M, Hirano A, Senjyu Y, Ogawa M, et al. Stroke patients with nearly independent transfer ability are at high risk of falling. J Stroke Cerebrovasc Dis. (2022) 31(1):106169. doi: 10.1016/j.jstrokecerebrovasdis.2021.106169
22. Peel NM, Paul SK, Cameron ID, Crotty M, Kurrle SE, Gray LC. Promoting activity in geriatric rehabilitation: a randomized controlled trial of accelerometry. PLoS One. (2016) 11(8):e0160906. doi: 10.1371/journal.pone.0160906
23. Holden MK, Gill KM, Magliozzi MR, Nathan J, Piehl-Baker L. Clinical gait assessment in the neurologically impaired. reliability and meaningfulness. Phys Ther. (1984) 64(1):35–40. doi: 10.1093/ptj/64.1.35
24. Sugishita M, Hemmi I, Takeuchi T. Reexamination of the validity and reliability of the Japanese version of the mini-mental state examination (mmse-J). Jpn J Cogn Neurosci. (2016) 18(3+4):168–83. doi: 10.11253/ninchishinkeikagaku.18.168
25. Klassen TD, Semrau JA, Dukelow SP, Bayley MT, Hill MD, Eng JJ. Consumer-based physical activity monitor as a practical way to measure walking intensity during inpatient stroke rehabilitation. Stroke. (2017) 48(9):2614–7. doi: 10.1161/strokeaha.117.018175
26. Bezuidenhout L, Thurston C, Hagströmer M, Moulaee Conradsson D. Validity of hip and ankle worn actigraph accelerometers for measuring steps as a function of gait speed during steady state walking and continuous turning. Sensors. (2021) 21(9):3154. doi: 10.3390/s21093154
27. Klassen TD, Simpson LA, Lim SB, Louie DR, Parappilly B, Sakakibara BM, et al. “Stepping up” activity poststroke: ankle-positioned accelerometer can accurately record steps during slow walking. Phys Ther. (2016) 96(3):355–60. doi: 10.2522/ptj.20140611
28. Scobbie L, Dixon D, Wyke S. Goal setting and action planning in the rehabilitation setting: development of a theoretically informed practice framework. Clin Rehabil. (2011) 25(5):468–82. doi: 10.1177/0269215510389198
29. Locke EA, Latham GP. Building a practically useful theory of goal setting and task motivation. A 35-year odyssey. Am Psychol. (2002) 57(9):705–17. doi: 10.1037//0003-066x.57.9.705
30. Dekker J, de Groot V, Ter Steeg AM, Vloothuis J, Holla J, Collette E, et al. Setting meaningful goals in rehabilitation: rationale and practical tool. Clin Rehabil. (2020) 34(1):3–12. doi: 10.1177/0269215519876299
31. Jones CJ, Rikli RE, Beam WC. A 30-S chair-stand test as a measure of lower body strength in community-residing older adults. Res Q Exerc Sport. (1999) 70(2):113–9. doi: 10.1080/02701367.1999.10608028
32. Berg KO, Wood-Dauphinee SL, Williams JI, Maki B. Measuring balance in the elderly: validation of an instrument. Can J Public Health. (1992) 83(Suppl 2):S7–S11. PMID: 1468055
33. Podsiadlo D, Richardson S. The timed “up & go": a test of basic functional mobility for frail elderly persons. J Am Geriatr Soc. (1991) 39(2):142–8. doi: 10.1111/j.1532-5415.1991.tb01616.x
34. Julious SA. Sample size of 12 per group rule of thumb for a pilot study. Pharm Stat. (2005) 4(4):287–91. doi: 10.1002/pst.185
35. Teranishi T, Kondo I, Tanino G, Miyasaka H, Sakurai H, Kaga J, et al. An analysis of falls occurring in a convalescence rehabilitation ward—a decision tree classification of fall cases for the management of basic movements. Jpn J Compr Rehabil Sci. (2013) 4:7–13. doi: 10.11336/jjcrs.4.7
36. Suzuki T, Sonoda S, Misawa K, Saitoh E, Shimizu Y, Kotake T. Incidence and consequence of falls in inpatient rehabilitation of stroke patients. Exp Aging Res. (2005) 31(4):457–69. doi: 10.1080/03610730500206881
37. Yamada R, Shimizu S, Suzuki Y, Nakachi Y, Takemura N, Taira K, et al. Factors related to daily step counts of stroke patients during hospitalization in a convalescent rehabilitation ward. J Stroke Cerebrovasc Dis. (2022) 31(5):106398. doi: 10.1016/j.jstrokecerebrovasdis.2022.106398
38. Waters L, Reeves M, Fjeldsoe B, Eakin E. Control group improvements in physical activity intervention trials and possible explanatory factors: a systematic review. J Phys Act Health. (2012) 9(6):884–95. doi: 10.1123/jpah.9.6.884
39. Renner KE, Williams DSB, Queen RM. The reliability and validity of the Loadsol(®) under various walking and running conditions. Sensors. (2019) 19(2):265. doi: 10.3390/s19020265
40. Ziagkas E, Loukovitis A, Zekakos DX, Chau TD, Petrelis A, Grouios G. A novel tool for gait analysis: validation study of the smart insole Podosmart(®). Sensors. (2021) 21(17):5972. doi: 10.3390/s21175972
41. Wilcox S, Dowda M, Dunn A, Ory MG, Rheaume C, King AC. Predictors of increased physical activity in the active for life program. Prev Chronic Dis. (2009) 6(1):A25. https://www.cdc.gov/pcd/issues/2009/jan/07_0244.htm19080031
Keywords: ambulatory monitoring, wearable electronic devices, fitness trackers, physical activity, gait dysfunction, randomized controlled trials
Citation: Otaka E, Oguchi K, Yagihashi K, Hoshino T, Munakata S, Hayakawa A and Otaka Y (2023) Feasibility and efficacy of an activity-monitoring approach using pedometer in patients undergoing subacute rehabilitation: A pilot study. Front. Rehabil. Sci. 4:1050638. doi: 10.3389/fresc.2023.1050638
Received: 25 September 2022; Accepted: 6 March 2023;
Published: 22 March 2023.
Edited by:
Peter Feys, University of Hasselt, BelgiumReviewed by:
Toru Ogata, The University of Tokyo, JapanColin K. Drummond, Case Western Reserve University, United States
Sabato Mellone, University of Bologna, Italy
© 2023 Otaka, Oguchi, Yagihashi, Hoshino, Munakata, Hayakawa and Otaka. This is an open-access article distributed under the terms of the Creative Commons Attribution License (CC BY). The use, distribution or reproduction in other forums is permitted, provided the original author(s) and the copyright owner(s) are credited and that the original publication in this journal is cited, in accordance with accepted academic practice. No use, distribution or reproduction is permitted which does not comply with these terms.
*Correspondence: Yohei Otaka b3Rha2ExMTlAbWFjLmNvbQ==
Specialty Section: This article was submitted to Strengthening Rehabilitation in Health Systems, a section of the journal Frontiers in Rehabilitation Sciences