- The Styring Group, Department of Chemical and Biological Engineering, The University of Sheffield, Sheffield, United Kingdom
Environmental sustainability assessments have been conducted around consumer goods since the 1960's, these adopted comparative approaches and followed no accepted methodology. As sustainability assessment rose to prominence, methodological standardization was universally called for. Furthermore, two additional “strands” of sustainability emerged, economic and societal; forming what has recently been termed the “triple helix”. Efforts have been made across the CCU (carbon capture and utilization) community to align, or “harmonize”, the respective assessment formats. Ultimately, targeting enhanced understanding of the interconnections and trade-offs between the three strands, and communication of findings to both industry and policymakers. This review examines key methodologies presented in the field. These were collated through targeted literature searches, focussing on standalone, CCU specific, and harmonized methodologies. Relevant guidance originates with ISO's 2007 standards and terminates in McCord et al's (2021) “triple helix framework”. Other key works reviewed include UNEP / SETAC's S-LCA (social life cycle assessment) guidelines, and GCIs (Global CO2 Initiative) integrated LCA and TEA (techno-economic assessment) guidelines. Analysis of the identified methodologies first considers each assessment strand in isolation, subsequently evaluating efforts toward their CDU specific harmonization and integration. Using the collated primary and secondary literature, a taxonomy of assessment methodologies leading to the triple helix framework is produced. Key methodological difficulties and divergent schools of thought are discussed, notably the prescription of system boundaries, impact indicators, and characterization methods. The overarching conclusion of the review is that while a robust combined LCA and TEA assessment methodology has been attained, holistic approaches incorporating social sustainability are still lacking; with substantial problems remaining unsolved. A majority of these originate from SIA's immaturity relative to LCA and TEA, causing issues around data availability and handling methods; exacerbated by the presence of qualitative data. Until a greater degree of maturity is achieved, SIA should be utilized within holistic assessments as a screening tool, determining the suitability of a process or system for more granular assessment.
1. Introduction
For many years, life cycle and techno-economic assessments have been seen as a vehicle through which products and processes can be compared to each other, or a relevant benchmark. Evaluating the performance of alternatives against widely accepted and quantifiable indicators provides insight into their respective performance profiles. A younger and less developed parallel sub-field (Venkatesh, 2019), societal sustainability assessment (SIA), is receiving increased attention. This has resulted in the development of its own assessment guidelines and reporting approaches. Together these aspects; environmental, economic, and social, can be considered the “three strands” of sustainability (United Nations, 2015a). When assessed in parallel for a given product or process, a holistic overview of their sustainability profile is obtained.
CCU technologies require these holistic assessments in order to manage and quantify burden shifts; occurring when improvements in one strand catalyze detrimental effects in another. A pertinent example from a parallel field is the transition to EVs for personal transport. While benefits can be seen through environmental metrics (European Environment Agency, 2018; Ricardo Inc, 2022), there is risk of producing a “mobility underclass” through high prices and lack of access to private off-street charging facilities. Lower income households, usually commanding no off-street parking, could expect to pay £20 per' charge or “tank” via commercial charging points; almost a threefold increase on the £7 realized by private charging (Witchalls, 2018); a trade-off that would only be revealed through combined assessments. Such insights are extremely valuable to engineers, entrepreneurs, and policy makers alike; all of whom must collaborate to see real movement toward widespread successful CCU deployment. However, to realize these meaningful insights, holistic assessment methodologies must be identified and improved, allowing for robust integration of previously stand-alone approaches.
Despite the clear advantages of integrated holistic assessments, differences in their respective methodological approaches, or lack thereof, have historically made the three pillars difficult to align simultaneously: most notably with respect to maintaining consistent, well defined system boundaries and assumptions. This led to calls for a harmonized set of methodologies to conduct comparable assessments (Zimmermann and Schomäcker, 2017; McCord et al., 2021; Wunderlich et al., 2021), providing the previously discussed holistic view of product or process performance. Outcomes from such studies have the potential to significantly improve current and future decision making, driving increased efficiency of resource allocation, particularly within R&D and policy making. When used to guide relatively fledgling fields such as CCU, this results in considerable influence over the selection of projects. Subsequently moderating progression rates toward both climate and wider sustainability goals such as the Paris Agreement (United Nations / Framework Convention on Climate Change, 2015) and UN SDGs (United Nations, 2015b).
While assessment methodologies are present for each independent strand, the research area is complex, harboring various schools of thought. An overarching critical review of assessment methodologies and their degree of alignment is currently absent from the literature. Since 2006 multiple different standards, guidelines and frameworks have been published, targeting both generic and CCU specific applications of sustainability assessments. Movement toward the integration of strands and respective methodologies was initially slow. However, owing to increased demand for such approaches, the pace has increased in recent years. The following review examines the ongoing pursuit of this goal within CCU focused integrated assessments, identifying the progress made, common pitfalls, sources of divergence, and areas for future development. Ultimately addressing the question; “to what extent has harmonization been achieved within holistic CCU sustainability assessment methodologies”?
Such integrated methodologies could play a pivotal role in the validation of CCU as a climate mitigation strategy. While the field has great potential to reduce global greenhouse gas emissions, this must be robustly quantified. Integrated assessments are the best tool for this, considering not only the origin and final fate of the CO2 incorporated within CCU products, but also the energy and resources required for its utilization. Furthermore, they can provide a direct comparison of CCU processes against the current industry standard, verifying better performance over a wide range of available impact categories, including economic and societal aspects.
CCU technologies are usually highly energy intensive. The green generation of this energy must be traced and accounted for in order to confirm any sustainability improvements; alternate fossil-based energy mixes result in highly ambiguous, and sometimes non-existent, emissions savings. Furthermore, issues around infrastructure (e.g., pipelines, engine fuel specification, etc.) and process operating costs have historically plagued the economic viability of CCU projects. The associated transition phase and elevated consumer prices go on to catalyze detrimental societal effects, as seen in the EV example previously. Understanding and combatting these interlinked sustainability issues will enable faster and more effective development within the field.
2. Methodology
Guidance around the conduction of sustainability assessments is broad, encompassing a diverse range of methodologies. This review aims to collate and assess only CCU specific guidance documents, or those from which these are derived. Their assessment is meaningful due to sustainability assessment's importance as a guidance tool in the development and roll out of CCU technologies. Secondly, to ratify that both the diversity of needs, and nature of methodological hurdles, exhibited by different sectors necessitates a departure from generalized guidance. The identified approaches are reviewed, identifying areas in which harmonization is achieved, and those where it is still lacking.
2.1. Identifying literature
A semi-systematic approach (Figure 1) was taken to the collation of literature within this review. Initial searches were conducted using bibliographic databases and academic search engines; primarily web of science, google scholar and science direct. Together these provide access to a vast majority of academic literature. Search terms initially focused on the individual assessment strands, identifying cornerstone guidelines. Common identifiers for the respective strands (e.g., LCA, E-LCA, life cycle assessment, etc.) were utilized, coupled with “standards”, “guidelines” and “framework”. This revealed the major guidance documents, later confirmed by their widespread proliferation within consulted assessments and inter-guideline cross-referencing.
Secondary literature was collated in the form of existing reviews, focusing on individual assessment strands. These are to determine the overall “topography” and development of the respective research areas. Additionally, these serve to provide large numbers of relevant pre-screened primary sources. Where possible, multiple secondary sources were consulted within the same strand, aiming to identify and prevent any potential propagation of bias.
A second round of literature identification was conducted, this time sequentially adding the terms CDU, CCU, and CCUS to fielded searches. The purpose of which was to identify any sector specific guidance documents or assessments. In some cases, this returned highly relevant documents, such as the GCI (Global CO2 Initiative) guidelines (discussed in Section 3.1.). Other times, no relevant publications were identified. In these lacking cases, it will be assumed that no specific guidance is available beyond the previously collated general guidelines. A third and final search of literature was carried out, including the terms “holistic”, “harmonized”, and “integrated”. This was by far the least lucrative round of literature searching; attributed to the infancy of integration efforts.
2.2. Approach to analysis
A review of harmonization and integration within CCU focussed sustainability assessment is novel within literature. Consequently, this work aims to evaluate the current best practices and most promising methodologies. While this requires an initially broad and general approach to methodological comparisons, it is hoped that a strong foundational understanding of the field and its current shortcomings and successes are contributed. Subsequent reviews around the specific issues identified herein would further enrich understanding and support continued improvement of assessment techniques.
Initially, relevant frameworks and guidelines pertaining to each independent strand are dissected. Examining the structures and principles of the methodologies facilitates the construction of a “taxonomy” of guidance, charting the development path toward a holistic approach. Integrated approaches are reviewed, identifying any loss of resolution or conflicting schools of thought. Comparability, clarity of results communication, robustness and transparency within each methodology will be considered the metrics for success.
Beyond these largely intangible aspects of the methodologies, the approaches to indicator calculation will be examined and comparisons drawn between strands for both independent and integrated approaches. The standardization of both procedures and assessment structure are deemed a core aspect of harmonization. Consequently, the LCA strand will be viewed as the template for methodological homologation, owing to its position as the most broadly utilized and mature technique within sustainability assessment. Constituent components within the goal and scope of each assessment strand are tabulated for cross comparison. As critical parts of initial assessment development and definition, this provides new insights into the levels of integration attainable and any residual sources of methodological conflict for future resolution.
3. Review of literature
To evaluate the state of holistic harmonization within sustainability assessment, the three constituent strands are initially examined independently. Each initially emerged as standalone assessment methodologies, yielding the single-faceted approaches found through the literature search. Most of these single stranded assessments focus on general application; however, some have been refined and iterated, producing CCU specific approaches. Guiding principles and key methodologies for each are laid out in the following sections, detailing the foundations upon which later integration efforts rest.
This subsequent examination of the shift toward integration focuses on the harmonization methodologies presented in literature, considering their success or failure in handling and homologating methodological hurdles. Notable areas include assessment structure, boundary alignment, indicator selection and calculation, scenario generation, and handling of assumptions. Common errors seen within the assessment of CCU projects will also be examined; reviewing the effectiveness of mitigation strategies provided by sector specific guidelines.
Assessments focusing on CCU projects necessitate methodological decision making support that is overlooked in general frameworks. For example, a benchmark system must be assessed; a consequence of the disruptive nature of CCU technologies. Further complications arise from the broad range of TRLs observed in CCU, generally spanning levels 3–8, stemming from issues around data availability and inter-assessment comparability.
3.1. Life cycle assessment
Environmental assessments of products predate the use of the term LCA, appearing within literature in one form or another since the 1960's (Guinée, 2012). Since then, large strides have been made as summarized by Figure 2; initially aiming to better define the field, latterly seeking a standardized, robust, and reproducible approach. The 1960s and 1970s see the conception of what would become modern LCA in response to increased public concerns around energy use, resource efficiency and pollution (Assies, 1992). These studies initially focused solely on energy flows (Sundström, 1971; Boustead, 1974; International Federation of Institutes for Advanced Study, 1974). System based approaches to assessment, similar to those now used, emerged in 1974 with a US EPA study on alternative beverage containers (Hunt, 1974); although this methodology was not initially adopted broadly. The 1980s and 1990s exhibit significant methodological divergence, while failing to cultivate meaningful scientific discussion (Guinée, 2012). This ultimately prevented a single methodology from gaining wide acceptance as an assessment tool, primarily due to comparability issues between studies.
Publications including the term “LCA” begin to appear in the late 1990s. Standardization was sought in this period, leading to the convergence of environmental impact studies under this unified umbrella. The number of published articles citing “life cycle assessment” as a key word can be seen in Figure 3. Publication data obtained through literature search demonstrates the increase in application over the early 21st century, exhibiting rapid growth of the field after 2005. Studies from 1960 and 1995 do not appear as the term LCA had not yet been coined.
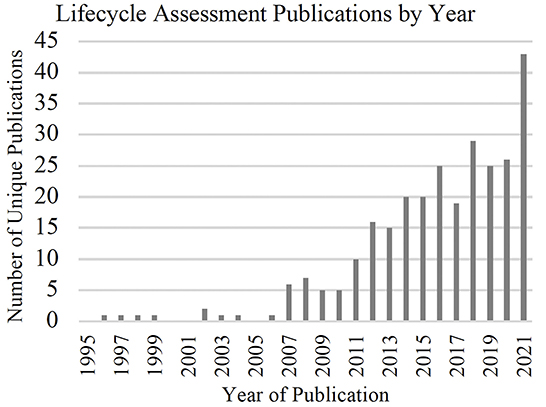
Figure 3. Distribution of LCA publications by year. Data collected using Web of Science, using filters of; author keywords of “life cycle assessment” and year published of “1960–2021”.
LCA in its modern form accounts for the environmental impacts of a product or service throughout its entire life cycle, spanning from cradle to grave; i.e., from raw material extraction through to final disposal. At each stage, the product system interacts with the environment, consuming natural resources and emitting pollutants. Assessments aim to quantitively assesses these interactions and their potential environmental impacts (McCord et al., 2022). Correct application delivers (Muralikrishna and Manickam, 2017):
1. A breakdown of environmental loads to constituent unit operations or life cycle stages; identifying areas for optimisation.
2. Internal comparisons of products or processes.
3.1.1. ISO standards
In 2006 ISO 14040, and the broader ISO 14K series, became the first internationally recognized and standardized methodological framework for LCA. Importantly for topic of this paper, the ISO technical committee recognized the need for parallel techniques assessing economic and societal impacts, an early call for holism. ISO's key achievement was the crystallization of fundamental principles for LCA. These have subsequently been adopted by all significant guidelines and include (ISO Technical Committee, 207, 2006a);
• A life cycle perspective, considering all operations from raw material extraction to end of life treatment or disposal.
• Environmental focus, recognizing the exclusion of economic and social factors from the scope of an LCA; suggesting the implementation of parallel assessments for more extensive studies.
• Relative approach and functional unit, analysis and results based on a quantifiable attribute of the product (e.g., mass, energy content, or function).
• Iterative approach, in which data is refined or revisited based on quality requirements implemented within subsequent stages.
• Transparency of execution and interpretation.
• Comprehensiveness, including all system attributes pertaining to natural environments, human health, and resource use.
• Priority of scientific approach; basing methodological decisions on natural science. Resorting to social and economic sciences only when necessary.
ISO present a four-phase methodological approach (shown in Figure 4, discussed further in Section 3.1.), subsequently adopted as a mainstay within broader sustainability assessment methodologies.
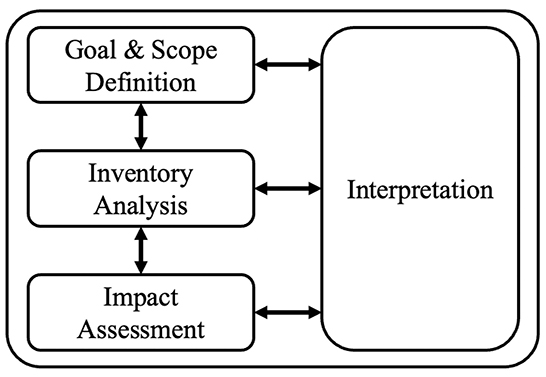
Figure 4. Standardized assessment phases for LCA as developed within ISO 14040 (ISO Technical Committee, 207, 2006a).
The respective components of this general methodological framework are subject to more detailed examination within ISO 14044 (ISO Technical Committee, 207, 2006b), an accompanying standard. This second document prescribes specific methodological requirements and guidance for the correct execution of studies. Many previously divergent aspects within assessments were standardized, reducing inconsistencies around methodological structure and practitioner choices. This aimed to eliminate comparability issues that had previously barred LCA from acceptance as an assessment tool.
Introduction of impact quantification in terms of a functional unit ensures that results are presented in a comparable format based on product functionality. Practitioner guidance around cut-off criteria is also developed, systematically handling gaps in assessment coverage, ensuring results are representative of the real world. While the methodological steps for this procedure are detailed, they lack quantification. Instead relying on the practitioner's good judgement, generating a novel source of study misalignment and comparability issues.
Multiproduct systems represent a historically persistent challenge within LCA. Questioning how burdens should be apportioned within such systems where more than one saleable product is generated. ISO approaches standardization around the issue pragmatically, introducing a hierarchy of handling methods. These are, from most to least preferable; avoidance, physical relationship (e.g., mass), or other relationship (e.g., economic value) (ISO Technical Committee, 207, 2006a). A one size fits all approach is not feasible for allocation, owing to the differing aims of studies and application of products. Recognition of these complex methodological considerations proves to be ISO 14040's strength.
Data collection is widely recognized as a complex and resource intensive procedure in LCA. Helping to navigate this, ISO14044 provides collection sheets for use by practitioners (ISO Technical Committee, 207, 2006b). This assists with the organization of data around given unit operations or life cycle stages, generating inventories for system inputs, outputs, transport distances, etc. Additional benefits are realized through this systematic data handling, most notably improved transparency. Data quality reporting is also scrutinized, requiring improvement where quality is insufficient (or where data is missing); reflecting the iterative approach laid out by the guiding principles. ISO assess quality in terms of 10 factors: temporal coverage, geographical coverage, technology coverage, precision, completeness, representativeness, consistency, reproducibility, source, and uncertainty (ISO Technical Committee, 207, 2006b). This approach invites the use of pedigree matrices to track validity across the factors, resulting in a robust and documented assessment of data quality.
Overall, ISOs 14K series likely constitutes the largest single leap forward in LCA methodology. It delivers broadly applicable and standardized approaches to structure, methodological hurdles (allocation, cut-off criteria, etc.), and fair comparative assessments. However, several key decisions remain at the discretion of practitioners, potentially affecting the integrity of the results and insights generated. Key examples include; selection of indicators and characterization methods, formulation of assumptions, and derivation of system boundaries. In attempts to mitigate these factors many offspring guidelines and frameworks have been developed upon the foundations laid down by ISO 14K.
3.1.2. ISO derived guidelines
Chronologically, the first significant ISO derived guidance is the International Reference Life Cycle Data System (ILCD) Handbook (European Commission–Joint Research Centre–Institute for Environment Sustainability, 2010). Requested by the European Commission, the objective of the handbook was to inform the development of subsequent product specific assessment guidelines, applying current best practices and facilitating more comparable studies. The outcome is consistent, robust, and quality-assured frameworks for assessments of similar products on an equivalent basis, validating ecolabelling claims. Resulting off-spring frameworks are ISO compliant, with the handbook acting as a more granular extension of ISO14044. Structured around Shall/Should/May guidance, the document lays out both required and recommended methodological steps, clarifying procedure for practitioners.
The ILCD Handbook makes progress through the narrowing of methodological choices left to the practitioner via bolstered guidance, reducing subjective influences on results. Two veins of assessment are addressed specifically; decision making (micro, meso, and macro) and accounting. Despite making progress, the handbook fails to tackle some legacy issues within LCA. Quantified rules around cut-off criteria are not developed in any meaningful way, only the reporting of targets (e.g., % reporting completeness) and omissions. Furthermore, the approach to selection of impact indicators is not developed. While these shortcomings are symptomatic of the handbook's broad objective, it prevents the comprehensive closure of many methodological gaps seen within the preceding ISO standards.
As a successor to the ILCD handbook, the European Commission instructed the development of ISO compliant “Product Environmental Footprint (PEF) Category Rules” [European Commission, (n.d.)]; reacting to regular calls for rules around specific product categories (ISO Technical Committee, 207, 2006c; British Standards Institution, 2011; World Resources Institute, 2011). The delivered rulesets develop upon the guidance provided in the ILCD Handbook, generating product specific LCA guidance through pilot schemes. PEF targets enhanced harmonization within product categories; they provide detailed instructions for goal and scope definition, system modeling requirements, and standardized impact assessment categories and characterization approaches (Kerkhof et al., 2017). While mandated impact indicators are desirable for comparability, they significantly reduce methodological applicability; primarily through a reduction in assessable goals and scopes. Despite this, the tightening of methodological procedure delivers notable benefits, such as an aggregated indicator score. However, for harmonized CCU applications, the PEF category rules' rigidity make them challenging to align with economic and social strands. The most likely origins for these issues are data collection and inventory structure. Consequently, the PEF Category Rules are not recommended for use in assessments targeting strand integration. Furthermore, the specification of guidance based on the product means that CCU relevant impact indicators and methodological decisions may be neglected; a consequence of the guidelines needing to accommodate competing, non-CCU, production routes.
The final standalone LCA guidance identified is the GCI's “LCA Guidelines for CO2 Utilization” (Müller et al., 2018). First published in 2018, a revised version was presented in 2022 (Müller, 2022). With LCA's general methodology having been developed significantly in the preceding decade, CCU specific guidance remained lacking. This impaired comparability through practitioners' divergent methodological decisions around factors including system boundaries and functional unit selection. GCI used the ISO standards (ISO Technical Committee, 207, 2006a,b), ILCD Handbook (European Commission–Joint Research Centre–Institute for Environment Sustainability, 2010, 2011), chemical engineering textbooks (Guinée, 2002; Baumann and Tillman, 2004; Curran, 2012; Hauschild and Huijbregts, 2015) and other literature sources (von der Assen et al., 2013, 2014) as their foundation. Again, following the Should/Shall/May format for guidance application, requirements of the ISO standards are adhered to fully. However, specific areas critical to CCU technologies and their deployment are necessarily more methodologically constrained. These include the selection of CO2 source, system boundaries, functional unit selection, and assumptions around utility sources.
CCU LCA results are highly sensitive to differences in system boundary, notably CO2 sourcing. GCI therefore require that the energy requirement and carbon intensity of CO2 capture must be included; tackling the commonly observed pitfall of treating anthropogenically captured CO2 as a negative emission without it's associated burdens (that is, for every kg fed; ) (Müller, 2022). Thus, any post-capture purification, compression, and transportation must be quantified and assessed. Standardization in this area delivers more comparable studies and legitimate impact quantification. An example GCI compliant system boundary for the correct inclusion of CO2 capture is detailed in Figure 5.
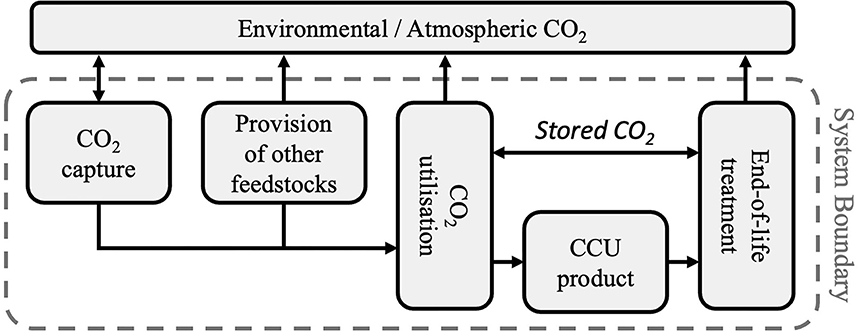
Figure 5. Generic example of correct system boundary for a cradle to grave CCU LCA. Adapted from the Global CO2 Initiative (von der Assen et al., 2013). “Provision of other feedstocks” includes the generation of electricity and other utilities.
Often, the assumptions made around utility provision in CCU assessments are regularly overly optimistic or infeasible. These range from excess green electricity generation to unrealistic grid capacity and resilience scenarios. When examining electricity supply, GCI give a hierarchy of options for assessing environmental burden; ideally utilizing real world (geographically specific) data, followed by representative grid mix, and finally net-zero (Müller, 2022).
Also targeted by GCI is the misalignment of functional unit selection in precursing literature. The result is a flow diagram (Figure 6), prescribing the appropriate functional unit (Müller et al., 2018). A unified approach is achieved, ensuring that products with the same intended application are assessed on an equivalent basis, while also accommodating the broad range of CCU technologies and products. This advancement demonstrates the need for GCI's work on CCU guidelines; disseminating tools for sector standardization that are not possible at a more generalized level due to differing methodological requirements.
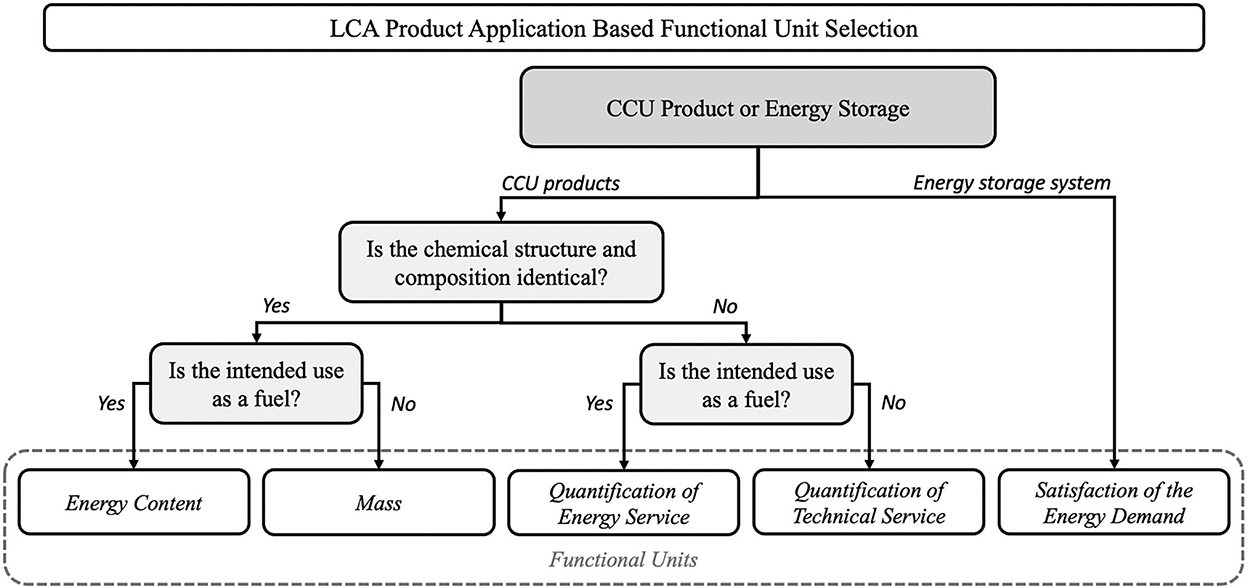
Figure 6. Flow diagram for the identification of the selection of an appropriate functional unit within CCU oriented LCAs. Adapted from the Global CO2 Initiative (Müller et al., 2018).
Examination of the impact assessment approach put forward in the GCI's methodology also reveals movement toward standardization. While a specific set of impact indicators is not mandated, it is suggested that European studies employ those curated by the JRC (European Commission–Joint Research Centre–Institute for Environment Sustainability, 2010; Müller, 2022). However, after indicators are selected, GCI require that CML (Institute of Environmental Science, University of Leiden) (Huijbregts et al., 2016) characterization models are employed. While impact indicators must be left at the practitioners discretion (discussed in Section 4) to allow for varied assessment goals and scopes, standardization of characterization models greatly aids comparability between assessments.
Overall, GCI meaningfully enhance guidance for the application of an ISO compliant LCA methodology within CCU. Major hurdles around practitioner choices have been removed, with steps taken to harmonize goal and scope setting between assessments of comparable products (e.g., functional unit selection and system boundary definition). The result is a strong environmental assessment strand, offering robustness and repeatability. Future work in the area may benefit from the partial standardization of impact indicator selection; potentially with assessment specific additions made at the practitioner's discretion to accommodate broader goals and scopes. Further guidance around allocation procedures would augment assessments, especially given the tendency of CCU processes to generate multiple products; currently the ISO methodological hierarchy is adopted without further development. However, care should be taken to ensure that allocation method selection does not encroach on the range of possible goals and scopes.
Beyond these examined methodologies, the NETL (National Energy Technology Laboratory) provide a US based framework for CCU specific LCA (Skone et al., 2022), developed in parallel to GCI's. However, due to the geographical specificity of the work it self-eliminates from broader standardization efforts. For this reason, it is not discussed further in this review, despite utility in North America.
3.1.3. Current state of LCA in CCU
To summarize the current state of LCA guidance, the field has reached a high degree of both maturity and holism. The ISO standards provided a broad but robust foundation for steady development, pioneering many procedural steps that see adoption in subsequent more specialized CCU guidance. Although some approaches' rigidity complicates harmonization (notably the PEF Category Rules), a clear path to CCU specific assessment can be identified (see Figure 9). The development of LCA can, in many ways, be seen as a cascading set of guidance documents; these hail from the ISO standards and incrementally grow more specific in nature. When pursuing a holistic and harmonized methodology, a trade-off must be managed between flexibility, in the interest of strand alignment, and rigidity to aid comparability.
Comparability has been enhanced through incremental refinements, significantly reducing the “apples vs. oranges” (Zimmermann and Schomäcker, 2017) problem. Furthermore, all current methodologies found in literature utilize a BFD (block flow diagram) approach toward system modeling [European Commission, (n.d.); ISO Technical Committee, 207, 2006a; European Commission–Joint Research Centre–Institute for Environment Sustainability, 2010; Müller et al., 2018; Zimmermann et al., 2020; McCord et al., 2022]. This identifies consequences, or impacts, of resulting environmental interactions via evaluation of material and energy flows crossing the system boundary (elementary flows). The functional units used, and therefore the basis of assessment results, are also standardized through GCI guidance. Impact characterization is universally achieved through the application of published approaches. However, despite the specified use of CML models, the selection of indicators assessed are not yet standardized; identified earlier as a potential area for tentative guidance development.
Beyond this, the application of cut-off criteria is handled non-uniformly, with guidelines failing to detail specified thresholds. This is unlikely to be resolved through future iteration of the guidelines. Their rigid specification would ensure that resulting frameworks are incompatible with many assessment goals and scopes, as exemplified by the PEF Category Rules. In essence, a one size fits all methodology would be inherently flawed.
The presence of life cycle databases such as Ecoinvent greatly supports practitioners, with over 18,000 inventory datasets (Ecoinvent, 2019). However, emergence of such tools necessitates methodological maturity in order provide utility, enabling the standardization of information formats and underpinning calculations. Three primary benefits can be observed as a consequence of its use; practitioner workload reduction, greater impact pathway transparency, and the use of common data inputs between assessments. Without Ecoinvent, LCAs would require significantly longer to complete and exhibit a much lesser degree of comparability.
3.2. Technoeconomic assessment
TEA evaluates the economic demands of research, development, demonstration, and deployment of technologies (SETIS EKRA, 2016; Zimmermann et al., 2018); quantifying the production costs and size of market. Approaches have historically exhibited non-standardized approaches, a symptom stemming from the lack of widely accepted guidelines (Kosan et al., 2016; Zimmermann et al., 2020), general or otherwise. In fact, it has been identified within literature that CCU is an area ripe for TEA standardization (Faber et al., 2021), seeking an answer to the continued “apples vs. oranges” issue around comparability (Zimmermann and Schomäcker, 2017). Furthermore, augmented policy development, decision making, and R&D funding allocation may be realized with such an assessment tool (SETIS EKRA, 2016). This lack of a standardized approach has not hindered the rate of TEA publication, demonstrating the assessments utility, even in non-standard form. Figure 7 shows the yearly number of TEAs observed in literature. Within this data a clear upwards trend can be observed, publication count increases year on year without exception.
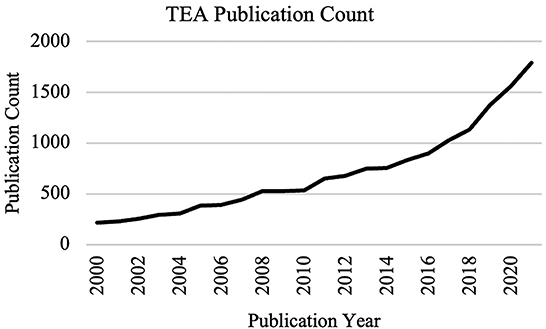
Figure 7. Distribution of TEA publications by year. Data collected using Web of Science, using filters of; author keywords of “technoeconomic assessment”, “techno-economic assessment”, and year published of “2000–2021”.
Assessments are not limited to one life cycle phase, many extend to include the upstream and downstream operations, although the production phase is typically the focus. LCC (life cycle Costing) has historically been used for this on a cradle to grave basis (Hunkeler et al., 2008; Swarr et al., 2011; Sell et al., 2014); with studies carried out around its potential integration to LCA (Swarr et al., 2011; Miah et al., 2017; Dong et al., 2018). LCC typically exhibits a cost driven focus, regularly neglecting technical and profit-based indicators (Zimmermann et al., 2020), inhibiting its application within a decision-making and development context. By instead utilizing TEA, increased scope flexibility aids potential integration with LCA. This wider approach to assessment of economic sustainability facilitates the identification of economic drivers throughout complex value chains, offering significant applicability within both CCU projects and technology development.
Zimmermann et al. identify that TEA assessments of CCU projects did not, at that point (2017), follow a common approach; this included analysis of both government reports and academic papers. The finding was later ratified by GCI, identifying two CCU-relevant methodologies (Sugiyama et al., 2008; Otto et al., 2015). However, after analysis, both were deemed too generic for direct application to CCU cases. Approaches seen in previous literature are broadly limited to those presented in chemical engineering textbooks (Zimmermann et al., 2020); primarily (Peters et al., 2003; Sinnott and Towler, 2009; Turton et al., 2012). Inevitably, utilization of varied approaches prevents meaningful comparisons between studies of similar systems. The application of generalized guidance encounters similar issues to those seen within LCA, divergence in methodological application and assumptions, necessitating both standardization and transparency. Typical pitfalls associated with CCU TEAs are identified; including but not limited to Zimmermann et al. (2020):
• Alignment with LCA methodologies.
• System boundaries.
• Indicator selection.
• Lack of TRL assessment.
• Characterization model application.
• Derivation of CO2 prices.
3.2.1. GCI guidelines
In 2018, GCI released standalone CCU specific TEA guidance (Zimmermann et al., 2018), developed in parallel with their LCA counterpart (discussed in Section 3.1.). These were produced in co-operation with around 50 international experts and informed by a systematic literature review; incorporating contemporary best practices within industry, academia, and policy. The resulting “first of a kind” approach is purposefully harmonized with LCA, taking structural inspiration from the ISO standards (ISO Technical Committee, 207, 2006a,b), and ILCD Handbook (European Commission–Joint Research Centre–Institute for Environment Sustainability, 2010). In addition to these structural developments, GCI deliver practitioner guidance around assessment areas that exhibit high sensitivity around CCU applications. In addition, they call for the development of a new TEA ISO standard This would be parallel to the ISO 14K series, potentially ratifying GCI's methodological approach (Zimmermann et al., 2020). The following section will outline CCU specific guidance from only the GCI work, owing to lack of alternative guidelines. GCI systematically tackle the common CCU TEA pitfalls identified by Zimmermann et al. through the provision of additional methodological requirements and guidance.
The same four phase structure is adopted as seen in LCA (see Figure 4), laying the groundwork for harmonization. This is supported by the original standards recognition of the need for parallel assessment methodologies (ISO Technical Committee, 207, 2006a). Analysis on the basis of a functional unit is also adopted, selected following the same flow diagram seen for CCU LCAs (see Figure 6). Contrary to prior TEA approaches, requirements are laid out for both the goal and scope definition; carrying benefits for both practitioners and comparability (Zimmermann et al., 2020).
Allocation, for multi-product systems, is handled in harmony with the LCA guidelines. ISO's methodological preference hierarchy is adopted; however, with additional scope for practitioners to “follow any principle that ensures meaningful results” (Zimmermann et al., 2020) when the goal or scope requires. This significantly reduces the lack of both standardization and harmonization previously seen around allocation in both general and CCU specific TEA. Given the financial orientation of the strand, it is perceivably of benefit to consider allocation through economic value when conducting stand-alone assessments.
Augmented guidance around the definition of system boundaries results in a significant reduction in the scope of practitioner decisions. However, in contrast to the LCA guidelines, there is a provision for the application of gate-to-gate boundaries. Consequently, R&D, or corporate perspective studies can assess over a single value actors' section of a supply chain, broadening applicability. Capability for cradle to gate and cradle to grave assessments is not impacted, safeguarding subsequent assessment strand harmonization and integration.
GCI refine practitioner support around product systems where carbon capture is employed, a target of the guidelines, defining boundaries using information provided within ISO 27912 (International Standards Organisation Technical Committee 265, 2016) and ISO 27919 (International Standards Organisation Technical Committee 265, 2018, 2021), the standards for carbon dioxide capture; a significant step toward easily comparable assessments. The procedure prescribed for the evaluation of CO2 source is based upon that of the counterpart LCA guidance, this time also accounting for the price of capture. Two levels of granularity are presented for the assessment of capture; selection is based largely on the goal and scope of the assessment. Most preferable is the assessment of a specific capture process and supplier, using primary data. Alternatively, where this is not feasible, an industry or technology average value (secondary data) can be used for technical and economic data points.
The nature of TEA requires data specificity both geographically and temporally; these factors influence employed assumptions and sources of input data. Consequently, guidance is developed to aid practitioners while navigating these aspects of assessment. As with GCI's parallel LCA guidelines, geographical and temporal considerations introduce nuances around the assessment of process inputs, outputs, utilities, and scenario modeling.
Identified as a common source of error within assessments (Zimmermann et al., 2020), derivation of feedstock prices (detailed by Figure 8) and sources are critical to study validity; perhaps the most obvious example within CCU projects is CO2. Prices seen in literature can vary widely. Costs between 5 USD−180 USD per ton CO2 are reported (a small number of sectors exceed this) (Metz, 2005; Zero Emission Platform, 2011; Henriette, 2016). With large quantities used within CCU, importance of correct estimation on an assessment specific basis is clear.
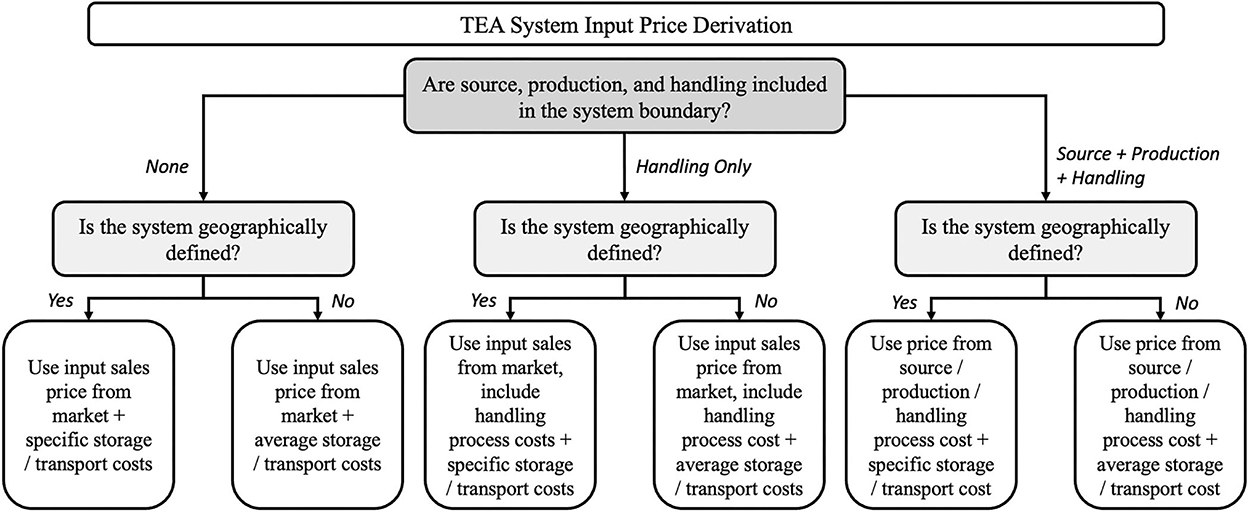
Figure 8. Derivation of commodity input prices as a function of system boundaries. Adapted from the Global CO2 Initiative (Zimmermann et al., 2020).
Clarification of the required concentrations and purification steps is crucial, facilitating selection of a suitable supply and relevant cost estimates. GCI mandate that the lowest concentration source technically feasible should be used (not simply that with the lowest price), while ensuring compatibility with the coupled process(es). This requirement demonstrates the guidelines specialization around CCU. Utilization of the lowest possible grade of CO2 preserves purer sources for other CCU deployments that require them. Furthermore, where the capture is within the system boundary, relevant CapEx, OpEx, purification, compression, and transport costs should be included when estimating CO2 price (Zimmermann et al., 2020). Where capture is excluded from the system boundary, literature should be used to estimate cost. Average prices should be obtained by consulting reports detailing specific capture technologies or sources; GCI detail multiple useful governmental and organizational data sources. Provisions are made for studies with varied geographic specificity, suggesting the use of prices at the highest degree of spatial accuracy available. Typical pitfalls around CO2 costing identified in literature include (Zimmermann et al., 2020):
1. Assumption of zero cost.
2. Assuming ETS (emissions trading scheme) price or emissions tax as the full procurement cost.
3. Use of GHG (greenhouse gas) abatement cost as a proxy for capture cost.
Such pellucid methodological guidance around a highly sensitive variable in CCU's economic performance significantly aid comparability. Furthermore, it safeguards assessments from the assumption of overly optimistic scenarios, result, and conclusions.
GCI recognize the importance of assessment scenario development within TEA. Large amounts of practitioner guidance are provided relative to that seen in LCAs (typically less sensitive to market, spatial and temporal factors, excluding grid mixes). Measures extend to requiring the adoption of a H2 production method that has reached a TRL of 9, indicating a mature and viable route [such production methods can be identified within Ullmann's Encyclopedia of Industrial Chemistry (Häussinger et al., 2011) and HIS Markit's Chemical Economics Handbook (IHS Markit, 2021)]. This ensures that overly optimistic scenarios are not used to make the assessed system appear more favorable across the selected indicators. The primary benefit seen through this bolstered guidance and methodological constriction is the mitigation of typical practitioner pitfalls surrounding the assessment of hydrogen pricing seen within previous TEAs (Zimmermann et al., 2020):
1. Assuming all low TRL production routes will reach technological maturity.
2. Economically favorable yet environmentally burdensome production (negating the proposed benefits of CCU).
3. Basing production on the utilization of intermittent electricity inputs. Omitting associated trade-offs such as repeated start-up and shutdown mechanisms, CapEx, and OpEx.
Pricing of electricity, a significant input to many CCU processes, is also examined. It is determined that full production costs should be evaluated, eliminating a previously major source of system boundary divergence in TEAs of the sector. If necessarily excluded from the system boundary, GCI state that market data must be used to avoid the assumption of a zero-input price (as seen in some TEA studies) (Zimmermann et al., 2020). The guidelines present several literature sources for electricity pricing: Eurostat's energy database [Eurostat, (n.d.)] for European cases, and EIA [US Energy Information Administration, (n.d.)] database (API Open Data) for North America. CCU TEAs for low TRL processes have previously made assumptions of zero or negative electricity prices, complicating literature comparisons. Furthermore, GCI identify that changes in relevant input markets should be accounted for (e.g., commodity prices, competing technology or regulatory differences); a practice not seen within the counterpart LCA guidelines. Returning to the previous example of CO2 procurement, prices are relatively uncertain as a result of these market forces; representing a common source of errors in literature assessments.
Within precursing CCU TEAs, indicator selection is far from standardized, often with multiple alternatives used for the same criterion (Zimmermann and Schomäcker, 2017). GCI identify TRL, OpEx (operational expenditure), and CapEx (capital expenditure) as frequently employed indicators. However, the guidelines note that their definitions and characterization methods differ greatly (Zimmermann et al., 2020). This is rectified within the developed TEA guidelines through provision of standardized definitions and calculation approaches. For both OpEx and CapEx calculations, differing approaches are suggested based on process TRLs, accounting for respective differences in data availability and uncertainty. If the goal and scope target more specific insights, additional indicators can be appended at the practitioners discretion utilizing previously noted textbooks (Peters et al., 2003; Sinnott and Towler, 2009; Turton et al., 2012), delivering valuable assessment flexibility.
3.2.2. Current state of TEA in CCU
Despite reliance on a single guidance document, notable progress has been made around CCU oriented TEAs. GCI's guidelines represent the first attempt at sector specific guidelines, strengthening many of the weakest methodological links. Making use of the ISO standards as a template, the developed framework is successfully harmonized with LCA, realizing the benefits of transparency and an iterative approach. Major assessment stages are subsequently congruent with the LCA methodology, facilitating integrated studies. Key aspects of application to CCU are addressed, primarily through enhanced practitioner guidance around the setting of boundaries around CO2 sources, generation of assessment scenarios, and the estimation of key input prices. These highly sensitive factors in the economics of CCU had not previously been assessed robustly. Compounding this, the suggestion of data sources for sensitive inputs should further improve homologation between studies. While data sources are available, TEA would significantly benefit from practitioner friendly databases comparable to Ecoinvent within LCA, providing support for the derivation of input and utility prices.
Despite this progress, the non-uniformity of impact indicators assessed still threatens inter-assessment comparability. However, the prescription of functional units as well as characterization models for commonly utilized indicators will partially alleviate this issue; effectively “shepherding” practitioners into using the same approach. Additionally, GCI do not list policy makers as a stakeholder group within the guidance document, a divergence from LCA. This may prove to be a limitation given the criticality of policy decisions on the development of emerging CCU technologies.
3.3. Social impact assessment
The third and final assessment strand examined in isolation is SIA (also commonly referred to as S-LCA). As the youngest and least standardized of the “strands”, social assessments represent an area of growing interest and relevance (Klöpffer, 2003; Jørgensen et al., 2007; Pollok et al., 2021).
SIA primarily focusses on corporate social responsibility, and can be defined as; “the process of identifying the future consequences of current or proposed actions, which are related to individuals, organizations and social macro-systems” (Becker, 2001; McCord et al., 2021). Vanclay (2003), proposes that any developed guidelines should be derived from core values and principles (Vanclay, 2003) such as the UN SDGs (United Nations, 2015a). When considering comparability of assessments, Pollok et al. (2021) notes that while the ISO format is generally accepted within social assessment, it is not implemented rigorously. This often results in major divergences and complicates the harmonization and subsequent integration of assessment strands.
Historically, the integration of social factors within engineering assessments has been recognized as challenging. The development of SIA methodologies is chronicled in a review by Huarachi et al., defining four distinct phases (summarized in Table 1). The final phase, fittingly for this review, is “the search for standardization”. Beginning in 2017, the endeavor is ongoing, tackling the methodological divergence exhibited within “the development years”. Despite this continued lack of standardization, the 2010s saw the emergence of SIA into the mainstream of sustainability assessment.
While many publications have evaluated the social acceptance of CCU, a majority reporting favorable community perceptions (Jones et al., 2015, 2017a,b; van Heek et al., 2017; Offermann-van Heek et al., 2020), the need for an impact assessment is highlighted in multiple works (Zimmermann and Schomäcker, 2017; Pieri et al., 2018; Pollok et al., 2021). Zimmermann et al. identify a general absence of SIA assessments within low TRL technologies. This gap in literature must be closed if meaningful SIAs are to be achieved around CO2 utilization. As of writing, no standalone CCU specific SIA methodology has been proposed; with one framework for integrated LCA/TEA/SIA developed by McCord et al. (2021) (discussed in Section 3.4.3.). Despite this, some sector orientated approaches have been discussed in literature for other fields, validating the concept; examples include minerals (Azapagic, 2004) and mining (Mancini and Sala, 2018).
3.3.1. Methodological approaches
Social impacts are most commonly reported relative to the UN SDGs (Sustainable Development Goals; United Nations, 2015b) or GRI (Kühnen and Hahn, 2017; McCord et al., 2021; Stiching Global Reporting Initiative, 2021; Global Reporting Initiative). However, these are typically carried out around deployed operations rather than during R&D (Zimmermann and Schomäcker, 2017; McCord et al., 2021), a problematic characteristic considering the low TRL nature of many CCU processes. Failure to assess social sustainability at early project stages may lead to development and investment in socially unsustainable technologies. Beyond the UN and GRI approaches Kühnen and Hahn (2017) identify;
1. UNEP (United Nations Environment Programme) and SETAC (Society of Environmental Toxicology and Chemistry) S-LCA guidelines.
2. SAI (Social Accountability International) SA 8000.
3. ISO 26000.
The UNEP and SETAC S-LCA guidelines are the most promising stand-alone methodology with respect to the harmonized integration of the three assessment strands. This is largely owing to the influence of ISO 14K on their development, delivering a process modeling approach to data collection and impact identification (UNEP, 2020). The guidelines utilize the same four methodological phases introduced by ISO 14040 (Figure 4), supporting harmonization by providing a common skeleton for assessments. The first iteration of the guidelines was published in 2009 (UNEP, 2009), receiving updates through a second version in 2020 to reflect progression within the field. These updates included more detailed methodological guidance, applicability to a wider audience, and attempted resolution of diverging approaches.
Impact indicator application within UNEP and SETAC's methodology follows an analogous approach to most LCA and TEA studies. Potential impacts arising from a project are identified through mid-point indicators. This reduces uncertainty but is necessarily less case specific than the end-point indicators available in LCA (although these are scarcely used) (Finnveden and Potting, 2014). However, deviating slightly from the methodologies developed for LCA and TEA, indicators are identified through a hierarchical approach. First, stakeholder groups are identified [aligned with the UN SDGs (United Nations, 2015b)]: workers, local community, value chain actors, consumers, children, and society. Notably, practitioners can exclude one or more of these groups if permissible by the assessment's specific goal and scope (UNEP, 2020). Within the stakeholder categories, potential impacts are identified, constituting “sub-categories”. Each of these can be quantified using several indicators; interestingly, the use of more than one indicator per sub-category is permitted (UNEP, 2020). This approach to indicator selection does ensure that studies utilize a common “indicator pool”, achieving a similar level of standardization to GCI's TEA guidelines. However, the use of multiple indicators in a single sub-category, or exclusion of others still provides scope for issues, primarily around consistency and comparability between assessments.
Two majorly contrasting schools of thought emerge regarding impact characterization within social assessments. The reference scale approach, and the impact pathway approach (UNEP, 2020). Reference scale assesses the social performance of a product system through the practices of organizations, examining effects on impact categories. This approach does not typically look at long term effects, a potential limitation in many applications. Conversely, the impact pathway approach, employed by UNEP/SETAC, uses casual, correlation or regression-based relationships between the product system or organization and social impact indicators (UNEP, 2020). For methodological harmonization, the impact pathway approach, involving generation of a process model and data inventories, is the most likely candidate for adoption. This is primarily a result of it being more analogous to the methods seen within LCA. Furthermore, the inventory is assessed with respect to potential social impacts at the mid-point level through characterization models. The lack of comparable characterization methods within the competing reference scale approach makes its homologation to LCA and TEA a significant challenge.
3.3.2. Current state of SIA in CCU
The progress made toward the standardization of social impact assessments is visible but still lacking. The EC JRC independently reviewed S-LCA/SIA, determining that it trails LCA significantly in terms of methodological development and standardization (Sala, 2015). In addition, Huarachi et al. (2020) also conclude that SIA cannot be considered a mature field until further development and case study applications are conducted. Furthermore, CCU specific guidelines still prove elusive beyond the integrated framework developed by McCord et al. (2021) (discussed in Section 3.4.3.). Significant progress is required in multiple areas if a standardized approach is to be attained.
Within SIA, qualitative, semi-quantitative, or quantitative data and indicators are present (Jørgensen et al., 2007; Chhipi-Shrestha et al., 2015), constituting a significant misalignment relative to LCA and TEA approaches. The presence of mixed data types introduces significant complications, primarily around data collection and impact characterization. These issues are only exacerbated when assessing lower TRL systems, as noted by Rafiaani et al. (2019). Quantification of qualitative data should be attempted using expert opinion, focussing on identified areas of importance (Rafiaani et al., 2019). Using scoring criteria, this qualitative data can then be applied to a numeric scale, as proposed by McCord et al. (2021). However, this naturally incorporates bias and uncertainty, resulting in a secondary issue. While a metric is derived for the application of a scoring scale, a degree of consistency and transparency will be lost, particularly around inter-assessment comparisons. However, this issue may be surmountable if scoring scales can be standardized on a product or sector specific basis.
The consideration and quantification of positive social impacts is another emerging issue within SIA literature (Huarachi et al., 2020). Both LCA and TEA inherently quantify positive impacts (e.g., negative GHG emissions or improved OpEx), requiring resolution for full strand alignment. Importantly, organizations stand to benefit from the inclusion of positive impacts, incentivising further improvements beyond mere legal requirements. Despite this, as of 2020, little progress has been made toward a consensus on the handling of these positive impacts. However, as pointed out by the UNEP/SETAC guidelines, several impact subcategories imply their consideration (UNEP, 2020). One of the major hurdles to overcome is the classification of what constitutes a positive impact. A unified view on this question is a prerequisite to a standardized assessment approach.
To conclude, the evident lack of CCU specific guidance risks leaving complex methodological decisions, such as the specification of assessment boundaries, at the discretion of the practitioner. The GCI integrated TEA and LCA methodology (discussed in Section 3.4.2.) should be consulted to attain guidance vicariously when practitioners are confronted with these choices.
3.4. Current integration efforts
Publications proposing integrated assessments are observed from 2007 onwards; however, recognize a deep lack of consensus around methodological approach (Remmen et al., 2007; Kloepffer, 2008). Tackling these misalignments is a complex proposition, often requiring a trade-off between scientific accuracy and pragmatic decision making support (Buytaert et al., 2011). Such assessments have potential to support a broad range of stakeholders in balancing conflicting goals and positions (Faber et al., 2021). Additionally, they deliver a deeper understanding of the economic, environmental, and social trade-offs (as highlighted in Section 1). In 2017, (Zimmermann and Schomäcker, 2017) recognized the need for a holistic CCU specific assessment methodology, stating that attainment of such guidelines would allow the systematic evaluation of emerging technologies; ultimately delivering more effective funding allocation and R&D efforts. Specifically, combined assessment could answer broader questions: is better performance in one dimension worth an offset in the other? And how much better does the performance in one dimension have to be to offset poorer performance in the other?
3.4.1. GRI standards
Aiming to collate the global “best practices” for public sustainability reporting, the GRI standards (Stiching Global Reporting Initiative, 2021) can be viewed as a sustainability audit. While considering all three pillars within sustainability, they offer significantly less granular results than many guidelines and standards discussed previously. GRI's standards aim to quantify “the most significant impacts” associated with operation (Stiching Global Reporting Initiative, 2021), indicating that less coverage will be achieved compared to assessments utilizing cut-off criteria. Furthermore, as identified by Zimmermann and Schomäcker (2017) they target evaluation of deployed activities, assessing projects based on real world (deployed) and internal organization data. This requirement prevents their use in assessments of early-stage projects owing to the inherent inability to evaluate potential impacts.
GRI take a differentiated approach from ISO's standards, contrasting GCI's homologated LCA and TEA standards. The process model based approach is not present, despite underpinning a majority of methodologies. Instead, identified sources of impacts are grouped under “material topics” (e.g., water and effluents, anti-corruption, etc.); although, no standardized approach is outlined for their selection. These material topics are loosely comparable to the impact categories seen in other assessment strands. Impact indicators, as used by ISO and GCI, are replaced by “reporting metrics”. The relevant metrics for assessment are self-determined by the organization and practitioner, introducing potential for manipulation. Secondly, the number of metrics available for use is vast, significantly hindering comparison between organizations or their technologies. Consequently, resulting assessments may examine the same impact but employ differing quantification metrics. Some similarities to the ISO and GRI approaches can be observed. For instance, a shall/should/can approach is taken to guidance provision, delivering comparable flexibility of application.
Overall, the GRI standards provide a generally robust methodology. However, owing to a variety of reasons, it is not well suited for application to CCU. Assessment of early-stage projects is the largest obstacle. With many CCU projects residing at lower TRLs, a framework that targets deployed technologies or operations is incompatible. Furthermore, CCU requires the assessment of potential impacts, necessitating a degree of uncertainty in the absence of real-world data.
3.4.2. GCI integrated TEA and LCA
GCI, having produced stand-alone CCU based LCA (Müller, 2022) and TEA guidelines (Zimmermann et al., 2020) (discussed in Sections 3.1.2 and 3.2.2., respectively), subsequently targeted integration. This double stranded thinking delivered the GCIs Integrated TEA and LCA Guidelines, first published in 2018 (McCord et al., 2018; Verhoeven et al., 2018). These were updated in 2022 along with the stand-alone guidance documents (McCord et al., 2022). No other accepted combined LCA and TEA methodologies were found through literature search. Preceding studies instead utilized ad hoc approaches (McCord et al., 2018).
GCI approach harmonization by aligning the goal, scope, boundaries, and assumptions of the individual strands; producing an assessment that is greater than the sum of its constituent parts (McCord et al., 2021). While integration is achieved, GCI ensure that the constituent LCA is still both functional as a standalone assessment and ISO compliant. In this approach, the goal and scope phases are unified, with inventory generation and impact analysis conducted in parallel. However, overlapping data inventories permit common data points, reducing the intensity of data gathering while simultaneously improving consistency (McCord et al., 2022). This is reinforced by the progress made around CCU specific system boundary specification; targeting pitfalls identified within previous assessments. A key example being the realistic evaluation of CO2 sources and procurement, a common source of errors leading to overly optimistic assessment outputs.
With the aggregation of the LCA and TEA guidelines, comprehensive integration guidance is now available for practitioners. When compared to ISO 14040 / 14044 or the ILCD Handbook, many methodological decisions have been standardized, unburdening practitioners while improving both reproducibility and validity of results. Despite this, numerous key aspects remain at the practitioner's discretion. A significant example is the selection of impact indicators. It is acknowledged in Section 3.1.2. that lacking standardization here is to ensure broad applicability of the guidelines to CCU projects. However, the challenge remains to define a core set of indicators upon which additions can be made. Integrated assessments add the novel capability of assessing combined indicators, expanding the range of possible insights. Many of these prove to be useful within CCU assessments, with cost of carbon avoided seen regularly within discussion of CCU projects.
The GCI CCU TEA guidelines bring with them the standardization of many indicator calculation methods, analogous to characterization models developed for LCA. The harmonization of these approaches greatly aids their integration. Historically, this has been a stumbling block to the comparability of TEAs from literature. Attention has also been paid in this area to the broad spectrum of TRL levels observed within CCU. Prescription of alternative calculation methods for technologies at varying degrees of development aids with the difficulties experienced around data availability. The use of contrasting calculations does invalidate comparisons of technologies at different development stages; however, these were already problematic owing to non-uniform development and optimisation efforts.
Offering CCU specific guidance, GCI have advanced best practice within combined LCA and TEA. The result is a highly robust yet broadly applicable methodology, ensuring applicability to all goals and scopes. Where such double stranded assessments are necessitated (e.g., applications for financial capital), it should be considered the benchmark. Furthermore, the case studies provided, along with the Should/Shall/May guidance provision, facilitate use by relatively inexperienced practitioners.
3.4.3. Triple helix framework
Published in 2021, the Triple Helix Approach developed by McCord et al. (2021) is the first CCU guidance to consider all three strands of sustainability in parallel. This is realized through the development of an SIA strand that is compatible with the existing ISO homologated TEA and LCA guidelines; delivering a well-integrated assessment when performed together. Given their already advanced state and CCU focus, the GCI's Integrated TEA and LCA guidelines are an ideal foundation for the addition of the third SIA strand.
In contrast to most social assessment methodologies, the framework aligns the SIA's focus around the process and its deployment rather than the organization itself (impact pathway approach). The proposed methodology is recognizably based on the UNEP/SETAC guidelines, providing a degree of alignment with ISO's LCA standards. (ISO Technical Committee, 207, 2006a). Consequently, the four phases seen in LCA and TEA (Figure 4) are once again employed.
Data collection is recognized as a major hurdle for social assessment. McCord et al. (2021) structure the methodology around the use of open-source data, facilitating use by a wider practitioner base; recognizing that utilization of organizational data makes assessment impossible for outside third parties. Due to the low TRL of many CCU projects, data is often unrepresentative of the deployed iteration of the process. Despite supporting supply chains often being fully developed or deployed, they are regularly opaque, making the tracing of materials to extraction a challenge. Consequently, SIA indicator calculations usually yield “fuzzy” results with significant degrees of uncertainty. However, insights into the surrounding potential social impacts remain attainable. Much of the required data is shared with the parallel LCA and TEA (mass and energy balances, workforce requirements, etc.), reducing practitioner workloads. Additional data is required in the form of geographically specific data evaluating indicators such as child labor and forced migration.
Developing a novel approach, McCord et al. determines key indicators for CCU social assessments; and approaches to their calculation. The framework adopts the UNEP/SETAC selection process, although removes several stakeholder categories due to reduced relevance within CCU. The presence of qualitative data often hinders the application of characterization models to generate quantitative indicator results. Consequently, an approach is developed for the conversion of this qualitative data to quantitative. In the triple helix framework, practitioners derive a scoring scale for each indicator, requiring experience and knowledge when assessing the system. Alternatively, indicators may be quantified by using world ranking statistics for the geographical area. A five- or nine-point scoring scale is recommended to ensure the correct level of granularity and differentiation (McCord et al., 2021). While practical, this approach carries some risk of inconsistent scoring, resulting from the degree of practitioner involvement. Furthermore, there must be consensus around whether a high or low score is favorable; this must be uniform across a given assessment. Despite employing these practices, many indicators evade expression in terms of a functional unit, presenting a persistent issue around the integration of assessment strands.
The indicators present within integrated assessments generate potential conflicts. For example, high wages would be positive from a SIA stance, yet detrimental from a TEA perspective (Sala, 2015). Opposing views are present on whether this constitutes a methodological issue. While making optimisation difficult, particularly with respect to deployment scenarios, it facilitates greater understanding of inter-strand performance trade-offs; one of the primary motivations for the development of integrated assessments. (McCord et al., 2021) This characteristic of combined assessments if flagged within the triple helix but the addition of conflicting indicators is not discouraged, helping to reflect complex real-life impact interactions.
In summary, while offering a significant advancement for CCU SIA, several methodological issues persist; primarily concerning standardization. The most notable example is calculation of impact indicator results. Despite offering a method of quantification, the scoring scale approach prevents comparisons between assessments in literature. The issue is complex to resolve due to the breadth of assessment goals and scopes. However, if the scales for commonly employed indicators can be standardized, potentially using world ranking data, the comparability difficulties may be alleviated. Given the timeframes required to reach and adopt accepted characterization models in both LCA and TEA, it should be expected that such approaches are multiple years away for SIA.
Guidance is also lacking around the specification of system boundaries. Developed to be compatible with the GCI combined TEA and LCA guidelines, the SIA boundaries should be defined accordingly. However, social impacts are, in many ways, more complex than their environmental or economic counterparts. The introduction of a new process plant or supply chain to a region may affect a plethora of peripheral stakeholders, acting through mechanisms such as access to energy or material goods. Further guidance around boundary rules or cut-off criteria would aid resolution.
The infancy of CCU SIA and the associated methodological shortcomings are recognized as a significant hurdle by the triple helix framework (McCord et al., 2021). It is proposed that the framework receive further development through repeated application and refinement. This first iteration is deliberately broad, allowing for further adaptation around specific CCU needs. Given that development targeted a “framework”, it could be argued that the provision of further practitioner support would result in a transition toward guidelines. Despite the advantages of this wide applicability, it does result in the need for significant practitioner experience and expertise, particularly around impact analysis. Significant further development is need in the CCU space to remove this barrier and encourage widespread use.
Despite unavoidable limitations within the first iteration of an assessment framework, multiple insights were revealed. The most significant being the identification of raw material sourcing as a key hotspot for social impacts around CCU projects (McCord et al., 2021). Secondly, cascading impacts associated with the use of large quantities of renewable energy; for instance, green H2 production often significantly impacts the surrounding community and society. Recognition of these broader impacts will be critical in the examination of Power-to-X processes within CCU, ensuring a “just transition” toward sustainability.
4. Discussion
Critical review of the current literature around CCU assessment reveals that the Triple Helix framework, in conjunction with the GCI guidelines, delivers the greatest degree of both holism and harmonization. Released in 2021, a 14-year gap can be observed between the first calls for a harmonized methodology and its realization. The successful alignment of the social strand with GCI's ISO compliant combined LCA and TEA delivers a first of a kind sector specific triple stranded approach.
Progress toward a harmonized and holistic approach to CCU specific sustainability assessment can be charted through examination of the guidelines identified. When viewed chronologically, this generates a “taxonomy” (Figure 9), detailing the lineage from which the triple helix approach hails. Of clear significance is the dependence of all strands on the ISO 14040 / 14044 standards. These documents have come to form the backbone of most subsequent approaches primarily in the form of assessment structure (Figure 4).
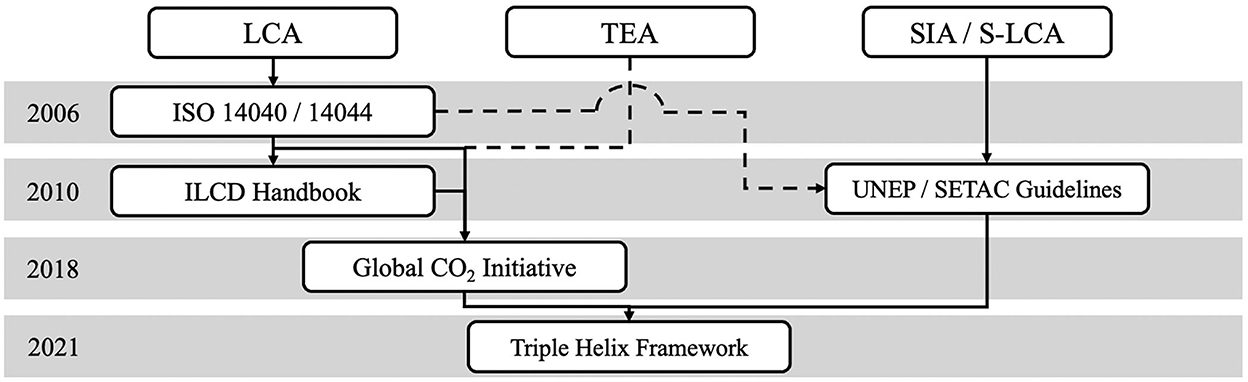
Figure 9. Taxonomy of standards, guidelines, and frameworks leading to a holistic environmental assessment framework for CCU applications. This figure excludes contempory methodological developments that were not directly utilized.
While the taxonomy omits standards, guidelines and frameworks that do not directly contribute to the triple helix framework, the bias of methodological development toward LCA is clear. This is an unsurprising discovery given its status as the oldest assessment strand, hailing to the 1960s, (Guinée, 2012) and the only strand with an ISO standard. The first attempt at TEA standardization is both CCU sector specific and surprisingly recent, published in 2018 (Zimmermann et al., 2018). While TEAs have been conducted on scale (as shown by Figure 7), lack of standardization is surprising given their ability to identify economic efficiency gains. It can be seen from TEA's representation in Figure 9 that the absence of standards is not necessarily indicative of a low count of assessment applications. SIA is found to be the least developed strand, as other non-CCU specific reviews have concluded (Klöpffer, 2003; Jørgensen et al., 2007; Zimmermann and Schomäcker, 2017; Huarachi et al., 2020; Pollok et al., 2021); implying that the observed phenomena is inter-disciplinary in nature. A first attempt at CCU specific SIA guidance was absent until 2021, with standardization of application still lacking.
The triple helix approach provides practitioners with guidance on the application of SIA within combined assessments, the UNEP / SETAC guidelines (UNEP, 2020) can be seen to provide a more appropriate degree of standardization, despite their generality. For this reason, it will subsequently be considered the best practice in SIA, particularly for full studies concerning final selection of CCU projects. The TEA strand is best represented in the field by GCI's guidelines (Zimmermann et al., 2020), owing to its sector specificity, ISO compliance and structural harmonization.
The degree of overall harmonization achieved around holistic sustainability assessments is therefore assessed based on the GCI's LCA and TEA guidelines (Zimmermann et al., 2020; Müller, 2022), and UNEP / SETAC's SIA guidelines (UNEP, 2020). Given their homologation to the same four phase assessment format (detailed by Figure 4), the constituent components within their goal and scope requirements can be compared. The findings are shown by Tables 2, 3.
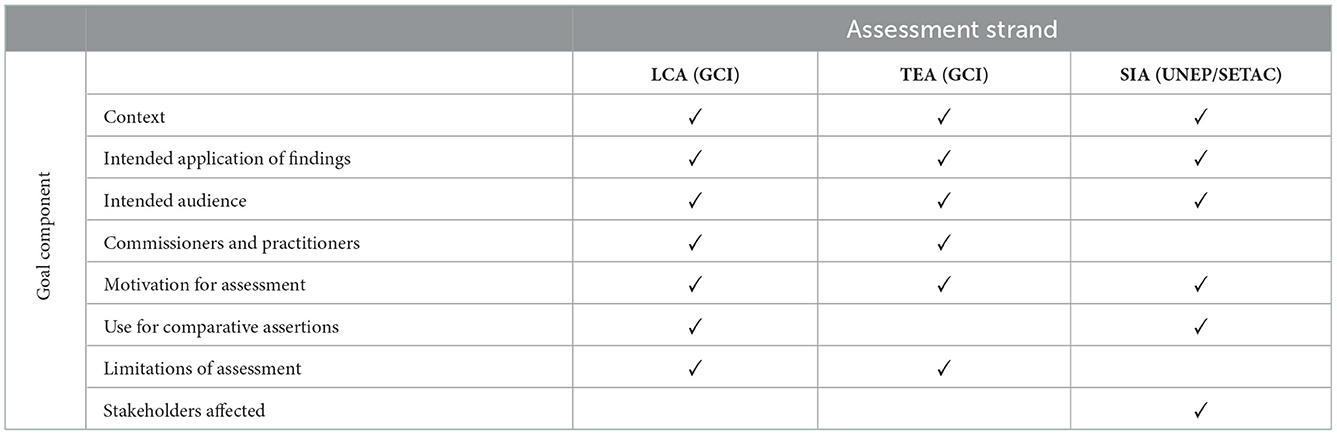
Table 2. Goal requirements for independent strand assessment methodologies (UNEP, 2020; Zimmermann et al., 2020; Müller, 2022).
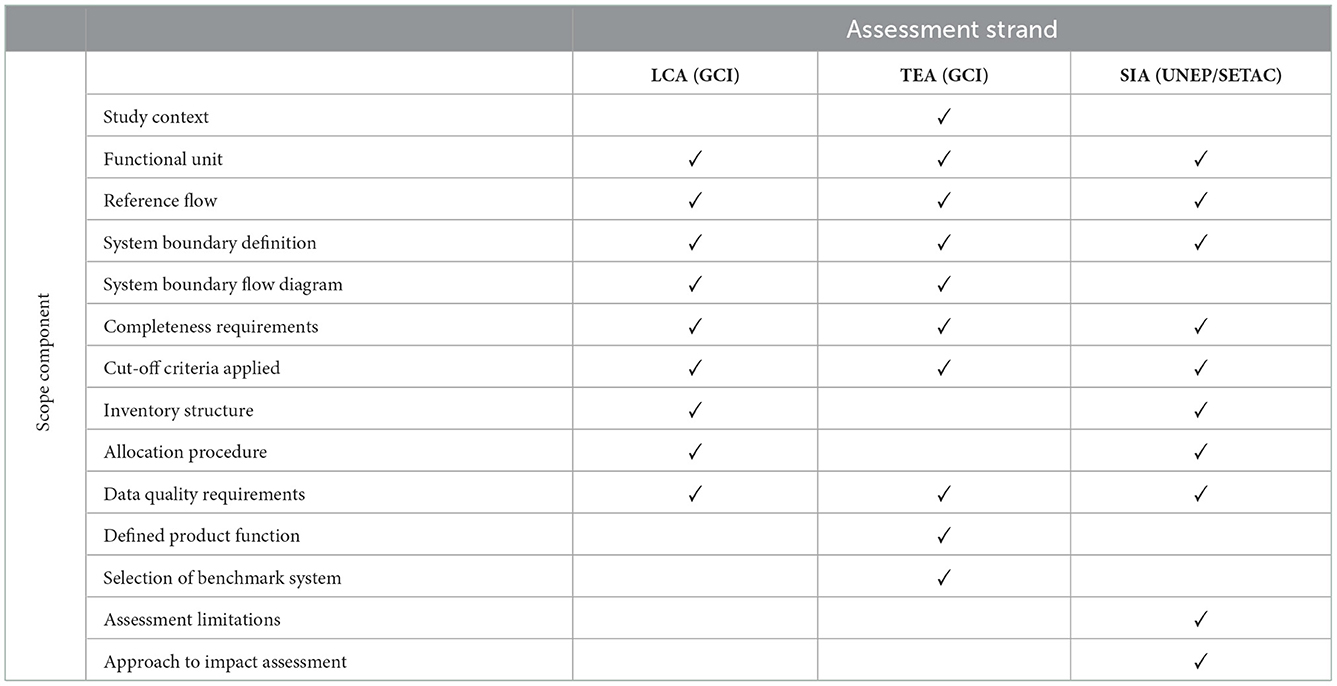
Table 3. Scope requirements for independent strand assessment methodologies (UNEP, 2020; Zimmermann et al., 2020; Müller, 2022).
As shown by Table 2, GCI's guidelines for CCU based LCA and TEA assessments are almost fully aligned. The only exception to this is the requirement to state whether the study will be used for comparative assertions. Clearly, this will not be problematic if incorporated within a TEA assessment, thus facilitating total alignment of study goals. Comparative assertions are less common within TEA studies as their application is usually for internal commercial use, incorporating sensitive data that prevents external communication. However, the context of the TEA must still be covered, giving insight as to whether the study is comparable to others, this details the assessed location, time horizon, scale, and commercial partners (Zimmermann et al., 2020).
Once again using GCI's LCA guidelines as the benchmark, the SIA strand can be seen to exhibit a moderate degree of alignment. The requirement to state the stakeholders affected reflects the strands interest in human societal impacts. In contrast to LCA and TEA, the stakeholders affected are not limited to the commissioner and intended audience. Furthermore, the limitations of a given study are not defined within the goal. Instead, details these reside within the assessment's scope. Due to their inclusion, albeit elsewhere, the SIA strand is only deemed to be partially misaligned in this respect.
Unanimously, the assessment scopes contain more information than the goals; a legacy of ISO 14044. As a partial consequence, a lesser degree of alignment is observed. As the most established and employed assessment type, LCA will once again be used as the benchmark for comparison.
LCA and TEA are largely aligned, sharing ~78% of the components present in LCA's scope, with TEA exhibiting two additional requirements. The primary being definition of a benchmark system, arising from the typical application of TEA to assess the benefits of a process or system against an existing or deployed one. LCA studies are regularly conducted without a benchmark system, aiming to quantity potential impacts of a technology in isolation. For combined assessments a benchmark should be encouraged to maintain the level of insight generated by standalone TEAs. Other discrepancies are present, including specification of allocation procedures, definition of product function and inventory structure. For combined LCA and TEA, these are relatively easily aligned, often with no alteration to methods. However, the selection of allocation procedure may be impacted by integration, requiring a harmonized approach. This may result in a compromised strand, caused by selection of a locally non-ideal allocation approach. For example, inclusion of TEA is likely to favor economic allocation, a less desirable option within LCA; as confirmed by ISO 14044's hierarchy (ISO Technical Committee, 207, 2006b).
SIA shares ~89% of the scope requirements present for LCA, a surprising amount given the strands lagging maturity. The only component not mirrored from LCA's scope is the definition of a system boundary flow diagram, required by LCA and TEA. This is a result of the inherently qualitative nature of social assessment and uncertainties within the impact chain. Despite this, the parallel presentation of a system flow diagram is not a source of incompatibility, potentially further supporting the identification of social impacts. The additional aspects required are assessment limitations and the approach to impact assessment. Limitations are covered within the goal for both LCA and TEA. For a combined assessment this is easily resolved through aligning the positioning of limitation evaluation. The second and more complex addition is the specification of an approach to impact assessment. As discussed in previous sections, the characterization models seen within other assessments, particularly LCA, are absent from SIA. This leaves much broader methodological scope for the quantification of impacts. The triple helix approach (McCord et al., 2021) offers perhaps the most robust approach to the handling of qualitative data. However, still elevates uncertainty relative to the two other strands.
Moving away from the requirements of goal and scope setting, the more general state of alignment can be considered. The three emerging assessment methodologies, examined for Tables 2, 3, utilize fully harmonized assessment structures; based on ISO. Critically, this enables the further alignment of constituent aspects for each phase. TEA and LCA are easier to homologate, owing to their sole reliance on quantitative data, generating the already noted challenge for SIA alignment. While constituent assessment phases are now common across all three strands, the methods employed within them vary, sometimes significantly.
Indicator characterization is the largest source of divergence within integrated assessments. The level of standardization achieved follows the trend of general strand maturity. LCA provides well defined indicators and standardized characterization models (Huijbregts et al., 2016; Universiteit Leiden, 2016; US EPA., 2021), delivering comparable methodologies even in the absence of aligned boundaries or assumptions. TEA is less refined. However, GCI deliver CCU specific guidance on the characterization of commonly used indicators (McCord et al., 2022). The recommended methods are stratified in accordance with TRL levels, preventing comparison between TRLs but augmenting accuracy as technology maturity increases and data quality improves. This is, in most cases, a favorable approach due to the complexity of comparing differing TRL technologies; usually requiring the application of learning curves and highly uncertain scale-up considerations.
Overall GCI deliver a largely analogous approach to LCA, incorporating current best practices throughout the TRL range. In contrast to this, UNEP/SETAC provide a significantly less developed approach. While outlining suggested indicators, there are many more than are observed in other strands, spanning a total of 30 categories (UNEP, 2020). Furthermore, their characterization evades standardization, hindering sector wide and comparable methodologies. This is largely caused by the presence of mixed data types; a problem that is partially resolved by the triple helix framework (McCord et al., 2021). However, despite its application of scoring scales for indicator quantification, they must be standardized for satisfactory resolution of the problem.
Precursing this, the selection of impact indicators for evaluation is another common source of divergence between otherwise comparable assessments (both independent and integrated). A “standard” selection of indicators to assess within each strand would, on the surface, greatly benefit integrated assessments; particularly the TEA strand. However, this is a similar approach to that taken by the PEF Category Rules [European Commission, (n.d.)]. As PEF demonstrated (discussed in Section 3.1.3.), rigid prescription of indicators hampers the application of assessment guidelines to sufficiently broad ranges of goals and scopes. For LCA and SIA, standardization within sector level guidelines, such as CCU, is impossible. Different CCU processes and products will involve different environmental and social impact pathways and effects, requiring a tailored selection of assessment indicators. TEA is the most promising opportunity for standardized indicator selection. Economic sustainability primarily rests upon OpEx, CapEx and product market prices, as supported by GCI's analysis (Zimmermann et al., 2018); assessment of just these factors would likely provide serviceable insights.
Resolution within assessment results has been safeguarded throughout strand integration by the consistent adoption of ISO's process flow diagram approach to system modeling. This encourages the generation of data inventories around individual process aspects. For “technical-level” assessments of processes this is the constituent unit operations; whereas for supply chain focussed, or “corporate-level” assessments, whole processes may be considered. Consequently, the granularity of detail obtained from assessments is primarily determined by the assessment scope and practitioner's workload restrictions. In this area GCI make significant contributions. Utilization of a shared inventory between strands reduces the complexity of the LCI phase, allowing for increased focus on areas such as cut-off criteria or enhanced impact characterization. This approach is supported throughout the triple helix approach, suggesting that shared mass and energy balances from the LCA and TEA form the backbone of the SIA inventory.
Across all guidance documents, allocation remains unstandardized representing one of the largest remaining sources of practitioner influence. While a methodological hierarchy is presented by ISO, and retained by most subsequent frameworks, the selection of approach must ultimately reflect and service the proposed goal and scope. Consequently, it is unlikely that a resolution will be reached in this area, necessitating transparency around the method employed. Alternatively, the standardized reporting of the mass and energy balance alongside the allocated indicator results will allow practitioners to latterly distribute impacts via an alternate approach. While this would greatly enhance the data availability in literature, many organizations would likely oppose the public disclosure of their process operations on the basis of commercial sensitivity.
Comparability between assessments, characterized by the “apples vs. oranges” problem identified by Zimmermann et al., has improved incrementally but consistently. Each guidance document on the path to a harmonized CCU assessments (shown by Figure 9) delivers more specific practitioner guidance, resulting in fewer methodological decision and therefore divergence. The PEF Category Rules provide the most comparable studies, rigorously specifying almost all decisions on behalf of practitioners. However, as discussed, this hinders harmonization with other stands. GCI deliver, for CCU projects, an exemplary level of guidance; limiting divergence while remaining relevant to broad varieties of goals and scopes. This partial constriction of methodological choices has the added benefit of enhancing study robustness through the reduction of erroneous methodological choices. Unsurprisingly, SIA exhibits a less constrained methodology compared to the other strands or methodologies. This is likely an inevitability within social assessments, precipitating from difficulties around boundary setting and the accurate tracing of impact pathways; both preventing intricate and standardized assessment procedures.
Significant gains could be made around combined assessments if more comprehensive databases were generated for the TEA and SIA strands. Currently LCA monopolizes on practitioner databases, with Ecoinvent being the most commonly employed. The technical data within TEA makes such collation difficult, with many studies focussing on novel processes for which data is not publicly available. However, aspects of the assessment, for example commodity and feedstock market prices, could be collected. If such data was organized by date, region and material specification, large comparability and quality improvements would be seen. SIA, however, is much more complicated. While broad data, likely country specific, could be collected; including slave labor, gender equality, safety standards, etc., it is unclear how this would be directly integrated to either the UNEP/SETAC or triple helix framework without introducing subjectivity.
5. Conclusion
In conclusion, the three strands of assessment have achieved remarkable levels of harmonization since 2007 when integrated methodologies were initially conceived. ISO's 2006 publication of LCA standards form the basis of development. GCI's subsequent generation of the first CCU specific guidelines successfully aligned both LCA and TEA, receiving broad acceptance. However, the final stage in harmonizing CCU specific assessments continues to represent significant challenges, the alignment of SIA. Successful first steps have been made in the form of the triple helix framework; however, the strand lags behind its environmental and economic counterparts. Consequently, SIA cannot yet be deemed to have achieved a satisfactory degree of harmonization.
As a result of its failure to reach maturity, CCU SIA is currently relegated to primarily “red flag” applications. In this capacity it can successfully identify potential sources of unsustainable social practices; however, remains vague and unprecise. Until the tracing of impacts and characterization methods can be improved, full harmonization is unlikely to be realized. Given the time periods required to generate this knowledge for LCA, attainment of such models is likely some years away. This presents practitioners with a dilemma within the CCU space; should SIA be implemented as a complimentary strand on a “red flag” basis, or discarded from assessments?
Differing schools of thought percolate within the community; however, a majority identify SIA as a critical component of holistic assessment. Practitioners are subsequently faced with selecting a mode of assessment application. From the literature reviewed, SIA appears suitable as an assessment “screen”. Application in this capacity, as indeed proposed by McCord et al., plays to the strength of lacking data availability; providing a go-no-go verdict on the value of further work and time intensive assessment. If successful, a system can then be evaluated on the basis of GCI's integrated TEA and LCA guidelines. If possible, at this stage, a more detailed iteration of the SIA should also be completed; again, using the triple helix framework's approach. Following this assessment pattern, a holistic overview of the systems sustainability profile is attained, with a respectable degree of methodological harmonization between strands.
If a “just transition” toward sustainable society is to be achieved, SIA cannot reasonably be relegated into a second strata of assessment. At the very least, application in its current form will prevent R&D efforts and economic investment into fundamentally unsustainable processes, systems, or supply chains. In many senses, this alone should be seen by industry, policy makers, and the public, as invaluable. Future work in the field should focus on the iterative improvement of SIA within integrated CCU sustainability assessments, using the triple helix framework as a competent first step. As discussed, many methodological aspects would benefit from greater degrees of standardization, most notably impact quantification methods, and agreed upon scoring scales.
Author contributions
AN: conceptualization, methodology, formal analysis, investigation, literature and data curation, writing—original draft, and review and editing. PS: supervision and writing—review. Both authors contributed to the article and approved the submitted version.
Funding
This project has received funding from Unilever PLC under a strategic partnership with PS at The University of Sheffield and in the form of a Ph.D. Studentship to AN. This work was also partly funded by the Engineering and Physical Sciences Research Council (EP/V011863/1) as part of the UKRI Interdisciplinary Center for Circular Chemical Economy.
Acknowledgments
The authors would like to acknowledge and thank Katy Armstrong, Dr. Chris Gibbs, and Dr. Alastair Sanderson of Unilever PLC who have engaged in discussions inspiring this work.
Conflict of interest
The authors declare that the research was conducted in the absence of any commercial or financial relationships that could be construed as a potential conflict of interest.
Publisher's note
All claims expressed in this article are solely those of the authors and do not necessarily represent those of their affiliated organizations, or those of the publisher, the editors and the reviewers. Any product that may be evaluated in this article, or claim that may be made by its manufacturer, is not guaranteed or endorsed by the publisher.
References
Assies, J. A. (1992). “Introduction paper to SETAC-Europe workshop on environmental life cycle analysis of products,” in Proceedings of a SETAC-Europe Workshop, eds J. Guinée and R. Heijungs.
Azapagic, A. (2004). Developing a framework for sustainable development indicators for the mining and minerals industry. J. Clean. Prod. 12, 639–662. doi: 10.1016/S0959-6526(03)00075-1
Baumann, H., and Tillman, A.-M. (2004). The hitchhikers guide to LCA: An Orientation in Life Cycle Assessment Methodology and Application. Los Angeles, CA: Professional Publishing House.
Becker, H. A. (2001). Socialimpact assessment. Eur. J. Oper. Res. 128, 311–321. doi: 10.1016/S0377-2217(00)00074-6
Boustead, I. (1974). Resource implications with particular reference to energy requirements for glass and plastic milk bottles. Int. J. Dairy Technol. 27, 159–165. doi: 10.1111/j.1471-0307.1974.tb01694.x
British Standards Institution (2011). PAS 2050:2011 Specification for the Assessment of the Life Cycle Greenhouse Gas Emissions of Goods and Services. London, United Kingdom: British Standards Institution.
Buytaert, V., Muys, B., Devriendt, N., Pelkmans, L., Kretzschmer, J. G., and Samson, J. (2011). Towards integrated sustainability assessment for energetic use of biomass: a state of the art evaluation of assessment tools. Renewable Sustainable Energy Rev. 15, 3918–3933. doi: 10.1016/j.rser.2011.07.036
Chhipi-Shrestha, G. K., Hewage, K., and Sadiq, R. (2015). ‘Socializing' sustainability: a critical review on current development status of social life cycle impact assessment method. Clean Technol. Environ. Policy. 17, 579–596. doi: 10.1007/s10098-014-0841-5
Curran, M. A. (2012). Life Cycle Assessment Handbook: A Guide for Environmentally Sustainable Products (1st Ed.). Beverly, MA: Wiley-Scrivener. doi: 10.1002/9781118528372
Dong, Y., Miraglia, S., Manzo, S., Geogrgiadis, S., Danielson, H. J., Boriani, E., et al. (2018). Environmental sustainable decision making– the need and obstacles for integration of LCA into decision analysis. Environm. Sci. Policy. 87, 33–44. doi: 10.1016/j.envsci.2018.05.018
Ecoinvent (2019). Ecoinvent. Available online at: https://ecoinvent.org (accessed 21 November, 2022).
European Commission (n.d.). Results and Deliverables of the Environmental Footprint Pilot Phase. Available online at: https://ec.europa.eu/environment/eussd/smgp/PEFCR_OEFSR_en.htm#final (accessed 11 August, 2022).
European Commission–Joint Research Centre–Institute for Environment and Sustainability (2010). International Reference Life Cycle Data System (ILCD) Handbook–General Guide for Life Cycle Assessment–Detailed Guidance. First edition March 2010. EUR 24708 EN. Luxembourg: Publications Office of the European Union.
European Commission–Joint Research Centre–Institute for Environment and Sustainability (2011). ILCD Handbook: Recommendations for Life Cycle Impact Assessment in the European Context. Luxembourg: Publications Office of the European Union.
European Environment Agency (2018). Electric Vehicles from Life Cycle and Circular Economy Perspectives TERM 2018: Transport and Environment Reporting Mechanism (TERM) report. Luxembourg: Publications Office of the European Union.
Eurostat (n.d.). Eurosta Energy Database. Available online at: https://ec.europa.eu/eurostat/web/energy/data/database (accessed 24 May, 2022).
Faber, G., Mangin, C., and Sick, V. (2021). Life cycle and techno-economic assessment templates for emerging carbon management technologies. Front. Sustainab. 2, 764057. doi: 10.3389/frsus.2021.764057
Finnveden, G., and Potting, J. (2014). “Life cycle assessment,” in Encyclopedia of Toxicology. Amsterdam, Netherlands: Elsevier. p. 74–77. doi: 10.1016/B978-0-12-386454-3.00627-8
Guinée, J. B. (2002). Handbook on Life Cycle Assessment: Operational Guide to the ISO Standards. Berlin, Germany: Springer Science and Business Media. doi: 10.1007/BF02978897
Hauschild, M. Z., and Huijbregts, M. A. J. (2015). Lifecycle Impact Assessment. Dordrecht, Netherlands: Springer. doi: 10.1007/978-94-017-9744-3
Häussinger, P., Lohmüller, R., and Watson, A. M. (2011). “Hydrogen, 2. production,” in Ullmann's Encyclopedia of Inductrial Chemistry. Weinheim, Germany: Wiley-VCH. doi: 10.1002/14356007.o13_o03
Henriette, N. (2016). Economics of carbon dioxide capture and utilization—a supply and demand perspective. Environ. Sci. Pollut. Res. 23, 22226–22241. doi: 10.1007/s11356-016-6810-2
Huarachi, D. A. R., Piekarski, C. M., Puglieri, F. N., and de Francisco, A. C. (2020). Past and future of social life cycle assessment: historical evolution and research trends. J. Cleaner Prod. 264. doi: 10.1016/j.jclepro.2020.121506
Huijbregts, M. A. J., Steinman, Z., Elshouf, P., Stam, G., Veronica, F., Zijp, M., et al. (2016). ReCiPe 2016 v1.1 A harmonized life cycle impact assessment method at midpoint and endpoint level Report I: Characterization. Bilthoven, The Netherlands: National Institute for Public Health and the Environment. doi: 10.1007/s11367-016-1246-y
Hunkeler, D., Lichtenvort, K., and Rebitzer, G. (2008). Environmental Life Cycle Costing (1st Edition). Boca Raton, Florida: CRC Press. doi: 10.1201/9781420054736
Hunt, R. G., et al. (1974). Resourceandenvironmental Profile Analysis of Nine Beverage Container Alternatives, Washington, DC: U.S. Environmental Protection Agency.
International Federation of Institutes for Advanced Study, Sverige, Styrelsen för Teknisk, Utveckling, and Fédération Internationale des Instituts de Hautes Etudes (1974). Energy Analysis Workshop on Methodology and conVentions, Guldsmedshyttan, Sweden: International Federation of Institutes for Advanced Study.
International Standards Organisation Technical Committee 265 (2016). ISO 27912 Carbon dioxide capture—Carbon Dioxide Capture Systems, Technologies and Processes, Geneve, Switzerland: International Standards Organisation.
International Standards Organisation Technical Committee 265 (2018). ISO 27919-1 Carbon Dioxide Capture—Part 1: Performance Evaluation Methods for Post-Combustion CO2 Capture Integrated with a Power Plant, Geneve, Switzerland: International Standards Organisation.
International Standards Organisation Technical Committee 265 (2021). ISO 27919-2 Carbon Dioxide Capture—Part 2: Evaluation Procedure to Assure and Maintain Stable Performance of Post-Combustion CO2 Capture Plant Integrated with a Power Plant, Geneve Switzerland: International Standards Organisation.
ISO Technical Committee, 207. (2006a). ISO14040:2006 Environmental Management—Lifecycle Assessment–Principles and Framework, Geneva, Switzerland: International Standards Organisation.
ISO Technical Committee, 207. (2006b). ISO 14044:2006 Environmental Management—Lifecycle Assessment–Requirements and Guidelines, Geneva, Switzerland: International Organisation for Standardization.
ISO Technical Committee, 207. (2006c). ISO 14025:2006: Environmental Labels and Declarations—Type III Environmental Declarations—Principles and Procedures, Geneva, Switzerland: International Organisation for Standardization.
Jones, C. R., Kaklamanou, D., Stuttard, W. M., Radford, R. L., and Burley, J. (2015). Investigating public perceptions of carbon dioxide utilization (CDU) technology: a mixed methods study. Faraday Discuss. 183, 327–347. doi: 10.1039/C5FD00063G
Jones, C. R., Olfe-Kräutlein, B., and Kaklamanou, D. (2017a). Lay perceptions of carbon dioxide utilisation technologies in the United Kingdom and Germany: an exploratory qualitative interview study. Energy Res. Social Sci. 34, 283–293. doi: 10.1016/j.erss.2017.09.011
Jones, C. R., Olfe-Kräutlein, B., Naims, H., and Armstrong, K. (2017b). The social acceptance of carbon dioxide utilization: a review and research agenda. Front. Ener. Res. 5, 1–24. doi: 10.3389/fenrg.2017.00011
Jørgensen, A., Le Bocq, A., Nazarkina, L., and Hauschild, M. Z. (2007). Methodologies for social life cycle assessment. Int. J. Lifecycle Assess. 13, 96–103. doi: 10.1065/lca2007.11.367
Kerkhof, A., Terlouw, W., Vieira, M., Alexandre, C., and Bagard, R. (2017). Technical Evaluation of the EU EF Pilot Phase, Brussels. Belgium: European Commission.
Kloepffer, W. (2008). Life cycle sustainability assessment of products. Int. J. Lifecycle Assess. 13, 89–95. doi: 10.1065/lca2008.02.376
Klöpffer, W. (2003). Life-Cycle based methods for sustainable product development. Int. J. Lifecycle Assess. 8, 157–159. doi: 10.1007/BF02978462
Kosan, R., Lee, J. H., and Gani, R. (2016). A methodological framework for the development of feasible CO2 conversion processes. Int. J. Greenhouse Gas Control. 47, 250–265. doi: 10.1016/j.ijggc.2016.01.028
Kühnen, M., and Hahn, R. (2017). Indicators in social life cycle assessmen–a review of frameworks, theories, and empirical experience. J. Indust. Ecol. 21, 1547–1565. doi: 10.1111/jiec.12663
Mancini, L., and Sala, S. (2018). Social impact assessment in the mining sector: review and comparison of indicators frameworks. Resources Policy. 57, 98–111. doi: 10.1016/j.resourpol.2018.02.002
McCord, S., Armstrong, K., and Styring, P. (2021). Developing a triple helix approach for CO2 utilization assessment. Faraday Dis. 231, 2021. doi: 10.1039/D1FD00002K
McCord, S., Zaragoza, A. V., Langhorst, T., Strunge, T., Cremonese, L., Schomäcker, R., et al. (2018). “Part E: Integrated TEA and LCA Guidelines,” in Techno-Economic Assessment and Life Cycle Assessment Guidelines for CO2 Utilization (Version 2). Ann Arbor: Global CO2 Initiative. p. 255–301.
McCord, S., Zaragoza, A. V., Langhorst, T., Strunge, T., Cremonese, L., Schomäcker, R., et al. (2022). “Part E: Integrated TEA and LCA Guidelines,” in Techno-Economic Assessment and Life Cycle Assessment Guidelines for CO2 Utilization (Version 2). Ann Arbor: CO2 Chem Media and Publishing Ltd. P. 255–301.
Metz, B., et al. (2005). IPCC special report on carbon dioxide capture and storage. Cambridge, United Kingdom: Cambridge University Press.
Miah, J. H., Koh, S. C. L., and Stone, D. (2017). A hybridised framework combining integrated methods for environmental life cycle assessment and life cycle costing. J. Clean. Prod. 168, 846–866. doi: 10.1016/j.jclepro.2017.08.187
Müller, L., Langhorst, T., Katelhon, A., Bachmann, M., Sternberg, A., and Bardow, A. (2018). “Part C: LCA Guidelines,” in Techno-Economic Assessment & Life Cycle Assessment Guidelines for CO2 Utilization (Ann Arbor, Michigan: CO2 Chem Media and Publishing Ltd), pp. 108–153.
Müller, L., Langhorst, T., Katelhon, A., Bachmann, M., Sternberg, A., and Bardow, A. (2022). “Part C: LCA guidelines,” in Techno-Economic Assessment & Life Cycle Assessment Guidelines for CO2 Utilization (Version 2) (Ann Arbor, MI: CO2 Chem Media and Publishing Ltd), 133–188.
Muralikrishna, I. V., and Manickam, V. (2017). “Chapter Five–Lifecycle Assessment,” in Environmental Management: Science and Engineering for Industry. Amsterdam, Netherlands: Elsevier. p. 57–75. doi: 10.1016/B978-0-12-811989-1.00005-1
O'Brien, M., Doig, A., and Clift, R. (1996). Social and environmental life cycle assessment (SELCA). Int. J. LCA 1, 231–237. doi: 10.1007/BF02978703
Offermann-van Heek, J., Arining, K., Sternberg, A., Bardow, A., and Ziefle, M. (2020). Assessing public acceptance of the life cycle of CO2-based fuels: does information make the difference?. Energy Policy. 143, 111586. doi: 10.1016/j.enpol.2020.111586
Otto, A., Grube, T., Schiebahn, S., and Stolten, D. (2015). Closing the loop: captured CO2 as a feedstock in the chemical industry. Energy Environ. Sci. 8, 3283–3297. doi: 10.1039/C5EE02591E
Peters, M. S., Timmerhaus, K. D., and West, R. E. (2003). Plant Design and Economics for Chemical Engineers. New York, New York: McGraw-Hill Education.
Pieri, T., Nikitas, A., Castillo-Castillo, A., and Angelis-Dimakis, A. (2018). Holistic assessment of carbon capture and utilization value chains. Environments. 5, 1–17. doi: 10.3390/environments5100108
Pollok, L., Spierling, S., Endres, H.-J., and Grote, U. (2021). Social life cycle assessments: a review on past development, advances and methodological challenges. Sustainability. 13, 10286. doi: 10.3390/su131810286
Rafiaani, P., Dikopoula, Z., Dael, M., Kuppens, T., Azadi, H., Lebailly, P., et al. (2019). Identifying social indicators for sustainability assessment of CCU technologies: a modified multi-criteria decision making. Soc. Indic. Res. 147, 15–44. doi: 10.1007/s11205-019-02154-4
Remmen, A., Jensen, A. A., and Frydendal, J. (2007). Life Cycle Management A Business Guide to Sustainability. New York, New York: United Nations Environment Programme.
Ricardo Inc (2022). Life Cycle Analysis Comparison: Electric and Internal Combustion Engine Vehicles. Alexandria, VA, USA: The Fuels Initiative.
Sala, S., et al. (2015). Social Life Cycle Assessment: State of the Art and Challenges for Supporting Product Policies. Brussels, Belgium: Publications Office of the European Union.
Sell, I., Ott, D., and Kralisch, D. (2014). Life cycle cost analysis as decision support tool in chemical process development. ChemBioEng Reviews. 1, 50–56. doi: 10.1002/cben.201300007
Sinnott, R., and Towler, G. (2009). Chemical Engineering Design. Oxford, United Kingdom: Butterworth-Heinemann.
Skone, T. J., Mutchek, M., Krynock, M., Moni, S., Rai, S., Chou, J., et al. (2022). Carbon Dioxide Utilization Life Cycle Analysis Guidance for the U.S. DOE Office of Fossil energy and Carbon Management. Pittsburgh: National Energy Technology Laboratory.
Stiching Global Reporting Initiative (2021). GRI 1: Foundation (2021). London, UK: Global Sustainability Standards Board.
Sugiyama, H., Fischer, U., Hungerbühler, K., and Hirao, M. (2008). Decision framework for chemical process design including different stages of environmental, health, and safety assessment. InterScience. 54, 1037–1053. doi: 10.1002/aic.11430
Sundström, G. (1971). Investigation of Energy Requirements From raw Material to Garbage Treatment for Four Swedish Beer and Packaging Alternatives. Malmö, Sweden: Rigello Pak AB.
Swarr, T. E., Hunkeler, D., Klopffer, W., Pesanon, H. -L., Ciroth, A., Brent, A. C., et al. (2011). Environmental life-cycle costing: a code of practice. Int. J. Life Cycle Assess. 16, 389–391. doi: 10.1007/s11367-011-0287-5
Turton, R., Bailie, R. C., and Whiting, W. B. (2012). Analysis, Synthesis, and Design of Chemical Processes. Hoboken, NJ: Prentice Hall.
UNEP (2009). Guidelines for Social Life Cycle Assessment of Products. Nairobi, Kenya: United Nations Environment Programme (UNEP).
UNEP (2020). Guidelines for Social Life Cycle Assessment of Products and Organizations (2020). Nairobi, Kenya: United Nations Environment Programme (UNEP).
United Nations (2015b). Transforming our world: the (2030). Agenda for Sustainable Development. New York, NY: United Nations General Assembly.
United Nations / Framework Convention on Climate Change (2015). Adoption of the Paris Agreement, 21st Conference of the Parties. Paris, United Nations.
Universiteit Leiden (2016). CML-IA Characterisation Factors. Available online at: https://www.universiteitleiden.nl/en/research/research-output/science/cml-ia-characterisation-factors (accessed 01 September, 2022).
US Energy Information Administration (n.d.). API Open Data. Available online at: https://www.eia.gov/opendata/ (accessed 24 May, 2022).
US EPA. (2021). Tool for Reduction and Assessment of Chemicals and Other Environmental Impacts (TRACI). Available online at: https://www.epa.gov/chemical-research/tool-reduction-and-assessment-chemicals-and-other-environmental-impacts-traci (accessed 01 September, 2022).
van Heek, J., Arning, K., and Ziefle, M. (2017). Reduce, reuse, recycle: acceptance of CO2-utilization for plastic products. Energy Procedia. 114, 7212–7223. doi: 10.1016/j.egypro.2017.03.1829
Vanclay, F. (2003). International principles for social impact assessment. Impact Assess. Project Appraisal. 21, 5–12. doi: 10.3152/147154603781766491
Venkatesh, G. (2019). Critique of selected peer-reviewed publications on applied social life cycle assessment: focus on cases from developing countries. Clean Technol. Environ. Policy. 21, 413–430. doi: 10.1007/s10098-018-1644-x
Verhoeven, J. I., van Lith, T. J., Ekker, M. S., Hilkens, N. A., Maaijwee, N. A. M., Rutten-Jacobs, L. C. A., et al. (2018). “Part C: LCA guidelines,” in Techno-Economic Assessment and Life Cycle Assessment Guidelines for CO2 Utilization. Ann Arbor, Michigan: CO2Chem Media and Publishing Ltd. p. 108–153.
von der Assen, N., Jung, J., and Bardow, A. (2013). Life-cycle assessment of carbon dioxide capture and utilization: avoiding the pitfalls. Energy Environ. Sci. 6, 2721. doi: 10.1039/c3ee41151f
von der Assen, N., Voll, P., Peters, M., and Bardow, A. (2014). Life cycle assessment of CO2 capture and utilization: a tutorial review. Chem. Soc. Rev. 42, 7982–7994. doi: 10.1039/C3CS60373C
Witchalls, S. (2018). Power poverty: the new paradigm for social and economic inequality of electric vehicles. Available online at: https://www.stantec.com/en/ideas/power-poverty-the-new-paradigm-for-social-and-economic-inequality-of-electric-vehicles (accessed 24 October, 2022).
World Resources Institute (2011). Product Life Cycle Accounting and Reporting Standard, Washington, DC: Greenhouse Gas Protocol.
Wunderlich, J., Armstrong, K., Buchner, G., Styring, P., and Schomäcker, R. (2021). Integration of techno-economic and life cycle assessment: Defining and applying integration types for chemical technology development. J. Clean. Prod. 287, 125021. doi: 10.1016/j.jclepro.2020.125021
Zero Emission Platform (2011). The Costs of CO2 Capture, Transport and Storage. Post-demonstration CCS in the EU. Brussels, Belgium: Zero Emission Platform.
Zimmermann, A., Wunderlich, J., Buchner, G., Armstrong, K., Marxen, A., Michailos, S., et al. (2018). “Part B: TEA Guidelines,” in Techno-Economic Assessment and Life Cycle Assessment Guidelines for CO2 Utilization. Ann Arbor, Michigan: CO2 Chem Media and Publishing. p. 25–97.
Zimmermann, A. W., and Schomäcker, R. (2017). Assessing early-stage co2 utilization technologies—comparing apples and oranges? Energy Technol. 5, 850–860. doi: 10.1002/ente.201600805
Keywords: life cycle assessment (LCA), sustainability, carbon capture and utilization (CCU), holistic, harmonization and alignment, techno-economic analysis (TEA), social impact analysis, social lifecycle assessment (sLCA)
Citation: Newman AJK and Styring P (2023) The pursuit of methodological harmonization within the holistic sustainability assessment of CCU projects: A history and critical review. Front. Sustain. 3:1057476. doi: 10.3389/frsus.2022.1057476
Received: 29 September 2022; Accepted: 20 December 2022;
Published: 23 January 2023.
Edited by:
Dominic C. Y. Foo, University of Nottingham Malaysia Campus, MalaysiaReviewed by:
John Frederick Tapia, De La Salle University, PhilippinesJully Tan, Monash University Malaysia, Malaysia
Copyright © 2023 Newman and Styring. This is an open-access article distributed under the terms of the Creative Commons Attribution License (CC BY). The use, distribution or reproduction in other forums is permitted, provided the original author(s) and the copyright owner(s) are credited and that the original publication in this journal is cited, in accordance with accepted academic practice. No use, distribution or reproduction is permitted which does not comply with these terms.
*Correspondence: Peter Styring, cC5zdHlyaW5nQHNoZWZmaWVsZC5hYy51aw==