- 1Instituto de Astrofísica de Canarias, Santa Cruz de Tenerife, Spain
- 2Departamento de Astrofísica, Universidad de La Laguna, Santa Cruz de Tenerife, Spain
- 3Department of Physics, University of Oxford, Oxford, United Kingdom
- 4Centro de Astrobiología (CSIC-INTA), Madrid, Spain
We present preliminary results on the potential of HARMONI, the first light integral field spectrograph for the ELT, to explore the evolution of central super massive black holes (SMBH)—host galaxy relation in the range from z~0.7 to z~2.5. We simulated HARMONI observations of QSO+host galaxy at different redshifts, assuming different morphologies for the host galaxy. As input, we combined MUSE observations of nearby galaxies and a theoretical QSO spectrum. These were dimmed and redshifted to the desired cosmic epoch. We scaled the total host galaxy luminosity to three different values, sampling three orders of magnitude. Likewise, we assumed two different luminosities for the central QSO. Simulations were performed for the 30×60 mas2 HARMONI spatial scale and LTAO working at 0.67 arcsec seeing. The selected wavelength range (i.e., 4,700–5,300 Å at rest-frame) was sampled at the lowest HARMONI spectral resolving power (i.e., R~3,200). This configuration included all the ingredients to estimate the host galaxy parameters and the SMBH mass, as well as for assessing the morphological type of the host galaxy.
1. Introduction
In the hierarchical buildup picture, larger galaxies are formed as the result of the merging of smaller-size galaxies across cosmic time. Besides, observational studies have established that the large-scale properties of nearby galaxies (e.g., bulge luminosity, bulge mass, or stellar velocity dispersion) generally scale with the mass of their central black holes (see e.g., Kormendy and Ho, 2013). However, there are exceptions to this picture: some pseudo-bulge or merging galaxies present some deviations from these correlations (see e.g., Saglia et al., 2016). Putting aside these exceptions, the fact that those more general relations do actually exist suggests a connection between the growth of SMBH and the way its host galaxy evolves: a co-growth scenario for galaxies and their central black holes. How this connection is established, and the precise details of the interplay between the SMBH and its host during their evolution are, however, not fully understood.
An important piece of information comes from the evolution of such relations over cosmic time. Knowing this would provide key constrains to discern between different (co-)evolution scenarios. This implies measuring both the SMBH masses and the properties of the host galaxy (i.e., luminosity, size, morphology, stellar velocity dispersion…) of a suitable sample of objects at different redshifts. Ideally, for the shake of homogeneity and time efficiency (among others), one would like to observe simultaneously all the needed observational features to derive the involved parameters. This can actually be done with spectroscopic information at optical wavelengths (rest-frame). SMBH masses can be estimated using the so-called single epoch virial method on type I AGN spectra (e.g., Kaspi et al., 2000; McLure and Jarvis, 2002) and, from the width of the broad component of emission lines and the continuum or emission line luminosities of the QSO through simple relations (e.g., Vestergaard and Peterson, 2006). Likewise, continuum can be used, if detected, to derive the properties of the host galaxy. From the technical point view, two competing requirements are at play here. On the one hand, proper virial broadening measurements require high signal-to-noise AGN spectra to control uncertainties. Hence, the most luminous QSOs are the ideal candidates to determine SMBH masses over cosmic time. On the other hand, bright QSO strongly hide the underlying host galaxy challenging the estimation of its morphological and kinematic parameters. The proper trade-off between the needs to estimate SMBH masses and host galaxies properties motivated the selection of the scaled luminosities for the QSOs and host galaxy (see below). Besides, the cosmic star formation history and the AGN population peak somewhere between redshift 1.5 and 2 (see e.g., Aird et al., 2015). Thus, our simulation should preferentially include a proper range of epochs sampling these highest activity regions in the Universe's timeline. At these redshifts, both the brightest QSO Balmer emission lines (Hβ and Hα) useful to estimate the SMBH masses, and several stellar absorption features (e.g., CaII triplet, Mgb triplet, and Ca H+K) are redshifted to infrared wavelengths. Notice that the prominent ultra-violet CIV emission line that is at visible wavelengths beyond redshift ~1.5 presents a complex profile, often blue-shifted; consequently, its use to estimate virial masses is doubtful (see e.g., Mej́ıa-Restrepo et al., 2016).
The evolution of the scaling relations over cosmic time requires exceptional observing capabilities in terms of sensitivity (to reach good enough S/N to derive the properties of the underlying host galaxy) and good spatial resolution (to resolve the QSO host at redshifts around epochs of activity peaks). Moreover, a proper QSO-host deblending needs of a good reconstruction of the PSF through the observed wavelengths. This is a key technical challenge. Current near infrared observational facilities (e.g., OSIRIS+LGS-AO/Keck, NIFS+LGS-AO/GEMINI) are limited in sensitivity and spatial resolution (see e.g., Vayner et al., 2016, 2017). Moreover, QSO-host deblending is quite difficult and dominated by residuals because the PSF halo is comparable to the host galaxy angular size (i.e., ~1 arcsec) at z ~ 1. In this contribution, we explore the potential of the High Angular Resolution Monolithic Optical and Near-infrared Integral field spectrograph (HARMONI), the first light IFS instrument for the Extremely Large Telescope (ELT), to unveil the co-growth of SMBH and their host galaxies.
2. HARMONI Simulations
HARMONI is conceived as a workhorse instrument that will support a broad range of science programs (see e.g., Thatte et al., 2016). It will provide visible and near infrared integral field spectroscopy at three possible resolving powers (~3,200, ~7,000, and ~18,000), collecting at once spectra of about 31,000 spatial positions, arranged in a 152×204 spaxels format. Four different spatial scales (i.e., 4×4, 10×10, 20×20, and 30×60 mas2) will be available. A full description of the instrument can be found at the HARMONI webpage1.
We are exploring the capabilities and limitations of HARMONI to measure the parameters involved in the relations between SMBH masses and their host galaxies through simulations. We selected the rest-frame range ~4,700–5,300 Å, including the Hβ emission line and some stellar features (e.g., MgI absorption lines). At redshifts between 0.7 and 2.5, all these spectral features lie in the near-IR range, well within the instrument specifications. Thus, by using these spectral features as observed with HARMONI, an homogenized methodology can be used to derive the required parameters (i.e., the BLR emission line profile and the host stellar velocity dispersion).
In order to obtain mock HARMONI observations, we used MUSE datacubes of nearby galaxies obtained in the framework of the All-weather MUse Supernova Integral field Nearby Galaxies (AMUSING) survey (see Galbany et al., 2016). In order to simulate two different morphologies for our QSO hosts at high redshifts, we selected two galaxies from the AMUSING project with apparent different morphologies:
• PGC 055442, an early-type barred spiral galaxy at redshift ~0.02358.
• NGC 7119N, a southern spiral galaxy at redshift ~0.03224. It has a companion (NGC 7119B) located within a projected distance of ~8.4 kpc and systemic velocity difference of ~76 km s−1.
We scaled the MUSE datacubes of these galaxies to sample three absolute V band luminosities (i.e., 1010L⊙, 1011L⊙, and 1012L⊙). In the Local Universe, the QSO are typically hosted in massive early-type galaxies (i.e., Mstar > 1010 M⊙) (e.g., Kauffmann et al., 2003). Also, luminous AGNs are typically triggered by interaction/mergers (see e.g., Ramos Almeida et al., 2011; Chiaberge et al., 2015). It is still unclear whether a similar picture applies at cosmological distances (see e.g., Floyd et al., 2013; Glikman et al., 2015). Yet, whatever the actual morphology of the QSO host galaxies in the z~0.7–2.5 cosmic range is, these HARMONI simulations also allow to evaluate up to which extent the morphology of the host galaxy can be recovered.
To mimic the emission of the central QSO, we generated a theoretical QSO spectrum by adding a power law function to reproduce the continuum emission (Neugebauer et al., 1987) and several Gaussian functions to reproduce the brightest emission lines in the 4,500–7,000 Å spectral range (i.e., Hβ, [OIII]λ4959, [OIII]λ5007, [NII]λ6548, Hα, [NII]λ6584, [SII]λ6716, and [SII]λ6731). The narrow component was modeled for both forbidden and permitted lines. We assumed a fixed width of 3 Å, corresponding to a velocity dispersion of ~185 km s−1 at Hβ rest-frame wavelength. The broad component was modeled in a similar manner, this time only for the permitted lines (i.e., Hβ and Hα) and assuming a fixed width of 60 Å (velocity dispersion of ~3,700 km s−1 at Hβ rest-frame wavelength). The QSO luminosity (at this stage in arbitrary units) was scaled to two different [OIII]λ5007 luminosities [i.e., log(L[OIII]λ5007 (erg s−1)) = 42 and 43], assuming an equivalent width of 10 Å for Lyα and standard emission line ratios (e.g., Davidson and Netzer, 1979) to match the continuum luminosity.
The combination of these ingredients was a set of 12 datacubes (i.e., 2 galaxy morphologies × 3 host luminosities × 2 QSO luminosities). We redshifted and dimmed them to three selected redshifts (i.e., 0.76, 1.50, and 2.30), using the cosmological parameters H0=67.9 km s−1 Mpc−1, ΩM=0.31 and ΩΛ=0.69 (Planck Collaboration et al., 2016). The resulting 36 datacubes constituted our targets and were used as inputs for HSIM22. This is the HARMONI simulator, and as such, it reproduces by software a real HARMONI observation by incorporating the effects of sky, telescope and instrument (see Zieleniewski et al., 2015 for details). Additionally, we also created the HARMONI datacubes for the redshifted galaxies and QSO separately.
The simulations presented in this contribution were obtained for the coarsest HARMONI spatial scale (i.e., 30×60 mas2), Laser Tomography Adaptive Optics (LTAO) working with standard seeing conditions (i.e., 0.67 arcsec). In all cases, we simulated observations at the lowest spectral resolution. This implied using the IzJ-band for redshifts 0.76 and 1.50 and the HK band for redshift 2.30. This way, we could observe simultaneously the needed emission (i.e., Hβ and [OIII]λ, λ4959, 5007) and absorption lines (e.g., MgI). We fixed the total exposure time to three hours per object since we wanted to explore up to which extent the required observables could be derived with a relatively modest amount of observing time. Finally, we assumed Poisson noise from sky and background emission as the only additional source of noise and a perfect sky subtraction.
3. Results and Conclusions
Figure 1 shows the mock HARMONI datacubes (i.e., two spatial and one spectral dimensions) for the simulated QSO+host observations collapsed along the spectral direction. Since we also simulated HARMONI datacubes of host-less QSOs (i.e., free of any host galaxy contribution), we could easily subtract out the QSO spectrum from our QSO+host simulations to reveal the underlying galaxy. This way, we do not address at this stage the complex task of recovering the PSF from observed QSO+host. A proper methodology minimizing uncertainties to recover the PSF are mandatory in these kind of studies as any residuals from PSF subtraction can drastically affect the host galaxy information (see e.g., Husemann et al., 2016). Thus, this step cannot be avoided in real observations. Yet, this is a challenging issue and it will be explored in a forthcoming independent experiment.
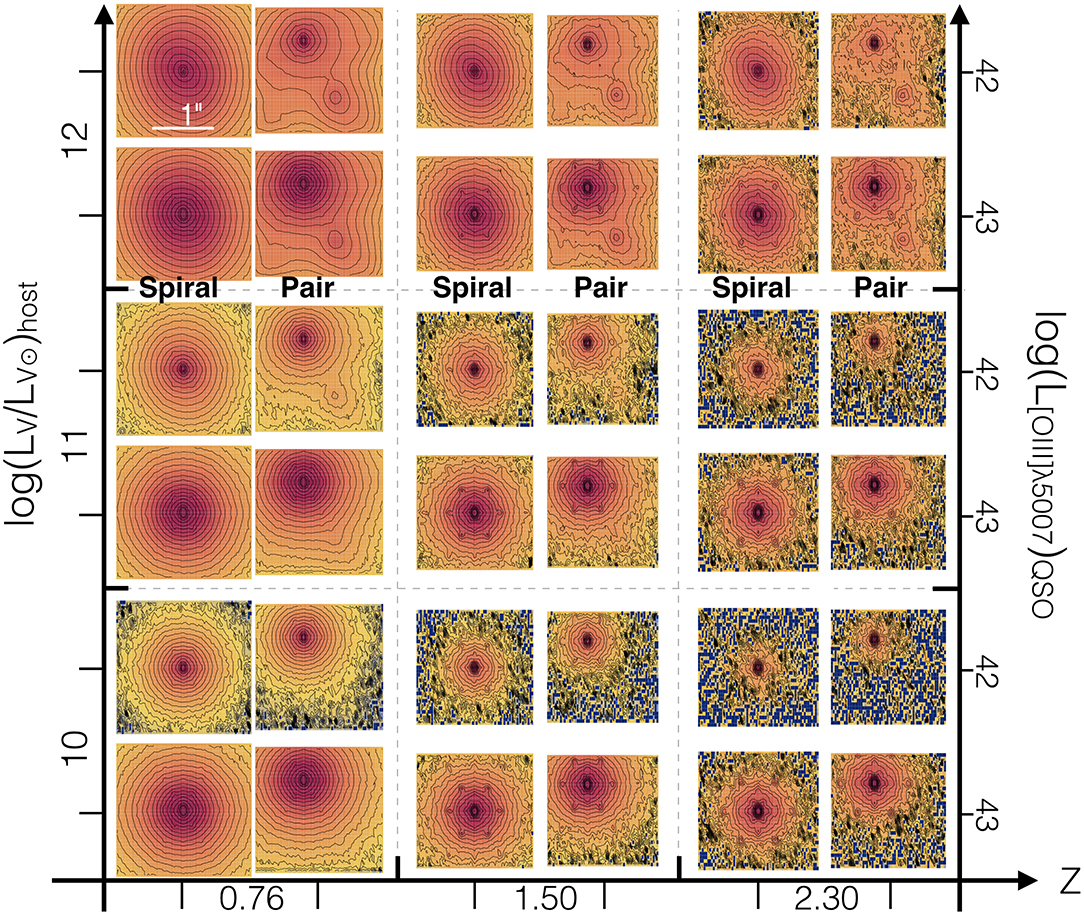
Figure 1. General view of the simulated HARMONI observations of QSO+host galaxies at redshift 0.76, 1.50, and 2.30 (X-axis). Images were obtained by integrating the mock HARMONI data cubes along the spectral direction in the ~4,700–5,300 Å rest-frame range. Left-Y-axis indicates host galaxies luminosities, while right-Y-axis corresponds to central QSO luminosity. The three upper panels correspond to the two host galaxies morphologies scaled to 1012 L⊙ for the two considered QSO luminosities (1042 and 1043 for the upper and lower subpanels, respectively). The three middle and bottom panels correspond to the two host galaxies morphologies scaled to 1011 L⊙ and 1010 L⊙, respectively, for the two QSO luminosities (1042 and 1043 for the upper and lower subpanels, respectively).
We obtained aperture-integrated spectra of the “observed” central QSO and the host galaxies by co-adding the simulated HARMONI datacubes along the spatial direction. Then we obtained the total flux and width of the Hβ broad component by fitting a single Gaussian function to each of the emission lines in the QSO aperture-integrated spectrum (i.e., [OIII]λλ4959,5007 and Hβ narrow and broad components) and assuming a linear continuum in the selected spectral range (i.e., 4,700–5,300 Å). This was used to estimate the black-hole mass (see Equation 6 in Vestergaard and Peterson, 2006). Then, we used pPXF code (Cappellari and Emsellem, 2004; Cappellari, 2017) and the MILES stellar library3 (Sánchez-Blázquez et al., 2006) to estimate the host galaxy stellar velocity dispersion. Table 1 summarizes the results of our analysis. The width and luminosity of the Hβ broad component were properly recovered for all the simulations performed. Therefore, the SMBH masses of QSO at the 0.76–2.30 redshift range and log(L([OIII]λ5007) between 42 and 43 can be properly derived from the HARMONI observations. Regarding the host galaxy, the stellar velocity dispersion can only be estimated within acceptable uncertainties (i.e., smaller than 40 km s−1) for the most massive and/or closest galaxies considered in the performed simulations.
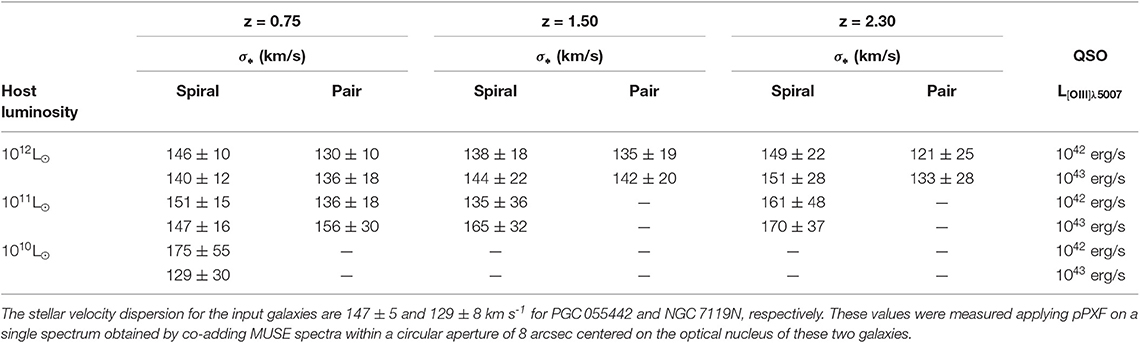
Table 1. Stellar velocity dispersion measured from the simulated HARMONI observations of QSO+host galaxies at three different redshifts.
In summary, from the analysis of these simulations, we conclude that the HARMONI instrument at the ELT will be able to obtain high quality observations of QSOs hosted by bright galaxies to unveil the cosmic evolution of SMBH-host scaling relations using reasonable observing times (i.e., 3 h per object).
Data Availability Statement
The datasets generated for this study are available on request to the corresponding author.
Author Contributions
BG-L, AM-I, and EM contributed to the conception and design of the work. NT and MP-S contributed to the conception, design, and development of the simulation tool. AM-I generated the input data and performed the simulations. BG-L analyzed the mock and wrote the first draft of the manuscript. All authors contributed to manuscript revision, read, and approved the submitted version.
Conflict of Interest
The authors declare that the research was conducted in the absence of any commercial or financial relationships that could be construed as a potential conflict of interest.
Acknowledgments
BG-L and AM-I acknowledge support from the State Research Agency (AEI) of the Spanish Ministry of Science, Innovation and Universities (MCIU) and the European Regional Development Fund (FEDER) under grant with reference AYA2105-68217-P. MP-S acknowledges support from the Comunidad de Madrid through Atracción de Talento Investigador Grant 2018-T1/TIC-11035 and STFC through grant ST/N000919/1. MP-S and NT acknowledge support from STFC through grant ST/N002717/1.
Footnotes
References
Aird, J., Coil, A. L., Georgakakis, A., Nandra, K., Barro, G., and Pérez-González, P. G. (2015). The evolution of the X-ray luminosity functions of unabsorbed and absorbed AGNs out to z~5. Mon. Not. R. Astron. Soc. 451, 1892–1927. doi: 10.1093/mnras/stv1062
Cappellari, M. (2017). Improving the full spectrum fitting method: accurate convolution with Gauss-Hermite functions. Mon. Not. R. Astron. Soc. 466, 798–811. doi: 10.1093/mnras/stw3020
Cappellari, M., and Emsellem, E. (2004). Parametric recovery of line-of-sight velocity distributions from absorption-line spectra of galaxies via penalized likelihood. Publ. Astron. Soc. Pacific 116, 138–147. doi: 10.1086/381875
Chiaberge, M., Gilli, R., Lotz, J. M., and Norman, C. (2015). Radio loud AGNs are mergers. Astrophys J. 806:147. doi: 10.1088/0004-637X/806/2/147
Davidson, K., and Netzer, H. (1979). The emission lines of quasars and similar objects. Rev. Modern Phys. 51, 715–766.
Floyd, D. J. E., Dunlop, J. S., Kukula, M. J., Brown, M. J. I., McLure, R. J., Baum, S. A., et al. (2013). Star formation in luminous quasar host galaxies at z = 1-2. Mon. Not. R. Astron. Soc. 429, 2–19. doi: 10.1103/RevModPhys.51.715
Galbany, L., Anderson, J. P., Rosales-Ortega, F. F., Kuncarayakti, H., Krühler, T., Sánchez, S. F., et al. (2016). Characterizing the environments of supernovae with MUSE. Mon. Not. R. Astron. Soc. 455, 4087–4099. doi: 10.1093/mnras/stv2620
Glikman, E., Simmons, B., Mailly, M., Schawinski, K., Urry, C. M., and Lacy, M. (2015). Major mergers host the most-luminous red quasars at z ~2: a hubble space telescope WFC3/IR study. Astrophys J. 806:218. doi: 10.1088/0004-637X/806/2/218
Husemann, B., Bennert, V. N., Scharwächter, J., Woo, J.-H., and Choudhury, O. S. (2016). The MUSE view of QSO PG 1307+085: an elliptical galaxy on the MBH-σ* relation interacting with its group environment. Mon. Not. R. Astron. Soc. 455, 1905–1918. doi: 10.1093/mnras/stv2478
Kaspi, S., Smith, P. S., Netzer, H., Maoz, D., Jannuzi, B. T., and Giveon, U. (2000). Reverberation measurements for 17 quasars and the size-mass-luminosity relations in active galactic nuclei. Astrophys. J. 533, 631–649. doi: 10.1086/308704
Kauffmann, G., Heckman, T. M., Tremonti, C., Brinchmann, J., Charlot, S., White, S. D. M., et al. (2003). The host galaxies of active galactic nuclei. Mon. Not. R. Astron. Soc. 346, 1055–1077. doi: 10.1111/j.1365-2966.2003.07154.x
Kormendy, J., and Ho, L. C. (2013). Coevolution (or not) of supermassive black holes and host galaxies. Annu. Rev. Astron. Astrophys. 51, 511–653. doi: 10.1146/annurev-astro-082708-101811
McLure, R. J., and Jarvis, M. J. (2002). Measuring the black hole masses of high-redshift quasars. Mon. Not. R. Astron. Soc. 337, 109–116. doi: 10.1046/j.1365-8711.2002.05871.x
Mejía-Restrepo, J. E., Trakhtenbrot, B., Lira, P., Netzer, H., and Capellupo, D. M. (2016). Active galactic nuclei at z 1.5 - II. Black hole mass estimation by means of broad emission lines. Mon. Not. R. Astron. Soc. 460, 187–211. doi: 10.1093/mnras/stw568
Neugebauer, G., Green, R. F., Matthews, K., Schmidt, M., Soifer, B. T., and Bennett, J. (1987). Continuum energy distributions of quasars in the Palomar-Green Survey. Astrophys. J. Suppl. Ser. 63, 615–644. doi: 10.1086/191175
Planck Collaboration, Ade, P. A. R., Aghanim, N., Arnaud, M., Ashdown, M., Aumont, J., et al. (2016). Planck 2015 results. XIII. Cosmological parameters. Astron. Astrophys. 594:A13. doi: 10.1051/0004-6361/201525830
Ramos Almeida, C., Tadhunter, C. N., Inskip, K. J., Morganti, R., Holt, J., and Dicken, D. (2011). The optical morphologies of the 2 Jy sample of radio galaxies: evidence for galaxy interactions. Mon. Not. R. Astron. Soc. 410, 1550–1576. doi: 10.1111/j.1365-2966.2010.17542.x
Saglia, R. P., Opitsch, M., Erwin, P., Thomas, J., Beifiori, A., Fabricius, M., et al. (2016). The SINFONI black hole survey: the black hole fundamental plane revisited and the paths of (co)evolution of supermassive black holes and bulges. Astrophys. J. 818:47. doi: 10.3847/0004-637X/818/1/47
Sánchez-Blázquez, P., Peletier, R. F., Jiménez-Vicente, J., Cardiel, N., Cenarro, A. J., Falcón-Barroso, J., et al. (2006). Medium-resolution Isaac Newton telescope library of empirical spectra. Mon. Not. R. Astron. Soc. 371, 703–718. doi: 10.1111/j.1365-2966.2006.10699.x
Thatte, N. A., Clarke, F., Bryson, I., Shnetler, H., Tecza, M., Fusco, T., et al. (2016). “The E-ELT first light spectrograph HARMONI: capabilities and modes,” in Society of Photo-Optical Instrumentation Engineers (SPIE) Conference Series, Vol. 9908 (Edinburgh) 99081X.
Vayner, A., Wright, S. A., Do, T., Larkin, J. E., Armus, L., and Gallagher, S. C. (2016). Providing stringent star formation rate limits of z~2 QSO host galaxies at high angular resolution. Astrophys. J. 821:64. doi: 10.3847/0004-637X/821/1/64
Vayner, A., Wright, S. A., Murray, N., Armus, L., Larkin, J. E., and Mieda, E. (2017). Galactic-scale feedback observed in the 3C 298 quasar host galaxy. Astrophys. J. 851:126. doi: 10.3847/1538-4357/aa9c42
Vestergaard, M., and Peterson, B. M. (2006). Determining central black hole masses in distant active galaxies and quasars. II. Improved optical and UV scaling relationships. Astrophys. J. 641, 689–709. doi: 10.1086/500572
Keywords: quasars, cosmology, extragalatic astronomy, active galactic nuclei, cosmological parameters
Citation: García-Lorenzo B, Monreal-Ibero A, Mediavilla E, Pereira-Santaella M and Thatte N (2019) Black Hole-Galaxy Scaling Relation Evolution From z~2.5: Simulated Observations With HARMONI on the ELT. Front. Astron. Space Sci. 6:73. doi: 10.3389/fspas.2019.00073
Received: 13 September 2019; Accepted: 20 November 2019;
Published: 06 December 2019.
Edited by:
Edi Bon, Astronomical Observatory, SerbiaReviewed by:
Daniela Bettoni, Osservatorio Astronomico di Padova (INAF), ItalyPaola Severgnini, Brera Astronomical Observatory, Italy
Copyright © 2019 García-Lorenzo, Monreal-Ibero, Mediavilla, Pereira-Santaella and Thatte. This is an open-access article distributed under the terms of the Creative Commons Attribution License (CC BY). The use, distribution or reproduction in other forums is permitted, provided the original author(s) and the copyright owner(s) are credited and that the original publication in this journal is cited, in accordance with accepted academic practice. No use, distribution or reproduction is permitted which does not comply with these terms.
*Correspondence: Begoña García-Lorenzo, bgarcia@iac.es