- 1National Renewable Energy Laboratory, Catalytic Carbon Transformation and Scale-Up Center, Golden, CO, United States
- 2National Renewable Energy Laboratory, Strategic Energy Analysis Center, Golden, CO, United States
Circular economy concepts—including a circular bioeconomy—aim to transition the current, essentially linear, economic system to a more sustainable one. However, organizations and researchers currently define the circular economy concept differently, resulting in inconsistencies and difficulty in effectively implementing the framework. In this paper, we provide our perspective on the conceptual definitions of the circular economy, bioeconomy, and circular bioeconomy, outlining potential overlaps and differences and proposing a harmonized interpretation that stresses the importance of the carbon cycle. We conclude that the key goal of a circular economy is to slow, narrow, and close material resource loops, built on the foundation of renewable energy and non-toxic materials. Further, a sustainable bioeconomy goes beyond simply switching fossil resources with renewable, biological resources. It requires low-carbon energy inputs, sustainable supply chains, and promising disruptive conversion technologies for the sustainable transformation of renewable bioresources to high-value bio-based products, materials, and fuels. The bio-based circular carbon economy, in particular, stresses capturing atmospheric carbon via photosynthesis and exploiting this unique feature to the fullest extent possible. It sits at the intersection between the circular economy and the bioeconomy concept, resulting in a framework that focuses on closing the carbon cycle and stressing the opportunity to create an additional carbon sink capability in the technosphere by utilizing biogenic carbon for products and materials that are circulated in same or improved use cycles. Lastly, a sustainable circular bioeconomy transition will necessitate a set of consistent metrics that fit all products and industries.
Introduction
Human actions since the Industrial Revolution have become the primary driver of global environmental change. There has been a flawed relationship between humans and the natural environment that has caused us to face thresholds and tipping points linked to planetary boundaries, such as biodiversity loss and a global climate crisis (Tan, 2021). Our take-make-use-dispose linear economic system has enabled societies globally to prosper, albeit while simultaneously exploiting planetary resources (i.e., raw materials) and primary energy (i.e., fossil fuels) at an exponential rate. A critical element in this is the mismanagement of limited natural resources. All forms of capital rely on natural capital, with natural capital being non-substitutable. The concept of a sustainable use of resources, one that understands that “we received this world as an inheritance from past generations, but also as a loan from future generations” (Yardley, 2015), must attempt to minimize the impact of human activities on the natural environment, human health, and natural resources (Dewulf et al., 2015). Strengthening the initial transition from our current linear economy to a circular one is one possible option to balance our use of finite natural resources while making our economic system more resilient.
A circular economy tries to reduce the dependency on (new) natural resource extraction while increasing the time resources spend within the technosphere through alternate use cycles. The circular economy can be complemented by the bioeconomy, a concept that can incorporate economic activities related to the invention, development, production, and use of biological products and processes for energy, materials, and chemicals (Biofuture Platform, 2018). The resulting intersection can be referred to as the “circular bioeconomy.” Although it can potentially guide a transition to more sustainable production and consumption, the concept is still in a nascent stage, with many remaining challenges and research questions to be addressed. Notably, organizations and researchers define the concept differently, resulting in confusion and difficulty in effectively implementing the framework. Additionally, academia, industry, and policymakers frequently and inexactly interchange the terms “circular bioeconomy” and “sustainability.”
Here, we provide our perspective on the conceptual definitions of the circular economy, bioeconomy, and circular bioeconomy, outlining potential overlaps and differences to inform a broad set of stakeholders and proposing a harmonized interpretation that stresses the importance of the carbon cycle.
Definitions and Interpretations
Circular Economy
There are over 100 definitions of circular economy (e.g., Bocken et al., 2016; Geissdoerfer et al., 2017; Gladek, 2017; Kirchherr et al., 2017; Korhonen et al., 2018; Ellen MacArthur Foundation, 2019; Schöggl et al., 2020; Alhawari et al., 2021). Circular economy principally means different things to different people. It has basically become an “essentially contested concept,” a phrase coined by Gallie (1956) in which there is agreement on the means and goals of the concept but disagreements on how it is defined; it has recently been used to characterize the circular economy concept (Korhonen et al., 2018).
The existing definitions of circular economy found in literature can technically be grouped into two lines of thoughts: based on the description provided by the Ellen MacArthur Foundation (EMAF) and based on definitions developed by other researchers, as summarized in Table 1 in Korhonen et al. (2018) recent work. EMAF has defined a circular economy as a framework for an economy that is restorative and regenerative by design (Ellen MacArthur Foundation, 2019). Specifically, EMAF's circular economic model aims to create economic, natural, and social capital based on three core principles: (1) design out of waste and pollution, (2) keep products and materials in use, and (3) regenerate natural systems an industrial system that is restorative or regenerative. In contrast to the current linear model, a circular economy model emphasizes economic growth and activities that are dissociated from the consumption of finite resources and minimize system wastes, ultimately achieving positive society-wide benefits.
Conversely, among many definitions developed by various researchers (i.e., the non-EMAF-based definitions), such as from a production-consumption nexus perspective, the circular economy is defined as a “sustainable development initiative with the objective of reducing the societal production-consumption systems' linear material and energy throughput flows by applying materials cycles, renewable and cascade-type energy flows to the linear system. [Circular economy] promotes high value material cycles alongside more traditional recycling and develops systems approaches to the cooperation of producers, consumers and other societal actors in sustainable development work” (Korhonen et al., 2018). Another example is from Metabolic, which defines the circular economy as “a new economic model for addressing human needs and fairly distributing resources without undermining the functioning of the biosphere or crossing any planetary boundaries” (Gladek, 2017).
Consequently, with so many various definitions, how an organization implements the circular economy framework will strongly depend on how its decision makers or stakeholders interpret the concept and its definitions. For example, when based on the definition of the circular economy from EMAF (Ellen MacArthur Foundation, 2019), companies' business models will include minimizing the consumption of natural resources (such as virgin raw materials) and maximizing the use of waste materials. On the other hand, when based on Metabolic's definition (Gladek, 2017), companies' top priorities are not focused on staying away from consuming finite resources, but instead on meeting demands. Companies will use as many resources as required to produce goods and services to satisfy human needs, provided that no disturbances are created to the extent that they irreparably alter the system—namely, exceeding a system's thresholds or tipping points.
Furthermore, the circular economy is also interpreted simply as the “4R” framework: reduce, reuse, recycle, and recovery (e.g., Lieder and Rashid, 2016). The first three Rs relate to the reduction of resource consumption and the preservation of natural capital. The last R relates to the recovery of the resource in the form of energy (e.g., incineration of wastes for heating). It is also evident that the main focus of the circular economy appears to be on economic prosperity, followed by environment quality, and the social aspect of sustainability (i.e., human well-being) is rarely explored. The desired outcome of the circular economy transition is to overcome the current production and consumption model based on continuous growth and increasing resource throughput by decoupling economic growth from environmental pressure (e.g., Ghisellini et al., 2016; Murray et al., 2017).
It is apparent that there is no single universal definition for the circular economy. Based on the aforementioned definitions, we conclude that to achieve a circular economy is principally to slow, narrow, and close the material resource loops, all built on the foundation of renewable energy and non-toxic materials, as well as through long-lasting design, maintenance, repair, reuse, remanufacturing, refurbishing, and recycling (as illustrated in Figure 1A). The resource cycles—namely slowing, closing, and narrowing material resource loops—were first introduced by Bocken et al. (2016) and can serve as a working definition that is simple yet practical for a circular economy practitioner to interpret and implement.
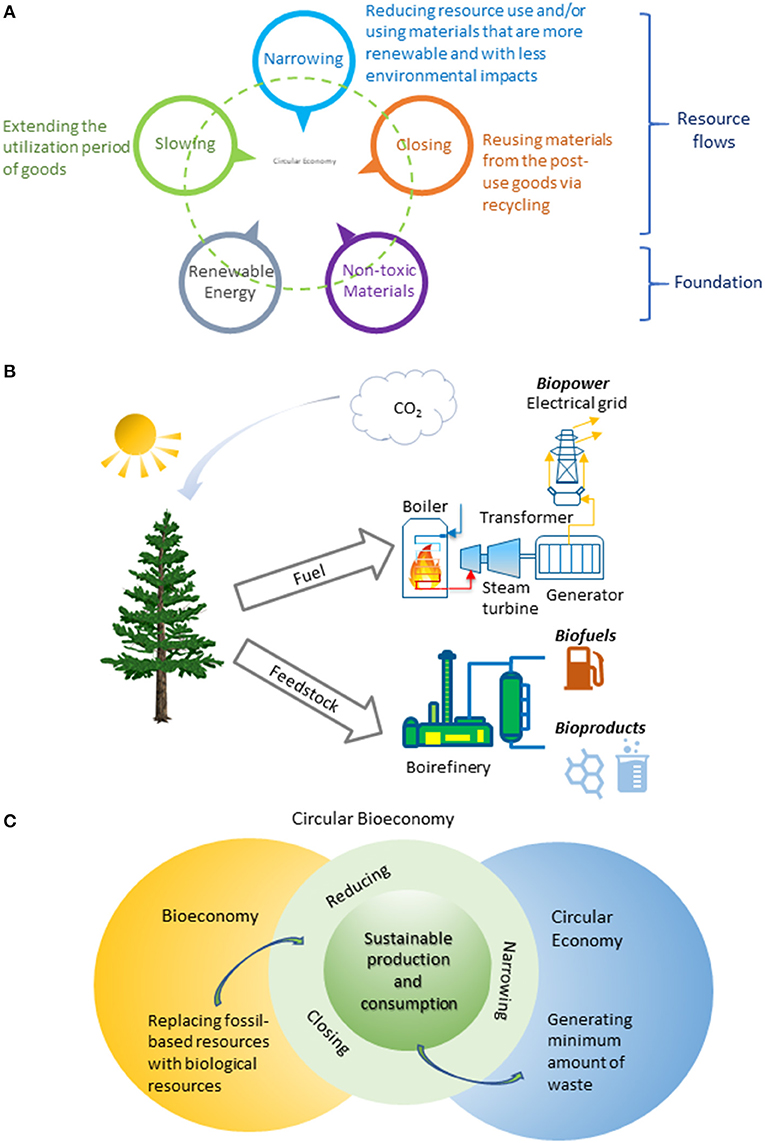
Figure 1. (A) Components of a proposed working circular economy definition; (B) Schematic representation of a sustainable bioeconomy—renewable biological resources to renewable products (i.e., biopower, biofuels, bioproducts); (C) Schematic representation of a circular bioeconomy resulting from the intersection between circular economy and bioeconomy concepts.
Bioeconomy
Similar to the circular economy, the bioeconomy is an emerging field whose definitions and interpretations are also surrounded by confusion (Aguilar et al., 2019; Giampietro, 2019). For example, according to the European Commission, the bioeconomy comprises the parts of the economy that utilize renewable biological resources to produce food, materials, and energy [European Commission Research Innovation Bioeconomy (ECRIB), 2019]. Similarly, the German Bioeconomy Council has defined the bioeconomy as all industrial and economic sectors, as well as their associated services, that produce or process biomass or use biological resources in any way (Efken et al., 2016). Meanwhile, the Biomass Research and Development Board in the United States adopts the bioeconomy definition from Golden and Handfield (2014) for its bioeconomy initiative [Biomass Research Development Board (BRDB), 2018], which defines the bioeconomy as the global industrial transition of sustainably utilizing renewable aquatic and terrestrial biomass resources in energy, intermediate, and final products for economic, environmental, social, and national security.
Within these definitions, it is implicit that the bioeconomy has to do with the use of renewable biological resources such as biomass to produce renewable biofuels, bioproducts, and biopower for economic, environmental, and social benefits (see Figure 1B). There is no disagreement that bio-based products are considered renewable and potentially offer reduced environmental impacts compared to their petroleum-derived counterparts (Adom et al., 2014). Bio-based products substitute fossil carbon with biogenic carbon originated from biomass, and their end-of-life carbon dioxide (CO2) emissions are biogenic CO2, which is considered carbon-neutral [Intergovernmental Panel on Climate Change (IPCC), 2014]. Additionally, when used as an energy source, biofuels and biopower are renewable energy that can displace fossil fuels and power to alleviate fossil resource depletion (Government Printing Office, 2012).
However, there is an ongoing debate on the sustainability aspect of the bioeconomy. Some definitions suggest that the bioeconomy is not intrinsically sustainable just because it is based on renewable resources (Pfau et al., 2014; Gawel et al., 2019). In fact, a non-sustainable bioeconomy can cause various sustainability conflicts. For example, an increase in biofuel demand will lead to an increase in biomass demand, which in turn will lead to competition for arable land use (i.e., land-grabbing for biomass feedstock production), freshwater consumption, and even food production (i.e., food vs. fuel), resulting in social unrest or social sustainability concerns. On the environmental sustainability aspect, there will also be negative environmental impacts due to the increase in land demand for biomass production, including more greenhouse gas emissions (responsible for global warming) due to indirect land-use changes (e.g., deforestation for growing energy crops [Plevin et al., 2010]). Another example is a recent study that reveals bio-based materials do not always exhibit positively in every environmental aspect (Vendries et al., 2020). When comparing petroleum- vs. bio-based packaging and food service ware, the latter exhibits lower global warming potential and fossil energy consumption but unfavorable environmental impacts in many areas, such as ozone depletion, acidification, water consumption, and eutrophication.
From a sustainability perspective, it has to be a sustainable bioeconomy—not merely a bioeconomy—to meet sustainability challenges. The current bioeconomy is still largely relying on non-renewable energy and fossil-based raw materials like nitrogen fertilizers, organic chemicals, and polymers that are predominantly derived from petroleum oil and gas. The sustainable bioeconomy is not just about substituting fossil resources with renewable resources; it will require sustainable biomass feedstock production, biomass conversion processes, and products. Moreover, a sustainable bioeconomy can be achieved by integrating with other interdisciplinary areas, such as the circular economy, discussed further in the next section.
Circular Bioeconomy
Most stakeholders would interpret the circular bioeconomy as the intersection between the two emerging concepts of the circular economy and the bioeconomy, as depicted in Figure 1C. However, the relationship between the circular economy and the bioeconomy is rather complex and an ongoing debate. The European Commission's bioeconomy strategy interprets the circular bioeconomy as a framework to reduce the dependence on natural resources; transform manufacturing; promote sustainable production of renewable resources from land, fisheries, and aquaculture; and promote their conversion into various bio-based products and bioenergy while growing new jobs and industries (European Commission, 2013). A circular bioeconomy is also interpreted as an idea to stimulate the economic growth of developed economies that combines a desirable “what” (circular economy) with a feasible, viable, and desirable “how” (bioeconomy) (Giampietro, 2019). Alternatively, a circular bioeconomy is simply interpreted as a more efficient resource management of bio-based renewable resources by integrating circular economy principles into the bioeconomy (D'Amato et al., 2018). The debate on the relationship between circular economy and bioeconomy can also be boiled down to any of the following five relationships: (1) the circular economy and bioeconomy as separate but reinforcing, (2) both concepts as completely integrated, (3) both concepts as partially antagonistic, (4) the bioeconomy as a precondition to a circular economy, or (5) the circular economy as a tool to move from a fossil economy to a bioeconomy (Leipold and Petit-Boix, 2018).
Additionally, some would also argue that the bioeconomy is already inherently “circular by nature” as it is operating on the basis of the renewable and circular character of the planetary ecosystems (Leipold and Petit-Boix, 2018). For example, bio-based products derived from renewable biological carbon (the main component of biological compounds such as biomass) are recycled and reused through the biosphere (i.e., carbon cycle). On the other hand, the bioeconomy is not entirely circular if it is based on EMAF's butterfly circular economy system diagram (Ellen MacArthur Foundation, 2019). According to the butterfly diagram, the circular economy includes both biological and technical cycles. The bioeconomy is only associated with the biological cycle, in which materials are made from biological nutrients (i.e., bio-based products) that can be safely reintroduced into the biosphere.
Ultimately, the challenge for stakeholders is that there is no consensus on the relationship between the circular economy and the bioeconomy, which is to say that there is no single definition of the circular bioeconomy besides stakeholders' own understandings of the two individual concepts. Nevertheless, we can alleviate the confusion by virtue of the fact that both the circular economy and bioeconomy have their potentials and limitations. On one hand, the circular economy focuses on efficiency gains and slowing, narrowing, and closing material loops to reduce resource consumption and system waste via input reductions, sustainable design, improved practices, waste reuse, and recycling (Bocken et al., 2016; Ghisellini et al., 2016; Geissdoerfer et al., 2017; Kirchherr et al., 2017; Murray et al., 2017; D'Amato et al., 2018; Ellen MacArthur Foundation, 2019). Similarly, the bioeconomy enables the transition from fossil-based to biomass-based industrial inputs and emphasizes the sustainable use of renewable resources for economic, environmental, and social benefits (Pfau et al., 2014; D'Amato et al., 2018; Gawel et al., 2019). For example, for biodegradable bioplastics, up to 90% of the organic carbon can be converted to CO2 in about 180 days; the carbon in the form of CO2 can return to the biosphere after the use phase (Karan et al., 2019). This can also serve as an example of a circular bioeconomy and how the bioeconomy can enable the circular economy to resolve sustainability challenges.
On the other hand, the limitations associated with the circular economy are the primary emphases on economic and environmental gains while neglecting the social dimension to any significant extent (Kirchherr et al., 2017; D'Amato et al., 2018; Korhonen et al., 2018). Additionally, efficiency gains often lead to rebound effects in terms of increasing production and consumption, thereby failing to achieve net environmental benefits. For example, the fuel efficiency gain for passenger vehicles may lead to more driving, resulting in more greenhouse gas emissions. Similarly, the bioeconomy cannot necessarily deliver the perceived environmental benefits solely through the substitution of fossil-based resources with bio-based ones. Moreover, both the circular economy and the bioeconomy are resource-oriented concepts (e.g., renewable materials, material recycle), which essentially fail to address synergies and conflicts with broader ecological processes and ecosystem services such as water, carbon and nutrient cycles (including emissions and waste), quality of energy source and efficiency in production and use, and maintenance of biodiversity, ecosystems, and related services (D'Amato et al., 2017, 2018). For example, in addition to competition for fresh water and food production, intensifying biomass production may conflict with other social, economic, and environmental functions of forests. An expanded bioeconomy could potentially displace economic output and jobs in different sectors and could also compete with other advanced technologies (e.g., electric vehicles) [Biomass Research Development Board (BRDB), 2018].
We agree with some researchers that the circular bioeconomy should be viewed as an emerging concept aiming to address the debate on the definite contribution of the circular economy and the bioeconomy to resolving sustainability challenges (D'Amato et al., 2017, 2018). The circular bioeconomy is not just about adopting circularity principles such as biomass cascading (i.e., putting materials into different uses after end of life across different value streams), waste hierarchy, and efficiency in the utilization of biomass or renewable resources (i.e., extracting the maximum practical benefits from products and generating the minimum amount of waste) (Bezama, 2016). Instead, the circular bioeconomy is actually “more than bioeconomy or circular economy alone” (D'Amato et al., 2018). Therefore, the existence of a circular bioeconomy makes sense only if the two concepts complement each other; each plays an enabling role.
Closing and Leveraging the Biogenic Carbon Cycle: Bio-Based Circular Carbon Economy
The common theme across all concepts is a push toward increasing circularity within the economic system, while the circular bioeconomy also stresses the use of bio-based materials. Independent of the concept, carbon will remain the core molecule that builds fuels, fiber, chemicals, and other products. This implies that, in our opinion, the circular bioeconomy is truly a bio-based circular carbon economy—one that removes, efficiently uses, and sequesters more carbon than it emits (Babson, 2020).
The bio-based circular carbon economy is a bioeconomy that focuses on leveraging and exploiting the atmospheric carbon drawdown potential of biomass feedstock to the fullest extent possible. The atmospheric carbon sequestration in plants via photosynthesis, the conversion of the biogenic carbon into a series of short- and long-lived products, and their continuous cycling are part of circular carbon economy strategies that capture carbon in the technosphere over the long term (Figure 2). Biogenic carbon capture and utilization routes offer additional avenues to support the decarbonization of the economy, in addition to carbon capture and belowground sequestration. Utilizing biogenic or renewable carbon as the core structural molecule offers many sectors new avenues to achieve net-zero emission targets, especially so-called hard-to-decarbonize industries like petrochemicals (Kätelhön et al., 2019; Fernández et al., 2020).
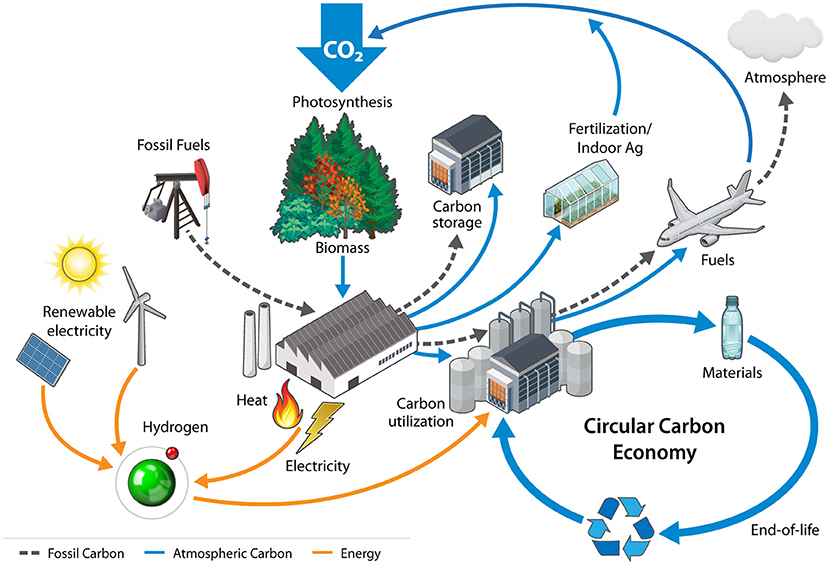
Figure 2. The bio-based circular carbon economy can create an additional carbon sink in the technosphere via photosynthetic atmospheric carbon sequestration in biomass feedstock, energy, and material conversion pathways plus carbon capture and (re)utilization in closed circular carbon economy loops.
The greenhouse gas emissions and resulting carbon efficiency of CO2 utilization routes, including CO2 to fuels or chemicals, heavily depend on the greenhouse gas intensity of key process inputs, foremost electricity and hydrogen (Grim et al., 2020). Thus, achieving net-zero emission sectors depends on decarbonizing the energy system and appropriately integrating and linking utilization routes. Figure 2 graphically represents this interdependency of the bio-based circular economy's sustainability as a system of systems.
Atmospheric carbon capture via plant growth is more energy-efficient than atmospheric carbon capture and utilization via direct air capture, which might require 10–25 times more electricity (Gabrielli et al., 2020). The critical assumption here is sustainable biomass production practices, including preventing potential adverse side effects from the utilization of primary (purpose-grown) biomass. If these parameters can be ensured, the bio-based circular carbon economy can sustainably produce food, feed, chemicals, fuels, and materials for a plethora of industries. It also introduces new, bio-based products that offer similar or superior characteristics to their petroleum-derived substitutes while maintaining a lower carbon footprint and full biodegradability (Meyer et al., 2020).
Conclusions and Recommendations
This paper evaluates the existing confusion around the conceptual definitions of the circular economy, bioeconomy, and circular bioeconomy to clarify some misperceptions and ambiguities for stakeholders and researchers. The scientific and research content of the circular economy concept is rather superficial and unorganized. The circular economy has become an “essentially contested concept,” which means there is agreement on the means and goals of the concept but disagreements on its definition. The existing circular economy definitions can basically be grouped into two lines of thought: those based on the definition provided by the Ellen MacArthur Foundation and those from other researchers. We conclude that to achieve a circular economy is to essentially slow, narrow, and close material resource loops, all built on the foundation of renewable energy and non-toxic material. Slowing material resource flows means extending the useful life of goods through activities such as sharing, repair, upgrades, resale, and initial product design choices. Narrowing resource flows means using less and/or different materials to minimize each product's environmental footprint. The goal is to use fewer resources to achieve the same purpose, and the strategy can be used in conjunction with the “slowing” strategy. Finally, closing resource flows means reducing or eliminating mining and unsustainable harvesting of new materials and ensuring products are ultimately recycled or composted at their end of life.
Regarding the bioeconomy, there is no consensus concerning what it actually implies. It is generally agreed that the bioeconomy implicitly has to do with the use of renewable biological resources to produce renewable biofuels, bioproducts, and biopower for economic, environmental, and social benefits. However, the bioeconomy is not intrinsically sustainable just because it is based on renewable resources. In our view, a sustainable bioeconomy will require more than the substitution of fossil resources with renewable biological resources; it will also require a sustainable supply chain, including sustainable biomass feedstock production and logistics, sustainable biomass conversion processes, and sustainable products. Therefore, the success of the bioeconomy will depend heavily on the development of disruptive biorefining technologies for the sustainable transformation of bio-based and renewable resources to high-value bio-based products, materials, and fuels.
The circular bioeconomy is essentially the intersection between the circular economy and the bioeconomy. Although the relationship between the circular economy and the bioeconomy is still debatable, we suggest viewing it as an emerging concept aiming to address the debate on the definite contributions of the circular economy and the bioeconomy to resolving sustainability challenges. Ultimately, the circular bioeconomy is more than the bioeconomy or circular economy alone; the two concepts complement each other, resulting in a more sustainable framework.
Additionally, the circular bioeconomy is truly a bio-based circular carbon economy. Carbon is an integral part of the circular bioeconomy; it is critical to close the carbon cycle and create an additional carbon sink capability in the technosphere by utilizing biogenic carbon for products and materials circulated in the same or improved use cycles. Therefore, a bio-based circular carbon economy represents a framework to increase circularity within the economic system.
Although multifaceted and still evolving, it is noteworthy that all these concepts are complementary and have the common goal of creating a more sustainable future. Future study will explore the interface between the biobased circular carbon economy and other global efforts for decarbonization, such as green economy (Loiseau et al., 2016) and low-carbon economy (Tavoni et al., 2012). It is also critical to develop a set of (bio)circularity metrics that fit all products and industries. Peter Drucker, a management thinker, is often quoted as saying that “you can't manage what you can't measure.” (Bio)circularity metrics are important for assessing the effects of a circular (bio)economy in terms of profitability, job creation, and environmental impacts. Circularity should also include some environmental and sustainability elements, including some life cycle environmental impact categories and new socio-economic indicators (D'Adamo et al., 2020; Kardung et al., 2021). The metrics should help identify which (bio)circular attributes and options are better than others. For example, material circularity is an informative and streamlined indicator that can assess how well a product or company performs in the context of a circular economy. Communicating among circular (bio)economy practitioners through publications, workshops, and conferences can help the community harmonize these (bio)circularity metrics and approaches. Stakeholders should also include regulators, environmental activists, businesses, and investors.
Data Availability Statement
The original contributions presented in the study are included in the article, further inquiries can be directed to the corresponding author.
Disclosure
The views expressed in the article do not necessarily represent the views of the DOE or the U.S. Government.
Author Contributions
ET initiated the work and wrote a draft. ET and PL both wrote the final manuscript. All authors contributed to the article and approved the submitted version.
Conflict of Interest
The authors declare that the research was conducted in the absence of any commercial or financial relationships that could be construed as a potential conflict of interest.
Acknowledgments
This work was authored by the National Renewable Energy Laboratory, managed and operated by Alliance for Sustainable Energy, LLC, for the U.S. Department of Energy (DOE) under Contract No. DE-AC36-08GO28308. The U.S. Government retains and the publisher, by accepting the article for publication, acknowledges that the U.S. Government retains a non-exclusive, paid-up, irrevocable, worldwide license to publish or reproduce the published form of this work, or allow others to do so, for U.S. Government purposes. The authors acknowledge Alfred Hicks, NREL, who helped design Figure 2.
References
Adom, F., Dunn, J. B., Han, J., and Sather, N. (2014). Life-cycle fossil energy consumption and greenhouse gas emissions of bioderived chemicals and their conventional counterparts. Environ. Sci. Technol. 48, 14624–14631. doi: 10.1021/es503766e
Aguilar, A., Twardowski, T., and Wohlgemuth, R. (2019). Bioeconomy for sustainable development. Biotechnol. J. 14:1800638. doi: 10.1002/biot.201800638
Alhawari, O., Usama, A., Bhutta, M. K. S., and Ülkü, M. A. (2021). Insights from circular economy literature: a review of extant definitions and unravelling paths to future research. Sustainability 13, 859–880. doi: 10.3390/su13020859
Babson, D. (2020). Changing What's Possible for the New Carbon Economy–Advanced Technologies to Value and Valorize Carbon Throughout the Bioeconomy Supply Chain. Available online at: https://arpa-e.energy.gov/?q=news-item/changing-whats-possible-new-carbon-economy#_ftnref1 (accessed April 15, 2021).
Bezama, A. (2016). Let us discuss how cascading can help implement the circular economy and the bio-economy strategies. Waste Manag. Res. 34, 593–594. doi: 10.1177/0734242X16657973
Biofuture Platform (2018). Creating the Biofuture: A Report on the State of the Low Carbon Bioeconomy. Available online at: http://www.biofutureplatform.org/post/new-biofuture-report-global-greenhouse-gas-goals-out-of-reach-without-biofuels-and-bioproducts (accessed April 15, 2021).
Biomass Research Development Board (BRDB) (2018). The Bioeconomy Initiative: Implementation Framework. DOE/EE-1865. Washington, DC: BRDB. Available online at: https://biomassboard.gov/sites/default/files/pdfs/Bioeconomy_Initiative_Implementation_Framework_FINAL.pdf (accessed April 15, 2021).
Bocken, N. M. P., de Pauw, I., Bakker, C., and van der Grinten, B. (2016). Product design and business model strategies for a circular economy. J. Ind. Prod. Eng. 33, 308–320. doi: 10.1080/21681015.2016.1172124
D'Adamo, I., Falcone, P. M., and Morone, P. (2020). A new socio-economic indicator to measure the performance of bioeconomy sectors in Europe. Ecol. Econ. 176:106724. doi: 10.1016/j.ecolecon.2020.106724
D'Amato, D., Droste, N., Allen, B., Kettunen, M., Lähtinen, K., Korhonen, J., et al. (2017). Green, circular, bio economy: a comparative analysis of sustainability avenues. J. Clean. Prod. 168, 716–734. doi: 10.1016/j.jclepro.2017.09.053
D'Amato, D., Veijonaho, S., and Toppinen, A. (2018). Towards Sustainability? forest-based circular bioeconomy business models in finnish SMEs. For. Policy Econ. 110:101848. doi: 10.1016/j.forpol.2018.12.004
Dewulf, J., Benini, L., Mancini, L., Sala, S., Blengini, G. A., Ardente, F., et al. (2015). Rethinking the area of protection “natural resources” in life cycle assessment. Environ. Sci. Technol. 49, 5310–5317. doi: 10.1021/acs.est.5b00734
Efken, J., Dirksmeyer, W., Kreins, P., and Knecht, M. (2016). Measuring the importance of the bioeconomy in germany: concept and illustration. NJAS Wagen. J. Life Sci. 77, 9–17. doi: 10.1016/j.njas.2016.03.008
Ellen MacArthur Foundation (2019). What Is a Circular Economy? Available online at: https://www.ellenmacarthurfoundation.org/circular-economy/concept (accessed April 13, 2019).
European Commission (2013). Bioeconomy. Available online at: https://ec.europa.eu/programmes/horizon2020/en/h2020-section/bioeconomy (accessed November 20, 2013).
European Commission Research Innovation Bioeconomy (ECRIB) (2019). European Commission Research and Innovation Bioeconomy. Available online at: https://ec.europa.eu/research/bioeconomy/index.cfm?pg=policy (accessed October 20, 2019).
Fernández, J. R., Garcia, S., and Sanz-Pérez, E. S. (2020). CO2 capture and utilization editorial. Ind. Eng. Chem. Res. 59, 6767–6772. doi: 10.1021/acs.iecr.0c01643
Gabrielli, P., Gazzani, M., and Mazzotti, M. (2020). The role of carbon capture and utilization, carbon capture and storage, and biomass to enable a net-zero-CO2 emissions chemical industry. Ind. Eng. Chem. Res. 59, 7033–7045. doi: 10.1021/acs.iecr.9b06579
Gallie, W. B. (1956). IX.—Essentially contested concepts. Proc. Arist. Soc. 56, 167–198. doi: 10.1093/aristotelian/56.1.167
Gawel, E., Pannicke, N., and Hagemann, N. (2019). A path transition towards a bioeconomy–the crucial role of sustainability. Sustainability 11:3005. doi: 10.3390/su11113005
Geissdoerfer, M., Savaget, P., Bocken, N. M. P., and Hultink, E. J. (2017). The circular economy—a new sustainability paradigm? J. Clean. Prod. 143, 757–768. doi: 10.1016/j.jclepro.2016.12.048
Ghisellini, P., Cialani, C., and Ulgiati, S. (2016). A review on circular economy: the expected transition to a balanced interplay of environmental and economic systems. J. Clean. Prod. 114, 11–32. doi: 10.1016/j.jclepro.2015.09.007
Giampietro, M. (2019). On the circular bioeconomy and decoupling: implications for sustainable growth. Ecol. Econ. 162, 143–156. doi: 10.1016/j.ecolecon.2019.05.001
Gladek, E. (2017). The Seven Pillars of the Circular Economy–From Linear to Circular. Available online at: https://www.metabolic.nl/news/the-seven-pillars-of-the-circular-economy/ (accessed February 6, 2017).
Golden, J. S., and Handfield, R. B. (2014). Why Biobased? Opportunities in the Emerging Bioeconomy. Washington, DC: U.S. Department of Agriculture. Available online at: https://www.biopreferred.gov/files/WhyBiobased.pdf (accessed April 15, 2021).
Government Printing Office (2012). Supplemental determination for renewable fuels produced under the final rfs2 program from grain sorghum. Fed. Regist. 77, 74592–74607. https://www.federalregister.gov/documents/2012/12/17/2012-30100/supplemental-determination-for-renewable-fuels-produced-under-the-final-rfs2-program-from-grain (accessed April 15, 2021).
Grim, R. G., Huang, Z., Guarnieri, M. T., Ferrell, J. R., Tao, L., and Schaidle, J. A. (2020). Transforming the carbon economy: challenges and opportunities in the convergence of low-cost electricity and reductive CO2 utilization. Energy Environ. Sci. 13, 472–494. doi: 10.1039/C9EE02410G
Intergovernmental Panel on Climate Change (IPCC). (2014). Intergovernmental Panel on Climate Change (IPCC) Fifth Assessment Report - Impacts, Adaptation and Vulnerability. http://www.ipcc.ch/report/ar5/wg2/
Karan, H., Funk, C., Grabert, M., Oey, M., and Hankamer, B. (2019). Green bioplastics as part of a circular bioeconomy. Trends Plant Sci. 24, 237–249. doi: 10.1016/j.tplants.2018.11.010
Kardung, M., Cingiz, K., Costenoble, O., Delahaye, R., Heijman, W., Lovrić, M., et al. (2021). Development of the circular bioeconomy: drivers and indicators. Sustainability 13:413. doi: 10.3390/su13010413
Kätelhön, A., Meys, R., Deutz, S., Suh, S., and Bardow, A. (2019). Climate change mitigation potential of carbon capture and utilization in the chemical industry. Proc. Natl. Acad. Sci. 116, 11187–11194. doi: 10.1073/pnas.1821029116
Kirchherr, J., Reike, D., and Hekkert, M. (2017). Conceptualizing the circular economy: an analysis of 114 definitions. Resour. Conserv. Recycling 127, 221–232. doi: 10.1016/j.resconrec.2017.09.005
Korhonen, J., Nuur, C., Feldmann, A., and Birkie, S. E. (2018). Circular economy as an essentially contested concept. J. Clean. Prod. 175, 544–552. doi: 10.1016/j.jclepro.2017.12.111
Leipold, S., and Petit-Boix, A. (2018). The circular economy and the bio-based sector–perspectives of european and german stakeholders. J. Clean. Prod. 201, 1125–1137. doi: 10.1016/j.jclepro.2018.08.019
Lieder, M., and Rashid, A. (2016). Towards circular economy implementation: a comprehensive review in context of manufacturing industry. J. Clean. Prod. 115, 36–51. doi: 10.1016/j.jclepro.2015.12.042
Loiseau, E., Saikku, L., Antikainen, R., Droste, N., Hansjürgens, B., Pitkänen, K., et al. (2016). Green economy and related concepts: an overview. J. Clean. Prod. 139, 361–371. doi: 10.1016/j.jclepro.2016.08.024
Meyer, V., Basenko, E. Y., Benz, J. P., Braus, G. H., Caddick, M. X., Csukai, M., et al. (2020). Growing a circular economy with fungal biotechnology: a white paper. Fungal Biol. Biotechnol. 7:5. doi: 10.1186/s40694-020-00095-z
Murray, A., Skene, K., and Haynes, K. (2017). The circular economy: an interdisciplinary exploration of the concept and application in a global context. J. Bus. Ethics 140, 369–380. doi: 10.1007/s10551-015-2693-2
Pfau, S. F., Hagens, J. E., Dankbaar, B., and Smits, A. J. M. (2014). Visions of sustainability in bioeconomy research. Sustainability 6, 1222–1249. doi: 10.3390/su6031222
Plevin, R. J., O'Hare, M., Jones, A. D., Torn, M. S., and Gibbs, H. K. (2010). Greenhouse gas emissions from biofuels' indirect land use change are uncertain but may be much greater than previously estimated. Environ. Sci. Technol. 44, 8015–8021. doi: 10.1021/es101946t
Schöggl, J.-P., Lukas, S., and Rupert, J. B. (2020). The narrative of sustainability and circular economy–a longitudinal review of two decades of research. Resour. Conserv. Recycling 163, 105073–105094. doi: 10.1016/j.resconrec.2020.105073
Tan, E. C. D. (2021). “Sustainable biomass conversion process assessment,” in Process Intensification and Integration for Sustainable Design, ed. D. C. Y. Foo and M. M. El-Halwagi (Weinheim: John Wiley and Sons, Ltd), 301–18.
Tavoni, M., De Cian, E., Luderer, G., Steckel, J. C., and Waisman, H. (2012). The value of technology and of its evolution towards a low carbon economy. Clim. Change 114, 39–57. doi: 10.1007/s10584-011-0294-3
Vendries, J., Sauer, B., Hawkins, T. R., Allaway, D., Canepa, P., Rivin, J., et al. (2020). The significance of environmental attributes as indicators of the life cycle environmental impacts of packaging and food service ware. Environ. Sci. Technol. 54, 5356–5364. doi: 10.1021/acs.est.9b07910
Yardley, J. (2015). Pope francis, in ecuador, calls for more protection of rain forest and its people. The New York Times. Available online at: https://www.nytimes.com/2015/07/08/world/americas/pope-francis-in-ecuador-calls-for-more-protection-of-rain-forest-and-its-people.html (accessed July 7, 2015).
Keywords: circular economy, bioeconomy, carbon cycle, carbon utilization, sustainability
Citation: Tan ECD and Lamers P (2021) Circular Bioeconomy Concepts—A Perspective. Front. Sustain. 2:701509. doi: 10.3389/frsus.2021.701509
Received: 28 April 2021; Accepted: 14 June 2021;
Published: 12 July 2021.
Edited by:
Andrea Lanzini, Politecnico di Torino, ItalyReviewed by:
Arkadiusz Piwowar, Wroclaw University of Economics, PolandDavide Settembre Blundo, Rey Juan Carlos University, Spain
Copyright © 2021 Tan and Lamers. This is an open-access article distributed under the terms of the Creative Commons Attribution License (CC BY). The use, distribution or reproduction in other forums is permitted, provided the original author(s) and the copyright owner(s) are credited and that the original publication in this journal is cited, in accordance with accepted academic practice. No use, distribution or reproduction is permitted which does not comply with these terms.
*Correspondence: Eric C. D. Tan, eric.tan@nrel.gov