- Biosphere Research Institute, Letham, United Kingdom
Concerns relating to resource extraction, manufacturing, waste in our supply chains, along with increasing consumption and with their consequences for the planet, have, to a greater or lesser extent, begun to impact the ideological approach and the decision-making of governments, business and consumers alike on the material world we continue to create. Parallel concerns circle around social and environmental resilience, recovery and sustainability. This paper seeks to explore what a sustainable economy should look like in order to contribute to a resilient, diverse and thriving planet, while furthering resilient, diverse and thriving communities. It is argued that at the center of any sustainability theory, whether economic, environmental or social, lies the Earth system. The Earth system represents much more than the organisms sharing this planet with ourselves. Rather, it involves the fluxes of radiation, food energy, water, nutrients and heat. More essentially, it determines our niche space [the multidimensional space where tolerances and requirements interact to determine the habitable space within which an organism can practice its way of life]. Given that humans are some of the most vulnerable organisms on the planet in terms of the narrow range of conditions within which they can survive, the dynamic nature of the planet that determines this range of conditions must surely form the prime focus of our attentions. The Earth System, like all complex, far-from-equilibrium systems, is self-organizing, self-assembling, emergent, non-linear and governed by real-time feedback and sub-optimality at each level of its organization. The consequences of these properties for our economic activities and, indeed, for our own species, are discussed. It is further argued that we need an embedded economy. Fundamental to this exploration are the concept of zusammenhang (literally, hanging together), as developed by Humboldt, and the concept of the Earth as a super-organism, as developed by Hutton. Natural economics within the Earth system is explored and key properties of this economy are highlighted. The embedded economy functions in a way that facilitates natural and social recovery, resilience and functionality, while in resonance with the key characteristics of the Earth system. Artificial intelligence, utilizing ecological intelligence rather than human intelligence, and the internet of things, are identified as potentially significant tools in the establishment of an embedded economy. The embedded economy is then compared and contrasted with current sustainable economics thinking, whose emphasis primarily focuses on resource cycling.
Introduction
The consequences of our recent economic activity have led to serious concerns in terms of environmental and social sustainability. Escalating energy fluxes, resource use, waste and habitat destruction, combined with externalized supply chains tainted with human trafficking and appalling working conditions, impact both environment and society. Inequality in wealth, health, opportunity and education, along with racial and gender discrimination, all sit uncomfortably with an increasingly informed population. Within-country inequality has increased while between country inequality has decreased (Ravallion, 2018). Indeed, it has been suggested that wealth inequality could be underestimated due to the impact of financial globalization (Zucman, 2019).
Some 40% of the human population now depend on nitrogen fertilizers for their existence, with almost all of any future population increasingly likely to be reliant upon them (Smil, 2001). These fertilizers have devastating effects on the hydrosphere [through eutrophication (Rabalais et al., 2009)] and the atmosphere [with nitrous oxide being both a greenhouse gas and an ozone-depleting chemical (Kanter et al., 2020)]. The production of nitrogen fertilizers is highly energy-consuming, accounting for significant carbon dioxide production where fossil fuels are used as the energy source. Thus, technologically elevated populations have significant consequences for the health of the planet in many ways.
Furthermore, resource use has far outpaced population growth. While the population has more than quadrupled in the last century, global economic output has increased 20-fold, requiring an 8-fold increase in materials, including a 34-fold increase in building materials and a 27-fold increase in industrial minerals (Maddison, 2001). Between 1948 and 1973 alone, non-renewable mineral use increased by 340%.
At a social sustainability level, a recent report (Fuller et al., 2022) highlights that one in six premature deaths in humans annually is currently caused by pollution from industry and urbanization. Again, inequality is evident, with 92% of these deaths occurring in low and middle income countries. Fuller et al. (2022) further report that deaths from toxic chemical pollution and ambient air pollution have increased by 66%.
Earth overshoot day (the date in a given year on which we have used the resources that can be re-supplied by the planet) in 2021 was 29th July, representing our current resource use as 1.6 times the Earth's biocapacity to provide for humanity's ecological footprint (Phenrat et al., 2022). The rest of the year represents the overshoot, or net spend. This figure does not include the damage to biocapacity functionality, further diminishing the ability to replace lost material.
Industrial production and consumer consumption lead to 80% of wastewater being discharged back into the Earth system without treatment, impacting the health of 1.8 billion individuals (WWAP, 2017). Over two billion tons of municipal solid waste is generated each year, a third of which is not adequately managed (World Bank, 2022).
The impact of all of this economic activity has significant consequences for the functionality of the Earth system and, as a result, for the human race. With a growing population and increasing consumption, it is now an urgent priority to address this potentially existential threat to our species and the many others that share the planet with us. How should we respond to this crisis, and what kind of economics can expedite our journey toward a sustainable future?
Objectives
In order to address these multiple issues emerging from our activities on the planet (see Steffen et al., 2011) for a discussion on our changing impact on the planet), two fundamental elements must be understood: the centrality of the Earth system itself (Schellnhuber, 1999; Richter and Billings, 2015) and an appropriate economic framework that can work within that system.
This paper sets out to examine the framework within which a sustainable future can be imagined and pursued. Importantly, it is recognized that humans emerged from and are part of the Earth system. Thus, in order to understand what actions we can take, we must fundamentally understand how the Earth system functions and recognize that our solution space is dependent upon the functionality of the Earth system and, thus, must be embedded within this system. Key issues facing us are identified, and the failure of economics and sustainability theory to address these issues is explored. Finally, the paper presents a new set of ideas, set within the Earth system.
The first part of the paper examines what the Earth system is, and the properties that define its functionality and responses to perturbation. Next, the development of human economics through time is scrutinized, charting key changes that have led to the current dominant economic model. An exploration of sustainable economics then follows, understanding how the many schools of thought in this area have developed. Next, Earth system economics is appraised, and the implications of feedback between this overarching economy and the human economy are considered. The concept of pricing the Earth, in the sense of the valuation, trading and commodification of ecosystem services, is critiqued. Finally, a new economic concept, the embedded economy, is outlined and explored, based on current ecology, systems theory and thermodynamics.
The earth system and why it matters
The Earth system is a complex, self-organizing, emergent, sub-optimal, open, non-equilibrium and dynamic planetary system, informed by real-time feedback across its many channels of communication. It shares these properties with many other non-equilibrium complex systems. The flow of energy across its multiple layers of organization is fundamentally guided by the laws of thermodynamics. The first law of thermodynamics states that the energy of the Universe is constant, while the second law states that the entropy (a measure of the thermal energy of a system per unit temperature that is unavailable for doing work) of the Universe tends toward a maximum. Increasing complexity in any system leads to increasing entropy output, and thus in any complex system with access to free energy (such as sunlight), we would expect entropy production to maximize, through increased complexity. This is because increasing complexity brings with it increasing energy throughput, leading to increasing entropy production. This is extremely important in terms of understanding how complex systems such as the Earth system evolve, recover and self-organize (Arango-Restrepo et al., 2019) and provides directionality to the functionality of the Earth system and its components (Skene, 2015). The maximum entropy production principle (MEPP) further states that non-equilibrium thermodynamic systems are organized in steady state such that the rate of entropy production is maximized (Kleidon et al., 2010). In this paper, we will explore the characteristics of the Earth system and their consequences in terms of what a sustainable economy should look like.
All complex systems display a number of consistent characteristics that are essential foundations for any theory on sustainable economics. Firstly, they are self-organizing and self-assembling (Odum, 1988; Braakman et al., 2017). Hierarchical organization represents the outcome of a vast communicative networking, wherein the conditions and components participate in a dynamic self-organization (Skene, 2020b). For almost all of its three and a half billion years of history, life on Earth has continued without Homo sapiens. It has managed to develop, evolve and recover from mass extinctions all by itself. This is important, in that any recovery from the damage done by human activities will be best repaired by the Earth system, rather than through our interventions into it. However, recovery requires some baseline diversity, connectivity and time. Furthermore, if the damage is significant enough, the entire system may re-organize. Self-assembly and self-organization are driven by the laws of thermodynamics (Bruinsma et al., 2003; Arango-Restrepo et al., 2019).
Secondly, complex systems exhibit emergent properties, in that the properties of the system belong to the system, not to any of the individual components such as humans (Bedau and Humphreys, 2008). von Bertalanffy (1933), the founder of modern systems theory, viewed the organism as a hierarchical complex whose properties at any given level of organization emerged from interactions among the parts at a lower level. It is the combination of manifold interactions that leads to the final outcomes, underpinned by real-time feedback and the laws of thermodynamics. The system consists of input signals and a black box where multiple interactions occur resulting in output signals (Figure 1). Each level of organization has its own complex set of interactions all feeding into the Earth system, which in turn signals back to its component parts.
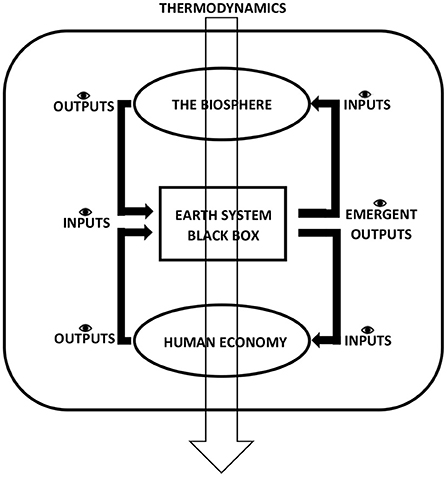
Figure 1. Input, output and the black box of an emergent Earth system. The human economy and the Biosphere both provide input signals to the Earth system and then are recipients of output signals. In order to monitor the impacts of our activities we need to observe real-time feedback of all inputs and outputs through remote sensing and the internet of things (indicated by an eye symbol). Thermodynamics runs through all components as the framework in terms of direction and dynamics, leading to system adjustments in order to maintain maximum entropy production at the system level, with necessary changes at the component level.
This brings us to the third point, which is that all components must operate sub-optimally in order to optimize for the overall system, in what is known as the maximum entropy production principle (Skene, 2020b). The maximum entropy production principle is very much in agreement with the maximum power concept, which Odum (1995) defined as “During self-organization, system designs develop and prevail that maximize power intake [and] energy transformation”. By maximizing power intake and energy transformation, entropy production is also maximized (Skene, 2015). Trade-offs are an essential part of existing within a complex system, where the increasing complexity demands solutions that are less optimal for any one component (MacArthur, 1972; Farnsworth and Niklas, 1995). Hence, we see imperfect correction of mutations in DNA, allowing genetic variation to increase. We encounter squirrels forgetting where they hid all of their nut stashes and foxes failing to catch all of their prey.
Such imperfections are essential. It is our attempt at optimizing for ourselves that has created much of the damage on the planet. A sustainable ecology relies on trade-offs. Of course, natural systems can also undergo destructive spirals, because nature is not resistant to profligate living (Skene, 2018). Fertilizers, applied to optimize food production for ourselves, reach our oceans, setting off population explosions and collapses, combined with anoxia, leading to red tides and dead zones. Unbalancing the naturally low nutrient levels has disastrous consequences for ecosystems such as coral reefs and upland heaths, which are built and function in naturally low nutrient conditions.
The fourth characteristic of complex systems is non-linearity. Here, changes do not always happen in a predictable, linear way but, rather, can suddenly occur at a rapid rate. Folke et al. (2016) note: “Causation is often non-linear in complex adaptive systems with the potential for chaotic dynamics, multiple basins of attraction, and shifts between pathways or regimes, some of which may be irreversible”. These events are called tipping points and can lead to regime shifts where persistent, rapid and large changes in processes and species occur (Scheffer et al., 2001; Biggs et al., 2012). Such regime shifts generally have huge social impacts in addition to their biological effects (Lade et al., 2013).
Finally, real-time feedback is an essential characteristic of any complex system (Jervis, 1997). Conversations continuously occur, leading to rapid and multiple outcomes that are adventitious and often irreversible. Feedback is important because of emergence. Only by tracking outcomes in real time can we monitor the impacts of our actions on the Earth system. Of course, we have huge resources to help us, such as the billions of smart devices and the internet of things, remote-sensing our planet from the surface and from orbiting satellites (see Figure 1). Artificial intelligence networks can bring together this information (Skene, 2020a). While we may have lost the ecological intelligence of our forebears, we have plenty of access to real-time feedback through such technologies.
The Earth system, as an example of a complex system, displays all of these key characteristics. This makes it a challenging entity. The linear, reductionist philosophy that dominates much of our thinking, can become lost in this emergent world of non-linearity and uncertainty. However, in order to shape our economic activities in such a way as to not awaken the monster that is regime shift, where we might find ourselves ejected from the system, or, at best, confined to a greatly diminished niche space, we must urgently familiarize ourselves with these characteristics.
Within the Earth system, humanity has a role to play, and, when suitably contextualized with regards to the functionality of the planet, the emphasis upon not only reducing damage, but facilitating recovery has been explored in such fields as passive re-wilding, process-based restoration ecology and in circular economy writings (e.g., Jørgensen, 2015; Palmer et al., 2016; Velenturf and Purnell, 2021). While the emphasis has often been placed on the damage we are doing to the Earth system, it can be forgotten that the Earth system represents the outcome of vast physical laws and forces that are cosmic in scale, and that our “impact” is merely a part of the real-time feedback that collectively informs the system, resulting in emergent reconfigurations. As such, the Earth system will persist. We cannot re-direct the outcomes of physics.
Complex systems have two types of signal: input and output. We can only control our input into the Earth system, but not the output, as the output is an emergent characteristic, whose properties belong to the whole, not to any single component. An error that is often made is that we can think of the outputs of our economies as linear processes, where materials used and released impact upon a particular species or process. However, we fail to recognize that these outputs become the inputs of the Earth system, and enter a black box, the outputs of which are emergent and non-linear, likely impacting multiple components along the way (Figure 1). Thus, uncertainty prevails across spatial and temporal considerations, increasing the possibility of regime shift reconfiguration. The outcomes of our economic activities go far beyond any calculation of economics growth or development. They reach into the greater Earth system economy, with potentially devastating consequences.
Regime shift reconfigurations have the capacity to completely decimate our economic activities, and eradicate our niche space. Thus, we should approach the issue of sustainability with humility, recognizing that our fates, to a large extent, are very much in the hands of an arbitrator founded in the laws of thermodynamics, rather than our own.
Economics: A brief history
Steffen et al. (2020, p. 54) write that: “For tens of thousands of years, indigenous cultures around the world have recognized cycles and systems in the environment, and that humans are an integral part of these”. Indigenous knowledge represents a way of living, embracing environment, society and economic activity. Early in our history, a limitation of resource availability led to a waste-is-food approach, wherein upcycling, reuse and reduction of use were commonly practiced (Desrochers, 2000, 2008). Supply chains were immediate, simple, short and with tight cycling, much as in the natural world. There was little trade and accountability was high, given that consumer behavior immediately impacted on resource base viability, rapidly impacting the same consumers.
Hunter-gatherers are thought to have lived in an equitable society as can be seen in many of the remnant groups, such as the Ogiek people of Kenya and the! Kung people of the Kalihari (Blackburn, 1971; Winterhalder, 2001). Even modern hunter-gatherer communities forage and hunt for only 3–5 h each day, spending the rest of the time in leisure activities or resting (Hayden, 1981). This contradicts images of relentless labor and difficult lives. This free time would allow opportunities for sharing traditional knowledge and strengthening community cohesion, so central to wellbeing, while heightening connectivity with the landscape and promoting environmental values (Dutcher et al., 2007). Females and males shared leadership roles within the tribe, bringing gender equality (Muchemi and Ehrensperger, 2011).
Many indigenous people today still have strong community regulation of resource use. The Ogiek people of Kenya cannot cut down a tree without permission from the elders (Ottenberg and Ottenberg, 1960). The Sami of Northern Europe each had access to a number of lakes, allowing a rotation, where each lake would have 1 or 2 years of fallow (Norstedt et al., 2014). The Shona of South Africa have taboos regarding the harvesting of wild fruit only at a particular point of ripening, with limitations as to when hunting can occur and what age of prey may be hunted (Mungwini, 2019).
And so humans have been practicing ethical environmental sustainability within the context of their landscapes for millennia. Indeed, recent research points to current sustainable indigenous practices as a blueprint for sustainable behavior (Kupa'a et al., 2021; Ferguson et al., 2022; Reeder-Myers et al., 2022).
The onset of agriculture and economic trade, together with urban conurbations, just after the last ice age, altered the relationship between humanity, economics and nature. Power-through-possession became the dominant mantra, exemplified by the building of empires and increasingly centric control. This reached its zenith many times through history, and was accompanied by increasing inequality (Skene and Murray, 2017).
A rapidly expanding economic program altered completely the energy and resource fluxes through the Anthroposphere relative to that of the rest of the Earth system (de Molina and Toledo, 2014), and, gradually, humanity began to disavow any integration within the Earth system, viewing it as a source and sink, rather than as an intrinsic complex system.
Short supply chains demand accountability, but as supply chains have stretched across the globe, the consequences of resource exploitation have been pushed over the horizon and become externalized (Phillips and Caldwell, 2005; Hofmann et al., 2018; Gualandris et al., 2021). Thus, the dimensions of any economy, in terms of supply chains, are important. Allen et al. (1999) point out that “Supply-side sustainability focuses upon the management of supply chains in terms of ecosystem functioning, not merely the resource in question.”
However, things are very different now than in our earlier history. The elevated human population is maintained by chemical fertilizers and vast energy consumption, while a consumer appetite for material wealth is encouraged by powerful advertising across multiple media platforms, leading, collectively, to a run-away economy. A vast resource extraction industry tightly linked to the ties between status, power, financial wealth and wellbeing, in addition to globalized branding, political processes and multinational companies, many of which are more wealthy than some countries, all pose a significant suite of challenges. Furthermore, increased inequality in terms of wealth, health, gender and opportunity, and the politicization of wealth and poverty all lead to a fractured society.
At the heart of all of this lies economics. We have seen that across our history, the practice of economics has shifted fundamentally, exorcizing contextualization with the Earth system and society. Any hope for a sustainable future at social and environmental levels surely cannot rely upon greening the current economic model, while fundamentally maintaining a business-as-usual approach to our human-centric optimization of conditions. The fruits from this tree are toxic to much of humanity. Rather, we may need to consider a restructuring from the ground up, in order to be fit-for-purpose.
Intrinsic within current economic theory is the mechanism of the invisible hand, developed by Adam Smith, where laissez-faire markets will lead to price control upon commodities, in that scarcity leads to rising prices, forcing less use, more expensive processing or substitution. Nordhaus and Tobin (1973, p. 525) observed that “If the past is any guide for the future, there seems to be little reason to worry about the exhaustion of resources, which the market already treats as economic good”. This Cornucopian position was sketched out by George (1879, p. 131), who wrote: “Both the jayhawk and the man eat chickens: but the more jayhawks, the fewer chickens, while the more men, the more chickens”.
This thinking, although still espoused in one form or another by many today, would appear deeply flawed in several ways. Firstly, in natural predator-prey population dynamics, once prey numbers decrease, predator numbers also decrease, leading to a rise in the prey population, followed by a rise in predator numbers, and so on (Lotka, 1925). Thus, fewer chickens lead to fewer jayhawks, and this leads to more chickens. Secondly, the ability of humans to increase chicken populations, in order to maintain elevated chicken and human populations, is dependent on the Earth system continuing to function in such a way as to allow this. Rapid disease spread (such as bird flu) in crowded chicken roosts, war (where food security issues lead to countries blocking exports), effluent, energy availability and supply of foodstuffs all can severely impact upon chicken-rearing and beyond.
Natural resource extraction generally follows an increasingly destructive path, because of the current economic system. The cheapest, most easily acquired material is extracted first, referred to as high-grading. As this supply becomes exhausted, prices rise and more challenging sources are mined, with greater environmental and social damage. Low-grade gold, for example, requires more water and produces much more cyanide waste (used for leaching the gold from the ore) than high-grade gold (Mudd, 2010).
Thus, it is not simply the market controlling use, but rather an acceleration of pollution in the effort to maintain supplies with diminishing materials available. Financial costs of the subsequent environmental damage also rachet upwards. Fines for polluting are often small compared to profit margins, and thus, in cold economic terms, merely represent investment (Coffee, 1980; Odeku and Gundani, 2017). Yet the costs of the consequences of the damage can be significant at the wider level. Intensification of agriculture, leading to soil erosion, costs the US government 40 billion dollars per year (Halopka, 2017). Nitrous oxide, released from fertilizers, depletes ozone levels, increasing skin cancer occurrence. Climate destabilization as a whole is estimated to cost some twenty trillion dollars by 2100 (Burke et al., 2018). The loss of coral reefs from fertilizer runoff and warming waters is estimated to cost one trillion dollars per year.
Thus, the consequences of increasingly low-grade mining, intensified agriculture and other responses to shortages of supply do not represent a market correction, but an exacerbation of an already difficult situation, akin to the treadmill of production theory (Schnaiberg, 1980). This results in a spiral, not a circle, and an existential threat from an erroneous belief in the free market as an self-governing arbitrator of safe practice and sustainability. This is seen no more clearly than in the recent critiques of the Kuznets curve, which claims that with increasing economic growth, inequality initially increases to a maximum, but then decreases, and the environmental Kuznets curve, which claims that with increasing economic growth, environmental damage initially increases to a maximum but then decreases (Stern et al., 1994; Jorgenson and Clark, 2011; Fujii and Managi, 2013; Kaika and Zervas, 2013).
With the loss of fifty percent of our topsoil in the last 150 years because of intensive agricultural practice (Pal and Chakrabortty, 2019), and the creation of dead zones in the coastal waters, leading to fishing collapse (Marques, 2020), we can see that the technological and industrial efforts to produce more food while defeating disease with antibiotics, in an attempt to overcome Malthus's horse riders of the apocalypse (famine and disease) have led to the release of new modern apocalyptic horse riders, such as eutrophication, antibiotic resistance, soil salinization, soil erosion, climate destabilization and life-threatening pollutants.
Pest-resistant, genetically modified crops also travel along the same path, as pests overcome the engineered resistance mechanism, as demonstrated with pink bollworm and Bt-cotton (Dhurua and Gujar, 2011; Naik et al., 2020). Given that the cotton is programmed to continuously (constitutively) produce the chemicals involved in resistance, once this resistance is overcome, these modified plants are now at a disadvantage to non-modified cotton, as they have the same losses to the pest, but must divert some of their energy to producing a, now useless, deterrent.
Similar resistance has been linked to herbicide-resistant crops (Clay, 2021), wherein ruderals have gained resistance to the herbicide and therefore the modified crops now waste energy producing herbicide resistance constitutively, while the ruderals are unaffected, putting the non-modified crops at an overall advantage.
All of these activities usher back the original horse riders, famine and disease. Meanwhile increasing environmental stress and social inequality increase the likelihood of the third horseman of Malthus, war. The consequences of our economic activities upon a range of security issues are summarized in Figure 2.
Pricing the earth system
The appropriation of nature
While the physiocrats viewed land as the primary source of value, classical economics tended to view the value of Nature as existing in the rent derived from the appropriation of Nature, rather than in Nature itself. This followed on from the works of Locke and Bacon, who viewed Nature as a commons that gained value through exploitation (Natura vexata). Neoclassical economics embraced exchange values in terms of monetary analysis, rather than use value. This ushered in the weak sustainability concept, where technical systems could replace natural systems, and so long as the total capital value remained constant, capital could be substituted between human, manufactured and natural capital pools, as opposed to strong sustainability thinking, which emphasizes non-substitution. Solow (1974, p. 11) wrote that: “The world can, in effect, get along without natural resources, so exhaustion is just an event, not a catastrophe”.
Ecosystem services
The concept of valuing Nature for what it can do for us, as represented by the term “ecosystem services”, was seen as a way of making relevant the importance of a functional planet. The emphasis on ecosystem services, while well-intentioned, had the unfortunate effect of continuing the conceptualization of the Earth system as servant, rather than arbitrator.
The term “ecosystem services” brings to mind a natural world that appears to be focused on keeping us alive, while we destroy it with our pursuit of luxury and high living. This, combined with our placing a monetary value on Nature, and with our belief that we can save the planet by actively fixing it or replacing it with technological solutions, sends a message steeped in reductionist thinking and neo-classical economics, a far cry from the real world. The Earth system is much more complicated and serves no-one. Its architect is thermodynamics and it runs to the laws of the Cosmos, reliant on our neighboring star and occasionally reset by extra-terrestrial bolides that collide with it. Energy is the currency, not species. It is raw physics that lies at the heart of our planet, not some servant, bowed down by our relentless abuse.
Hardin (1968) had argued that resource regimes lacking property rights could be vulnerable to exploitation. Costanza et al. (1997) set out to extend the monetary valuation approach to ecology, failing to sufficiently consider systems theory and thermodynamics, so important in modern ecological thinking. While Costanza et al. admit that the true value of the Earth system is infinite, through their persistence in calculating marginal value, the Earth system is fundamentally reduced to some anthropocentric imagining, rather than the actuality of a great river of energy flowing through the planet and allowing order to be established, through the generation of disorder (in recognition of the second law of thermodynamics).
By diminishing the Earth system to a fiscal value, we fail to grasp its true meaning. Current sustainability policy and action commonly utilize the concepts of incremental or marginal valuations of ecosystem services, defining these as the estimated rate of change of value with changes in ecosystem services from their current levels.
Trading in ecosystem services has long been promoted as an extension of commodification and monetization of Nature, but remains controversial. Carbon trading, sulfur dioxide trading and wetland trading continue to occur. The EU emission trading system began in 2005, covering six significant greenhouse gases (Gómez-Baggethun et al., 2010). The Kyoto protocol transformed into a carbon trading mechanism. Scott (2008, p.4) reflected “What is the best way to tackle climate destabilization? If we have a global carbon price, the market sorts it out”. However, Lohmann (2010, p. 81) observed that a commodity approach “abstracts from where, how, when and by whom the cuts are made, dis-embedding climate solutions from history and technology and re-embedding them into neo-classical economic theory, trade treaties, property law, risk management and so forth”.
Issues with the commodification of nature
A number of serious issues are apparent. Firstly, an underlying assumption is that the current economic model is fit for purpose in delivering solutions to our predicament, despite its central role in creating the problems in the first place. Secondly, there may be substitution of pollutants rather than substitution of capital. Thirdly, the calculation of the carbon budget is open to manipulation. Russia and Ukraine were awarded excessively generous sink credits, each claiming baseline projections for the whole of the former USSR (Böhringer and Vogt, 2004). The Czech energy giant, CEZ, was allocated 30% of the Czech carbon allowances. The company then sold them and bought them back when prices were lower, using the profit to expand coal production. Fourthly, carbon offset is not carbon reduction. Fifthly, there is no attempt to address social sustainability and finally, akin to indulgences which could be bought from the church, allowing the rich to avoid penance, the rich can also buy pollution rights (Spash, 2010).
At Kyoto, the Australian government managed to insert an additional clause that allowed them to take into account a huge rise in deforestation in 1990, followed by a huge decline in the following years due to a change in legislation. The rise was in response to the imminent banning of much deforestation, with businesses felling as many trees as possible before the ban came in. Thus, the inclusion of forest clearing as a net producer of carbon dioxide in the 1990 baseline measure meant that any reduction that followed, due to the legislation preventing much deforestation, would offset much of this excessive carbon production elsewhere (Macintosh, 2012).
Hence, although Australia committed to not exceeding an 8% rise in CO2 output from 1990 to 2007, in actual fact they were able to increase their carbon production by almost 30%, offset by a one-off large-scale deforestation event in 1990. If the year 1989 or 1991 had been used as the baseline, there would have been much less deforestation then, meaning that much greater cuts would have been needed to reach the target agreed at Kyoto (Howarth and Foxall, 2010).
Turner (2000, p. 705) observed that “the unfettered markets fail to allocate environmental resources efficiently”. Anderson (2012) concluded: “Carbon offsetting is without scientific legitimacy and is dangerously misleading”.
The Intergovernmental Science-Policy Platform of Biodiversity and Ecosystem Services (IPBES) recently replaced the term “ecosystem services” (ES) with the concept of “Nature's contributions to people” in an effort to better include cultural elements, indigenous people, and local communities (Diaz et al., 2018). However, this still omits any reference to the centrality of the Earth system, wherein we must contribute to it, rather than focusing on its contribution to us. It is we who are embedded within the Earth system, rather than the reverse. Thus, any hope of a sustainable future requires a complete focus on what we need to do to increase our embeddedness. Akin to the concept of repurposing our swords into plowshares, we must convert our most powerful and destructive force, economics, into a tool to embed us afresh into the Earth system (see, for example, Woodward, 1985; Raworth, 2017; Chen et al., 2021).
It is, surely, ecosystem functionality that must be central to any economic program, not ecosystem services nor contributions. Furthermore, without recognizing that we are part of the Earth system, but, instead, representing it as somehow separate from us and defined only in terms of human economics, we fail to set the correct foundations for meaningful action. Gómez-Baggethun and Ruiz-Pérez (2011, p. 1) conclude that “economic valuation is likely to pave the way for the commodification of ecosystem services with potentially counterproductive effects in the long term for biodiversity conservation”.
As we can see, many attempts have been made to integrate the Earth system into the economic model rather than the reverse, mostly by attempting to evaluate the costs of production as damage to the Earth system in terms of lost ecosystem services. Westman (1977) was one of the first economists to set out a formulation in order to value products, inclusive of loss or damage in ecosystem functionality. Most efforts cycle around extending the social welfare function to include the services of natural capital.
However, this paper suggests that this will not resolve the current crisis. Instead, we must re-imagine our economic model and integrate it into the Earth system. However, an inherent assumption in green accounting has been that the correct value of resource depletion is the change in the total value of the resource stock. This fails to take into account the integrity and interconnectivity of the Earth system, the depletion of ecosystem functionality and the inability of damaged ecosystems to “renew” apparently renewable resources (see also Suárez-Eiroa et al., 2019).
Indeed, as recycling science continues to improve, formerly unrenewable resources, such as metals, are becoming more renewable than formerly renewable resources (Skene and Murray, 2017). For example, industrial deforestation leads to soil compaction and erosion, damaging the soil and its ability to regenerate and support reforestation. Tree pathogenesis have increased, driven by environmental perturbation (Buras and Menzel, 2019). Furthermore, any perturbation will be communicated through real-time feedback into every component of the Earth system, leading to emergent, unpredictable and non-linear outcomes.
Green accounting
Given the fact that GDP fails to include the degradation and depletion of natural capital (Carson, 1995), and the importance of both natural resources and the environment in terms of food security, water security, energy security, resource security and national security (Figure 2), a new way of incorporating these costs was developed under the umbrella term of green accounting. One of the most significant approaches in the USA was devised by the Bureau of Economic Analysis and termed the integrated economic and environmental satellite accounts (IESSA) (Carson, 1995). The term “satellite accounts” referred to the idea that these would supplement, rather than replace existing accounts. The IEESA calculates net domestic product (NDP), which represents gross domestic product (GDP) minus depletion of natural resources in addition to depreciation of structures and equipment. Estimates of this type, in terms of all natural and environmental resources would allow an assessment of whether or not the current level of GDP can be maintained by a given nation's natural resource base.
The United Nations' System of Environmental and Economic Accounting (SEEA), established in 2012, had two new accounting aggregates: depletion-adjusted net saving and depletion-adjusted national income (Hamilton, 2016). The Philippine Environmental and Natural Resources Accounting Project (ENRAP) was originally developed to produce accounting systems to assist the Philippine government in managing environmental and natural resource policy (Peskin, 1989). It differed from IEESA, in that it wasn't an add-on or satellite, but rather a complete environmental and non-environmental approach, wherein all inputs and outputs were measured.
It also differed from SEEA in that it included non-market goods and services. In ENRAP, the monetary value of non-marketed natural goods and services were added to the marketable goods and services by using estimated shadow prices that would be expected were these goods and services marketed (Peskin and Delos Angeles, 2001). However, this still has the issue of commodification and monetization, along with a failure to account for the system-wide connectivity.
A huge difficulty exists in terms of extractive industries positioned offshore, where pollution and resource use are externalized. Supply chains disappear over the horizon, and may not be accounted for, due to a lack of transparency or a lack of a legal framework that requires accountability. Recycling may also occur overseas or within the particular nation whose IEESA is being determined, and thus may or may not contribute positively to that nation's accounting. Furthermore, the sadly common practices of exporting unsavory aspects of our manufacturing offshore to other, mostly developing nations, while exploiting and therefore maintaining low wages in these countries, have significant ethical implications as well as damaging impacts.
Thus, accounting must cover the supply and waste chains across the entirety of their reaches for a true measurement, and requires transparency in terms of social and environmental impacts. We need to re-shore and own the perturbation of the Earth system, in our accounting if not also physically, rather than externalize it. However, the point is not to commodity Nature, but to gather feedback in order to more appropriately alter our economic activities to embed within the Earth system.
The commodification of Nature has, increasingly, been impacting upon economic policy decision-making, with instruments such as Payments for Ecosystem Services (Landell-Mills and Porras, 2002; Pagiola, 2008) and Markets for Ecosystem Services (Bayon, 2004), potentially impacting how humans relate to Nature. This is counterproductive in terms of any hope of a sustainable future (Rees, 1998; Soma, 2006). Ayres (1998), Sciubba (2001), and Szargut et al. (2002) all point to energy as a qualitative and quantitative measure of economic impact and environmental damage, and this offers an alternative approach for green accounting.”
Sustainable economics
A brief history of sustainable economics
As we have noted, early in our history, resource and energy constraints meant that careful use of available resources was paramount, with repurposing, reuse and recycling all being important aspects of daily life. Mellaart (1967) observed that in Çatal Hüyük, a Neolithic town in Anatolia, bones left from food would be repurposed as knives, punches, awls, cutlery, pins, belt hooks and many other things. The path followed thereafter became one of greater optimization toward the human condition, with less trade-offs and recycling, as we developed technologies to free ourselves from these so-called constraints, accessing more resources and energy and altering our ecological surroundings to maximize our own success. However, by the mid-nineteenth century, the realization of the consequences of this optimization were beginning to be seen as problematic.
Simmonds (1862, p.10) observed that: “One of the greatest benefits that Science can confer on man is the rendering useful those substances which being the refuse of manufactures are either got rid of at great expense, or when allowed to decompose produce disease and death”. Marx (1909, p. 120–121) noted that the recovery of industrial waste was “the second great branch of economies in the conditions of production”, the first being economies of scale. An early conceptualization of a circular economy can be found in the work of Playfair (1852, p. 165–166) who wrote that “The economy of the chemistry of art is only in imitation of what we observe in the chemistry of nature. Animals live and die; their dead bodies, passing into putridity, escape into the atmosphere, whence plants again mold them into forms of organic life; and these plants, actually consisting of a past generation of ancestors, form our present food”.
The emphasis on how sustainable practice in manufacturing can be a profitable exercise has been a major message in recent times, such as in natural capitalism and the blue economy. Acting as an incentive to companies in terms of protecting their profitability, this thinking also finds its origins in the nineteenth century. Babbage (1835, p. 217) observed that “competition between firms spontaneously resulted in a more efficient use of resources, particularly since one of its main results was the care that is taken to prevent the absolute waste of any part of the raw material in order to create as much value as possible out of inputs”.
Koller (1918, p. 1) noted that “the rational treatment and utilization of such waste products either increases very considerably the general profits of an industry or even forms a separate and not inconsiderable source of gain”. However, this really does not address the deeper issue, in terms of what an economy can contribute to environmental and social sustainability. Indeed, these historical origins of current sustainable economic theory all aim to support the business-as-usual model, while failing to clearly prioritize environmental and social functionality.
Most other schools of sustainable economics have emerged from these historic roots founded on waste-is-food, wherein waste is reduced in terms of companies utilizing waste products from other companies (industrial symbiosis, industrial metabolism and industrial ecology), forming cycles rather than linear source-to-sink approaches (closed loop economics, cradle to cradle and the circular economy), and purifying the production processes (cleaner production, green chemistry, clean technology, life cycle analysis and design for environment). Figure 3 highlights the phylogeny of current sustainable economic schools from these early beginnings.
Issues with current sustainability policy and practice
While these ideas all have merits, none of them are rooted in Earth system thinking, nor include a social dimension of any significance (Nielsen, 2007; Jensen et al., 2011; Skene, 2021a). Bocken et al. (2022) highlight several issues with the circular economy, including the facts that recycling of products can be financially, energetically and technically challenging, that the appetite for material goods continues to increase and that rebound effects can have a negative impact. Instead, they argue for a sufficiency-based circular economy. Velenturf et al. (2019) emphasize the importance of overcoming silo thinking, particularly in terms of separating material categories into isolated lines of action, emphasizing that the recognition “that resources can be mixed and flow between sectors, would have great potential to accelerate the transition toward greater circularity”. Such integrated thinking is key to systems theory, as discussed in this paper.
Murray et al. (2017, p. 377) placed emphasis on the economy serving both society and environment, defining a meaningful sustainable economy as “an economic model wherein planning, resourcing, procurement, production and reprocessing are designed and managed, as both process and output, to maximize ecosystem functioning and human wellbeing.”
To truly address the issues confronting us, this paper suggests that a completely different concept is required, rooted in much more fundamental foundations, which we term “embedded economics”. Only if our activities are resonant with the Earth system, defined by its key precepts and embedded within the greater Earth system economy, can we have any hope of avoiding extremely serious and mounting danger in terms of our continuance on the planet in some meaningful way.
However, we will not achieve this goal by focusing on it. In other words, it is a functioning system that will allow us the niche space needed, and so trying to engineer our path to sustainability in isolation (and, even, denial) of the primacy of the Earth system is a strategy of doubtful value. Additionally, any economy must strengthen social sustainability if we are to live together in such a way as to maximize wellbeing in terms of physical and mental health, community cohesion, equality and international collaboration.
The challenge of change: Myopic utilitarianism
Many economists dismiss such changes as impossible or counter-productive. Stern (2009, p. 3) wrote that “it is neither economically necessary nor ethically responsible to stop or drastically slow economic growth to manage climate change. Not only would it be analytically unsound, it would also pose severe ethical difficulties and be so politically destructive as to fail as policy”. Such isolationist, myopic utilitarianism, as described by Manes (1990), completely ignores the centrality of systems theory and the Earth system. Our prosperity is tightly embedded within the functionality of the Earth system in terms of niche space. Trade-offs and feedback determine the rate of growth, or degrowth, at every level of organization, that will produce the maximum possible entropy production at the system level (Skene, 2020b).
Both growth and maintenance of the estate of the Earth system always result in an increase in entropy overall, since the increase and maintenance of complexity within an entropic universe demands dissipation (Fenchel, 1974). Maintenance of the system as a whole requires a limit to the entropy produced from within its components (Farnsworth and Niklas, 1995), as delineated by the maximum entropy production principle.
Exceeding the maximum entropic production leads to the requirement to utilize more energy, but ultimately, the increasingly entropic environment will result in the emergent and non-linear reconfiguration of the system as a whole, representing degrowth across the system, a potentially catastrophic outcome for organisms with specialized niche space such as ourselves. Every one of the many millions of species must tailor their own economies to fit within the greater whole if they are to retain their places within the Earth system, as must we. Degrowth, represented by ecological simplification in the natural economy, is usually the outcome of exceeding the maximum entropy production at any given level of organization, and can lead to ecosystem collapse and reconfiguration.
Furthermore, the dynamic, non-linear and emergent nature of the Earth system, particularly when perturbed, can quickly lead to a very different context. The male Irish elk (Megaloceros giganteus) is thought to have become extinct due to increasingly enormous antlers, driven by sexual selection. While it has been suggested that these huge appendages led to the elk becoming trapped in forests and then more easily predated, recent work points to the increasing dietary challenge of acquiring sufficient phosphate, calcium and nitrogen to construct them due to a temperature-driven regime shift.
During the Younger Dryas period, temperatures dropped in northwest Europe and as a result, the willow-spruce community was replaced by a tundra vegetation in Ireland, with much lower levels of calcium, phosphate and nitrogen, reducing foraging efficiency. This would have led to a physiological crisis for the Irish elk (Moen et al., 1999). Furthermore, the onset of the Younger Dryas and the return to ice age conditions took only 75 years, most likely due to the collapse of lake Agassiz and the sudden disruption of the Atlantic meridional overturning circulation (Murton et al., 2010; Keigwin et al., 2018). Thus, rapid regime shift exposed the weakness of the economic strategy of the elk, which had developed a structurally profligate lifestyle, only to find it unsupportable once conditions changed.
So what are the implications of Earth system economics for our own economic activities?
Earth system economics
We can learn much from the economics of nature, but must be wary not to consider that it can be used as a complete model for human economics. Misappropriation of natural processes, lifted and applied to economic theory, is never going to provide solutions. Many of the current sustainable economics schools of thought are riddled with such misappropriation (Skene, 2018, 2021a). The Earth system cannot be described as the inspiration for a sustainable economy because its economy is far from circular. Its wealth derives from our neighboring star in the form of electromagnetic radiation, which funds this economy. Indeed, the complexity of the Biosphere is almost completely reliant upon this daily import of energy (the exception being the miniscule contribution by hydrothermal vents). The natural economy is a leaky colander, not an airtight spaceship, with 90% of energy lost between each trophic level.
The second law of thermodynamics dictates that there must be a net production of entropy, and that the Earth system, like any complex, open system, is, fundamentally, a dissipative entity. Indeed it is the flow of energy through the Earth system from the Sun that allows it to remain far from thermodynamic equilibrium (Prigogine, 1976). The clearest evidence of this is when incoming solar radiation is blocked, such as in the K/T mass extinction, by dust from the impact of an extra-terrestrial bolide (either an asteroid or a comet), leading to an impact winter and the collapse of the Biosphere (Pope et al., 1994). If the Earth system ran as a circular economy, no such collapse would have occurred. However, the flow of energy, and the waste accompanying this flow through the Biosphere, are realities. For a human economy to operate like Nature, vast amounts of capital would need to be introduced every day, akin to the incoming radiation from the Sun.
So what can the Earth system teach us and what implications do these lessons have for how we practice sustainable economics? At the outset, it is recognized that our fates lie within the Earth system, a complex, self-organizing, emergent, sub-optimal, non-linear and interconnected entity. We evolved from within it and are reliant upon it for our existence. Whether we like it or not, the Earth system is the arbitrator of our futures, and, thus, its characteristics must surely form the basis of our focus in terms of a sustainable economy. So how should these characteristics inform our sustainability thinking?
Self-organization
Complex systems develop and function as a result of the interactions that occur within them. Any conceptualization that we can steer the Earth system by deliberate intervention is baseless, given the complexity. Thus, we cannot attain sustainability by design, but rather must reduce our perturbation, allowing self-healing from within the system. Any economic program must recognize this reality. It is clear that Nature often undergoes resetting, such as fire that quickly burn through a forest, allowing new plants to germinate from seed, recycling nutrients, clearing aging, diseased trees and representing ecosystem regeneration at a local level.
However, global changes really only occur when the driver is extreme, such as when an asteroid or comet strikes the Earth, or when huge volcanic events such as the Siberian traps or Deccan traps occur (Black et al., 2018; Keller et al., 2020). Anthropogenic changes also fall into this category, such as the rapid alteration of atmospheric and hydrological chemistry.
Our own socio-economic behavior, utilizing increasingly large amounts of energy (Fischer-Kowalski and Haberl, 1998), is in line with the laws of thermodynamics, as we build increasing complex societies, but if this impacts upon the Earth system (through changes in energy flux through climate destabilization, soil erosion, fertilizer run-off, habitat destruction and species extinction), then the greater natural economy may reset, which in turn damages our economy and society.
This is a much darker form of a circular economy, where the human economy impacts upon the natural economy, which in turn impacts upon the human economy (Figure 4). The natural economy will re-organize and this will determine the success or otherwise of the human economy. This is why this paper stresses the need for an embedded economy, whose functioning must be contextualized within the Earth system as a whole in terms of decision-making, much as in Nature.
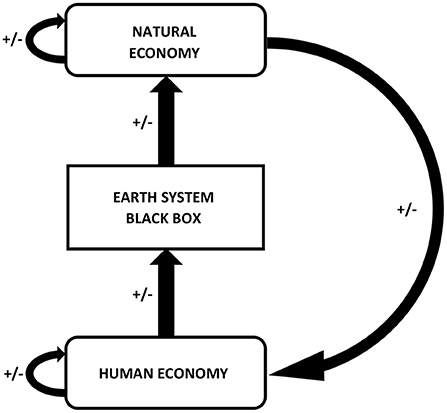
Figure 4. The circular relationship between the Earth system, the natural economy and the human economy. The Earth system black box output feeds into the natural economy, which in turn impacts either positively or negatively upon the human economy. Both economies may grow or contract depending on the overall dynamics and the thermodynamic outputs of the system and its components. In extreme cases, a complete reset may occur.
Emergence
Because of the complexity of the Earth system, the properties of the system belong to the system itself, rather than to any given component, such as ourselves. Emergent entities arise from the interactions of more fundamental entities, but cannot be reduced to those entities (Mill, 1843). Thus, we cannot construct an ecological recovery, designing our way out of trouble. Rather, the Earth system will deliver its future, and our own. Only an embedded economy, in resonance with the Earth system, can work. In order to understand what this would look like, we need to focus on the outputs of this economy, particularly in terms of its environmental and social impacts. This can only be achieved through real-time feedback (see below). The emergent nature of the Earth system requires us to be completely focused upon inputs and outputs (Figure 1), as the black box between these is beyond our cognition and represents a version of Heisenberg's uncertainty principle, but at a much greater level of uncertainty, given we are dealing with multiple unknowns, not merely velocity and position.
Sub-optimality
Perhaps the most challenging characteristic of complex systems is sub-optimality. All components must incorporate sub-optimality because it is impossible to optimize for each of the multitude of simultaneous demands across the system. Farnsworth and Niklas (1995) explain that as an increasing number of challenges are encountered, there must be increasing trade-offs in order to adequately respond to each and every one of these. Optimizing for any single component will threaten the success of the whole system, leading to failure elsewhere. For example, if we target agricultural productivity in order to maximize the energy flow to ourselves, impacts such as soil erosion, soil salinity, eutrophication, habitat destruction and water pollution will all feed back to the system as a whole, leading to a decline in soil productivity and broader issues such as fishery collapse and coral death. For example, as already noted, since the industrialization of agriculture over the last 150 years, we have lost around fifty percent of our topsoil (Pal and Chakrabortty, 2019).
Complex systems operate in such a way as to sub-optimize at the component level in order to optimize overall functionality (and entropy production) at the system level. To be embedded within the Earth system means to participate in the trade-offs required at our own level. Thus, in our example, we should use less intensive approaches, incorporating agroforestry, fallow fields, increased organic matter, reduced compaction and minimal synthetic fertilizer application. This will reduce the efficiency of agricultural output for humans, but will lead to sustainable agriculture at the broader system level. With a concomitant reduction in food waste [currently some 40% loss between field and plate (Spiker et al., 2017)], we can still feed our populations without damaging nature's functionality.
Artificial intelligence algorithms involved in economics tend to seek optimal solutions, hence exacerbating the problems (Skene, 2020a). The dangers inherent in continuing to optimize for ourselves are significant. In any situation, we must inquire at the outset as to how much sub-optimality we need to introduce. This needs to be a deliberate and calculated trade-off in all of our actions. Real-time feedback will inform us of what is appropriate. This relates strongly to the intermediate disturbance hypothesis (He et al., 2019), where diversity and functionality are optimized at the system level through sub-optimality (intermediate disturbance) at the component level.
There is a sweet spot of sub-optimality for any given component, reflected in optimizing for the system rather than any one of the components. Akin to a mixing desk in a recording studio, each aspect of our economic behavior must be adjusted for the good of the recording, not ourselves. Any complex system requires this. For example, if the sustainable development goals are to work, trade-offs must be the priority, rather than something we try to design out of the system (Skene, 2021b).
The words of de Condorcet (1955), that Nature has fixed no limits to our hopes, represent a failure to recognize a fundamental truth, that these limits are core characteristics of any functioning complex system. Only a human economy embedded within the greater economy of the Earth system, rather than struggling against it, will provide resonance with the Earth system. Trade-offs are a sign of a working system, not a failed one, and must take the top seat. What can we relinquish for the overall good? The issue is not how to design sub-optimality out of processes, but how to design it into process at sufficient levels. Current sustainable economics thinking emphasizes ecoefficiency and optimization as core characteristics, but, in reality, the polar opposite is needed.
Non-linearity
As a consequence of the complexity of the Earth system and the importance of emergence, non-linearity is a key characteristic. Dramatic, non-linear changes can and do occur when a complex system crosses a tipping point, leading to regime shift (Cooper et al., 2020). Such dramatic changes have occurred many times in Earth's history, but, given the multiple, steep gradients of change across many key ecophysiological domains that we have created, it is highly likely that these events will become much more common. Furthermore, ecological regime shifts, because of the stressors that emerge from them, can set off similar non-linear shifts across societies and financial institutions (Scheffer, 2009).
There is no certainty of reversing these shifts (Hastings and Wysham, 2010) as we head into uncharted territory due to the large number of anthropogenic assaults upon the Earth system, with resilience hugely reduced as species redundancy and habitat connectivity collapse. This has important repercussions in terms of any sustainable economic approach. The uncertainty and risk of continuing to operate in isolation from the Earth system, while increasingly perturbing it, is likely to create the conditions for catastrophic change, but with little opportunity to predict it. Thus, it would be advisable to immediately take action ex abundanti cautela (out of an abundance of caution).
Real-time feedback
Complex systems are tightly connected across and between all levels of organization. The components are unified through feedback. Trophic relations play key roles, where populations of predators and prey are tightly linked through time. Pheromones also play a significant role. One example is the response of sagebrush to simulated herbivory. Neighboring wild tobacco plants gained increased resistance to herbivores as a result. The tobacco plant was able to “eavesdrop” on what was happening to the neighboring sagebrush, alerting it to the impending threat of herbivores, and allowing it to switch on its defensive response ahead of time (Karban et al., 2003; Bilas et al., 2021).
Of course, we are unable to detect many of the signals within the Earth system. However, as already noted, technology now offers us unprecedented access to real-time feedback. Billions of smart devices and remote sensing satellites, forming the internet of things, are constantly acquiring data on social and environmental conditions, monitoring the physiology of the Earth system, as proposed by Hutton (1788). Skene (2020a) argues that remote sensing and the internet of things can analyze this data flow, informing us of the emergent outcomes of our interactions with the Earth system.
Such feedback provides us with the ability to monitor our impacts resulting from our economic behavior upon the Earth system. Robbins (1932) wrote: “Economics is the science which studies human behavior as a relationship between ends and scarce means which have alternative uses.” The point is that economics is not some independent organism, akin to some Colossus, overarching us, but, rather is the outcome of decisions, choices and actions, a behavioral science. Embedded economics remains a behavioral science, but with the emphasis on adjusting our behavior, informed by feedback from the Earth system, in such a way as to strengthen environment and society.
Feedback also informs us as to how sub-optimal we should be in our behavior, giving us insight into the system as a whole. Thus, economics must, figuratively, develop a very large pair of ears, listening for the signals that are central to the Earth system in terms of resource allocation, planning and productivity. We now have the technology to graft ourselves onto the circulatory system of information flowing through the rest of the Earth system, allowing us to adjust our inputs appropriately. True embeddedness requires connectivity.
Skene (2020b) notes that the Earth system itself is a pluriverse, wherein many different ways of being and doing exist across the natural world, dictated by geography and energy gradients. Because of the curvature of the Earth, the density of incoming solar radiation differs across the globe, being densest at the equator, and least dense at the poles. Seasonality, wind and precipitation are also impacted by these energy gradients. The location of the major land masses, and the topography also impact climate. Thus, the Earth system expresses itself differently depending on where on the planet you are and how much energetic financing and expenditure is possible.
Anthropogenic impacts also vary with location. For example, UV levels are highest near the poles, because the ozone depletion reactions occur more effectively at low temperatures (Fioletov and Shepherd, 2005). The Earth system is organized as a series of local ecosystems, embedded within the greater whole, each with its own ways of operating. Temperate rainforests, tropical rainforests, savanna, coral reefs, tundra and taiga all have their own challenges and solution spaces. Yet there is also a global element to each. Particularly, the laws of thermodynamics apply across the globe (and the cosmos), and thus for life to exist and for growth and maintenance of the living estate, free energy must be taken in and dissipated.
Indeed, the second law of thermodynamics drives increasing complexity, as this results in greater dissipation (Fenchel, 1974). Thus, an economic pluriverse is more likely to allow more appropriate embedding across the ecological and social pluriverse. This more localist approach, bringing with it shortened supply chains, culturally appropriate practice and environmentally resonant manufacturing and production methods, is much more likely to reflect the inherent differences in the many facets of the Earth system.
Globalized approaches are much less likely to be successful, as a result of the inherent characteristics of the Earth system as pluriverse. Indeed given these core characteristics, celebrating difference and ways of being, it is, surely, essential that we embed our economic activities within this pluriverse template. Only with homogeneity in social and ecological terms could a globalized free-market approach really work. Heterogeneity is the status quo in the pluriverse.
Links between society and environment: Geddes and Humboldt
In terms of transition and ways of being and doing, Geddes highlighted three important human behavioral characteristics that focus on the key psychological instruments for sustainable change and relate to the will to make a difference (Geddes, 1904; Shaw, 2017). Synergy represents the bringing together of thought and action in terms of physical engagement and co-operation. Sympathy refers to the concept of empathy, as explored by Adam Smith in his book, The Theory of Moral Sentiments (Smith, 1759). Synthesis represent the balance of heart, hand and head, underpinned by generalism across the arts, humanities, engineering and science.
These three elements can be seen in the work of Humboldt, who had a significant influence on Geddes. Humboldt referred to the living breath of nature (lebendiger hauch der Natur), considering Nature as an organic whole, born out of the harmonious inter-relationship between all living and non-living objects.
He wrote: “In considering the study of physical phenomena, not merely in its bearings on the material wants of life, but in its general influence on the intellectual advancement of mankind; we find its noblest and most important result to be a knowledge of the chain of connection, by which all natural forces are linked together, and made mutually dependent upon each other; and it is the perception of these relations that exalts our views and ennobles our enjoyments” (von Humboldt, 1997, p. 23).
This passage is extremely relevant, particularly in terms of his reference to the “knowledge of the chain of connection by which all natural forces are linked together”. If we include our resource supply chains as part of this, as we should in any embedded economy, then we can elucidate how these manufacturing chains can fit together with the larger chains within the Earth system, and manage them in an educated, ecologically sensitive manner. Knowledge requires transparency, and we can see that accountability as well as materials must flow through such chains (see, for example, Phillips and Caldwell, 2005; Hofmann et al., 2018; Gualandris et al., 2021).
By connecting our “ways of being” with that of the Earth system, we then come into resonance with it and, most importantly, act in ways that enable it, in its entirety, including humanity, to heal and diversify, simultaneously providing us with the essential basis for our own existence.
The embedded economy
What then have we learned in terms of a sustainable economy? For our economic activities to contribute to the future prospects of the human race, they must build upon the Earth system at their foundations. The reason for this is because our niche space (the multidimensional set of conditions that provides us with all that we need to live) is an emergent outcome, determined by the Earth system. The complexity of this space and the dynamic functionality of all of the manifold components that contribute to its architecture, mean that we cannot design or control it ourselves.
Therefore, we must rely on careful monitoring of the inputs of our activities and the subsequent outputs of the system, as the processes between these two signals are emergent, akin to non-symbolic artificial intelligence (Skene, 2020a) (Figure 1). The Earth system alone determines the outputs, with its myriad communication channels and dynamic non-linear responsiveness. Importantly, our inputs are not the only inputs to the system. Every component is inputting and is impacted by the combined signaling across the system. Thus, we cannot predict what will happen, only measure the outputs. This is where the internet of things and artificial intelligence can help in providing us with essential real-time feedback, allowing us to adjust our own inputs to the system.
Technology now offers us unprecedented access to feedback from the Earth system. Remote sensing from space and the billions of SMART devices linked together in the Internet of Things on the surface of the planet, offer unprecedented volumes of data. Skene (2020a) argues that artificial intelligence can analyze this data flow, informing us of the emergent outcomes of our interactions with the Earth system at scales from single leaves to entire biomes, while allowing us to understand the impact of our activities. Such feedback provides us with the ability to monitor how any changes we make in our behavior impacts upon the Earth system (Moriguchi, 2007; Rodrigues et al., 2016).
Thus, our economic activity, which represents the most significant elements of our input, must be embedded within the greater whole. In fact, in reality, we are already part of the whole, because, whether we recognize it or not, all of our activities are perceived as inputs and impact on the outputs of the Earth system as a whole and at each component level. Hence, it is not a matter of having to actively embed ourselves within the Earth system, but, rather, we only need to recognize our place in the greater system. The embedded economy must be a listening economy, tightly tuned into the impacts of our actions, at a local and global level.
An embedded economy must also be fundamentally designed to be appropriately sub-optimal at its core. This is essential. Areas of sub-optimality include massively reducing energy and material use, living less comfortably, altering our diets, reducing food waste throughout the agricultural chain, reducing fertilizer use and increasing reuse and recycling. This very much resonates with the Sufficiency-based circular economy concept of Bocken et al. (2022).
Only with appropriate trade-offs can we hope to maintain our niche space into the future. We sit on one arm of a multi-armed seesaw, wherein we must constantly adjust our position on our axis in order to maintain the balance of the whole. The Earth system is a dynamic, non-equilibrium system, constantly shifting. The system changes in order to maintain maximum entropy production, meaning that each component will operate at its own maximum level of entropy production in such a way as to maximize entropy production of the whole system (Skene, 2020b).
An economic pluriverse
An embedded economy should also work as a pluriverse, where many sizes fit all, rather than attempting to force a single peg into many differently shaped holes. An embedded economic approach needs to resonate with local conditions since landscape, climate and ecological functionality differ across the globe. Thus, a localist approach makes most sense.
Furthermore, culture can be seen to emerge from locality (Cloke and Jones, 2001), and, thus, social resilience is increased when our economic activities are in tune with the biogeography and where cultural contexts guide our economics. This is clearly seen in buen vivir economics in South America. Here, individual rights to buy, sell or own are secondary to the rights of community and environment (Dent and Peters, 2019). A pluriverse approach lies at the heart of this thinking (Escobar, 2011).
This thinking is also echoed in African philosophy. Ela (1998, p. 3) wrote “Africa is not against development. It dreams of other things than the expansion of a culture of death or an alienating modernity that destroys the fundamental values so dear to Africans… Africa sees further than an all-embracing world of material things and the dictatorship of the here and now, that insists on trying to persuade us that the only valid motto is ‘I sell therefore I am'. In a world often devoid of meaning, Africa is a reminder that there are other ways of being.”
Revisiting sustainability
Current approaches in the field of sustainable economics have a number of significant flaws. Firstly, most of them promote the ideas of eco-efficiency and a greener business-as-usual, with focus on sources and sinks rather than any acknowledgment of the Earth system as the fundamental arbitrator.
Secondly, circular economics tends to omit any acknowledgment of the significance of real-time feedback from the Earth system, while failing to provide any deliberate steering in terms of contributing to social and environmental recovery. This is partly to do with a failure to recognize that economics must be primarily a contributor to society and the environment if we are to sustain into the future.
Thirdly, given that natural systems undergo growth and degrowth as a normal part of the journey (including seasonality and mast year oscillations, where fruit trees produce vastly increased numbers of fruit every few years), and that true economic growth, if environmental and social damage are included, would likely be negative, then improved social and environmental health, with economic degrowth, will actually produce improved net economic conditions. In fact, with an embedded economy, we will have less net degrowth, as social and natural resilience will increase rather than decrease, leaving us much richer in real terms. Manufactured capital degrades social and natural resilience, incurring costs, and so a decrease in manufactured capital will increase net capital. This needs to be recognized, and completely transforms degrowth theory.
Fourthly, commodification and monetization of so called “ecosystem services” evades any real understanding of the integrity of the Earth system, and its emergent and transformative character.
Fifthly, reductionist, silo thinking prevents many schools of sustainable economics from being able to deal adequately with trade-offs and non-linearity.
Sixthly, there is a lack of focus on the key system characteristics of emergence, non-linearity, sub-optimality, feedback and self-organization.
Finally, most sustainable economics approaches fail to embrace the pluriverse, instead pursuing the standard, free-market, globalized approach.
An exception recently, is the dual circulation strategy (DCS) of China (Skene, 2022a). Central to this strategy is an attempt to release China's vast potential domestic market (domestic circulation), while balancing its foreign trade (external circulation). This new development pattern, where domestic and foreign markets can boost each other, places the domestic market as the mainstay. Supply chain issues, it is argued, are central in terms of sources and sinks. Localism, in terms of a strengthened internal market and re-homing of supply chains from extraction to consumption, brings less uncertainty.
Sanctions from the USA and trade issues with Australia, combined with the COVID pandemic excising foreign markets, have focused China's collective government mind upon the vulnerability of an export-led economy, dominant since Deng Xiaopeng's reforming policies of 1978. Aside from the protective aspects of re-homing one's economic activities, a lesson learned from the vulnerability of Europe in its dependence upon Russian gas and oil supply during the recent invasion of Ukraine, more fundamental benefits include accountability for producers and consumers within shortened supply chains and a greater awareness of the local landscape in terms of decision-making.
This has the potential to obviate Hardin's tragedy of the commons, in that if you live in the commons you are more willing to adjust your behavior in a sustainable way (see Ostrom, 1990, 1999) for further insight into commons approaches). You are much more likely not to bite the hand that feeds you if the hand lives in your neighborhood. Re-cycling, re-use and re-purposing are all more easily done within short supply chains, as the food-is-waste circle is tightened, allowing rapid and relevant circularity.
Functionality over form
Finally, an embedded economy is much more focused on process and functionality, Hutton's planetary physiology, than on structures and forms. Adaptability, resilience, energy flow and nutrient levels are core functional entities. Forms and structures, such as industrial agriculture technologies and green energy equipment, must be viewed through functional lenses, in terms of the impacts across the planet's metabolism. Functionality is a systems trait, embracing key concepts such as sub-optimality and real-time feedback, rather than the static, optimized and reductionist structuralist approach. Fundamentally, energetic relations dominate physiology, both at the cellular and the planetary level. Thus, flexibility is an important aspect to an embedded economy, responsive and alive to the feedback received from the dynamic system as a whole.
Embedded economics also significantly differs from the position taken by most sustainable economics schools in that it fundamentally challenges the accepted position on green energy technologies. Here, embedded economics emphasizes two key priorities: reduced energy use and questioning how green our green energy alternatives actually are. While it is essential to reduce greenhouse gas levels in the atmosphere, many of the sustainable energy technologies involve environmentally and socially damaging supply chains (Skene, 2021c). Important aspects such as decommissioning (Invernizzi et al., 2020) and resource criticality (Roelich et al., 2014; Velenturf et al., 2021; Mendoza et al., 2022) are increasingly being identified as significant challenges. An urgent emphasis upon reduced energy use must be the priority—a functional approach that is a core message of the embedded economy. Energy conservation represents the first tier of the energy hierarchy (Arbon, 2012). This will allow less efficient but much more environmentally friendly renewable energy technologies to be adopted, such as electric vehicles without cobalt, lithium mining without damaging indigenous populations, replacement of permanent magnets with electro-magnets (avoiding the use of rare earth metals), replacing glass fiber with bamboo or plant-based materials in wind turbines, and shifts to de-centralized energy production such as micro-hydropower (Skene, 2021c). Green energy must be truly green. Recognition that tree planting may not lead to net carbon sequestration must also be recognized (Friggens et al., 2020).
The benefits of embedded economics go far beyond the functioning of the Earth system. By re-unifying society, environment and economics, we bring greater resilience to each, whereas by fighting against the Earth system rather than integrating within it, we immediately destabilize our framework and increase stress on all three of these facets. As we have seen in Figure 2, the consequences of an isolated human economy are food, water, energy, resource, economic, social, environmental and national insecurity. These once again release the three horse riders of Malthus's apocalypse, disease, famine and war, upon humanity. Embedded economics also protects our niche space, the basis of our hopes of a sustainable future.
We have responded to many challenges in recent years, requiring decisions and actions that we could not have foreseen, such as wars, national lockdowns, travel bans and rapid vaccine development and delivery. The existential risks associated with our current economic model require more than a greening of current economic practice, and more than a singular focus on material circularity. Rather, a completely new economic approach is required, wherein economics contributes to the social and environmental crisis by being embedded within the Earth system.
The invisible hand of Smith must become a very visible embrace of the core system characteristics outlined in this paper. Tools exist to direct us. Remote sensing and the internet of things gives us access to the lifeblood of the greater system, providing feedback on its outputs. Artificial intelligence, when programmed with sufficient sub-optimality, calculated from data from the internet of things, can give us insights into how we can progress (Skene, 2022b). More fundamentally, by hanging together, as in the sense of Humboldt, social resilience and community empowerment will be strengthened. This is embedded economics, wherein we re-integrate into the greater circularity of the Earth system, rather than our own little eddy in the powerful river of energy that flows through the Biosphere.
Conclusions
At the base of any concept of sustainable economics must lie the Earth system. This paper has highlighted the key characteristics of this system, emphasizing the primacy of placing our own economics within that greater context, wherein we operate in such a way as to contribute positively to the resilience, diversification and functionality of the system as a whole. The importance of sub-optimality and real-time feedback are emphasized, wherein we adopt systems thinking in how we approach the task. Ultimately, the Earth system responds in an emergent and non-linear way, given its complexity, and so it is only as a component embedded within this system that we can find sustainability.
Humboldt's emphasis on connectivity and “hanging together” (Zusammenhang) points to this need for embeddedness. Shortening supply chains, decreasing the diameter of human economics and moving toward more immediate and rapid cycling are all properties of the economy of Nature, and of humanity for 95% of our existence, offering an insight into what has been sustainable over the course of the last 3.5 billion years in the wider natural world.
However, it is not a matter of attempting to appropriate aspects of the natural economy, but, rather, of embedding our activities within the Earth system. It is not a matter of biomimicry, where we copy small, isolated structures and components, recontextualizing them within our own, damaged economic model, but of bio-participation (Murray et al., 2017; Skene, 2021a). The natural economy is not circular, but relies on vast amounts of financing from the Sun, representing a leaky colander rather than spaceship Earth. Our interactions with it form a rapid feedback loop, operating both positively and negatively.
Essential to all of this is real-time feedback. Given the complexity of the Earth system, the best we can do is to be aware of the impacts of our activities upon the emergent outcomes of the system, informing us of our direction of travel. It is this embeddedness, and the importance of transparency, that will allow us to make the decisions as humans, in order to find a path toward our own sustainability.
Thus, this paper would suggest that an embedded economy, enhancing rather than threatening both environmental and social sustainability, whilst maintaining our niche space, should be:
- embedded within, cognizant of and focused upon the Earth system and its key characteristics of self-organization, emergence, non-linearity and sub-optimality;
- integrated across all three arenas (economics, environment and society);
- focused on real-time feedback, acting as a compass in terms of any path corrections needed;
- a pluriverse of approaches as defined by landscape and culture, as part of the natural world;
- accountable and transparent, facilitating clear and rapid decision-making;
- focused on processes, not structures.
Data availability statement
The original contributions presented in the study are included in the article/supplementary material, further inquiries can be directed to the corresponding author.
Author contributions
The author confirms being the sole contributor of this work and has approved it for publication.
Conflict of interest
The author declares that the research was conducted in the absence of any commercial or financial relationships that could be construed as a potential conflict of interest.
Publisher's note
All claims expressed in this article are solely those of the authors and do not necessarily represent those of their affiliated organizations, or those of the publisher, the editors and the reviewers. Any product that may be evaluated in this article, or claim that may be made by its manufacturer, is not guaranteed or endorsed by the publisher.
References
Allen, T. F., Tainter, J. A., and Hoekstra, T. W. (1999). Supply-side sustainability. Syst. Res. Behav. Sci. 16, 403–427.
Anderson, K. (2012). The inconvenient truth about carbon offsets. Nature 484, 7. doi: 10.1038/484007a
Arango-Restrepo, A., Rubi, J. M., and Barragán, D. (2019). The role of energy and matter dissipation in determining the architecture of self-assembled structures. J. Phys. Chem. B 123, 5902–5908. doi: 10.1021/acs.jpcb.9b02928
Arbon, I. (2012). “The energy hierarchy approach to optimum use of energy infrastructure-sharing ideas from the UK and other parts of Europe,” in Proceedings of the International Symposium on Engineering Lessons Learned from the 2011 Great East Japan Earthquake, March 1-4, Tokyo.
Ayres, R. U. (1998). Eco-thermodynamics: economics and the second law. Ecol. Econ. 26, 189–209. doi: 10.1016/S0921-8009(97)00101-8
Bayon, R. (2004). Making Environmental Markets Work: Lessons From Early Experience With Sulfur, Carbon, Wetlands, And Other Related Markets. Locarno: Forest Trends, Katoomba Group Meeting.
Bedau, M. A., and Humphreys, P. E. (2008). Emergence: Contemporary Readings in Philosophy and Science. Boston, MA: MIT Press.
Biggs, R., Blenckner, T., Folke, C., Gordon, L., Norström, A., Nyström, M., et al. (2012). “Regime shifts”, in Encyclopedia of Theoretical Ecology, eds. A. Hastings and L. J. Gross (Berkeley, CA: University of California Press), 609–617.
Bilas, R. D., Bretman, A., and Bennett, T. (2021). Friends, neighbours and enemies: an overview of the communal and social biology of plants. Plant Cell. Environ. 44, 997–1013. doi: 10.1111/pce.13965
Black, B. A., Neely, R. R., Lamarque, J. F., Elkins-Tanton, L. T., Kiehl, J. T., Shields, C. A., et al. (2018). Systemic swings in end-Permian climate from Siberian Traps carbon and sulfur outgassing. Nature Geosci. 11, 949–954. doi: 10.1038/s41561-018-0261-y
Blackburn, R. H. (1971). Honey in the Ogiek Personality Culture and Society. East Lansing, MI: Michigan State University.
Bocken, N. M., Niessen, L., and Short, S. W. (2022). The Sufficiency-based circular economy—an analysis of 150 companies. Front. Sustain. 3, 289. doi: 10.3389/frsus.2022.899289
Böhringer, C., and Vogt, C. (2004). The dismantling of a breakthrough: the Kyoto Protocol as symbolic policy. Eur. J. Polit. Econ. 20, 597–617. doi: 10.1016/j.ejpoleco.2004.02.004
Braakman, R., Follows, M. J., and Chisholm, S. W. (2017). Metabolic evolution and the self-organization of ecosystems. PNAS 114, E3091–E3100. doi: 10.1073/pnas.1619573114
Bruinsma, R. F., Gelbart, W. M., Reguera, D., Rudnick, J., and Zandi, R. (2003). Viral self-assembly as a thermodynamic process. Phys. Rev. Lett. 90, 248101. doi: 10.1103/PhysRevLett.90.248101
Buras, A., and Menzel, A. (2019). Projecting tree species composition changes of European forests for 2061–2090 under RCP 4.5 and RCP 8.5 scenarios. Front. Plant Sci. 9, 1986. doi: 10.3389/fpls.2018.01986
Burke, M., Davis, W. M., and Diffenbaugh, N. S. (2018). Large potential reduction in economic damages under UN mitigation targets. Nature 557, 549–553. doi: 10.1038/s41586-018-0071-9
Carson, C. S. (1995). Integrated economic and environmental satellite accounts. Nonrenewable Resour. 4, 12–33. doi: 10.1007/BF02257015
Chen, X., Memon, H. A., Wang, Y., Marriam, I., and Tebyetekerwa, M. (2021). Circular economy and sustainability of the clothing and textile Industry. Mater. Circ. Econ. 3, 1–9. doi: 10.1007/s42824-021-00026-2
Clay, S. A. (2021). Near-term challenges for global agriculture: Herbicide-resistant weeds. Agron. J. 113, 4463–4472. doi: 10.1002/agj2.20749
Cloke, P., and Jones, O. (2001). Dwelling, place, and landscape: an orchard in Somerset. Environ. Plan A 33, 649–666. doi: 10.1068/a3383
Coffee, Jr. J. C. (1980). Making the punishment fit the corporation: the problem of finding an optimal corporation criminal sanction. North. Ill. Univ. Law Rev. 3, 3–36.
Cooper, G. S., Willcock, S., and Dearing, J. A. (2020). Regime shifts occur disproportionately faster in larger ecosystems. Nature Commun. 11, 1–10. doi: 10.1038/s41467-020-15029-x
Costanza, R., d'Arge, R., de Groot, R., Farber, S., Grasso, M., Hannon, B., et al. (1997). The value of the world's ecosystem services and natural capital. Nature 387, 253–260. doi: 10.1038/387253a0
de Condorcet, M. J. A. (1955). Sketch for a Historical Picture of the Progress of the Human Mind. Translated by June Barraclough. London: Weidenfeld and Nicolson.
de Molina, M. G., and Toledo, V. M. (2014). The Social Metabolism: a Socio-ecological Theory of Historical Change, Vol. 3. London: Springer.
Dent, M., and Peters, B. (2019). The Crisis of Poverty and Debt in the Third World. Abingdon: Routledge.
Desrochers, P. (2000). Market processes and the closing of “industrial loops”: a historical reappraisal. J. Ind. Ecol. 4, 29–43. doi: 10.1162/108819800569276
Desrochers, P. (2008). Did the invisible hand need a regulatory glove to develop a green thumb? Some historical perspective on market incentives, win-win innovations and the Porter hypothesis. Environ. Resour. Econ. 41, 519–539. doi: 10.1007/s10640-008-9208-x
Dhurua, S., and Gujar, G. T. (2011). Field-evolved resistance to Bt toxin Cry1Ac in the pink bollworm, Pectinophora gossypiella (Saunders) (Lepidoptera: Gelechiidae), from India. Pest Manag. Sci. 67, 898–903. doi: 10.1002/ps.2127
Diaz, S., Pascual, U., Stenseke, M., Martin-López, B., Watson, R. T., Molnár, Z., et al. (2018). Assessing nature's contributions to people. Science 359, 270–272. doi: 10.1126/science.aap8826
Dutcher, D. D., Finley, J. C., Luloff, A. E., and Johnson, J. B. (2007). Connectivity with nature as a measure of environmental values. Environ. Behav. 39, 474–493. doi: 10.1177/0013916506298794
Ela, J.-M. (1998). Western Development has Failed: Looking to a New Africa. Paris: Le Monde Diplomatique.
Escobar, A. (2011). Sustainability: design for the pluriverse. Development 54, 137–140. doi: 10.1057/dev.2011.28
Farnsworth, K. D., and Niklas, K. J. (1995). Theories of optimization, form and function in branching architecture in plants. Funct. Ecol. 9, 355–363. doi: 10.2307/2389997
Fenchel, T. (1974). Intrinsic rate of natural increase: the relationship with body size. Oecologia 14, 317–326. doi: 10.1007/BF00384576
Ferguson, C. E., Bennett, N. J., Kostka, W., Richmond, R. H., and Singeo, A. (2022). The tragedy of the commodity is not inevitable: indigenous resistance prevents high-value fisheries collapse in the Pacific islands. Glob. Environ. Change 73, 102477. doi: 10.1016/j.gloenvcha.2022.102477
Fioletov, V. E., and Shepherd, T. G. (2005). Summertime total ozone variations over middle and polar latitudes. Geophys. Res. Lett. 32, 80. doi: 10.1029/2004GL022080
Fischer-Kowalski, M., and Haberl, H. (1998). Sustainable development: socio-economic metabolism and colonization of nature. Int. Soc. Sci. J. 50, 573–587. doi: 10.1111/1468-2451.00169
Folke, C., Biggs, R., Norstrom, A. V., Reyers, B., and Rockstrom, J. (2016). Social-ecological resilience and biosphere-based sustainability science. Ecol. Soc. 21, 41. doi: 10.5751/ES-08748-210341
Friggens, N. L., Hester, A. J., Mitchell, R. J., Parker, T. C., Subke, J. A., and Wookey, P. A. (2020). Tree planting in organic soils does not result in net carbon sequestration on decadal timescales. Glob. Change Biol. 26, 5178–5188. doi: 10.1111/gcb.15229
Fujii, H., and Managi, S. (2013). Which industry is greener? An empirical study of nine industries in OECD countries. Energy Policy 57, 381–388. doi: 10.1016/j.enpol.2013.02.011
Fuller, R., Landrigan, P. J., Balakrishnan, K., Bathan, G., Bose-O'Reilly, S., Brauer, M., et al. (2022). Pollution and health: a progress update. Lancet Planet. Health. 6:e545–547. doi: 10.1016/S2542-5196(22)00090-0
Geddes, P. (1904). Civics: as applied sociology, Part I. Sociological Papers 1904, 105. doi: 10.1177/0038026104SP100110
Gómez-Baggethun, E., De Groot, R., Lomas, P. L., and Montes, C. (2010). The history of ecosystem services in economic theory and practice: from early notions to markets and payment schemes. Ecol. Econ. 69, 1209–1218. doi: 10.1016/j.ecolecon.2009.11.007
Gómez-Baggethun, E., and Ruiz-Pérez, M. (2011). Economic valuation and the commodification of ecosystem services. Prog. Phys. Geog. 35, 613–628. doi: 10.1177/0309133311421708
Gualandris, J., Longoni, A., Luzzini, D., and Pagell, M. (2021). The association between supply chain structure and transparency: a large-scale empirical study. J. Oper. Manag. 67, 803–827. doi: 10.1002/joom.1150
Halopka, R. (2017). The High Cost of Erosion. Farm Progress. Available online at: www.farmprogress.com/soil-health/high-cost-soil-erosion (accessed August 29, 2022).
Hamilton, K. (2016). Measuring sustainability in the UN system of environmental-economic accounting. Environ. Resour. Econ. 64, 25–36. doi: 10.1007/s10640-015-9924-y
Hardin, G. (1968). The tragedy of the commons. Science 162, 1243–1248. doi: 10.1126/science.162.3859.1243
Hastings, A., and Wysham, D. B. (2010). Regime shifts in ecological systems can occur with no warning. Ecol. lett. 13, 464–472. doi: 10.1111/j.1461-0248.2010.01439.x
Hayden, B. (1981). “Subsistence and ecological adaptations of modern hunter-gatherers”, in Omnivorous Primates: Gathering and Hunting in Human Evolution, eds. R. S. O. Harding and G. Teleki (New York, NY: New Columbia University Press), 344–441.
He, T., Lamont, B. B., and Pausas, J. G. (2019). Fire as a key driver of Earth's biodiversity. Biol. Rev. 94, 1983–2010. doi: 10.1111/brv.12544
Hofmann, H., Schleper, M. C., and Blome, C. (2018). Conflict minerals and supply chain due diligence: an exploratory study of multi-tier supply chains. J. Bus. Ethics 147, 115–141. doi: 10.1007/s10551-015-2963-z
Howarth, N. A., and Foxall, A. (2010). The Veil of Kyoto and the politics of greenhouse gas mitigation in Australia. Polit. Geogr. 29, 167–176. doi: 10.1016/j.polgeo.2010.03.001
Hutton, J. (1788). Theory of the earth. Trans. Roy. Soc. Edin. 1, 209–304. doi: 10.1017/S0080456800029227
Invernizzi, D. C., Locatelli, G., Velenturf, A. P., Love, P. E., Purnell, P., and Brookes, N. J. (2020). Developing policies for the end-of-life of energy infrastructure: coming to terms with the challenges of decommissioning. Energy Policy 144, 111677. doi: 10.1016/j.enpol.2020.111677
Jensen, P. D., Basson, L., and Leach, M. (2011). Reinterpreting industrial ecology. J. Ind. Ecol. 15, 680–692. doi: 10.1111/j.1530-9290.2011.00377.x
Jørgensen, D. (2015). Rethinking rewilding. Geoforum 65, 482–488. doi: 10.1016/j.geoforum.2014.11.016
Jorgenson, A. K., and Clark, B. (2011). Societies consuming nature: a panel study of the ecological footprints of nations, 1960–2003. Soc. Sci. Res. 40, 226–244. doi: 10.1016/j.ssresearch.2010.09.004
Kaika, D., and Zervas, E. (2013). The environmental Kuznets curve (EKC) theory. Part B: critical issues. Energy Policy 62, 1403–1411. doi: 10.1016/j.enpol.2013.07.130
Kanter, D. R., Ogle, S. M., and Winiwarter, W. (2020). Building on Paris: integrating nitrous oxide mitigation into future climate policy. Curr. Opin. Environ. Sustain. 47, 7–12. doi: 10.1016/j.cosust.2020.04.005
Karban, R., Maron, J., Felton, G. W., Ervin, G., and Eichenseer, H. (2003). Herbivore damage to sagebrush induces resistance in wild tobacco: evidence for eavesdropping between plants. Oikos 100, 325–332. doi: 10.1034/j.1600-0706.2003.12075.x
Keigwin, L. D., Klotsko, S., Zhao, N., Reilly, B., Giosan, L., and Driscoll, N. W. (2018). Deglacial floods in the beaufort sea preceded younger dryas cooling. Nat. Geosci. 11, 599–604. doi: 10.1038/s41561-018-0169-6
Keller, G., Mateo, P., Monkenbusch, J., Thibault, N., Punekar, J., Spangenberg, J. E., et al. (2020). Mercury linked to Deccan Traps volcanism, climate change and the end-Cretaceous mass extinction. Glob. Planet. Change 194, 103312. doi: 10.1016/j.gloplacha.2020.103312
Kleidon, A., Malhi, Y., and Cox, P. M. (2010). Maximum entropy production in environmental and ecological systems. Proc. R. Soc. Lond. B 365, 1297–1302. doi: 10.1098/rstb.2010.0018
Koller, T. (1918). The Utilization of Waste Products: A Treatise on the Rational Utilization, Recovery and Treatment of Waste Products of all Kinds. Third Edition. New York, NY: D. Van Nostrand Company.
Kupa'a, K., Winter, K. B., Vaughan, M. B., Barca, N., and Price, M. R. (2021). Understanding the co-evolutionary relationships between Indigenous cultures and non-native species can inform more effective approaches to conservation: the example of pigs (pua'a; Sus scrofa) in Hawaii. Pac. Conserv. Biol. 27, 442–450. doi: 10.1071/PC20086
Lade, S. J., Tavoni, A., Levin, S. A., and Schlüter, M. (2013). Regime shifts in a social-ecological system. Theor. Ecol. 6, 359–372. doi: 10.1007/s12080-013-0187-3
Landell-Mills, N., and Porras, I. T. (2002). Silver Bullet or Fool's Gold? A Global Review of Markets for Environmental Services and their Impact on the Poor. London: IIED.
Lohmann, L. (2010). “Neoliberalism and the calculable world: the rise of carbon trading”, in: The Rise and Fall of Neo-liberalism: the Collapse of an Economic Order?, eds. K. Birch, V. Mykhnenko, and K. Trebeck (London: Zed Books), 77–93.
Macintosh, A. (2012). The Australia clause and REDD: a cautionary tale. Climatic Change 112, 169–188. doi: 10.1007/s10584-011-0210-x
Manes, C. (1990). Green Rage: Radical Environmentalism and the Unmaking of Civilization. New York, NY: Back Bay Books.
Marx, K. (1909). Capital: A Critique of Political Economy. Volume III: The Process of Capitalist Production as a Whole, ed. Frederick Engels. Trans. from the first German edition by Ernest Untermann. Chicago, IL: Charles H. Kerr and Company Cooperative.
Mendoza, J. M. F., Gallego-Schmid, A., Velenturf, A. P., Jensen, P. D., and Ibarra, D. (2022). Circular economy business models and technology management strategies in the wind industry: Sustainability potential, industrial challenges and opportunities. Renew. Sust. Energ. Rev. 163, 112523. doi: 10.1016/j.rser.2022.112523
Mill, J. S. (1843). A System of Logic, Ratiocinative and Inductive: Being a Connected View of the Principles of Evidence and the Methods of Scientific Investigation. New York, NY: John W. Parker.
Moen, R. A., Pastor, J., and Cohen, Y. (1999). Antler growth and extinction of Irish elk. Evol. Ecol. Res. 1, 235–249.
Moriguchi, Y. (2007). Material flow indicators to measure progress toward a sound material-cycle society. J. Mater. Cycles Waste Manag. 9, 112–120. doi: 10.1007/s10163-007-0182-0
Muchemi, J. G., and Ehrensperger, A. (2011). Ogiek Peoples Ancestral Territories Atlas: Safeguarding Territories, Cultures and Natural Resources of Ogiek Indigenous People in the Eastern Mau Forest, Kenya. Nairobi/Bern: ERMIS Africa and CDE.
Mudd, G. M. (2010). The environmental sustainability of mining in Australia: key mega-trends and looming constraints. Resour. Policy 35, 98–115. doi: 10.1016/j.resourpol.2009.12.001
Murray, A., Skene, K., and Haynes, K. (2017). The circular economy: an interdisciplinary exploration of the concept and application in a global context. J. Bus. Ethics 140, 369–380. doi: 10.1007/s10551-015-2693-2
Murton, J. B., Bateman, M. D., Dallimore, S. R., Teller, J. T., and Yang, Z. (2010). Identification of younger dryas outburst flood path from lake agassiz to the arctic ocean. Nature 464, 740–743. doi: 10.1038/nature08954
Naik, V., Pusadkar, P. P., Waghmare, S. T., Raghavendra, K. P., Kranthi, S., Kumbhare, S., et al. (2020). Evidence for population expansion of cotton pink bollworm Pectinophora gossypiella (Saunders) (Lepidoptera: Gelechiidae) in India. Sci. Rep. 10, 1–11. doi: 10.1038/s41598-020-61389-1
Nielsen, S. N. (2007). What has modern ecosystem theory to offer to cleaner production, industrial ecology and society? The views of an ecologist. J. Clean. Prod. 15, 1639–1653. doi: 10.1016/j.jclepro.2006.08.008
Nordhaus, W. D., and Tobin, J. (1973). “Is growth obsolete?”, in The Measurement of Economics and Social Performance. Studies in Income and Wealth. Vol. 38, ed M. Moss (Cambridge, MA: National Bureau of Economic Research), 509–532.
Norstedt, G., Axelsson, A. L., Östlund, L., and Giguère, N. (2014). Exploring pre-colonial resource control of individual Sami households. Arctic 67, 223–237. doi: 10.14430/arctic4389
Odeku, K. O., and Gundani, S. R. (2017). Accentuating criminal sanctions for environmental degradation: issues and perspectives. Environ. Econ. 8, 28–37. doi: 10.21511/ee.08(2).2017.03
Odum, H. T. (1988). Self-organization, transformity, and information. Science 242, 1132–1139. doi: 10.1126/science.242.4882.1132
Odum, H. T. (1995). “Self-organization and maximum empower”, in Maximum Power: The Ideas and Applications of H.T. Odum, ed. C. A. S. Hall (Colorado: Colorado University Press), 311–330.
Ostrom, E. (1990). Governing the Commons: The Evolution of Institutions for Collective Action. Cambridge: Cambridge University Press.
Ostrom, E. (1999). Coping with tragedies of the commons. Annu. Rev. Polit. Sci. 2, 493–535. doi: 10.1146/annurev.polisci.2.1.493
Ottenberg, S., and Ottenberg, P. (1960). Cultures and Societies of Africa. New York, NY: Random House.
Pagiola, S. (2008). Payments for environmental services in Costa Rica. Ecol. Econ. 65, 712–724. doi: 10.1016/j.ecolecon.2007.07.033
Pal, S. C., and Chakrabortty, R. (2019). Modelling of water induced surface soil erosion and the potential risk zone prediction in a sub-tropical watershed of Eastern India. MESE 2, 369–393. doi: 10.1007/s40808-018-0540-z
Palmer, M. A., Zedler, J. B., and Falk, D. A. (2016). “Ecological theory and restoration ecology,” in Foundations of Restoration Ecology, eds. M. A. Palmer, J. B. Zedler, D. A. Falk (Washington, DC: Island Press), 3–26.
Peskin, H. M. (1989). Accounting for Natural Resource Depletion and Degradation in Developing Countries. Environmental Department Working Paper No. 13. Washington, DC: The World Bank.
Peskin, H. M., and Delos Angeles, M. S. (2001). Accounting for environmental services: contrasting the SEEA and the ENRAP approaches. Rev. Income Wealth 47, 203–219. doi: 10.1111/1475-4991.00012
Phenrat, T., Soratana, K., Kumar, V., Kanel, S. R., and Landis, A. E. (2022). Technological and policy innovations toward cleaner development. Clean Technol. Environ. Policy 24, 1009–1011. doi: 10.1007/s10098-022-02312-2
Phillips, R., and Caldwell, C. B. (2005). Value chain responsibility: a farewell to arm's length. Bus. Soc. Rev. 110, 345–370. doi: 10.1111/j.0045-3609.2005.00020.x
Playfair, L. (1852). “On the chemical principles involved in the manufactures of the Exhibitionas indicating the necessity of industrial instruction”, in Lectures on the Results of the Great Exhibition of 1851, ed D. Bogue (London: Royal Society of Arts Great Britain), 147–208.
Pope, K. O., Baines, K. H., Ocampo, A. C., and Ivanov, B. A. (1994). Impact winter and the Cretaceous/Tertiary extinctions: results of a Chicxulub asteroid impact model. Earth Planet. Sci. Lett. 128, 719–725. doi: 10.1016/0012-821X(94)90186-4
Prigogine, I. (1976). “Order through fluctuation: self-organization and social system”, in Evolution and Consciousness: Human Systems in Transition, eds. E. Jantsch and C. H. Waddington (London: Addison-Wesley), 93–130.
Rabalais, N. N., Turner, R. E., Diaz, R. J., and Justić, D. (2009). Global change and eutrophication of coastal waters. ICES J. Mar. Sci. 66, 1528–1537. doi: 10.1093/icesjms/fsp047
Ravallion, M. (2018). Inequality and globalization: a review essay. J. Econ. Lit. 56, 620–642. doi: 10.1257/jel.20171419
Raworth, K. (2017). A Doughnut for the Anthropocene: humanity's compass in the 21st century. Lancet Planet. Health 1, e48–e49. doi: 10.1016/S2542-5196(17)30028-1
Reeder-Myers, L., Braje, T. J., Hofman, C. A., Elliott Smith, E. A., Garland, C. J., Grone, M., et al. (2022). Indigenous oyster fisheries persisted for millennia and should inform future management. Nat. Commun. 13, 1–13. doi: 10.1038/s41467-022-29818-z
Rees, W. E. (1998). How should a parasite value its host? Ecol. Econ. 25, 49–52. doi: 10.1016/S0921-8009(98)00015-9
Richter, D. D., and Billings, S. A. (2015). ‘One physical system': Tansley's ecosystem as Earth's critical zone. New Phytol. 206, 900–912. doi: 10.1111/nph.13338
Rodrigues, V. P., Pigosso, D. C. A., and McAloone, T. C. (2016). Process-related key performance indicators for measuring sustainability performance of ecodesign implementation into product development. J. Clean. Prod. 139:416–428. doi: 10.1016/j.jclepro.2016.08.046
Roelich, K., Dawson, D. A., Purnell, P., Knoeri, C., Revell, R., Busch, J., et al. (2014). Assessing the dynamic material criticality of infrastructure transitions: a case of low carbon electricity. Appl. Energy 123, 378–386. doi: 10.1016/j.apenergy.2014.01.052
Scheffer, M. (2009). Critical Transitions in Nature and Society. Princeton, NJ: Princeton University Press.
Scheffer, M., Carpenter, S., Foley, J. A., Folke, C., and Walker, B. (2001). Catastrophic shifts in ecosystems. Nature 413, 591–596. doi: 10.1038/35098000
Schellnhuber, H. J. (1999). ‘Earth system' analysis and the second copernican revolution. Nature 402, C19-C23. doi: 10.1038/35011515
Schnaiberg, A. (1980). The Environment: From Surplus to Scarcity. New York, NY: Oxford University Press.
Sciubba, E. (2001). Beyond thermoeconomics? The concept of extended exergy accounting and its application to the analysis and design of thermal systems. Int. J. Exergy 1, 68–84. doi: 10.1016/S1164-0235(01)00012-7
Shaw, M. (2017). “Aestheticism and decadence in Patrick Geddes's socioeconomics,” in Victorian Sustainability in Literature and Culture, ed. W. Parkins (Abingdon: Routledge), 165–179.
Skene, K. R. (2015). Life's a gas: a thermodynamic theory of biological evolution. Entropy 17, 5522–5548. doi: 10.3390/e17085522
Skene, K. R. (2018). Circles, spirals, pyramids and cubes: why the circular economy cannot work. Sustain. Sci. 13, 479–492. doi: 10.1007/s11625-017-0443-3
Skene, K. R. (2020a). Artificial Intelligence and the Environmental Crisis: Can Technology Really Save the World? Abington: Routledge.
Skene, K. R. (2020b). In pursuit of the framework behind the biosphere: S-curves, self-assembly and the genetic entropy paradox. BioSystems 190, 104101. doi: 10.1016/j.biosystems.2020.104101
Skene, K. R. (2021a). Sustainability policy and practice: is nature an appropriate mentor? Environ. Dev. Sustain. 23, 18167–18185. doi: 10.1007/s10668-021-01432-x
Skene, K. R. (2021b). No goal is an island: the implications of systems theory for the sustainable development goals. Environment. Environ. Dev. Sustain. 23, 9993–10012. doi: 10.1007/s10668-020-01043-y
Skene, K. R. (2021c). The dark shadows of the jolly green giants: urgent policy and research priorities in renewable energy technologies. Sustain. Clim. Change 14, 335–357. doi: 10.1089/scc.2021.0035
Skene, K. R. (2022a). “Chapter 15. Steering the circular economy: a new role for Adam Smith's invisible hand,” in Sustainability and the Circular Economy, eds. A. Stefanakis and I. Nikolaou (London: Elsevier), 21–33.
Skene, K. R. (2022b). “The circular economy: a critique of the concept,” in Towards a Circular Economy: Transdisciplinary Approach for Business, eds. A. Alvarez-Risco, M. A. Rosen and S. Del-Aguila-Arcentales (Cham: Springer), 99–116.
Skene, K. R., and Murray, A. (2017). Sustainable Economics: Context, Challenges and Opportunities for the 21st-Century Practitioner. Abington: Routledge.
Solow, R. M. (1974). The economics of resources or the resources of economics. Am. Econ. Rev. 64, 1–14. doi: 10.1057/9780230523210_13
Soma, K. (2006). Natura economica in environmental valuation. Environ. Values 15, 31–50. doi: 10.3197/096327106776678979
Spash, C. L. (2010). The brave new world of carbon trading. New Political Econ. 15, 169–195. doi: 10.1080/13563460903556049
Spiker, M. L., Hiza, H. A., Siddiqi, S. M., and Neff, R. A. (2017). Wasted food, wasted nutrients: nutrient loss from wasted food in the United States and comparison to gaps in dietary intake. J. Acad. Nutr. Diet. 117, 1031–1040. doi: 10.1016/j.jand.2017.03.015
Steffen, W., Grinevald, J., Crutzen, P., and McNeill, J. (2011). The Anthropocene: conceptual and historical perspectives. Philos. Trans. R. Soc. A 369, 842–867. doi: 10.1098/rsta.2010.0327
Steffen, W., Richardson, K., Rockström, J., Schellnhuber, H. J., Dube, O. P., Dutreuil, S., et al. (2020). The emergence and evolution of Earth System Science. Nat. Rev. Earth Environ. 1, 54–63. doi: 10.1038/s43017-019-0005-6
Stern, D. I., Common, M. S., and Barbier, E. B. (1994). “Economic growth and environmental degradation: a critique of the environmental Kuznets curve,” in Discussion Papers in Environmental Economics and Environmental Management No. 9409. Heslington, York: University of York.
Stern, N. (2009). A Blueprint for a Safer Planet: How we can Save the World and Create Prosperity. London: The Bodley Head.
Suárez-Eiroa, B., Fernández, E., Méndez-Martínez, G., and Soto-Oñate, D. (2019). Operational principles of circular economy for sustainable development: linking theory and practice. J. Clean. Prod. 214, 952–961. doi: 10.1016/j.jclepro.2018.12.271
Szargut, J., Ziebik, A., and Stanek, W. (2002). Depletion of the non-renewable natural exergy resources as a measure of the ecological cost. Energy Convers. Manag. 43, 1149–1163. doi: 10.1016/S0196-8904(02)00005-5
Turner, R. K. (2000). Markets and Environmental Quality. CSERGE Working Paper PA 2000-01. London: Centre for Social and Economic Research on the Global Environment.
Velenturf, A. P., Archer, S. A., Gomes, H. I., Christgen, B., Lag-Brotons, A. J., and Purnell, P. (2019). Circular economy and the matter of integrated resources. Sci. Total Environ. 689, 963–969. doi: 10.1016/j.scitotenv.2019.06.449
Velenturf, A. P., and Purnell, P. (2021). Principles for a sustainable circular economy. Sustain. Prod. Consum. 27, 1437–1457. doi: 10.1016/j.spc.2021.02.018
Velenturf, A. P. M., Purnell, P., and Jensen, P. D. (2021). Reducing material criticality through circular business models: challenges in renewable energy. One Earth 4, 350–352. doi: 10.1016/j.oneear.2021.02.016
von Bertalanffy, L. (1933). Modern Theories of Development: An Introduction to Theoretical Biology. London: Oxford University Press.
von Humboldt, A. (1997). Cosmos: A Sketch of the Physical Description of the Universe, trans. E.C. Otté. Baltimore, MD: Johns Hopkins University Press.
Westman, W. (1977). How much are Nature's services worth? Science 197, 960–964. doi: 10.1126/science.197.4307.960
Winterhalder, B. (2001). “The behavioural ecology of hunter-gatherers,” in Hunter-gatherers: An Inter-disciplinary Perspective, eds. C. Panter-Brick, R. Layton and P. Rowley-Conwy (Cambridge: Cambridge University Press), 13–38.
Woodward, D. (1985). “Swords into ploughshares”: recycling in pre-industrial England. Econ. Hist. Rev. 38, 175–191. doi: 10.2307/2597142
World Bank (2022). Trends in Solid Waste Management. Available online at: https://datatopics.worldbank.org/what-a-waste/trends_in_solid_waste_management.html (accessed August 22, 2022).
WWAP (United Nations World Water Assessment Programme) (2017). The United Nations World Water Development Report 2017: Wastewater, The Untapped Resource. Paris, UNESCO.
Keywords: circular economy, earth system, embedded economics, emergence, non-linearity, self-organization, sub-optimality, zusammenhang
Citation: Skene KR (2022) How can economics contribute to environmental and social sustainability? The significance of systems theory and the embedded economy. Front. Sustain. 3:980583. doi: 10.3389/frsus.2022.980583
Received: 28 June 2022; Accepted: 23 August 2022;
Published: 09 September 2022.
Edited by:
Anne P. M. Velenturf, University of Leeds, United KingdomReviewed by:
Paul D. Jensen, University of Leeds, United KingdomSøren Nors Nielsen, Aalborg University, Denmark
Copyright © 2022 Skene. This is an open-access article distributed under the terms of the Creative Commons Attribution License (CC BY). The use, distribution or reproduction in other forums is permitted, provided the original author(s) and the copyright owner(s) are credited and that the original publication in this journal is cited, in accordance with accepted academic practice. No use, distribution or reproduction is permitted which does not comply with these terms.
*Correspondence: Keith R. Skene, a3Jza2VuZUBiaW9zcmkub3Jn