- 1Department of Landscape Architecture, University of Sheffield, Sheffield, United Kingdom
- 2St Andrews Botanic Garden, St Andrews, United Kingdom
- 3Bio-Integrated Design Lab, Bartlett School of Architecture, London, United Kingdom
- 4University Centre Myerscough, Preston, United Kingdom
- 5Lancaster Environment Centre, Lancaster University, Lancaster, United Kingdom
- 6SLU Alnarp, Alnarp, Sweden
- 7Gothenburg Botanical Garden, Gothenburg, Sweden
- 8Gothenburg Global Biodiversity Centre, Gothenburg, Sweden
Urban forests in northern Europe are threatened by climate change and biosecurity risks, and in response, city planners are urged to select a wider portfolio of tree species to mitigate the risks of species die-off. However, selecting the right species is a challenge, as most guidance available to specifiers focuses on ecosystem service delivery rather than the information most critical to tree establishment: the ability of a species to tolerate the stresses found in a given place. In this paper, we investigate the potential of using ecological techniques to describe ecological traits at the level of species selection, and the potential of functional ecology theories to identify species that are not widely discussed or specified at present but might be suitable. We collected trait data on 167 tree species across 37 genera, including 38 species within a case study genus, Magnolia L., and tested four theories that posit ways in which traits trade off against each other in predictable ways. We found that at this scale, most species recommended for urban forestry tend to be ordinated along an axis of variation describing pace of life and stress tolerance, and that most Magnolia species are described as being fast-growing rather than stress-tolerant, although there is a degree of inter-specific variation. Further, we found that only one theory offers a succinct and reliable way of describing physiological strategies but translating ecological theory into a form appropriate for urban forestry will require further work.
Introduction
It is widely acknowledged that urban forestry's challenge of optimising the fit between a tree and a site is likely to become increasingly difficult (Conway and Vander Vecht, 2015; Esperon-Rodriguez et al., 2019; Núñez-Florez et al., 2019) as the rate and extent of global urban tree habitat decline is likely to be uneven under climate change (Burley et al., 2019). To address this challenge, growing bodies of ecological and arboriculture literature identify different causes and types of environmental stress (Sack and Buckley, 2020; Mertens et al., 2021), accompanied by an increasing understanding of the physiological responses to these different stresses (Morgenroth et al., 2016). However, a substantial gap exists between our understanding of these stress-response processes and the methods that urban foresters use to apply this knowledge when it comes to selecting species for urban environments. In this paper, we attempt to close this gap by exploring the potential of ecological trait-based theories to improve species selection in urban environments.
Ecological traits are plant characteristics that fulfil functional purposes (functional traits) or describe ontological stages (life history traits) and are used for a wide range of purposes, including assessments of plant development, ecosystem services, and interactions between plant individuals and communities. Large bodies of literature have grown around both schools of trait ecology (Franco and Silvertown, 1996; Salguero-Gómez et al., 2015; Díaz et al., 2016; Kunstler et al., 2016) and the links between them are currently being explored (Adler et al., 2014; Struckman et al., 2019) but common to both are quantitative methods for describing plant functioning and the interactions between an individual and a community or a site. Recent research has shown that reproductive trait trade-offs scale from cell to population (English and Bonsall, 2019), strengthening the argument that relatively simple physiological processes can be used to describe complex strategies for resource allocation and community dynamics. As such, these fields hold significant potential for urban forestry, not only regarding species selection but also for improving many other aspects of urban forest management such as the quantification of ecosystem service provision or preparing management plans.
A central tenet of trait-based theory is that plants are constrained from performing alternative functions simultaneously (Law, 1979; Lavorel et al., 2007; Funk et al., 2017), such that they are traded-off against each other in predictable ways. For example, isohydric and anisohydric physiologies represent two viable but alternative strategies for a species to persist in stressful environments (Skelton et al., 2015) by either avoiding or tolerating drought. Building on this, broad principles for the coordination of traits within an individual or community have been developed, with numerous hypotheses proposed that describe strategies for coordinating resource allocation within a plant (Schimper, 1898; Herms and Mattson, 1992; Gitay and Noble, 1997; Westoby, 1998), structuring community dynamics (MacArthur and Wilson, 2001; Franco and Silvertown, 1996), and linking these processes to environmental variability (Grime, 2001; Southwood, 1988). Today, trait-based approaches are becoming increasingly made use of with functional traits being used to rank or ordinate species within schemes across international boundaries (Cerabolini et al., 2010) or map community successional stages (Chai et al., 2016). A major advance by Díaz et al. (2016) identified six key traits that explain the majority of variation in plant form and function, of which plant height and seed mass were among the most significant. This study was seminal in that it covered a global range of herbaceous and woody plants, identifying that some traits (such as Specific Stem Density and Leaf Mass per Area) are decoupled from height or size, showing instead that these traits describe the metabolic pace of an organism. Moreover, the functional hotspots identified in this study show that most plants occupy intermediate positions along trade-off boundaries, with relatively few species occupying extreme positions of variation.
The potential for using traits to understand global trends in plant ecology is increasingly supported through new capabilities in data sharing (Kattge et al., 2020), statistical analysis and computation power (Moles, 2018) alongside genetic studies that map traits to stress tolerance (González-Martínez et al., 2008). Traditionally, trait data have been used by ecologists to assess habitat changes, plant responses to climate change or the delivery of ecosystem services (Asner et al., 2016), or by researchers to identify superior phenotypes in forestry and cereal crops. However, methodological advances (Moreno-Martínez et al., 2018; Verbeeck et al., 2019) open new opportunities for researchers to collaborate across disciplines and identify new applications for this knowledge.
Nevertheless, there remain major barriers that need to be overcome in order to apply trait-based strategies to designed landscapes such as the urban forest. Firstly, finding global support for functional strategies remains elusive, with ongoing disagreements over fundamental issues such as what a trait is, for example (Walker et al., 2017) or the scale at which they operate (Messier et al., 2017). Even traits that are well-recognised, such as Specific Leaf Area (SLA) present paradoxes in that they can be highly plastic but nevertheless repeatedly trade off against or correlate with other traits (such as Relative Growth Rate or Leaf Lifespan) (Sancho-Knapik et al., 2020) and explain fundamental dimensions of plant variation (Pierce et al., 2013). Further, traits appear to vary in the extent to which they are plastic or under genetic control (Saccone et al., 2017; Walker et al., 2017), whilst others have been shown to vary in significance over time (Gray et al., 2019) leading to changing relationships between traits as plants age (Gibert et al., 2016; Falster et al., 2018). These conceptual challenges are compounded by the characteristics of data that are used to develop theoretical advances: whilst major databases are being developed (Kattge et al., 2011, 2020; Salguero-Gómez et al., 2015; Maitner et al., 2018) that can advance macro-ecological questions, data are patchy in terms of species covered and traits measured, making it difficult to find sufficient traits for a species in order to ordinate them within a scheme. Further, inconsistent recording methodologies are used in spite of accepted protocols (Pérez-Harguindeguy et al., 2013), limiting the confidence with which we can interpret the results.
Alongside the barriers presented by working with an emerging theoretical field, the silo thinking that exists within urban forestry research needs to be overcome. Practitioners still place considerable emphasis on the heuristic literature (i.e., based on experience rather than standardised trials per Watkins et al., 2020), resulting in a slow feedback loop between research and practise, with tree nurseries yet to report changing sales patterns in spite of recent advances in the scope and focus of species selection guidance (e.g., Hirons and Sjöman, 2018). Where attempts have been made to use Plant Functional Types (PFT) in urban forestry, these have predominantly used traits that describe hypothetical ecosystem service delivery (Baraldi et al., 2019; Núñez-Florez et al., 2019) or include aesthetic criteria (Conway and Vander Vecht, 2015; Goodness et al., 2016; Goodness, 2018) rather than the fundamental ability of a tree to persist in an environment, which is the prerequisite for any other values that a tree might provide once it is mature. In this study, we seek to overcome this barrier by exploring whether quantitative, trait-based approaches can be used to identify potentially appropriate species for urban forestry by providing predictions of the fit between a tree and a planted environment. To do this, we evaluate four widely-discussed theories of functional ecology that describe how different traits are integrated using a large dataset, covering species that are currently used in north European urban forestry or recommended for this purpose as well as a case study genus that is not well-understood in this context. As a case study, we selected Magnolia L. as it has a wide distribution across a range of geographic and climatic zones and in theory, should display a range of adaptations. Finally, we use the findings of this research to propose a simple scheme for integrating PFTs and functional traits in urban forestry.
Materials and Methods
Experiment Design
When it comes to applying ecological theory, one of the differences between urban forestry and the fields of restoration ecology or eco-evo where much of this theory originates, us that urban forestry is more concerned with the fit between a plant and a site and characteristics such as stress tolerance rather than other processes such as reproduction, or population dynamics. As such, we focus on aspects of plant functioning relating to how quickly a tree might establish and the ability to tolerate stresses arising from nutrient, oxygen or water deprivation. Whilst there is general agreement over the conceptual premise that the traits that reflect the pace of growth are traded off against plant size and the “investment” of resources in producing tissue (Wright et al., 2004; Salguero-Gómez et al., 2016), a consensus has yet to be reached over which of several competing theories is most effective at describing this trade-off and identifying species that might then be appropriate for species selection. To explore which (if any) theories might be most appropriate for use in urban forestry, four of the most widely discussed theories were studied (Figure 1): Universal Adaptive Theory, originally proposed by Grime (2001) as CSR theory and expanded in Grime and Pierce (2012), the Leaf-Height-Seed Scheme proposed by Westoby (1998) in response to Grime's CSR theory, the Fast-Slow spectrum formalised by Reich (2014) and the Global spectrum of plant form and function (Díaz et al., 2016).
Each of these theories aims to explain trait relationships that scale from the level of the organ to the whole plant and in turn to population and ecosystem, with the promise of providing elegant insights into complex functional relationships using simple, easy-to-measure traits. As can be seen in Table 1, the theories deploy similar traits: most rely upon a trade-offs between “soft” traits (e.g., Leaf area, per Hodgson et al., 1999), with only the Fast-Slow spectrum incorporating hard traits, and the varying emphasis on relationships between them and the ways in which they are correlated. Note that hereafter, the following abbreviations are used to identify traits: LA (Leaf Area), LDMC (Leaf Dry Matter Content), SLA (Specific Leaf Area), SSD (Specific Stem Density), WP-TLP (Leaf Water Potential at turgor loss-point), SM (Seed Mass), and H (Height). In this experiment, we collected trait data for the traits above with the aim of testing the extent to which (a) these relationships hold true at this scale and (b) they can be used to inform species selection. As such, a successful scheme would be based on strong and significant relationships and also provide an interpretable framework for ordinating species.
Species Selection
An increasing diversity of species are proposed for use in urban forestry in response to existential threats such as biosecurity risks and climate change (Kim et al., 2019; Hanley et al., 2021). To test whether trait schemes can aid selection of novel species or identify species that are currently used but that are likely to be inappropriate under climate change, we selected species that are discussed in current industry guidance (see Supplementary Table 1) and used a case study genus (Magnolia L.) to explore the potential of different schemes to identify species (shown in Table 2) that would be appropriate for different urban forestry purposes such as street trees, parks, or swales.
Magnolia L. is a flagship genus with active programmes in conservation and horticulture but is largely unused in high stress environments such as streets or urban podium planting, presenting opportunities for urban forestry to diversify the range of species that are currently used. In spite of its reputation of being non-robust and drought-prone, Magnolia L. is a potentially interesting genus, partly due to its high ornamental qualities but also due to the wide range of genotypic variation and the wide range of environmental niches it occupies, as shown in Table 2. Further, the ornamental qualities of Magnolia L. offer an opportunity to address concerns that the aesthetically striking trees that are preferred by residents do not generally possess the traits needed to survive in harsh environments (Pataki et al., 2013; Conway, 2016).
Data Collection
A number of sources were needed to collect sufficient trait data for this research. The TRY database (Kattge et al., 2020) and unpublished data from Hirons and Sjoman provided the baseline data, complemented by experimental research on genus Magnolia L. collected in the summers of 2017 and 2018, as shown in Table 3. A request was submitted to TRY for the traits in Table 1 across all the species listed in Supplementary Table 1. These data were filtered to remove juvenile plants (Data ID 413), non-healthy plants (Data ID 1961), records with a high error risk (>3) or those that were too small (H <5 m).
This research highlights the logistical challenges of evaluating novel species for urban forestry as many of the species in Table 2 are found only in specialist collections in northern Europe, where restrictions exist over the type of trait data (e.g., SSD) that collection managers permitted to be collected.
Magnolia trait data were collected in the summers of 2017 and 2018, using sites listed in Table 3. Mature, healthy trees were identified, aiming to select six individuals of each species, although in some cases this was not possible. Cultivars were not included, as this study was designed to test inter-specific trait relationships and these selections were not considered to be representative as they are generally selected for outstanding horticultural qualities. Standard protocols were used to collect trait data (Pérez-Harguindeguy et al., 2013).
Analysis
Statistical analysis was carried out using MS Excel v16.30 and R Studio v3.6.3. Data were checked for normality, and LA and SM data were log transformed. The Pearson correlation coefficient was calculated for each trait relationship and trait distributions were summarised in a matrix using the R packages Hmisc, ggplot2, GGally, and plyr (Harrell, 2020; Schloerke et al., 2020; Wickham, 2020; Wickham et al., 2020).
Using the trait data, species were ordinated within plant functional type schemes using ggplot2 and ggtern (Hamilton and Ferry, 2018). For CSR, the methodology of Pierce et al. (2013) was preferred to that of Hodgson et al. (1999) as it reduces the reliance upon artificial trait categories and can be rapidly calculated using basic morpho-physiological traits. Further, the methodology has been shown to be reliable through replication by Li and Shipley (2017) and can be applied to all vascular plants, rather than merely herbaceous species. CSR ternary plots were created using the C, S, and R values from the StrateFy tool (Pierce et al., 2017). To ordinate species within the Leaf-Height-Seed scheme, species were plotted in a 3 dimensional scatterplot as per Westoby's methodology (Westoby, 1998). Reich's Fast-Slow Spectrum hypothesis (Reich, 2014) does not identify a methodology for ordinating species along the Fast-Slow Spectrum but based on the central hypotheses that (a) being fast at any organ-level at acquiring carbon, nutrients, or water requires being fast for the other resources at the same organ-level and (b) that being fast for resources at any one organ level requires being fast for all other resources at the other organs, two dimensional scatter plots were drawn for the two trait relationships hypothesised in Reich's Hypothesis 1a (the coordination of Carbon and Water within the leaf) and Hypothesis 1i (the coordination of carbon between leaf and stem) and the trait relationships identified in GSPFF.
Finally, a table of trait relationships, their Pearson coefficients of correlation and significance was created and ranked according to significance.
Results
Summary
A dataset was built comprising 1,922 Magnolia trait records gathered specifically for this study, 1,309 records of Leaf water potential at turgor loss point from the unpublished Hirons and Sjoman dataset and 26,643 trait records from TRY v6.0 (Kattge et al., 2020), of which 17,570 met the criteria set out above. There were 21 possible trait combinations of the 7 traits in this study, of which 10 show significance at p < 0.05 (Table 4). Four of these 10 significant relationships represent the same axis of variation (cost of investment in tissue) and are not part of any of the trait schemes considered within this study. Of the 10 trait combinations that were significant, only 2 of the trait combinations (LDMC-SLA and LDMC-SSD) displayed strong trade-offs (r >0.5 or < -0.5), with the relationship between LDMC-SLA supporting Grime and Pierce's hypothesis that the relationship between investment in tissue and metabolic pace represents a fundamental trade-off. The strongly significant positive correlation between LDMC-SSD is not formally discussed by any of the four trait schemes, although this result does support a central tenet of Reich's formulation of the Fast-Slow Spectrum that investment in tissue is controlled at a whole-plant level and is consistent across organs.
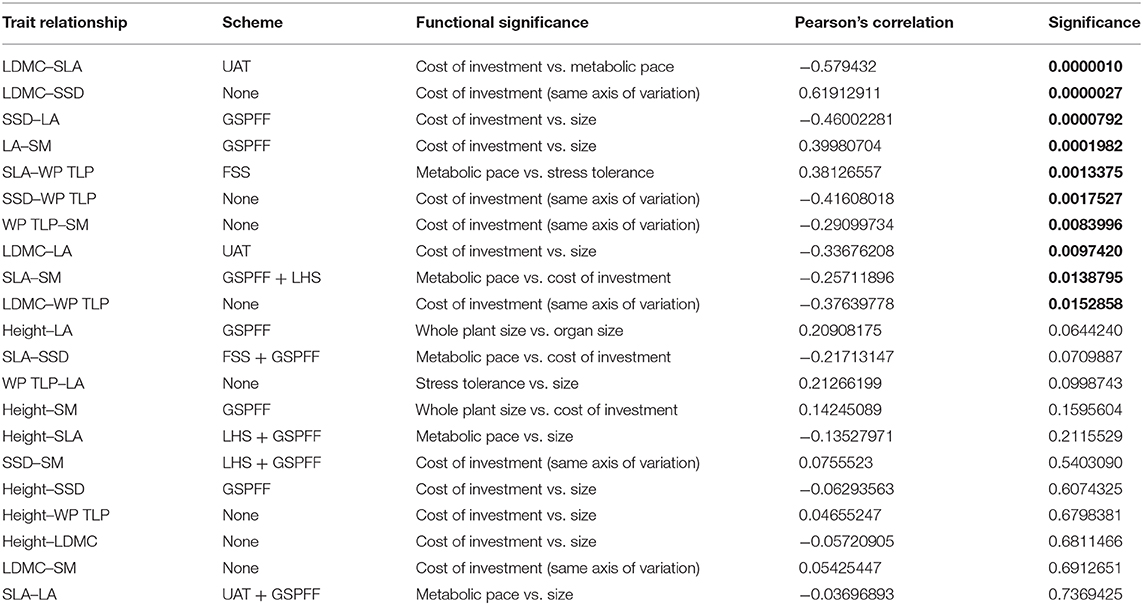
Table 4. Significance and strength of correlation between functional traits (significant relationships shown in bold).
The strength and significance of these trait relationships are summarised in Figure 2, which distinguishes the case study genus, Magnolia, from the species that are more commonly used in northern European urban forestry. Figure 2 suggests that it is possible to make generalisations about Magnolia but not necessarily in ways that support heuristic or cultural assumptions (Watkins et al., 2020): for example, in relation to the other species in this study, the genus tends to have relatively heavy seeds, low density woody tissue, and relatively low resistance to cavity embolism but on the other hand, it has other leaf traits that are comparable to many species that are already used in urban forestry, suggesting that whilst some species in the genus may be more susceptible to stresses such as drought, they may also possess traits that allow them to establish quickly in the right conditions. Further, the range of trait values found in genus Magnolia appears to support biogeographical assessment of the wide range of environmental niches that the genus is distributed across (Table 2).
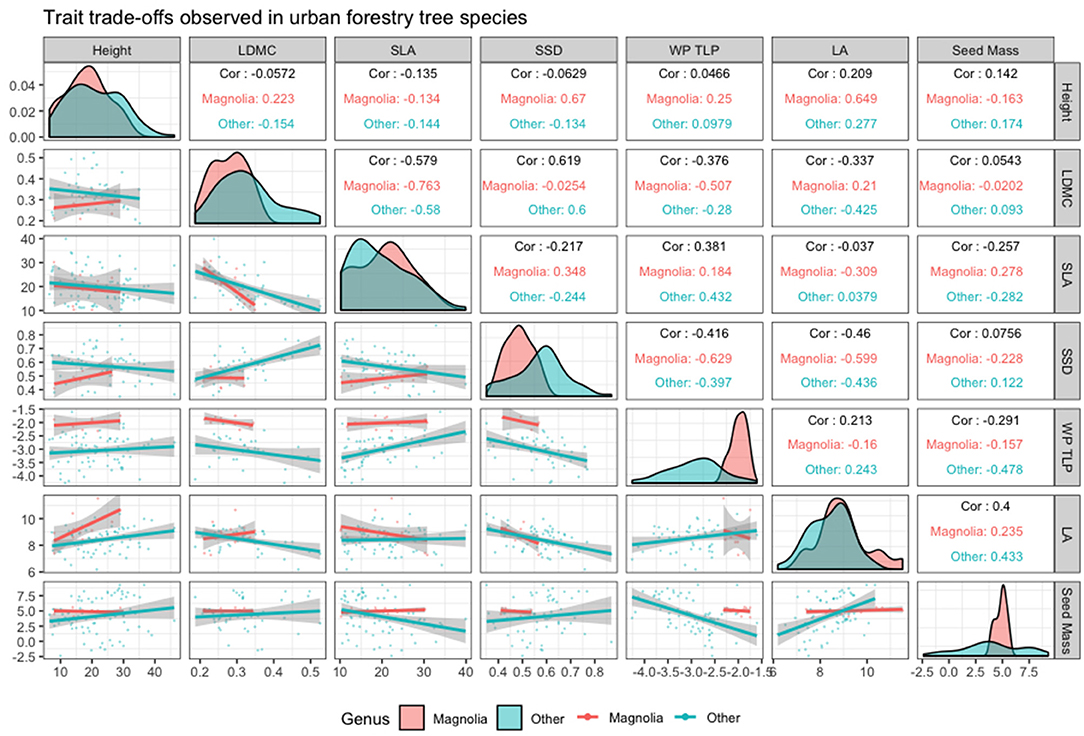
Figure 2. Summary of relationships between functional traits and their Pearson correlation coefficient.
Fast Slow Spectrum
This study evaluated two of the hypotheses within the Fast Slow Spectrum, assessing the balance of resources within a single organ (Reich's Hypothesis 1a) and the coordination of resources between organs (Hypothesis 1i). SLA plays an important role in Reich's hypotheses and the wide range of values for SLA found in Magnolia suggests that using relatively plastic traits (such as SLA) in a paired study presents issues at this scale as it is possible for non-representative data to distort findings. Whilst the relationship between traits within a single organ (H1a, Figure 3A) may be significant, this relationship is weak and especially so in the case of genus Magnolia. We found a weak and non-significant relationship between SLA and SSD, showing that whilst Magnolia traits support Reich's hypothesis (H1i) that being “fast” for one trait requires being fast for others, this does not hold for the other species within this study, as shown in Figure 3B.
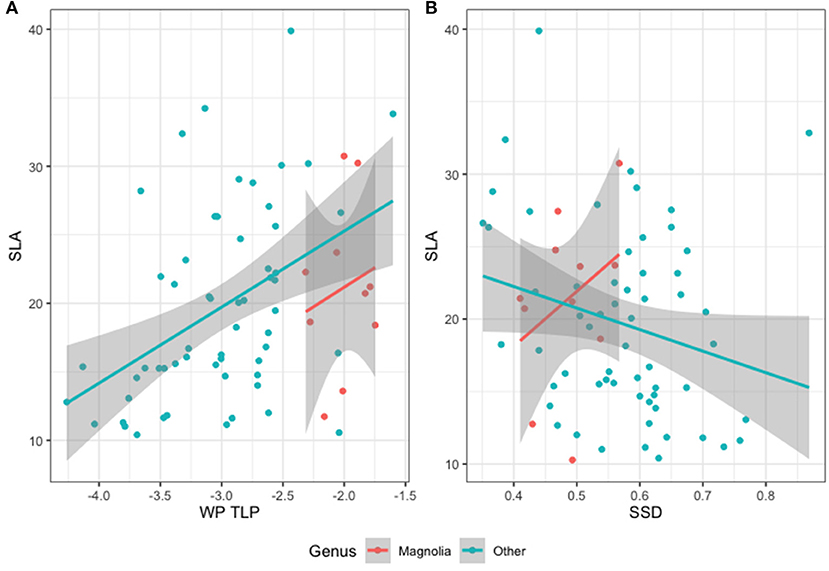
Figure 3. Species ordinated within trait relationships hypothesises H1a and H1i by Reich (2014). (A) SLA-WP TLP and (B) SLA-SSD.
Global Spectrum of Plant Form and Function
The Global Spectrum of Plant Form and Function identifies six traits that “capture the essence of plant form and function” (Díaz et al., 2016). The five soft traits that are used in other theories were measured in this study and of the 10 possible trait combinations, only three were significant (p < 0.5): LA-SM, LA-SSD, and SM-SLA. None of these three were strongly correlated (r < 0.5 or r > −0.5), and strongest correlations were between LA-SSD (r = −0.46, Figure 4C), and LA-SM (r = 0.39). Correlations for SSD-H (Figure 4A), SLA-SM (Figure 4B), and SLA-LA (Figure 4D) replicate the findings of Figure 3B, where data for Magnolia supports the hypothesised trade-offs, whilst the correlation between these traits in other species were in the opposite direction to that which was found in the global study by Diaz et al.
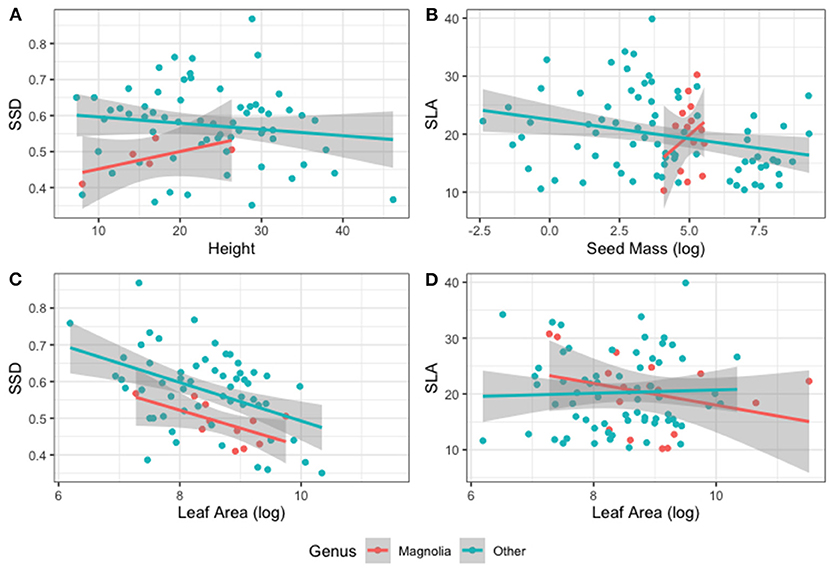
Figure 4. Four dimensions of the global spectrum of plant form and function. (A) SSD-Height, (B) SLA-Seed mass (log), (C) SSD-Leaf area (log), and (D) SLA-Leaf area (log).
Leaf Height Seed Scheme
The Leaf Height Seed scheme was proposed in response to Grime's CSR theory, with a number of points of difference that allowed for replicable studies that use functional traits in a consistent manner to ordinate species. Crucial differences include the delimited, unconstrained axes in three dimensional space (as opposed to a ternary plot, as shown in Figure 5) and using traits to explicitly relate to functional characteristics, with SLA capturing the ability of species to respond to opportunities for rapid growth and H and SM reflecting species ability to respond to the effects of resource-depletion through competition by investing in stature and reproductive traits. In this study, none of the relationships between these three traits were strong: the strongest relationship was also non-significant (SM-SLA: r = −0.25, p = 0.13), with the other two trait combinations decoupled and not significant (SM-H and SLA-H, p > 0.05).
Universal Adaptive Theory
The Universal Adaptive Theory (Grime and Pierce, 2012) is the most advanced iteration of the CSR hypothesis, extending the principles to all forms of life across trophic boundaries, rather than just vascular plants. In Grime's original formulation of CSR (Grime, 2001), three strategies are identified that allow organisms to respond to stress and disturbance, with organisms displaying each of these three strategies to different extents but always in ways that are constrained, hence the use of the ternary plot with extreme positions of C, S, and R in each corner. In this study, we used the methodology developed by Pierce et al. (2013, 2017) on the basis that it allows for species to be ordinated within CSR space in a manner that is replicable and uses only “soft” traits that can be measured within a single leaf. This study found that two of the three trait combinations were found to be significant (SLA-LDMC and LDMC-LA, p < 0.05), with the relationship between SLA-LDMC being the strongest of all trait combinations studied in this research (r = −0.57) and most significant (p = 0.000001). Within the ternary plot in Figure 6, most of the species studied are shown to be stress-tolerant generalists whilst Magnolia species tend to be competitive generalists, broadly in line with the conditions found within their natural ranges.
Discussion
Interpretation of the Ordinations Within Functional Trait Schemes
Whilst the individual trait data reflect or mediate specialised evolutionary adaptions within a specific organ and allow comparisons to be made between species, three key findings can be reported from the review of the different trait-based schemes when applied to urban forestry species selection. Firstly, our hypothesised trade-off between pace of growth and stress tolerance was identified in some of the schemes in this study, but at the scale of this study, only one of the 21 relationships employed by the theories studied was both strong and significant (LDMC-SLA). Secondly, it is clear that whilst specific characteristics can be inferred from a single trait (Blanuša et al., 2020), a single trait alone cannot be used to guide specification of trees within urban forests, not only because traits need to be understood in the context of other traits and whole-plant functioning (Violle, 2007) but also because urban forests are highly heterogenous stress environments, with sites placing specific constraints on an organism that affect fitness. For this reason, the case for finding an effective suite of traits to measure or a scheme that explains the coordination of these traits is strengthened.
Thirdly, it appears that some trait schemes are more suited to some purposes than others, suggesting that alongside the mantra of “the right tree for the right place,” urban foresters need to use the right scheme for the right scale. The Fast-Slow Spectrum and Global Spectrum of Plant Form and Functioning are compelling hypotheses that are well-supported at macro-ecological scales but it is not clear to what extent these are confounded by abiotic influences on plant form at a local scale. The results of this study show that the trait relationships that these schemes are based upon are not sufficiently precise for urban foresters engaging in species selection. In this review, we can see that only 10 trait combinations are statistically significant and that of these, the C-S axis of the UAT is able to explain the most meaningful degree of variation.
The Fast-Slow Spectrum is attractive due to its simplicity: the hypothesis that if a plant is fast for one trait, it will by necessity be fast for all, has general support for the integration of hydraulic traits with carbon and nutrient cycling found by Jin et al. (2016) and extends the widely tested Leaf Economic Spectrum (Wright et al., 2004). However, correlation strength between pairs of leaf traits constrains but does not predict whether the traits respond similarly to different drivers of variation (Messier et al., 2017) and in a study of the causes of trait variability, Laughlin (2014) found that leaf and stem traits were the most consistently meaningful, with seed traits and height less consistent. Further, the lack of consistency in findings across the literature in correlations between SSD and other functional traits is counter to the hypothesis that SSD is part of a fast-slow spectrum (Hypotheses 1c, d, i, and j in Reich, 2014) and suggests that we still have much to learn about stem traits and their roles in plant ecology. For this reason, the caution advised by Rosas et al. (2019) when interpreting comparatively simple trait covariation structures that are revealed at global scales should similarly be applied when using the Global Spectrum of Plant Form and Function.
Whereas, the schemes above hypothesise that one or two axes are sufficient to explain a large proportion of variation in plant form, Westoby's LHS scheme uses three, thereby potentially increasing the potential for statistically meaningful results, irrespective of the meaningfulness of the traits themselves. When each possible combination of trait relationships within this scheme is tested separately (i.e., H-SLA, SLA-SM, SM-H) it can be seen that the clustering indicated by this study provides a reasonable picture of plant functioning but is not as precise or accurate as it could be if other traits were used. Nevertheless, the LHS scheme should be interpreted within the context within which it was created, in that it was proposed as an advance on CSR theory in two ways: firstly, it did not rely upon a priori categories and secondly, it ordinated species within unconstrained axes, thereby allowing more meaningful comparisons of different plant life forms. Whilst there remain drawbacks to the scheme (such as the impossibility of ordinating non-seed-bearing plants), the ability to use unconstrained axes in three-dimensional space is a powerful conceptual advantage, even if it is difficult to read the graph itself.
It is partly in response to Westoby's LHS scheme that Pierce et al. (2013) developed their methodology for ordinating plants in CSR. Whereas others (Brzeziecki and Kienast, 1994; Hodgson et al., 1999; Cerabolini et al., 2010) used combinations of ordinal and categorical values to ordinate species in CSR, the major advance in this scheme was to find a way of ordinating plants using traits common to all plants, harnessing the Leaf Economic Spectrum proposed by Wright et al. (2004). Our study suggests that in using the StrateFy calculator proposed by Pierce et al., this scheme presents the best way of representing (if not explaining) variation in plant strategies. There remain substantial aspects that need to be investigated: for example, Liese et al. (2017) found that the Leaf Economic Spectrum is not mirrored below ground, suggesting that the coordinated resource use as predicted by Reich (2014) also needs further testing and refinement. The role of disturbance as a limit to plant growth and its explanation in CSR theory in the context of woody plants is not yet clear: classically, ruderal plants are those that prioritise reproduction and fast growth, and using this scheme, some Magnolia species are ordinated in the same location as generalist herbaceous species. There are many viable traits and strategies for responding to disturbance and we need to ask, how many of these (such as fire, soil disturbance, or grazing) are relevant to urban forestry?
Although the schemes tested in this study reveal some dimensions of trait coordination, there remain aspects that are not captured, some of which could be potentially significant as revealed by traits that the genus Magnolia specialise in. For example, aside from Seed Mass, none of the schemes explicitly account for the costs and benefits of different reproductive strategies. Magnolia species typically do not sexually reproduce until sufficient reserves of non-structural carbohydrates are maintained, as predicted by Grime's competitive strategy, but once this stage is reached, considerable quantities of these reserves are used in floral thermogenesis to facilitate pollination by invertebrates (Wang et al., 2013; Wang and Zhang, 2015). None of the schemes tested in this study, nor any other to our knowledge, offer a way of accounting for the effect processes such as these might have on whole-plant functioning.
Magnolia Traits Suggest Relatively Competitive Strategies
Magnolia species are generally considered to have characteristics that are not particularly well-suited to the harsher environments found in urban forestry, such as moisture stress or reflected heat. The results of the trait-based experiments in this study tend to support this view, with the Leaf Water PotentialTLP study suggesting that most of the species in this study have evolved isohydric strategies that allow populations to escape the effects of stress through fast growth and high investment in reproduction (Bartlett et al., 2016). This perception is supported by findings in other traits, such as SSD and SLA, which indicate strategies in Magnolia species that prioritise the speed of growth over tolerance of stress through relatively light or cheap investment in tissue. However, each of the studies in this experiment indicated that although these Magnolia species are relatively closely related, there was nonetheless a degree of inter-specific variation in trait values, although this feature was more marked in some studies than others, with LA and LDMC showing higher degrees of variation.
The variations in trait values found in this study compared to others within the functional ecology literature raise the question of how much explanatory power each trait presents. In spite of numerous studies including replications, global studies (Bruelheide et al., 2018; Kissling et al., 2018), and even computer game simulations (Barbe et al., 2020), the degrees to which these traits are individually meaningful pose significant conceptual and practical challenges in their interpretation in urban forestry. For example, in traits which have smaller ranges in variation (such as SSD), it is necessary to ask how and why do these traits vary, to what extent are minor differences significant, and what physiological processes are captured in these traits (Onoda et al., 2017)? In the case of SSD, Magnolias are diffuse-porous species, so early and latewood are likely to be differently influenced by ecological factors (Bergès et al., 2008): SSD expresses a range of physiological processes and in itself reflects a series of trade-offs that an organism needs to make, therefore “blunting” its explanatory power.
Similarly, Messier et al. (2017) found that SLA's sensitivity to multiple drivers of variation has the potential to blur interspecific differences unless their effects are taken into account experimentally or statistically. This study also found that although Leaf Nitrogen Content (LNC) is more difficult to capture, LNC is better suited to detecting community-level responses to large-scale environmental gradients such as climate, whilst LDMC is best suited to detecting plastic responses within individuals to micro-environmental gradients. As a result, the study hypothesised that Leaf Lifespan may potentially be a more useful trait than SLA as (a) it is more meaningful and (b) it is under stronger genetic control.
Toward a Functional Trait Scheme for Urban Forestry
Recognising the heterogeneity of stress created by urban environments, dimensionality in plants is a blessing not a curse as it offers us an opportunity to recognise nuance in the variation of plant traits and strategies, and then apply these theories more precisely to decision making. There does however appear to be a tractable upper limit to the dimensionality of plant traits (Laughlin, 2014), suggesting that a parsimonious trait-based scheme may be within reach for urban foresters- the question this then leads to is, which traits are most effective for this purpose? Numerous studies support the argument that although the trend in functional ecology research has been toward soft traits with large explanatory power, other traits that are more difficult to capture may be more meaningful and reveal more precise trade-offs. Drawing on the orthogonal nature of traits revealed in the Principal Components Analysis carried out by Díaz et al. (2016) and work by Baraloto et al. (2010), in Figure 7A we set out a hypothetical relationship between two dimensions of plant functioning and then identify in Figure 7B how this scheme might be used to select species in urban forests.
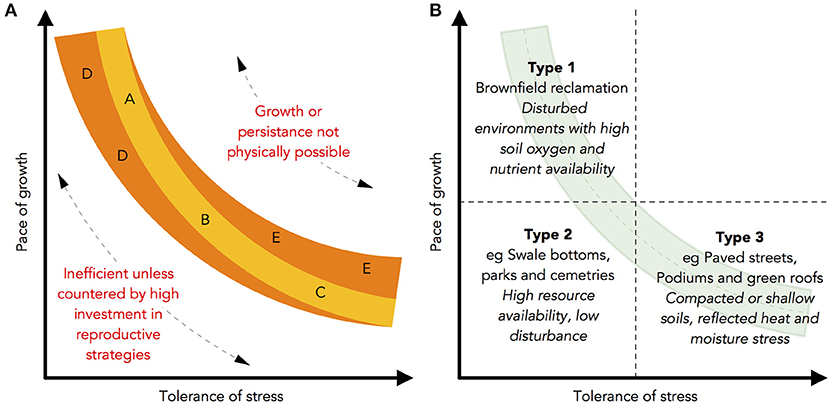
Figure 7. (A,B) Hypothetical trait-based scheme for urban foresters. Building on CSR theory, (A) shows the variety of viable plant strategies in a trade-off between fast growth and high tolerance of stress. Positions A and C equate to different extremes of the trade-offs between competitive-stress tolerant strategies, with position B representing a generalist strategy. A greater investment in reproduction and faster growth is required in disturbed environments, resulting in more ruderal strategies (D), whilst in more stressful situations, delayed sexual maturity allows for greater investment in dense structural and photosynthetic tissues (E). Note that unlike other similar graphs, the trait trade-off is fitted by a quadratic rather than linear line of best fit. (B) Overlays environments found in urban forests upon this model, resulting in a method for best fitting tree species to urban forestry sites.
Figure 8 illustrates how this model could work, using the ordinations from the UAT study. Applying this scheme to the species in this study, it is possible to hypothesise that Magnolia species are more suited to less stressful environments, being better adapted to sites with higher resource availability. The scheme makes it possible to translate the observed inter-specific differences into practical guidance in ways that are not currently captured by the heuristic literature. Whilst quantitative associations between climate and traits were not part of this study, the traits recorded in this study appear to support hypotheses generated by Jardine et al. (2020) and Watkins et al. (2020): for example, in a study of 5 bottomland species, Nash and Graves (1993) found that M. virginiana was well-suited to urban environments with high oxygen and nutrient availability. A wider implication from this scheme is that it appears many of the species currently recommended for urban planting may need to be investigated in greater detail, with comparatively few species appearing to be suitable for planting in stressful, paved environments.
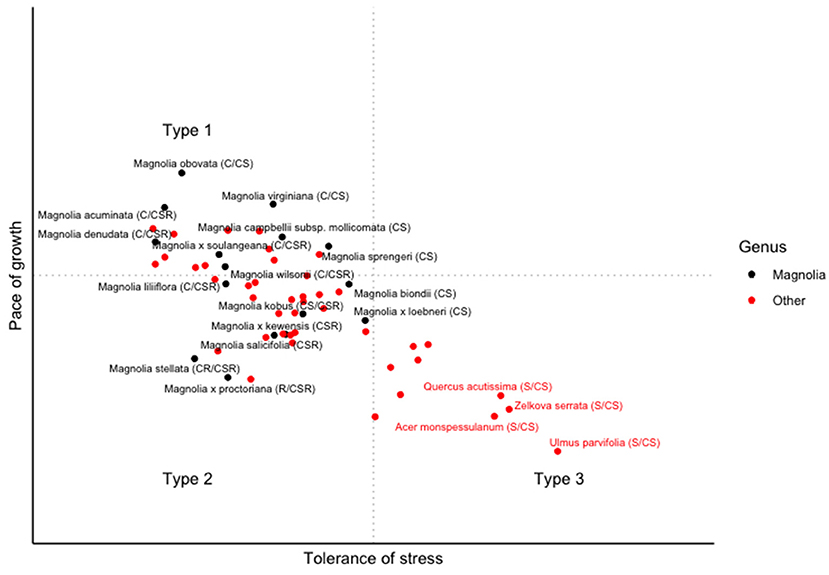
Figure 8. Preliminary ordination of species according to hypothesised urban forestry environments, with Magnolia species UAT ordinations shown in brackets.
To develop this model for species selection in urban forestry, a number of issues need to be resolved. Firstly, counter to other Plant Functional Type proposals in urban forestry, it is necessary to emphasise the importance of ecological criteria (which describe a tree's ability to survive and grow in a given condition) over design criteria (where ecosystem services such as aesthetic values are prioritised). Secondly, from the perspective of data analysis, the number of axes of variation sufficient for this purpose will need to be established and weighed against their ease of interpretation for practitioners. In separate studies, each with their own advantages and constraints, Jin et al. (2016) developed a two axis Fast-Slow Spectrum model for ten woody plants in China whilst Lavorel et al. (2007) propose four axes of variation. As discussed in this paper, Grime and Westoby use different configurations of three axes, but it is possible to identify three strategies using two axes of variation as shown in 7a and by Grime (2001), who originally used 2 axes to create a ternary arrangement. Alongside these conceptual considerations, quadratic (instead of linear) equations should also be explored to identify trade-off boundaries, and recent progress in understand the changing roles played by traits through time should be harnessed to acknowledge the different impacts of biotic and abiotic stress on maturing and senescing trees.
Thirdly, the potential combinations of traits that could be studied show that further correlative studies are unlikely to yield meaningful advances until clear rationales for selecting traits are established and our understanding of their significances are resolved (López et al., 2021): in the age of big data and powerful statistical software, it is increasingly important to emphasise the importance of hypothesis-driven research (Moles, 2018) and incorporating evolutionary adaptions (McGaughran et al., 2021) if industry guidance is to be generated. A periodic table of traits has long been mooted by ecologists (Salguero-Gómez et al., 2016) but remains a distant prospect in spite of recent efforts. In this spirit, we call for an Urban Forestry Periodic Table of Traits that builds on the emerging standardisation of trait definitions (Garnier et al., 2017) and trait-performance studies (Scheres and Van Der Putten, 2017; Boisvert-Marsh et al., 2020; Sack and Buckley, 2020), and that can be tested by researchers to become the foundation for a model of species selection. The Urban Forestry Periodic Table of Traits would assist specifiers and researchers in knowing which traits or axes of variation are relevant in a given context and how they are integrated (Laughlin, 2014; Barry et al., 2019; Mertens et al., 2021), thereby allowing urban foresters to select the right traits for the right place and make informed decisions about the performance of a taxon given certain trait combinations. For example, traits relating to reproduction are of high importance to ecologists but are rarely considered by urban foresters except in the context of the trade-offs that these traits constrain on other traits relating to persistence, stature or growth rate. Similarly, traits such as SSD or WP TLP may be appropriate for assessing suitability to paved sites, for example, where moisture stress is a limiting factor on growth, whilst Leaf N may be more appropriate to consider when designing species mixes so that the role of competition can be factored into a specification.
Conclusion
This study considers the potential of applying ecological trait-based schemes to urban forestry practise and found that whilst there is no single trait that is sufficient for selecting taxa in an urban environment, it may be that combinations of traits hold promise for future development. We used a case study genus (Magnolia L.) and other species used in urban forestry in northern Europe, drawing on experimental research and data derived from global trait databases. We found that Magnolia species tend to prioritise speed of growth over stress tolerance and using a novel scheme, suggest that it is possible to identify inter-specific differences within the genus that make them suitable to sites with high resource provision rather than harsh environments such as paved streets. Using this scheme, we hypothesise that many species currently used (or recommended for use) in urban forestry in northern Europe are more suited to resource-rich sites and that further nuance is needed in industry guidance. It appears that most of the schemes discussed in the ecological literature may be too blunt for the purposes of urban forestry specification as selecting the right combination of traits is a significant challenge, with only one axis of variation in this study showed a strong and significant relationship between the two traits. As such, a periodic table of traits that recognises the demands and scales specific to urban forestry would be a significant development and provide a framework for practitioners to select the right tree for the right place based on the right traits.
Data Availability Statement
The original contributions presented in the study are included in the article/Supplementary Material, further inquiries can be directed to the corresponding author/s.
Author Contributions
HW and JH: research design. HW, AH, and HS: data collection. HW, AH, HS, RC, and JH: data analysis and manuscript drafting. All authors contributed to the article and approved the submitted version.
Funding
Research by HW was funded by a Doctoral Scholarship from the University of Sheffield.
Conflict of Interest
The authors declare that the research was conducted in the absence of any commercial or financial relationships that could be construed as a potential conflict of interest.
Supplementary Material
The Supplementary Material for this article can be found online at: https://www.frontiersin.org/articles/10.3389/frsc.2021.654618/full#supplementary-material
References
Adler, P. B., Salguero-Gómez, R., Compagnoni, A., Hsu, J. S., Ray-Mukherjee, J., Mbeau-Ache, C., et al. (2014). Functional traits explain variation in plant life history strategies. Proc. Natl. Acad. Sci. U.S.A. 111, 740–745. doi: 10.1073/pnas.1410430111
Asner, G. P., Knapp, D. E., Anderson, C. B., Martin, R. E., and Vaughn, N. (2016). Large-scale climatic and geophysical controls on the leaf economics spectrum. Proc. Natl. Acad. Sci. U.S.A. 113, E4043–E4051. doi: 10.1073/pnas.1604863113
Baraldi, R., Chieco, C., Neri, L., Facini, O., Rapparini, F., Morrone, L., et al. (2019). An integrated study on air mitigation potential of urban vegetation: From a multi-trait approach to modeling. Urban For. Urban Green. 41, 127–138. doi: 10.1016/j.ufug.2019.03.020
Baraloto, C., Paine, C. E. T., Poorter, L., Beauchene, J., Bonal, D., Domenach, A. M., et al. (2010). Decoupled leaf and stem economics in rain forest trees. Ecol. Lett. 13, 1338–1347. doi: 10.1111/j.1461-0248.2010.01517.x
Barbe, L., Mony, C., and Abbott, B. W. (2020). Artificial intelligence accidentally learned ecology through video games. Trends Ecol. Evol. 35, 557–560. doi: 10.1016/j.tree.2020.04.006
Barry, K. E., Mommer, L., van Ruijven, J., Wirth, C., Wright, A. J., Bai, Y., et al. (2019). The future of complementarity: disentangling causes from consequences. Trends Ecol. Evol. 34, 167–180. doi: 10.1016/j.tree.2018.10.013
Bartlett, M. K., Klein, T., Jansen, S., Choat, B., and Sack, L. (2016). The correlations and sequence of plant stomatal, hydraulic, and wilting responses to drought. Proc. Natl. Acad. Sci. U.S.A. 113, 13098–13103. doi: 10.1073/pnas.1604088113
Bergès, L., Nepveu, G., and Franc, A. (2008). Effects of ecological factors on radial growth and wood density components of sessile oak (Quercus petraea Liebl.) in Northern France. For. Ecol. Manag. 255, 567–579. doi: 10.1016/j.foreco.2007.09.027
Blanuša, T., Qadir, Z. J., Kaur, A., Hadley, J., and Gush, M. B. (2020). Evaluating the effectiveness of urban hedges as air pollution barriers: Importance of sampling method, species characteristics and site location. Environments MDPI 7, 1–21. doi: 10.3390/environments7100081
Boisvert-Marsh, L., Royer-Tardif, S., Nolet, P., Doyon, F., and Aubin, I. (2020). Using a trait-based approach to compare tree species sensitivity to climate change stressors in eastern Canada and inform adaptation practices. Forests 11:989. doi: 10.3390/f11090989
Bruelheide, H., Dengler, J., Purschke, O., Lenoir, J., Jiménez-Alfaro, B., Hennekens, S. M., et al. (2018). Global trait–environment relationships of plant communities. Nat. Ecol. Evol. 2, 1906–1917. doi: 10.1038/s41559-018-0699-8
Brzeziecki, B., and Kienast, F. (1994). Classifying the life-history strategies of trees on the basis of the Grimian model. For. Ecol. Manage. 69, 167–187. doi: 10.1016/0378-1127(94)90227-5
Burley, H., Beaumont, L. J., Ossola, A., Baumgartner, J. B., Gallagher, R., Laffan, S., et al. (2019). Substantial declines in urban tree habitat predicted under climate change. Sci. Total Environ. 685, 451–462. doi: 10.1016/j.scitotenv.2019.05.287
Cerabolini, B. E. L., Brusa, G., Ceriani, R. M., de Andreis, R., Luzzaro, A., and Pierce, S. (2010). Can CSR classification be generally applied outside Britain? Plant Ecol. 210, 253–261. doi: 10.1007/s11258-010-9753-6
Chai, Y., Yue, M., Wang, M., Xu, J., Liu, X., Zhang, R., et al. (2016). Plant functional traits suggest a change in novel ecological strategies for dominant species in the stages of forest succession. Oecologia 180, 771–783. doi: 10.1007/s00442-015-3483-3
Chen, H., Niklas, K. J., and Sun, S. (2012). Testing the packing rule across the twig-petiole interface of temperate woody species. Trees Struct. Funct. 26, 1737–1745. doi: 10.1007/s00468-012-0742-3
China Expert Workshop (2014). Magnolia decidua. The IUCN Red List of Threatened Species 2014: e.T193923A2290949.
China Expert Workshop (2015). Magnolia wilsonii. The IUCN Red List of Threatened Species 2015: e.T31274A2803140. doi: 10.2305/IUCN.UK.2015-2.RLTS.T31274A2803140.en
Conway, T. M. (2016). Tending their urban forest: residents' motivations for tree planting and removal. Urban For. Urban Green. 17, 23–32. doi: 10.1016/j.ufug.2016.03.008
Conway, T. M., and Vander Vecht, J. (2015). Growing a diverse urban forest: Species selection decisions by practitioners planting and supplying trees. Landsc. Urban Plan. 138, 1–10. doi: 10.1016/j.landurbplan.2015.01.007
Díaz, S., Kattge, J., Cornelissen, J. H. C., Wright, I. J., Lavorel, S., Dray, S., et al. (2016). The global spectrum of plant form and function. Nature 529, 167–171. doi: 10.1038/nature16489
English, S., and Bonsall, M. B. (2019). Physiological dynamics, reproduction-maintenance allocations, and life history evolution. Ecol. Evol. 9, 9312–9323. doi: 10.1002/ece3.5477
Esperon-Rodriguez, M., Power, S. A., Tjoelker, M. G., Beaumont, L. J., Burley, H., Caballero-Rodriguez, D., et al. (2019). Assessing the vulnerability of Australia's urban forests to climate extremes. Plants People Planet 1, 387–397. doi: 10.1002/ppp3.10064
Falster, D. S., Duursma, R. A., and FitzJohn, R. G. (2018). How functional traits influence plant growth and shade tolerance across the life cycle. Proc. Natl. Acad. Sci. U.S.A. 115, E6789–E6798. doi: 10.1073/pnas.1714044115
Franco, M., and Silvertown, J. (1996). Life history variation in plants : An exploration of the fast-slow continuum hypothesis. Philos. Trans. R. Soc. B Biol. Sci. 351, 1341–1348. doi: 10.1098/rstb.1996.0117
Funk, J. L., Larson, J. E., Ames, G. M., Butterfield, B. J., Cavender-Bares, J., Firn, J., et al. (2017). Revisiting the Holy Grail: using plant functional traits to understand ecological processes. Biol. Rev. 92, 1156–1173. doi: 10.1111/brv.12275
Garnier, E., Stahl, U., Laporte, M. A., Kattge, J., Mougenot, I., Kühn, I., et al. (2017). Towards a thesaurus of plant characteristics: an ecological contribution. J. Ecol. 105, 298–309. doi: 10.1111/1365-2745.12698
Gibbs, D., and Wheeler, L. (2014). Magnolia biondii. The IUCN Red List of Threatened Species 2014: e.T193906A2290345.
Gibert, A., Gray, E. F., Westoby, M., Wright, I. J., and Falster, D. S. (2016). On the link between functional traits and growth rate: meta-analysis shows effects change with plant size, as predicted. J. Ecol. 104, 1488–1503. doi: 10.1111/1365-2745.12594
Gitay, H., and Noble, I. R. (1997). “What are functional types and how should we seek them?” in Plant Functional Types: Their Relevance to Ecosystem Properties and Global Change, eds T. M. Smith, H. H. Shughart, and F. I. Woodward (Cambridge: Cambridge University Press).
Global Tree Specialist Group (2014a). Magnolia denudata. The IUCN Red List of Threatened Species 2014: e.T193934A221273.
Global Tree Specialist Group (2014b). Magnolia liliiflora. The IUCN Red List of Threatened Species 2014: e.T191488A1985387. doi: 10.2305/IUCN.UK.2014-3.RLTS.T191488A1985387.en
Global Tree Specialist Group (2014c). Magnolia macrophylla. The IUCN Red List of Threatened Species 2014: e.T6295A3116514.
González-Martínez, S. C., Huber, D., Ersoz, E., Davis, J. M., and Neale, D. B. (2008). Association genetics in Pinus taeda L. II. Carbon isotope discrimination. Heredity 101, 19–26. doi: 10.1038/hdy.2008.21
Goodness, J. (2018). Urban landscaping choices and people's selection of plant traits in Cape Town, South Africa. Environ. Sci. Policy 85, 182–192. doi: 10.1016/j.envsci.2018.02.010
Goodness, J., Andersson, E., Anderson, P. M. L., and Elmqvist, T. (2016). Exploring the links between functional traits and cultural ecosystem services to enhance urban ecosystem management. Ecol. Indic. 70, 597–605. doi: 10.1016/j.ecolind.2016.02.031
Gray, E. F., Wright, I. J., Falster, D. S., Eller, A. S. D., Lehmann, C. E. R., Bradford, M. G., et al. (2019). Leaf:wood allometry and functional traits together explain substantial growth rate variation in rainforest trees. AoB Plants 11, 1–11. doi: 10.1093/aobpla/plz024
Grime, J. P. (2001). Plant Strategies, Vegetation Processes, and Ecosystem Properties, 2nd Edn. Chichester: John Wiley & Sons.
Grime, J. P., and Pierce, S. (2012). The Evolutionary Strategies that Shape Ecosystems. London: John Wiley & Sons, Ltd. doi: 10.1002/9781118223246
Hamilton, N. E., and Ferry, M. (2018). Ggtern: ternary diagrams using ggplot2. J. Stat. Softw. 87:4513. doi: 10.18637/jss.v087.c03
Hanley, P. A., Arndt, S. K., Livesley, S. J., and Szota, C. (2021). Relating the climate envelopes of urban tree species to their drought and thermal tolerance. Science of the Total Environment 753. doi: 10.1016/j.scitotenv.2020.142012
Harrell, F. E. J. (2020). Package “Hmisc.” CRAN. Retrieved from: https://hbiostat.org/R/Hmisc/ (accessed December 18, 2020).
Harvey-Brown, Y. (2019). Magnolia stellata. The IUCN Red List of Threatened Species 2019: e.T194010A152592969.
Herms, D. A., and Mattson, W. J. (1992). The dilemma of plants to grow or defend. Quart. Rev. Biol. 67, 283–335.
Hirons, A., and Sjöman, H. (2018). Tree Species Selection for Green Infrastructure: A Guide for Specifiers, Vol. 1. Tree and Design Action Group.
Hodgson, A. J. G., Wilson, P. J., Hunt, R., Grime, J. P., Thompson, K., Hodgson, J. G., et al. (1999). Allocating C-S-R plant functional types: a soft approach to a hard problem. Oikos 85, 282–294.
Jardine, E. C., Thomas, G. H., Forrestel, E. J., Lehmann, C. E. R., and Osborne, C. P. (2020). The global distribution of grass functional traits within grassy biomes. J. Biogeogr. 47, 553–565. doi: 10.1111/jbi.13764
Jin, Y., Wang, C., Zhou, Z., and Li, Z. (2016). Co-ordinated performance of leaf hydraulics and economics in 10 Chinese temperate tree species. Funct. Plant Biol. 43, 1082–1090. doi: 10.1071/FP16097
Kattge, J., Bönisch, G., Díaz, S., Lavorel, S., Prentice, I. C., Leadley, P., et al. (2020). TRY plant trait database – enhanced coverage and open access. Glob. Chang. Biol. 26, 119–188. doi: 10.1111/gcb.14904
Kattge, J., Díaz, S., Lavorel, S., Prentice, I. C., Leadley, P., Bönisch, G., et al. (2011). TRY - a global database of plant traits. Glob. Chang. Biol. 17, 2905–2935. doi: 10.1111/j.1365-2486.2011.02451.x
Kehla, S. (2014a). Magnolia acuminata. The IUCN Red List of Threatened Species 2014: e.T193896A2289975.
Kehla, S. (2014b). Magnolia campbellii. The IUCN Red List of Threatened Species 2014: e.T193912A2290545.
Kehla, S. (2014c). Magnolia fraseri. The IUCN Red List of Threatened Species 2014: e.T193941A2291599.
Kehla, S. (2014d). Magnolia grandiflora. The IUCN Red List of Threatened Species 2014: e.T193948A2291865.
Kehla, S. (2014g). Magnolia rostrata. The IUCN Red List of Threatened Species 2014: e.T32426A2818758.
Kehla, S. (2014h). Magnolia salicifolia. The IUCN Red List of Threatened Species 2014: e.T193999A2293796.
Kehla, S. (2014i). Magnolia sieboldii. The IUCN Red List of Threatened Species 2014: e.T194003A2293976.
Kehla, S. (2014j). Magnolia sprengeri. The IUCN Red List of Threatened Species 2014: e.T194003A2294150.
Kehla, S. (2014k). Magnolia virginiana. The IUCN Red List of Threatened Species 2014: e.T194018A2294506.
Kehla, S., Wheeler, L., and Rivers, M. C. (2014). Magnolia tripetala. The IUCN Red List of Threatened Species 2014: e.T194013A2294339. doi: 10.2305/IUCN.UK.2014-3.RLTS.T194013A2294339.en
Kim, Y., Park, C., Koo, K. A., Lee, M. K., and Lee, D. K. (2019). Evaluating multiple bioclimatic risks using Bayesian belief network to support urban tree management under climate change. Urban For. Urban Green. 43:126354. doi: 10.1016/j.ufug.2019.05.016
Kissling, W. D., Walls, R., Bowser, A., Jones, M. O., Kattge, J., Agosti, D. P., et al. (2018). Towards global data products of Essential Biodiversity Variables on species traits. Nat. Ecol. Evol. 2, 1531–1540. doi: 10.1038/s41559-018-0667-3
Kunstler, G., Falster, D., Coomes, D. A., Hui, F., Kooyman, R. M., Laughlin, D. C., et al. (2016). Plant functional traits have globally consistent effects on competition. Nature 529, 204–207. doi: 10.1038/nature16476
Laughlin, D. C. (2014). The intrinsic dimensionality of plant traits and its relevance to community assembly. J. Ecol. 102, 186–193. doi: 10.1111/1365-2745.12187
Lavorel, S., Díaz, S., Cornelissen, J. H. C., Garnier, E., Harrison, S. P., McIntyre, S., et al. (2007). “Plant functional types: are we getting any closer to the holy grail?,” in Terrestrial Ecosystems in a Changing World, Global Change — The IGBP Series, eds J. G. Canadell, D. E. Pataki, and L. F. Pitelka (Berlin, Heidelberg: Springer), 149–164. doi: 10.1007/978-3-540-32730-1_13
Law, R. (1979). Optimal life histories under age-specific predation. Am. Soc. Natural. 114, 399–417.
Li, J., Wu, Y., Wu, T., Cao, L., Chen, J., Qiu, Z., et al. (2017). Significance of stump-sprouting for the population size structure and spatial distribution patterns of endangered species, Magnolia Cylindrica. Polish J. Ecol. 65, 247–257. doi: 10.3161/15052249PJE2017.65.2.007
Li, Y., and Shipley, B. (2017). An experimental test of CSR theory using a globally calibrated ordination method. PLoS ONE 2:e0175404. doi: 10.1371/journal.pone.0175404
Liese, R., Alings, K., and Meier, I. C. (2017). Root branching is a leading root trait of the plant economics spectrum in temperate trees. Front. Plant Sci. 8:315. doi: 10.3389/fpls.2017.00315
López, R., Cano, F. J., Martin-StPaul, N. K., Cochard, H., and Choat, B. (2021). Coordination of stem and leaf traits define different strategies to regulate water loss and tolerance ranges to aridity. New Phytol. 497–509. doi: 10.1111/nph.17185
MacArthur, R. H., and Wilson, E. O. (2001). The Theory of Island Biogeography. Princeton, NJ: Princeton University Press.
Maitner, B. S., Boyle, B., Casler, N., Condit, R., Donoghue, J., Durán, S. M., et al. (2018). The bien r package: a tool to access the Botanical Information and Ecology Network (BIEN) database. Methods Ecol. Evol. 9, 373–379. doi: 10.1111/2041-210X.12861
McGaughran, A., Laver, R., and Fraser, C. (2021). Ecology and evolution evolutionary responses to warming. Trends Ecol. Evol. 1–10. doi: 10.1016/j.tree.2021.02.014
Mertens, D., Boege, K., Kessler, A., Koricheva, J., Thaler, J. S., Whiteman, N. K., et al. (2021). Predictability of biotic stress structures plant defence evolution. Trends Ecol. Evol. 1–13. doi: 10.1016/j.tree.2020.12.009
Messier, J., McGill, B. J., Enquist, B. J., and Lechowicz, M. J. (2017). Trait variation and integration across scales: is the leaf economic spectrum present at local scales? Ecography 40, 685–697. doi: 10.1111/ecog.02006
Moles, A. T. (2018). Being John Harper: using evolutionary ideas to improve understanding of global patterns in plant traits. J. Ecol. 106, 1–18. doi: 10.1111/1365-2745.12887
Moreno-Martínez, Á., Camps-Valls, G., Kattge, J., Robinson, N., Reichstein, M., van Bodegom, P., et al. (2018). A methodology to derive global maps of leaf traits using remote sensing and climate data. Remote Sens. Environ. 218, 69–88. doi: 10.1016/j.rse.2018.09.006
Morgenroth, J., Östberg, J., Konijnendijk van den Bosch, C., Nielsen, A. B., Hauer, R., Sjöman, H., et al. (2016). Urban tree diversity-taking stock and looking ahead. Urban For. Urban Green. 15, 1–5. doi: 10.1016/j.ufug.2015.11.003
Nash, L. J., and Graves, W. R. (1993). Drought and flood stress effects on plant development and leaf water relations of five taxa of trees native to bottomland habitats. J. Am. Soc. Hortic. Sci. 118, 845–850. doi: 10.21273/jashs.118.6.845
Núñez-Florez, R., Pérez-Gómez, U., and Fernández-Méndez, F. (2019). Functional diversity criteria for selecting urban trees. Urban For. Urban Green. 38, 251–266. doi: 10.1016/j.ufug.2019.01.005
Onoda, Y., Wright, I. J., Evans, J. R., Hikosaka, K., Kitajima, K., Niinemets, Ü., et al. (2017). Physiological and structural tradeoffs underlying the leaf economics spectrum. New Phytol. 214, 1447–1463. doi: 10.1111/nph.14496
Pataki, D. E., McCarthy, H. R., Gillespie, T., Jenerette, G. D., and Pincetl, S. (2013). A trait-based ecology of the Los Angeles urban forest. Ecosphere 4, 1–20. doi: 10.1890/ES13-00017.1
Pérez-Harguindeguy, N., Díaz, S., Garnier, E., Lavorel, S., Poorter, H., Jaureguiberry, P., et al. (2013). New handbook for standardised measurement of plant functional traits worldwide. Aust. J. Bot. 61, 167–234. doi: 10.1071/BT12225
Pierce, S., Brusa, G., Vagge, I., and Cerabolini, B. E. L. (2013). Allocating CSR plant functional types: the use of leaf economics and size traits to classify woody and herbaceous vascular plants. Funct. Ecol. 27, 1002–1010. doi: 10.1111/1365-2435.12095
Pierce, S., Negreiros, D., Cerabolini, B. E. L., Kattge, J., Díaz, S., Kleyer, M., et al. (2017). A global method for calculating plant CSR ecological strategies applied across biomes world-wide. Funct. Ecol. 31, 444–457. doi: 10.1111/1365-2435.12722
Reich, P. B. (2014). The world-wide “fast-slow” plant economics spectrum: a traits manifesto. J. Ecol. 102, 275–301. doi: 10.1111/1365-2745.12211
Rivers, M. C. (2015). Magnolia officinalis. The IUCN Red List of Threatened Species 2015: e.T34963A2857694.
Rosas, T., Mencuccini, M., Barba, J., Cochard, H., Saura-Mas, S., and Martínez-Vilalta, J. (2019). Adjustments and coordination of hydraulic, leaf and stem traits along a water availability gradient. New Phytol. 223, 632–646. doi: 10.1111/nph.15684
Saccone, P., Hoikka, K., and Virtanen, R. (2017). What if plant functional types conceal species-specific responses to environment? Study on arctic shrub communities. Ecology 98, 1600–1612. doi: 10.1002/ecy.1817
Sack, L., and Buckley, T. N. (2020). Trait Multi-Functionality in Plant Stress Response. Integrative and Comparative Biology. Oxford University Press. doi: 10.1093/icb/icz152
Salguero-Gómez, R., Jones, O. R., Archer, C. R., Buckley, Y. M., Che-Castaldo, J., Caswell, H., et al. (2015). The compadre Plant Matrix Database: an open online repository for plant demography. J. Ecol. 103, 202–218. doi: 10.1111/1365-2745.12334
Salguero-Gómez, R., Jones, O. R., Blomberg, S. P., Hodgson, D. J., Zuidema, P. A., and Kroon, H. De. (2016). Fast–slow continuum and reproductive strategies structure plant life-history variation worldwide. Proc. Natl. Acad. Sci. U.S.A. 113, 230–235. doi: 10.1073/pnas.1717717114
Sancho-Knapik, D., Escudero, A., Mediavilla, S., Scoffoni, C., Zailaa, J., Cavender-Bares, J., et al. (2020). Deciduous and evergreen oaks show contrasting adaptive responses in leaf mass per area across environments. New Phytol. 230, 521–534. doi: 10.1111/nph.17151
Scheres, B., and Van Der Putten, W. H. (2017). The plant perceptron connects environment to development. Nature 543, 337–345. doi: 10.1038/nature22010
Schloerke, B., Cook, D., Larmarange, J., Briatte, F., Marbach, M., Thoen, E., et al. (2020). Ggally: Extension to ggplot2. R Package Version 0.5. 0. Retrieved from: https://cran.r-project.org/web/packages/GGally/index.html (accessed December 18, 2020).
Skelton, R. P., West, A. G., and Dawson, T. E. (2015). Predicting plant vulnerability to drought in biodiverse regions using functional traits. Proc. Natl. Acad. Sci. U.S.A. 112, 5744–5749. doi: 10.1073/pnas.1503376112
Struckman, S., Couture, J. J., LaMar, M. D., and Dalgleish, H. J. (2019). The demographic effects of functional traits: an integral projection model approach reveals population-level consequences of reproduction-defence trade-offs. Ecol. Lett. 22:1396–1406. doi: 10.1111/ele.13325
Verbeeck, H., Bauters, M., Jackson, T., Shenkin, A., Disney, M., and Calders, K. (2019). Time for a plant structural economics spectrum. Front. For. Global Change 2, 1–5. doi: 10.3389/ffgc.2019.00043
Violle, C. (2007). Let the concept of trait be functional! Oikos 116, 882–892. doi: 10.1111/j.2007.0030-1299.15559.x
Walker, A. P., McCormack, M. L., Messier, J., Myers-Smith, I. H., and Wullschleger, S. D. (2017). Trait covariance: the functional warp of plant diversity? New Phytol. 216, 976–980. doi: 10.1111/nph.14853
Wang, R., Liu, X., Mou, S., Xu, S., and Zhang, Z. (2013). Temperature regulation of floral buds and floral thermogenicity in Magnolia denudata (Magnoliaceae). Trees Struct. Funct. 27, 1755–1762. doi: 10.1007/s00468-013-0921-x
Wang, R., and Zhang, Z. (2015). Floral thermogenesis: an adaptive strategy of pollination biology in Magnoliaceae. Commun. Integr. Biol. 8, 24–27. doi: 10.4161/19420889.2014.992746
Watkins, J. H. R., Cameron, R. W. F., Sjöman, H., and Hitchmough, J. D. (2020). Using big data to improve ecotype matching for Magnolias in urban forestry. Urban For. Urban Green. 48:126580. doi: 10.1016/j.ufug.2019.126580
Westoby, M. (1998). A leaf-height-seed (LHS) plant ecology strategy scheme. Plant Soil 199, 213–227. doi: 10.1023/A:1004327224729
Wickham, H., Chang, W., Henry, L., Pedersen, T. L., Takahashi, K., Wilke, C., et al. (2020). Package ‘ggplot2.’ CRAN. Retrieved from: http://ggplot2.tidyverse.org (accessed December 18, 2020).
World Conservation Monitoring Centre (1998). Magnolia macrophylla var. ashei. The IUCN Red List of Threatened Species 1998: e.T39001A10160188.
Keywords: urban forestry, species selection, functional ecology, plant traits, ecological theories and technologies
Citation: Watkins H, Hirons A, Sjöman H, Cameron R and Hitchmough JD (2021) Can Trait-Based Schemes Be Used to Select Species in Urban Forestry? Front. Sustain. Cities 3:654618. doi: 10.3389/frsc.2021.654618
Received: 16 January 2021; Accepted: 29 March 2021;
Published: 23 April 2021.
Edited by:
Kumelachew Yeshitela Habtemariam, Addis Ababa University, EthiopiaReviewed by:
Tenley M. Conway, University of Toronto Mississauga, CanadaChi Xu, Nanjing University, China
Copyright © 2021 Watkins, Hirons, Sjöman, Cameron and Hitchmough. This is an open-access article distributed under the terms of the Creative Commons Attribution License (CC BY). The use, distribution or reproduction in other forums is permitted, provided the original author(s) and the copyright owner(s) are credited and that the original publication in this journal is cited, in accordance with accepted academic practice. No use, distribution or reproduction is permitted which does not comply with these terms.
*Correspondence: Harry Watkins, aHdhdGtpbnNAc3RhbmRyZXdzYm90YW5pYy5vcmc=