- 1Metsähovi Radio Observatory, Aalto University, Espoo, Finland
- 2Department of Electronics and Nanoengineering, Aalto University, Espoo, Finland
- 3Tartu Observatory, Tõravere, Estonia
The large-scale environment is believed to affect the evolution and intrinsic properties of galaxies. It offers a new perspective on narrow-line Seyfert 1 galaxies (NLS1) which have not been extensively studied in this context before. We study a large and diverse sample of 960 NLS1 galaxies using a luminosity-density field constructed using Sloan Digital Sky Survey. We investigate how the large-scale environment is connected to the properties of NLS1 galaxies, especially their radio loudness. Furthermore, we compare the large-scale environment properties of NLS1 galaxies with other active galactic nuclei (AGN) classes, for example, other jetted AGN and broad-line Seyfert 1 (BLS1) galaxies, to shed light on their possible relations. In general NLS1 galaxies reside in less dense large-scale environments than any of our comparison samples, thus supporting their young age. The average luminosity-density and distribution to different luminosity-density regions of NLS1 sources is significantly different compared to BLS1 galaxies. This contradicts the simple orientation-based unification of NLS1 and BLS1 galaxies, and weakens the hypothesis that BLS1 galaxies are the parent population of NLS1 galaxies. The large-scale environment density also has an impact on the intrinsic properties of NLS1 galaxies; the radio loudness increases with the increasing luminosity-density. However, our results suggest that the NLS1 population is indeed heterogeneous, and that a considerable fraction of them are misclassified. We support a suggested description that the traditional classification based on the radio loudness should be replaced with the division to jetted and non-jetted sources.
1. Introduction
An intriguing class of active galactic nuclei (AGN) are narrow-line Seyfert 1 (NLS1) galaxies. They are characterized by narrow permitted emission lines (Goodrich, 1989), relatively weak [O III] (Osterbrock and Pogge, 1985) and strong Fe II (Osterbrock and Pogge, 1985). They host low or intermediate mass black holes (Peterson et al., 2000) accreting close to the Eddington limit (Boroson and Green, 1992). NLS1 sources are preferably, but not exclusively, hosted by disk-like galaxies. These remarkable properties indicate that they are evolutionarily young, or possibly rejuvenated, AGN (Mathur et al., 2001).
Only ~7% of these sources are radio-loud (RL) (Komossa et al., 2006). They usually show only a compact radio core, but recent studies suggest that pc- and kpc-scale structures are not that rare either (e.g., Gliozzi et al., 2010; Doi et al., 2013; Richards and Lister, 2015; Lister et al., 2016). Moreover, Fermi detected gamma-ray emission in some NLS1 galaxies (e.g., Abdo et al., 2009), confirming the presence of powerful relativistic jets.
NLS1 population is seemingly heterogeneous including radio- and gamma-loud sources as well as totally radio-silent (RS) sources. This divergence complicates the studies of the whole NLS1 population. An alternative take on this issue is to study the environments of NLS1 galaxies. The environment affects the evolution of galaxies, and consequently their nuclear activity. The environment can be divided into several scales: (1) the host galaxy; (2) the local environment, including the closest galaxies and the group or cluster the galaxy belongs to; and (3) the large-scale environment, tracing the cosmic web of voids, filaments and superclusters.
Here we present the main results of a study of the large-scale environments of NLS1 galaxies. The large-scale environment position of the galaxy affects its properties; the galaxies residing in the densest environments are preferably ellipticals, and the fraction of late-type galaxies increases as the density decreases (Lietzen et al., 2012; Einasto et al., 2014; Chen et al., 2017; Kuutma et al., 2017; Pandey and Sarkar, 2017). Using a diverse and large, statistically significant sample we examine the large-scale environments of NLS1 galaxies. We compare the environment properties of NLS1 galaxies and other types of AGN, as well as study the trends within the NLS1 population. In addition, we investigate whether the large-scale environment is connected to the intrinsic properties of NLS1 galaxies. We assume a cosmology with H0 = 73 km s−1 Mpc−1, Ωmatter = 0.27 and Ωvacuum = 0.73 (Spergel et al., 2007).
2. Sample and Data
Our sample includes 960 sources from Zhou et al. (2006), Komossa et al. (2006), Whalen et al. (2006), Yuan et al. (2008), and Foschini (2011) at a redshift range of z = 0.0726−0.3996, and with a mean redshift of z = 0.2340. The radio-loudness (R, the ratio between the radio flux density, FR, and the optical flux density, FO) was computed for sources with radio (1.4 GHz) and optical (468.6 nm) data, obtained from ASI Science Data Center (ASDC1). The archival data are non-simultaneous.
K-correction was not applied since the variability of NLS1 sources in radio and optical bands affects R significantly more than the K-correction. Even though R is not an ideal way to estimate the radio characteristics of AGN, it is commonly used and enables us to compare our results with earlier studies. Accordingly, 74 of our sources are RL (10 < R < 100), 13 are very radio-loud (VRL, R > 100) and 73 RQ (R < 10). 161 sources are radio-detected (RD), leaving 799 seemingly RS sources. Some RS NLS1 galaxies have been detected at Metsähovi Radio Observatory at 37 GHz (Lähteenmäki et al. in preparation.), indicating that a fraction of them is misclassified. The arbitrary boundary between RL and RQ sources, their variability, and misclassifications might affect the data analysis. Additionally we use a subsample of 15 jetted NLS1 galaxies in which the radio emission is known to be dominated by a relativistic jet (see section 5.3).
We estimated black hole masses (MBH) using the FWHM(Hβ)—luminosity mass scaling relation (Greene and Ho, 2005)
The λL5100 and FWHM(Hβ) values were from Zhou et al. (2006). We were able to estimate MBH for 937 sources. This method does not take into account possible inclination effects caused by the geometry of the BLR (Decarli et al., 2011), and for jetted sources it overestimates the MBH (Wu et al., 2004). Regardless, this method can be used as an order of magnitude estimate in statistical studies.
3. Large-scale Environment
3.1. SDSS LRG LDF
SDSS LRG LDF is a three-dimensional low-resolution luminosity-density field (LDF) limited to 225–1,000 h−1 Mpc and constructed using a sample of luminous red galaxies (LRG) in SDSS DR7 (Abazajian et al., 2009). The mean luminosity-density around each source is calculated in a volume of 3 h−1 Mpc, using a 16 h−1 Mpc smoothing. Detailed description is available in Lietzen et al. (2011) and Liivamägi et al. (2012).
We study the large-scale environments of the subsamples and the total NLS1 sample and compare them to AGN samples studied in Lietzen et al. (2011) using the same LDF. Table 1 lists average luminosity-densities and the distributions into different luminosity-density regions. For the average luminosity-densities the error is the standard error of the mean and for the percentages it is the Poissonian error. Seyfert 1 (Sy1) galaxies from Lietzen et al. (2011) are also listed. They used a cut of [O III]/Hβ> 3 when selecting their Sy1 sample thus it is a pure BLS1 sample. The regions are defined as in Lietzen et al. (2011); in a void the luminosity-density is <1.0, in an intermediate luminosity-density region it is between 1.0 and 3.0, and in a supercluster >3.0. The intermediate luminosity-density regions might correspond to either physical filaments or other areas of intermediate density, e.g., the outer parts of superclusters. It should be kept in mind that whereas superclusters are the densest structures at these scales this study does not probe smaller-scale galaxy clusters which are the densest regions in the Universe.
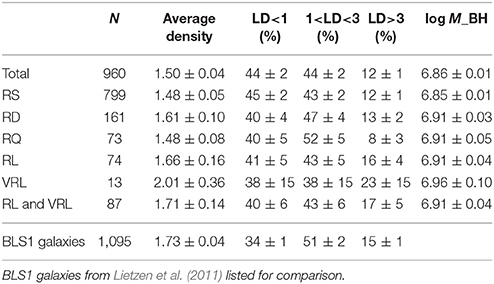
Table 1. Average density of the total SDSS LRG sample and the NLS1 subsamples, percentage in voids, intermediate density regions, and superclusters, and average black hole masses of different NLS1 subsamples.
The large-scale environment properties of NLS1 galaxies are distinct when compared to samples in Lietzen et al. (2011); they have smaller average luminosity-density than any comparison sample, and a different spatial distribution. Of the samples in Lietzen et al. (2011) NLS1 galaxies most resemble BLS1 and Sy2 galaxies; this was expected since Seyfert galaxies in general are young, late-type galaxies, whereas other AGN classes in Lietzen et al. (2011) are evolved, older galaxies, for example, BL Lac objects and Fanaroff-Riley I and II galaxies. Nevertheless, the difference is significant and supported by the divergence in their spatial distributions; compared to BLS1 and Sy2 galaxies a larger fraction of NLS1 galaxies reside in voids and a smaller fraction in intermediate-density regions. In superclusters the percentage is almost the same. Welch's t-test for the average luminosity-densities of the NLS1 and BLS1 samples gives a t-value of 3.96 which corresponds to the p-value of 0.0001, so it is highly unlikely that the averages are different by chance.
Among the NLS1 subsamples the average luminosity-density seems to increase with increasing R. Statistically the differences in the averages are not so significant and suffer from the small sample sizes; for RS and RQ, RS and RL, and RQ and RL samples the p-values according to Welch's t-test are 0.98, 0.11, and 0.23, respectively. Interestingly, the average luminosity-density of RL subsample is similar to the average luminosity-density of BLS1 sources in Lietzen et al. (2011). Increasing R also seems to induce changes in the spatial distribution, but confirming this would require a larger sample.
4. Principal Component Analysis
Principal component analysis (PCA, e.g., Abdi and Williams, 2010) is a useful tool in AGN studies. It can be utilized to find underlying connections and the most dominant variables in a data set, and helps to identify the physical properties connected with the eigenvectors (EV). Previous PCA studies of AGN (Boroson and Green, 1992; Boroson, 2002; Grupe, 2004; Xu et al., 2012; Järvelä et al., 2015) have led to the discovery of the 4DE1 parameter space, defined by FWHM(Hβ), F([O III]) λ5007, R4570, and C IV λ1549, explaining a host of differences observed in AGN, and possibly tracing the general AGN evolution (Marziani et al., 2006; Sulentic et al., 2007).
We performed weighted PCA using the pca2 function in MATLAB Statistics and Machine Learning Toolbox. Including a large-scale environment parameter to the PCA allows us to investigate its connection to the AGN correlation space. In PCA we used FO, FWHM(Hβ), R4570, F([O III]), and the luminosity-density. FWHM(Hβ), R4570, and F([O III]) were from Zhou et al. (2006). The results are presented in Table 2. The coefficients have been grouped together based on their sign, i.e., whether they correlate or anticorrelate with the EV and each other. The sign of the coefficient is insignificant as such and only their respective direction matters.
4.1. Eigenvectors and Their Correlations
4.1.1. EV1
EV1 accounts for 30% of the variance. It is dominated by FO and F([O III]), which are anticorrelated with R4570. It is not exactly similar to the traditional EV1 driven by the anticorrelation of FWHM(Hβ) and F([O III]), and R4570. EV1 correlates with the Eddington ratio3 to some extent (Spearman rank correlation coefficient ρ = −0.506, and probability value p = 0.000) and weakly with the MBH (ρ = 0.291, p = 0.000).
4.1.2. EV2
EV2 25% of the variance is explained by EV2. It is dominated by the anticorrelation of R4570 and FO, and FWHM(Hβ), and is similar to EV1 found in Boroson and Green (1992), Boroson (2002), and Xu et al. (2012). It indeed correlates strongly with the Eddington ratio (ρ = -0.764, p = 0.000), and significantly also with MBH (ρ = 0.394, p = 0.000).
4.1.3. EV3
EV3 explains 20% of the variance. Density has the strongest impact on EV3 and all the other parameters are negligible. EV3 does not correlate strongly with the Eddington ratio (ρ = -0.157, p = 0.000) or MBH (ρ = 0.100, p = 0.002). These results indicate that the large-scale environment density does not directly affect any singular properties, but rather the overall evolution of the galaxies.
5. Discussion
5.1. NLS1s Compared to Other AGN
Earlier studies have found NLS1 galaxies to be morphologically young, late-type sources. In the framework of the density—morphology relation (Hubble and Humason, 1931; Dressler, 1980)—more evolved galaxies are found in denser regions—our results support this and confirm the young nature of the NLS1 population.
There is a clear difference in the average luminosity-densities of the NLS1 and BLS1 sources, contradicting the simple orientation-based unification model in which they can be unified when the geometrical effects are taken into account (Decarli et al., 2008; Rakshit et al., 2017). However, the diversity of BLS1 galaxies was not taken into account in Lietzen et al. (2011) and might affect the results; for example, BLS1 galaxies with pseudobulges might be alike to NLS1 galaxies and possibly part of their parent population. Alternatively, NLS1 and BLS1 galaxies might be unified through evolution; if the narrower broad lines of NLS1 galaxies compared to BLS1 galaxies are due to an undermassive black hole, growing NLS1 galaxies would eventually evolve to have higher mass black holes and broader broad lines, thus, BLS1 galaxies.
Recently, a few higher-inclination NLS1 sources have been found (Doi et al., 2015; Congiu et al., 2017), proving that the narrow lines in these sources are due to the undermassive black hole and indicating that no additional broad-line parent population is necessarily needed. However, the parent population studies are in the early stage and include a variety of scenarios, for example, compact steep-spectrum sources and disk-hosted radio galaxies have been proposed as possible parent populations of jetted NLS1 sources (Berton et al., 2015, 2016, 2017).
PCA supports the idea that NLS1 sources are a part of the AGN continuum; NLS1 EVs 1 and 2 are a manifestation of the 4DE1 AGN correlation space. Shen and Ho (2014) propose that the variations in FWHM(Hβ), R4570, and [O III], and their correlations can be explained with the Eddington ratio and the orientation; increasing Eddington ratio leads to increasing R4570 and decreasing [O III], and the scatter in FWHM(Hβ) is mainly a geometrical effect. NLS1 galaxies follow the same trend as broad-line AGN and complete the high R4570, low [O III] extreme of the continuum. We can not confirm or disprove the role of the orientation, but if the FWHM(Hβ) was orientation-dependent BLS1 sources should form the parent population of NLS1 galaxies, which, based on our large-scale environment results, seems improbable.
5.2. NLS1s as a Class
The incidence of RL sources increases toward the denser regions, indicating that the large-scale environment holds the capacity to alter the radio characteristics of NLS1 sources. The average MBH of the subsamples at fixed redshift are similar, as can be seen in Table 1, and R does not correlate with MBH, suggesting that MBH and the radio properties do not have a strong connection. The average MBH within the different luminosity-density regions are similar. For example, RL sources have similar average MBH in voids, intermediate-density regions, and superclusters, as well as do RQ and RS sources. This might indicate that, at least up to this point, the accretion history of these sources has been similar in all density regions.
There is some indication that the spatial distributions of subsamples change with the changing R, which would be a natural consequence of the changing average luminosity-density, but a larger, cafefully classified, sample will be needed to verify this result. Heterogeneous samples are one of the caveats in extensive AGN population studies, and a possible reason why large statistical studies might give complex, or even inconsistent, results. Our study is no exception; some faint radio sources might be incorrectly in the RS subsample, R might be too high for sources with enhanced star formation, and the variability of NLS1 sources causes R to be time-dependent. Indeed, according to the two-sample Kolmogorov-Smirnov test our subsamples are drawn from the same luminosity-density distribution, further indicating that they are, in some degree, mixed.
5.3. Special Case of Jetted NLS1s
Padovani (2016) suggests that the main difference between RQ and RL sources is the presence of a powerful relativistic jet, and that definitions RQ and RL should be replaced with non-jetted and jetted. While this is true for most sources the situation is more complicated, for example, we know that radio-loudness can be induced by enhanced star formation (Caccianiga et al., 2015) and some RS sources do host relativistic jets (Lähteenmäki et al. in preparation.). Instead of using radio-loudness as a proxy for the nuclear activity the real physical properties, specifically the presence of a jet, should be used, but this is often impossible due to the lack of data.
We can begin to address this issue by concentrating on the sources known to have jets. We implement this by selecting a sample of NLS1 galaxies whose radio emission is dominated by the AGN; gamma-detected NLS1 galaxies, NLS1 sources with extended jets, sources detected at Metsähovi (Lähteenmäki et al. (2017) and Lähteenmäki et al. in preparation), and sources in which the radio emission is jet dominated based on the q22 parameter [log(F22μm/F1.4GHz), Caccianiga et al., 2015].
After excluding two sources from the Metsähovi sample, initially selected for the observing programme due to their extraordinarily dense environments, we have 15 almost certainly jetted sources. For these sources the average luminosity-density is 1.75 ± 0.31 (N = 15). The average luminosity-densities for the mixed and star formation (SF) dominated subsamples (based on q22) are 1.68 ± 0.15 (N = 82) and 1.44 ± 0.14 (N = 57), respectively.
Despite the rather small subsamples it seems clear that the jetted NLS1 galaxies tend to reside in denser environments. Interestingly, the average luminosity-density of these is almost similar to the traditionally defined RL NLS1 sources (1.71 ± 0.14) and the average luminosity-density of the SF dominated sources is similar to the RS sources.
These results are consistent with the LRG LDF results; the increasing R increases the probability that the radio emission originates from the jet, and in SF dominated sources the contribution of the probably faint AGN to the radio emission is negligible. This does not exclude the possibility that jetted sources could have enhanced SF.
It is noteworthy that the average large-scale environment density of jetted NLS1 galaxies is similar to that of BLS1 galaxies (Lietzen et al., 2011). Jetted AGN samples in Lietzen et al. (2011) include BL Lac objects (2.50 ± 0.20), flat-spectrum radio galaxies (2.60 ± 0.07), FR I radio galaxies (3.01 ± 0.07), and FR II radio galaxies (3.20 ± 0.04), all with significantly higher average luminosity-densities than the jetted NLS1 galaxies (1.75 ± 0.31). This pronounced difference confirms that jets are not exclusive to evolved galaxies in high density regions, but it is possible to trigger a jet in diverse environments. The large-scale environment affects the incidence of jets, but the triggering mechanism remains unclear.
6. Summary
We examined the large-scale environment of NLS1 galaxies and its effect on their intrinsic properties. The environment provides an interesting new perspective on the AGN phenomena and helps us to understand how changes at the largest cosmic scales affect the smaller-scale properties of AGN and their evolution. Our main conclusions are the following.
1. Our results further establish the young, unevolved nature of NLS1 galaxies, and show that their radio properties are affected by the environment; RL and jetted NLS1 galaxies are more frequent in denser regions compared to RS, RQ and non-jetted sources.
2. As a class NLS1 galaxies are diverse and heterogeneous, and a considerable amount of them are misclassified. Our study supports the proposition to start using definitions non-jetted and jetted instead of RQ and RL (Padovani, 2016), and we further suggest that this division should be based on observed physical properties instead of radio-loudness parameter.
3. Our study suggests that NLS1 and BLS1 galaxies can not be unified only based on orientation. It is improbable that BLS1 galaxies would constitute the parent population of NLS1 galaxies. However, an evolutionarily link might exist.
4. NLS1 sources are part of the AGN 4DE1 continuum, but clearly distinctive in their nature when compared to the other gamma-ray emitting AGN.
The large-scale environment has an impact on the properties and evolution of AGN, amongst them NLS1 galaxies. However, it likely affects at very long time-scales and thus its connection to specific characteristics of variable and intermittent nuclear activity is weak. To effectively exploit this novel perspective the NLS1 environment studies should be extended to concern smaller-scale environment; the group and cluster scales, and host galaxy properties, both of which are likely to induce changes in AGN properties at shorter time-scales. The local environment studies (e.g., Dultzin-Hacyan et al., 1999; Krongold et al., 2001; Koulouridis et al., 2006, 2013; Koulouridis, 2014; Villarroel and Korn, 2014) indicate that Sy1 and Sy2 galaxies differ in their smaller-scale environments and host galaxies, but comprehensive studies including NLS1 galaxies are still needed. Extensive studies at various scales will clarify how the environment affects the AGN evolution, and help us establish the big picture of AGN. In addition, multiwavelength observations will be crucial to correctly classify the various NLS1 sources. This is essential to properly perform population-wide studies and address the heterogeneity issue of the NLS1 class.
Author Contributions
All of the authors mentioned below fulfil the criteria for an authorship. EJ: data analysis and interpretation, main writer, final acceptance. AL: original research idea, writing and revision of the draft, final acceptance. HL: construction of the luminosity-density field, revision of the draft, final acceptance.
Funding
HL is funded by PUT1627 grant from Estonian Research Council.
Conflict of Interest Statement
The authors declare that the research was conducted in the absence of any commercial or financial relationships that could be construed as a potential conflict of interest.
Acknowledgments
This research has made use of the NASA/IPAC Extragalactic Database (NED) which is operated by the Jet Propulsion Laboratory, California Institute of Technology, under contract with the National Aeronautics and Space Administration.
The National Radio Astronomy Observatory is a facility of the National Science Foundation operated under cooperative agreement by Associated Universities, Inc.
Funding for the Sloan Digital Sky Survey (SDSS) has been provided by the Alfred P. Sloan Foundation, the Participating Institutions, the National Aeronautics and Space Administration, the National Science Foundation, the U.S. Department of Energy, the Japanese Monbukagakusho, and the Max Planck Society. The SDSS Web site is http://www.sdss.org/.
The SDSS is managed by the Astrophysical Research Consortium (ARC) for the Participating Institutions. The Participating Institutions are The University of Chicago, Fermilab, the Institute for Advanced Study, the Japan Participation Group, The Johns Hopkins University, the Korean Scientist Group, Los Alamos National Laboratory, the Max-Planck-Institute for Astronomy (MPIA), the Max-Planck-Institute for Astrophysics (MPA), New Mexico State University, University of Pittsburgh, University of Portsmouth, Princeton University, the United States Naval Observatory, and the University of Washington.
Footnotes
2. ^https://se.mathworks.com/help/stats/pca.html
3. ^Lbol / LEdd = 9λ L5100 / 1.3 × 1038 MBH / M⊙
References
Abazajian, K. N., Adelman-McCarthy, J. K., Agüeros, M. A., Allam, S. S., Allende Prieto, C., An, D., et al. (2009). The seventh data release of the sloan digital sky survey. Astrophys. J. Suppl. 182, 543–558. doi: 10.1088/0067-0049/182/2/543
Abdi, H., and Williams, L. J. (2010). Principal component analysis. Wiley Interdiscip. Rev. 2, 433–459. doi: 10.1002/wics.101
Abdo, A. A., Ackermann, M., Ajello, M., Axelsson, M., Baldini, L., Ballet, J., et al. (2009). Fermi/large area telescope discovery of gamma-ray emission from a relativistic jet in the narrow-line quasar PMN J0948+0022. Astrophys. J. 699, 976–984. doi: 10.1088/0004-637X/699/2/976
Berton, M., Caccianiga, A., Foschini, L., Peterson, B. M., Mathur, S., Terreran, G., et al. (2016). Compact steep-spectrum sources as the parent population of flat-spectrum radio-loud narrow-line Seyfert 1 galaxies. Astron. Astrophys. 591:A98. doi: 10.1051/0004-6361/201628171
Berton, M., Foschini, L., Caccianiga, A., Ciroi, S., Congiu, E., Cracco, V., et al. (2017). An orientation-based unification of young jetted active galactic nuclei. ArXiv:1705.07905.
Berton, M., Foschini, L., Ciroi, S., Cracco, V., La Mura, G., Lister, M. L., et al. (2015). Parent population of flat-spectrum radio-loud narrow-line Seyfert 1 galaxies. Astron. Astrophys. 578:A28. doi: 10.1051/0004-6361/201525691
Boroson, T. A. (2002). Black hole mass and eddington ratio as drivers for the observable properties of radio-loud and radio-quiet QSOs. Astrophys. J. 565, 78–85. doi: 10.1086/324486
Boroson, T. A., and Green, R. F. (1992). The emission-line properties of low-redshift quasi-stellar objects. Astrophys. J. Suppl. 80, 109–135. doi: 10.1086/191661
Caccianiga, A., Antón, S., Ballo, L., Foschini, L., Maccacaro, T., Della Ceca, R., et al. (2015). WISE colours and star formation in the host galaxies of radio-loud narrow-line Seyfert 1. Month. Notices R. Astron. Soc. 451, 1795–1805. doi: 10.1093/mnras/stv939
Chen, Y.-C., Ho, S., Mandelbaum, R., Bahcall, N. A., Brownstein, J. R., Freeman, P. E., et al. (2017). Detecting effects of filaments on galaxy properties in the Sloan Digital Sky Survey III. Month. Notices R. Astron. Soc. 466, 1880–1893. doi: 10.1093/mnras/stw3127
Congiu, E., Berton, M., Giroletti, M., Antonucci, R., Caccianiga, A., Kharb, P., et al. (2017). Kiloparsec-scale emission in the narrow-line Seyfert 1 galaxy Mrk 783. ArXiv:1704.03881.
Decarli, R., Dotti, M., Fontana, M., and Haardt, F. (2008). Are the black hole masses in narrow-line Seyfert 1 galaxies actually small? Month. Notices R. Astron. Soc. 386, L15–L19. doi: 10.1111/j.1745-3933.2008.00451.x
Decarli, R., Dotti, M., and Treves, A. (2011). Geometry and inclination of the broad-line region in blazars. Month. Notices R. Astron. Soc. 413, 39–46. doi: 10.1111/j.1365-2966.2010.18102.x
Doi, A., Asada, K., Fujisawa, K., Nagai, H., Hagiwara, Y., Wajima, K., et al. (2013). Very long baseline array imaging of parsec-scale radio emissions in nearby radio-quiet narrow-line seyfert 1 galaxies. Astrophys. J. 765:69. doi: 10.1088/0004-637X/765/1/69
Doi, A., Wajima, K., Hagiwara, Y., and Inoue, M. (2015). A fanaroff-riley type I candidate in narrow-line seyfert 1 galaxy Mrk 1239. Astrophys. J. Lett. 798:L30. doi: 10.1088/2041-8205/798/2/L30
Dressler, A. (1980). Galaxy morphology in rich clusters - implications for the formation and evolution of galaxies. Astrophys. J. 236, 351–365. doi: 10.1086/157753
Dultzin-Hacyan, D., Krongold, Y., Fuentes-Guridi, I., and Marziani, P. (1999). The close environment of seyfert galaxies and its implication for unification models. Astrophys. J. Lett. 513, L111–L114. doi: 10.1086/311925
Einasto, M., Lietzen, H., Tempel, E., Gramann, M., Liivamägi, L. J., and Einasto, J. (2014). SDSS superclusters: morphology and galaxy content. Astron. Astrophys. 562:A87. doi: 10.1051/0004-6361/201323111
Foschini, L. (2011). “Evidence of powerful relativistic jets in narrow-line Seyfert 1 galaxies,” in Narrow-Line Seyfert 1 Galaxies and their Place in the Universe (Milano).
Gliozzi, M., Papadakis, I. E., Grupe, D., Brinkmann, W. P., Raeth, C., and Kedziora-Chudczer, L. (2010). A panchromatic view of PKS 0558-504: an ideal laboratory to study the disk-jet link. Astrophys. J. 717, 1243–1252. doi: 10.1088/0004-637X/717/2/1243
Goodrich, R. W. (1989). Spectropolarimetry of ‘narrow-line’ Seyfert 1 galaxies. Astrophys. J. 342, 224–234. doi: 10.1086/167586
Greene, J. E., and Ho, L. C. (2005). Estimating black hole masses in active galaxies using the Hα emission line. Astrophys. J. 630, 122–129. doi: 10.1086/431897
Grupe, D. (2004). A complete sample of soft X-ray-selected AGNs. II. Statistical analysis. Astron. J. 127, 1799–1810. doi: 10.1086/382516
Hubble, E., and Humason, M. L. (1931). The velocity-distance relation among extra-galactic nebulae. Astrophys. J. 74:43. doi: 10.1086/143323
Järvelä, E., Lähteenmäki, A., and León-Tavares, J. (2015). Statistical multifrequency study of narrow-line Seyfert 1 galaxies. Astron. Astrophys. 573:A76.
Komossa, S., Voges, W., Xu, D., Mathur, S., Adorf, H.-M., Lemson, G., et al. (2006). Radio-loud narrow-line type 1 quasars. Astron. J. 132, 531–545. doi: 10.1086/505043
Koulouridis, E. (2014). The dichotomy of Seyfert 2 galaxies: intrinsic differences and evolution. Astron. Astrophys. 570:A72. doi: 10.1051/0004-6361/201424622
Koulouridis, E., Plionis, M., Chavushyan, V., Dultzin, D., Krongold, Y., Georgantopoulos, I., et al. (2013). Activity of the Seyfert galaxy neighbours. Astron. Astrophys. 552:A135. doi: 10.1051/0004-6361/201219606
Koulouridis, E., Plionis, M., Chavushyan, V., Dultzin-Hacyan, D., Krongold, Y., and Goudis, C. (2006). Local and large-scale environment of seyfert galaxies. Astrophys. J. 639, 37–45. doi: 10.1086/498421
Krongold, Y., Dultzin-Hacyan, D., and Marziani, P. (2001). Host galaxies and circumgalactic environment of “narrow line” seyfert 1 nuclei. Astron. J. 121, 702–709. doi: 10.1086/318768
Kuutma, T., Tamm, A., and Tempel, E. (2017). From voids to filaments: environmental transformations of galaxies in the SDSS. Astron. Astrophys. 600:L6. doi: 10.1051/0004-6361/201730526
Lähteenmäki, A., Järvelä, E., Hovatta, T., Tornikoski, M., Harrison, D. L., López-Caniego, M., et al. (2017). 37 GHz observations of narrow-line Seyfert 1 galaxies. ArXiv: 1703.10365.
Lietzen, H., Heinämäki, P., Nurmi, P., Liivamägi, L. J., Saar, E., Tago, E., et al. (2011). Large-scale environments of z < 0.4 active galaxies. Astron. Astrophys. 535:A21. doi: 10.1051/0004-6361/201116550
Lietzen, H., Tempel, E., Heinämäki, P., Nurmi, P., Einasto, M., and Saar, E. (2012). Environments of galaxies in groups within the supercluster-void network. Astron. Astrophys. 545:A104. doi: 10.1051/0004-6361/201219353
Liivamägi, L. J., Tempel, E., and Saar, E. (2012). SDSS DR7 superclusters. The catalogues. Astron. Astrophys. 539:A80. doi: 10.1051/0004-6361/201016288
Lister, M. L., Aller, M. F., Aller, H. D., Homan, D. C., Kellermann, K. I., Kovalev, Y. Y., et al. (2016). MOJAVE: XIII. Parsec-scale AGN jet kinematics analysis based on 19 years of VLBA observations at 15 GHz. Astron. J. 152:12. doi: 10.3847/0004-6256/152/1/12
Marziani, P., Dultzin-Hacyan, D., and Sulentic, J. W. (2006). Accretion onto Supermassive Black Holes in Quasars: Learning from Optical/UV Observations. New York, NY: Nova Science Publishers. 123.
Mathur, S., Kuraszkiewicz, J., and Czerny, B. (2001). Evolution of active galaxies: black-hole mass-bulge relations for narrow line objects. NewA 6, 321–329. doi: 10.1016/S1384-1076(01)00058-6
Osterbrock, D. E., and Pogge, R. W. (1985). The spectra of narrow-line Seyfert 1 galaxies. Astrophys. J. 297, 166–176. doi: 10.1086/163513
Padovani, P. (2016). The faint radio sky: radio astronomy becomes mainstream. Astron. Astrophys. Rev. 24:13. doi: 10.1007/s00159-016-0098-6
Pandey, B., and Sarkar, S. (2017). How much a galaxy knows about its large-scale environment?: an information theoretic perspective. Month. Notices R. Astron. Soc. 467, L6–L10. doi: 10.1093/mnrasl/slw250
Peterson, B. M., McHardy, I. M., Wilkes, B. J., Berlind, P., Bertram, R., Calkins, M., et al. (2000). X-ray and optical variability in NGC 4051 and the nature of narrow-line seyfert 1 galaxies. Astrophys. J. 542, 161–174. doi: 10.1086/309518
Rakshit, S., Stalin, C. S., Chand, H., and Zhang, X.-G. (2017). A catalog of narrow line seyfert 1 galaxies from the sloan digital sky survey data release 12. Astrophys. J. Suppl. 229:39. doi: 10.3847/1538-4365/aa6971
Richards, J. L., and Lister, M. L. (2015). Kiloparsec-scale jets in three radio-loud narrow-line seyfert 1 galaxies. Astrophys. J. Lett. 800:L8. doi: 10.1088/2041-8205/800/1/L8
Shen, Y., and Ho, L. C. (2014). The diversity of quasars unified by accretion and orientation. Nature 513, 210–213. doi: 10.1038/nature13712
Spergel, D. N., Bean, R., Doré, O., Nolta, M. R., Bennett, C. L., Dunkley, J., et al. (2007). Three-year Wilkinson microwave anisotropy probe (WMAP) observations: implications for cosmology. Astrophys. J. Suppl. 170, 377–408. doi: 10.1086/513700
Sulentic, J. W., Bachev, R., Marziani, P., Negrete, C. A., and Dultzin, D. (2007). C IV λ1549 as an eigenvector 1 parameter for active galactic nuclei. Astrophys. J. 666, 757–777. doi: 10.1086/519916
Villarroel, B., and Korn, A. J. (2014). The different neighbours around Type-1 and Type-2 active galactic nuclei. Nat. Phys. 10, 417–420. doi: 10.1038/nphys2951
Whalen, D. J., Laurent-Muehleisen, S. A., Moran, E. C., and Becker, R. H. (2006). Optical properties of radio-selected narrow-line seyfert 1 galaxies. Astron. J. 131, 1948–1960. doi: 10.1086/500825
Wu, X.-B., Wang, R., Kong, M. Z., Liu, F. K., and Han, J. L. (2004). Black hole mass estimation using a relation between the BLR size and emission line luminosity of AGN. Astron. Astrophys. 424, 793–798. doi: 10.1051/0004-6361:20035845
Xu, D., Komossa, S., Zhou, H., Lu, H., Li, C., Grupe, D., et al. (2012). Correlation analysis of a large sample of narrow-line seyfert 1 galaxies: linking central engine and host properties. Astron. J. 143:83. doi: 10.1088/0004-6256/143/4/83
Yuan, W., Zhou, H. Y., Komossa, S., Dong, X. B., Wang, T. G., Lu, H. L., et al. (2008). A population of radio-loud narrow-line seyfert 1 galaxies with blazar-like properties? Astrophys. J. 685, 801–827. doi: 10.1086/591046
Keywords: active galactic nuclei, narrow-line Seyfert 1 galaxies, large-scale environment, radio loudness, relativistic jets
Citation: Järvelä E, Lähteenmäki A and Lietzen H (2017) Large-Scale Environment Properties of Narrow-Line Seyfert 1 Galaxies at z < 0.4. Front. Astron. Space Sci. 4:54. doi: 10.3389/fspas.2017.00054
Received: 25 August 2017; Accepted: 16 November 2017;
Published: 30 November 2017.
Edited by:
Ascensión Del Olmo, Instituto de Astrofísica de Andalucía (CSIC), SpainReviewed by:
Daniela Bettoni, Osservatorio Astronomico di Padova (INAF), ItalyElias Koulourids, CEA Saclay, France
Copyright © 2017 Järvelä, Lähteenmäki and Lietzen. This is an open-access article distributed under the terms of the Creative Commons Attribution License (CC BY). The use, distribution or reproduction in other forums is permitted, provided the original author(s) or licensor are credited and that the original publication in this journal is cited, in accordance with accepted academic practice. No use, distribution or reproduction is permitted which does not comply with these terms.
*Correspondence: Emilia Järvelä, emilia.jarvela@aalto.fi