- 1Department of Physics, Engineering and Astronomy, Stephen F. Austin State University, Nacogdoches, TX, United States
- 2Department of Physics and Astronomy, University of North Carolina at Chapel Hill, Chapel Hill, NC, United States
- 3Institute for Astronomy, University of Hawai‘i at Mānoa, Hilo, HI, United States
- 4Division of Physics, Mathematics, and Astronomy, California Institute of Technology, Pasadena, CA, United States
- 5Cerro Tololo Inter-American Observatory, La Serena, Chile
In the past decade, space-based transit surveys have delivered thousands of potential planet-hosting systems. Each of these needs to be vetted and characterized using follow-up high-resolution imaging. We perform comprehensive imaging surveys of the candidate exoplanets detected by the Kepler and TESS missions using the fully autonomous Robo-AO system and the largely autonomous SOAR speckle imaging system. The surveys yielded hundreds of previously unknown close binary systems hosting exoplanets and resulted in verification of hundreds of exoplanet systems. Evidence of the interaction between binary stars and planetary systems was also detected, including a deep deficit of planets in close binary systems.
1 Introduction
Over the past decade, the Kepler telescope (Borucki et al., 2010) and its follow-up mission, the Transiting Exoplanet Survey Satellite (TESS, Ricker et al., 2014), have detected the majority of known exoplanets. Each satellite consists of high-precision photometers, able to measure the brightness of thousands of stars simultaneously. A planet passing in front of one of these stars as seen from Earth, a transit, will result in a slight dip in brightness (the size of the dip being related to relative sizes of the planet and star). Periodic dimming of a star is therefore potential evidence of an orbiting exoplanet.
The addition of a second star in the system, so that the light from both is blended together, results in a shallower brightness dip during transit. The size of the planet, which is estimated based on the depth of the brightness dip, is biased small when the light from a second object is included. The nearby star may actually be an eclipsing binary system. When blended with the brighter target star, the large dips from the eclipsing stars may result in a planet-like signal. Both the Kepler and TESS missions were blind to wide binary stars, which were not removed from either Kepler (Brown et al., 2011) or TESS (Stassun et al., 2019) input catalogs. The majority of close binary stars (those within an arcsecond of separation) are not known in advance due to the typically low-resolution of seeing-limited observations and the resolution limit of Gaia DR2 (Ziegler et al., 2018b).
Resolving close binary systems requires high-resolution imaging from the ground. Conventional systems, such as laser GuideStar adaptive optics (LGS-AO) instruments, require long overheads before observations can begin, typically on the order of 15–20 min [e.g., Keck-AO (Wizinowich et al., 2006)], and are generally only available on large telescopes (apertures greater than 8 m). With thousands of targets requiring such observations, approximately a hundred dedicated nights would be required to complete a comprehensive survey. Practically, this is outside the allocated time that will be provided for this purpose. In the first few years of the Kepler mission, the follow-up campaign proceeded with a patchwork of smaller surveys performed on different telescopes observing in both visible and infrared bands (Howell et al., 2011; Adams et al., 2012; Adams et al., 2013; Horch et al., 2012; Lillo-Box et al., 2012; Dressing et al., 2014; Horch et al., 2014; Lillo-Box et al., 2014; Marcy et al., 2014). These disparate sources of data limited the broad statistical studies that could be performed to understand how planets form and evolve in tight binary systems.
A high-resolution instrument which also has high observing efficiency is therefore required to perform such a large survey. Through full automation, Robo-AO achieves observing time efficiencies that are an order-of-magnitude greater than those of conventional high-resolution instruments. Between 2012 and 2016, Robo-AO was used by our team to observe every Kepler Object of Interest (KOI) system (Law et al., 2014; Baranec et al., 2016; Ziegler et al., 2017; Ziegler et al., 2018a; Ziegler et al., 2018c). These observations were typically sensitive to nearby stars as close as the diffraction limit of the telescope (approximately 0.15ʺ) and to stars up to six magnitudes fainter than the target star. Within this survey, nearly 95% of Kepler planetary candidates host stars (3,857 KOIs in total) were observed, and 620 stars with separations less than a few arcseconds were detected.
Beginning in late 2018 and continuing to present, the Southern Astrophysical Research telescope (SOAR) has performed speckle observations of TESS planet candidates. Speckle imaging on SOAR typically reaches the diffraction limit on bright targets
This article provides a summary of the surveys and their results. We describe in detail the observations from each instrument in Section 2 and summarize the results of the surveys in Section 3. We conclude in Section 4.
2 Observations
2.1 Robo-AO
The objective of the Robo-AO Kepler survey was to take image in high-resolution of every candidate planet host star detected by the Kepler telescope. We therefore targeted every KOI from the available data releases (culminating with the Kepler DR25 catalog based on Q1-Q17 data) (Borucki et al., 2010; Borucki et al., 2011a; Borucki et al., 2011b; Batalha et al., 2013; Burke et al., 2014; Rowe et al., 2014; Coughlin et al., 2016; Mathur et al., 2017). We removed KOIs that were flagged as false positives using Kepler data at the time of the observation runs.
The properties of targeted KOIs in the Robo-AO survey are presented in Figure 1. The distributions in magnitude, planetary radius, planetary orbital period, and stellar temperature of the observed stars are similar to the full set of KOIs from Q1 to Q17 that have CANDIDATE dispositions based on only Kepler data. This is a result of the comprehensive nature of this survey. An example of the Robo-AO images within this survey is presented in Figure 2.
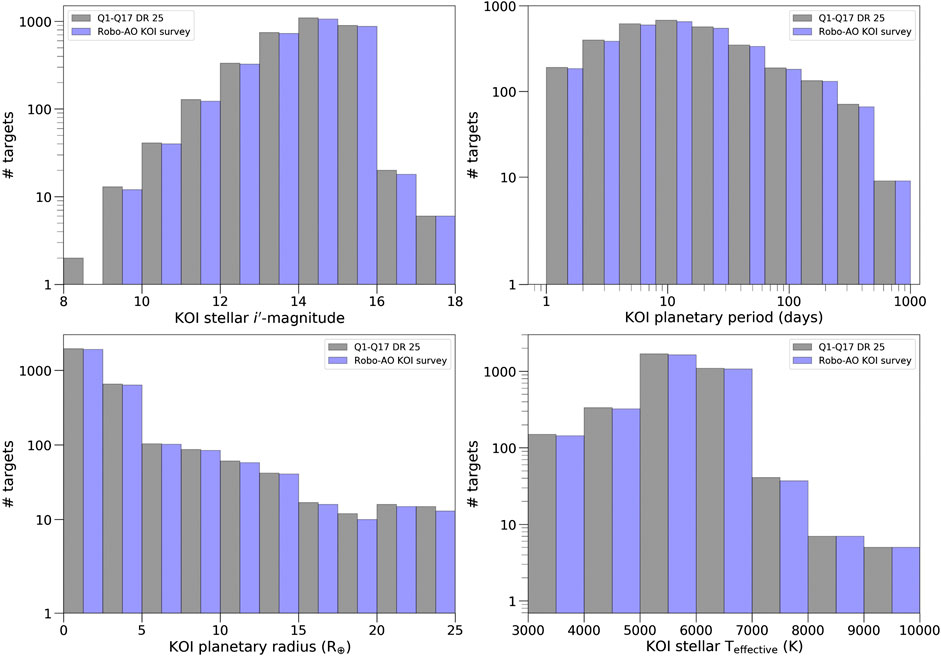
FIGURE 1. The properties of KOIs observed in the Robo-AO survey are compared to the full set of KOIs from Q1–Q17 (Borucki et al., 2010; Borucki et al., 2011a; Borucki et al., 2011b; Batalha et al., 2013; Burke et al., 2014; Rowe et al., 2014; Coughlin et al., 2016; Mathur et al., 2017).
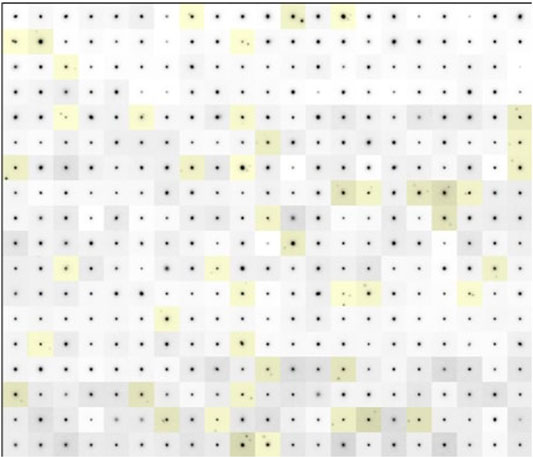
FIGURE 2. Robo-AO centered cutout images of Kepler planetary candidate host stars. Systems with discovered nearby stars are highlighted. Shown are ∼10% of the targets from the Robo-AO survey of KOIs, the largest adaptive optics survey of exoplanet hosts yet performed. Results from this survey have been used to validate over a thousand planets.
The Robo-AO instrument was mounted on telescopes at Palomar and Kitt Peak during the course of this survey (Baranec et al., 2014b; Baranec et al., 2017; Jensen-Clem et al., 2018). To correct high-order wavefront aberrations introduced by atmospheric turbulence, the adaptive optics system of Robo-AO runs at a loop rate of 1.2 kHz. The delivered performance of the system (median Strehl ratios of 9% and 4% in the
The Robo-AO system achieves a typical FWHM resolution of 0.15ʺ (at the diffraction limit). An electron-multiplying CCD (EMCCD) is used to record the images. This camera allows short frame rates, useful for software corrections for tip and tilt using a faint
A currently in-development Robo-AO 2 system (Baranec et al., 2014a) mounted on the UH-88 in telescope on Maunakea will be used in the future to take image in high-resolution of Northern TESS planet candidate hosts.
2.2 SOAR Speckle Imaging
We are observing TESS planet candidate hosts with the high-resolution camera (HRCam) imager on the 4.1 m SOAR telescope. TESS targets have been observed during 13 separate runs in 2018–2020. Over the course of these observations, 95% (707) of the 742 bright
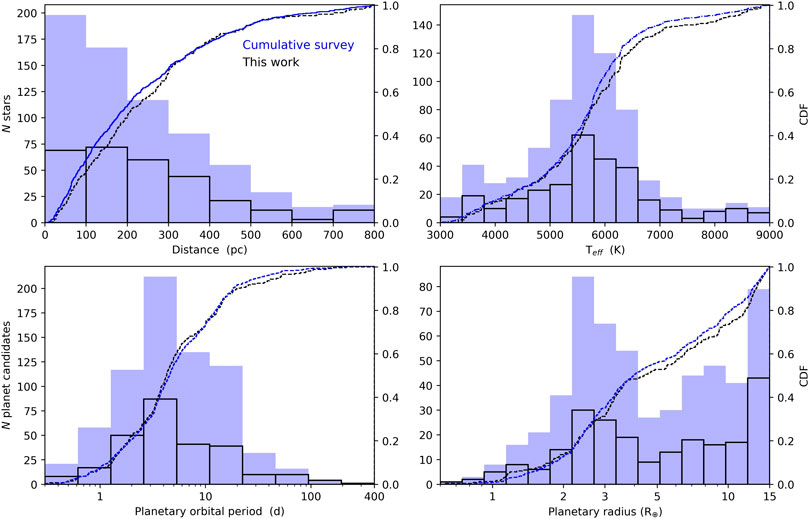
FIGURE 3. The properties of the 653 TESS planet candidate hosts observed in the SOAR TESS survey from Ziegler et al., (2020) and in preparation (this work). A cumulative density function for each property is overplotted in black. Targets identified as known or likely false positives, 196 in total, have been removed.
The observation procedure and data reduction are described in detail in Tokovinin (2018) and in Ziegler et al. (2020). In summary, an 11 s burst of 400 images is taken with an Andor iXon-888 camera. Each image consists of 200 × 200 binned pixels that are centered on the target star. The images subtend an angular region on the sky that is 6.3″ on a side, giving a plate scale of 0.01575″/pixel. A custom IDL script reduces the resulting datacube. A power spectrum is computed, and, if the target star is a binary, characteristic fringes are apparent. Modeling the power spectrum provides the separation, magnitude difference, and position angle of the companion. The Fourier transform of the power spectrum is the speckle autocorrelation function (ACF). Secondary stars will appear as mirrored peaks in the ACF, appearing at the separation and position angle of the companion. The frames in the datacube are shift-and-added, centering each on the brightest pixel, to determine the true position of the companion and remove the 180-degree ambiguity. Examples of typical speckle data are available in Figure 4 in Tokovinin (2018). The observations in the SOAR TESS survey were taken in I-band. This bandpass (λcen = 824 nm, Δλ = 170 nm) is similar to the bandpass used by TESS.
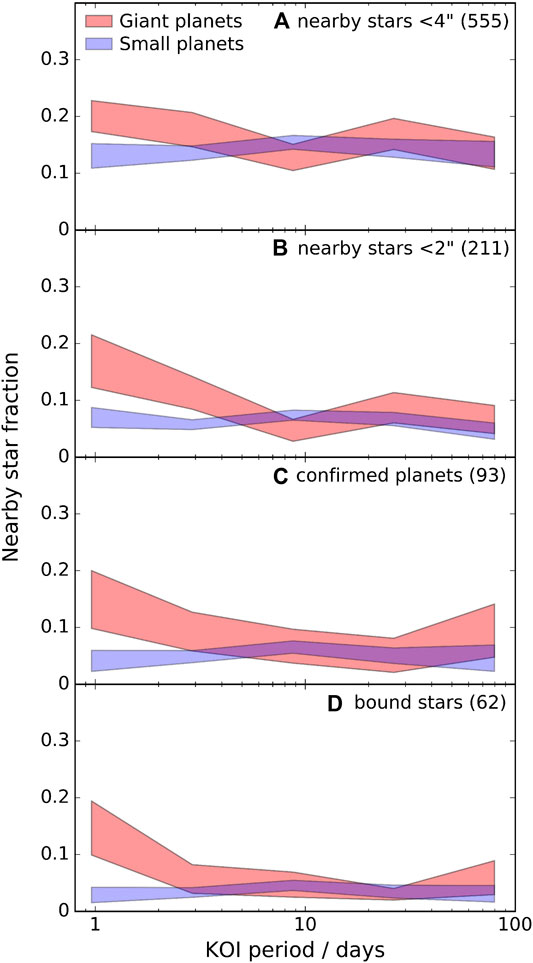
FIGURE 4. The binary fraction for two planetary populations (giant and small) is shown as a function of orbital period, with
3 Impact of Binary Stars on Planetary Systems
3.1 Binary Fractions
Within the Robo-AO Kepler survey, we found 610 stars within 4″ of 559 planetary candidate hosts (out of an observed total of 3,857 KOIs). This implies a nearby star fraction rate with the detection sensitivity of Robo-AO (separations between ∼0.15″ and 4.0″ and typically
Simulations using simulated galactic star fields and observational evidence suggest that most nearby stars at separations
The SOAR TESS survey finds companion rates to transiting exoplanet candidate hosts within 1.5″ and 3″ of 16.2 ± 1.7% and 23.2 ± 2.0% within 1.5″ and 3″, respectively.
The TESS nearby star rates are significantly higher than the Kepler rates. If we assume a physical separation distribution for binaries around exoplanet hosts as we find for field stars (Raghavan et al., 2010) which peaks at 50 AU, many more real binaries would be resolvable around TESS planet hosts (average distance of ∼200 pc) compared to Kepler planet hosts (average distance of ∼500 pc). The TESS system is also generally brighter (by ∼3 mags on average), making fainter companions more readily detectable.
3.2 Radius Corrections
The additional flux from a stellar companion will reduce the transit depth in a photometric light curves. This dilution will result in an underestimated planetary radius. In general, it is not known which of the two stars hosts the planet in an S-type configuration (i.e., a planet in a binary system that orbits only one of the stars) (Horch et al., 2014). Gaidos et al., (2016) provide some evidence, however, that typically the primary (brightest) star is more likely to be the planet host. We therefore estimated correction factors for radius estimates for either host scenario. The detailed description of how the radii of planet candidates are corrected for the presence of a previously unknown stellar companion is provided in Ziegler et al., (2018c).
We find, for the Kepler planets, that, if we assume that all the planets orbit around the primary stars, the planetary radii increase by a factor of 1.08 on average. This factor is relatively small, as generally the companions are much fainter than the primary stars and thus the dilution of the transit is small. We found a similar correction factor for TESS planets of 1.11. Instead, if we assume all planets orbit around the secondary stars (and assuming these are not line-of-sight asterisms, but gravitationally bound to the primary), the radii of the TESS planets will increase, on average, by a factor of 2.55, slightly less than 3.29 found for Kepler planets. If we instead assume that the planet candidates are equally likely to be hosted by the primary or secondary star, we find average radius correction factors for Kepler planets of 2.18 and for TESS planets of 1.82.
Unassociated background or foreground stars are typically found at larger separations from the primary star. If we limit our sample to just TESS systems with separations less than 1ʺ (to increase the fraction of gravitationally bound companions), we find, using the assumptions of all primary star hosts, all secondary star hosts, and equal mix of primary and secondary star hosts, correction factors of 1.14, 1.90, and 1.55, respectively. The final figure agrees with the correction factor from the Robo-AO survey of Kepler planets of 1.54, as well as from two independent studies of 1.6 (Ciardi et al., 2015) and 1.64 (Hirsch et al., 2017).
In summary, it is clear that the presence of a previously unknown stellar companion has a significant effect on our understanding of any possible planets within the system (increasing their radii by ∼60% on average). The composition of smaller planets, in particular, is highly dependent on their estimated radius, particularly if they fall below or above the radius gap at approximately 1.6–1.9 Earth radii (Rogers, 2015; Fulton et al., 2017; Van Eylen et al., 2018) between rocky planets (super-Earths) and those with large gaseous envelopes (sub-Neptunes).
3.3 Giant Planet Migration
It is expected from theoretical planet formation models that the gravitational influence of a stellar companion may drive planets that form at large separations inward, into short-period orbits (Fabrycky and Tremaine, 2007; Katz et al., 2011; Naoz et al., 2012). Smaller planets may be ejected by migrating larger planets in this scenario, resulting in a high fraction of short period giant planets in systems with stellar companions (Xie et al., 2014).
We searched for evidence of these effects using a cleaned sample of binary targets from the Robo-AO Kepler survey, removing known or suspected false positives (Morton and Johnson, 2011; Fressin et al., 2013) and only using likely bound systems as determined by photometric distance estimates Ziegler et al. (2018a).
We find that, after successive cuts to improve the sample (see Figure 4), short-period (1–3 days) giant and small planets have a binarity rate of
Ngo et al., (2015) found a similar result in an NIR survey of hot Jupiter hosts, which were twice as likely to have stellar companions as compared to field stars at a
3.4 Close Binary Suppression of Planets
A close stellar companion can significantly reduce the probability that planets can form and survive around a star. Yet, we still find planets in close binary systems. We use the data from the SOAR TESS survey to understand how binary stars interact with planetary systems.
Kraus et al., (2016) found that few Kepler host stars are in solar system scale binary systems (separations within 50 AU), implying that planets are significantly less likely to form in these systems. The TESS planets take generally larger and shorter periods than the Kepler planets, due to the reduced photometric precision of TESS and survey strategy. Unlike Kepler, however, the TESS planets are spread across the sky, not in a limited region, and allow us to sample a more diverse set of the Galactic stellar population.
Similar to Kraus et al., (2016), we use the field binary statistics of Raghavan et al., (2010) to compare the planet candidate hosting planets. Any differences between the two samples may be a result of planet formation suppression. For this analysis, we first cull the sample of 875 observed TOIs using several parameters (non-Solar type stars, false positives, and high contrast systems) to a final sample consisting of 484 stars. We supplement the SOAR observations with common proper motion pairs found in Gaia DR2 (Gaia Collaboration et al., 2018).
A histogram of the observed distribution of binaries based on projected separation compared to the simulated survey of field stars is shown in Figure 5. A deep deficit of observed exoplanet candidate systems with close binaries is apparent, indicating that these systems are treacherous for planet formation and evolution. A simple two-parameter suppression model, a step function reduction in binaries by
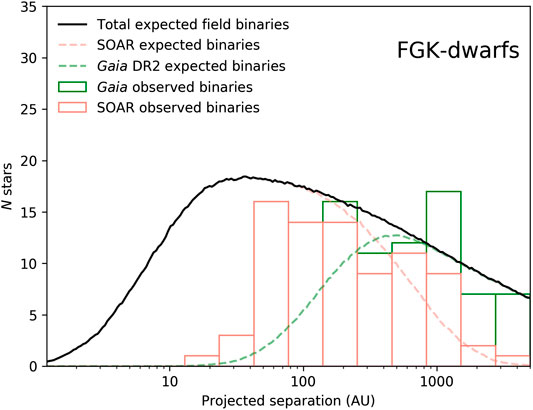
FIGURE 5. In red and green, the number of observed companions from SOAR and in Gaia DR2 for solar-type TESS planet candidate hosts in logarithmic bins of projected separation of 0.25 dex width. Companions found by both SOAR and Gaia are included in the SOAR sample. In black is the expected distribution from a multiplicity study of field stars (Raghavan et al., 2010), combining both field binaries that would be detected by SOAR and Gaia. The expected binaries from SOAR and Gaia, individually, are also plotted. These distributions take into account the detection sensitivity of both SOAR and Gaia. The observed distribution shows a clear paucity of TESS planet candidate host binaries at projected separations less than ∼40 AU compared to the field stars and are consistent with field expectations at wider separations.
The exact mechanism that suppresses the survival of planets in these systems is unclear, but several theories have been put forth. Quintana et al., (2007) suggest that stellar companions may stir planetesimals, increasing their velocity and reducing their density in the protoplanetary disc. Naoz et al., (2012) suggest that binary stars perturb planetary orbits, resulting in tidal migration that can lead to planetary ejection. Jang-Condell et al., (2008) and Kraus et al., (2012) find evidence that stellar companions reduce the material in, and lifetime of, protoplanetary discs. Lastly, Alexander (2012) suggests that the additional radiation from the companion increases photoevaporation in the disc, stripping gaseous planets of their atmospheres. Why can some systems survive while others are destroyed? More observations of these systems (to determine association between the two stars, identify the planet host, and map out physical rather than the snapshot projected separation) and the detection of more systems, particularly close binary systems that do host planets, will likely bring more insight. These systems are relatively rare and serve as the most stringent tests for theoretical formation models.
4 Conclusion
The Kepler and TESS missions provided the community the significant challenge of needing thousands of high-resolution images to confirm and characterize exoplanet systems. Robo-AO and SOAR speckle imaging are uniquely suited to perform those observations in a comprehensive and uniform manner. Over four years, Robo-AO imaged nearly all of the Kepler planet candidates. This corrected the planetary radius estimates for over 600 systems and led to the verification of over a thousand planets (Morton et al., 2016). The TESS survey is observing every TESS planet candidate visible from the South. Currently over 800 targets have been observed, with over 200 having nearby companions, and the speckle observations have contributed to the confirmation of over 40 planets [e.g.,Espinoza et al., (2019); Jones et al. (2019); Quinn et al., (2019); Rodriguez et al., (2019); Vanderburg et al., (2019)]. This has resulted in the best evidence yet that close, Solar System scale binary systems suppress planet formation. The TESS survey is ongoing with targets from the extended mission being observed.
Data from the Robo-AO survey of Kepler planet candidate host stars are available at the survey website2. Data from the SOAR telescope observations of TESS planet candidate host stars are available on the Exoplanet Follow-up Observation Program website3.
Data Availablity Statement
Publicly available datasets were analyzed in this study. This data can be found here: Kepler ExoFOP.
Author Contributions
CZ performed the data reduction for the Robo-AO survey. NL, CB, and RR built and maintained the Robo-AO instrument and ran the observations of the exoplanet hosts. AT performed the speckle observations on the SOAR telescope.
Funding
This research was supported by the NASA Exoplanets Research Program, grant No. NNX 15AC91G. CZ and WH acknowledge support from the North Carolina Space Grant consortium. TM was supported by NASA, grant No. NNX 14AE11G under the Kepler Participating Scientist Program.
Acknowledgments
We thank the observatory staff at Kitt Peak for their efforts to assist Robo-AO KP operations and are grateful to the Palomar Observatory staff for their support of Robo-AO on the 1.5 m telescope. The Robo-AO instrument was developed with support from the National Science Foundation under grants AST-0906060, AST-0960343, and AST-1207891, IUCAA, the Mt. Cuba Astronomical Foundation, and by a gift from Samuel Oschin. The Robo-AO team thanks NSF and NOAO for making the Kitt Peak 2.1 m telescope available. Robo-AO KP is a partnership between the California Institute of Technology, the University of Hawai’i, the University of North Carolina at Chapel Hill, the Inter-University Centre for Astronomy and Astrophysics (IUCAA) at Pune, India, and the National Central University, Taiwan. The Murty family feels very happy to have added a small value to this important project. Robo-AO KP is also supported by grants from the John Templeton Foundation and the Mt. Cuba Astronomical Foundation. Some data are based on observations at Kitt Peak National Observatory, National Optical Astronomy Observatory (NOAO Prop. ID: 15B-3001), which is operated by the Association of Universities for Research in Astronomy (AURA) under cooperative agreement with the National Science Foundation. This research used observations obtained at the Southern Astrophysical Research (SOAR) telescope, which is a joint project of the Ministério da Ciência, Tecnologia, Inovações e Comunicações (MCTIC) do Brasil, the U.S. National Optical Astronomy Observatory (NOAO), the University of North Carolina at Chapel Hill (UNC), and Michigan State University (MSU). This article includes data collected by the TESS mission. Funding for the TESS mission is provided by the NASA Explorer Program. This work has made use of data from the European Space Agency (ESA) mission Gaia (https://www.cosmos.esa.int/gaia), processed by the Gaia Data Processing and Analysis Consortium (DPAC, https://www.cosmos.esa.int/web/gaia/dpac/consortium). This research has made use of the Exoplanet Follow-up Observation Program website, which is operated by the California Institute of Technology, under contract with the National Aeronautics and Space Administration under the Exoplanet Exploration Program. This research has made use of the NASA Exoplanet Archive, which is operated by the California Institute of Technology, under contract with the National Aeronautics and Space Administration under the Exoplanet Exploration Program. Facilities: PO:1.5 m (Robo-AO), KPNO:2.1 m (Robo-AO), SOAR (HRCam).
Conflict of Interest
The authors declare that the research was conducted in the absence of any commercial or financial relationships that could be construed as a potential conflict of interest.
Footnotes
1Errors for both populations are based on Poissonian statistics (Burgasser et al., 2003).
3https://exofop.ipac.caltech.edu/tess/index.php.
References
Adams, E. R., Ciardi, D. R., Dupree, A. K., Gautier, T. N., Kulesa, C., and McCarthy, D. (2012). Adaptive optics images of kepler objects of interest. AJ 144, 42. doi:10.1088/0004-6256/144/2/42
Adams, E. R., Dupree, A. K., Kulesa, C., and McCarthy, D. (2013). Adaptive optics images. II. 12 kepler objects of interest and 15 confirmed transiting planets. AJ 146, 9. doi:10.1088/0004-6256/146/1/9
Alexander, R. (2012). The dispersal of protoplanetary disks around binary stars. ApJ 757, L29. doi:10.1088/2041-8205/757/2/L29
Baranec, C., Riddle, R., and Law, N. M. (2017). Automated adaptive optics. ArXiv e-prints. Available at: https://arxiv.org/abs/1709.07103
Baranec, C., Riddle, R., Law, N. M., Chun, M. R., Lu, J. R., Connelley, M. S., et al. (2014a). “Second generation Robo-AO instruments and systems,” In Adaptive optics systems IV (Bellingham, WA: SPIE), Vol. 9148, 914812. doi:10.1117/12.2055552
Baranec, C., Riddle, R., Law, N. M., Ramaprakash, A. N., Tendulkar, S., Hogstrom, K., et al. (2014b). High-efficiency autonomous laser adaptive optics. ApJ 790, L8. doi:10.1088/2041-8205/790/1/L8
Baranec, C., Ziegler, C., Law, N. M., Morton, T., Riddle, R., Atkinson, D., et al. (2016). Robo-AO kepler planetary candidate survey. II. Adaptive optics imaging of 969 kepler exoplanet candidate host stars. AJ 152, 18. doi:10.3847/0004-6256/152/1/18
Batalha, N. M., Rowe, J. F., Bryson, S. T., Barclay, T., Burke, C. J., Caldwell, D. A., et al. (2013). Planetary candidates observed by kepler. III. Analysis of the first 16 months of data. ApJs 204, 24. doi:10.1088/0067-0049/204/2/24
Borucki, W. J., Koch, D., Basri, G., Batalha, N., Brown, T., Caldwell, D., et al. (2010). Kepler planet-detection mission: introduction and first results. Science 327, 977. doi:10.1126/science.1185402
Borucki, W. J., Koch, D. G., Basri, G., Batalha, N., Boss, A., Brown, T. M., et al. (2011a). Characteristics of kepler planetary candidates based on the first data set. ApJ 728, 117. doi:10.1088/0004-637X/728/2/117
Borucki, W. J., Koch, D. G., Basri, G., Batalha, N., Brown, T. M., Bryson, S. T., et al. (2011b). Characteristics of planetary candidates observed by kepler. II. Analysis of the first four months of data. ApJ 736, 19. doi:10.1088/0004-637X/736/1/19
Gaia Collaboration Brown, A. G. A., Vallenari, A., Prusti, T., de Bruijne, J. H. J., Babusiaux, C., et al. (2018). Gaia data release 2. Summary of the contents and survey properties. Astron. Astrophys. 616, A1. doi:10.1051/0004-6361/201833051
Brown, T. M., Latham, D. W., Everett, M. E., and Esquerdo, G. A. (2011). Kepler input catalog: photometric calibration and stellar classification. AJ 142, 112. doi:10.1088/0004-6256/142/4/112
Burgasser, A. J., Kirkpatrick, J. D., Reid, I. N., Brown, M. E., Miskey, C. L., and Gizis, J. E. (2003). Binarity in Brown dwarfs: T dwarf binaries discovered with the hubble space telescope wide field planetary camera 2. ApJ 586, 512–526. doi:10.1086/346263
Burke, C. J., Bryson, S. T., Mullally, F., Rowe, J. F., Christiansen, J. L., Thompson, S. E., et al. (2014). Planetary candidates observed by kepler IV: planet sample from Q1-Q8 (22 Months). ApJs 210, 19. doi:10.1088/0067-0049/210/2/19
Ciardi, D. R., Beichman, C. A., Horch, E. P., and Howell, S. B. (2015). Understanding the effects of stellar multiplicity on the derived planet radii from transit surveys: implications for kepler, K2, and TESS. ApJ 805, 16. doi:10.1088/0004-637X/805/1/16
Coughlin, J. L., Mullally, F., Thompson, S. E., Rowe, J. F., Burke, C. J., Latham, D. W., et al. (2016). Planetary candidates observed by kepler. VII. The first fully uniform catalog based on the entire 48-month data set (Q1-Q17 DR24). ApJs 224, 12. doi:10.3847/0067-0049/224/1/12
Dressing, C. D., Adams, E. R., Dupree, A. K., Kulesa, C., and McCarthy, D. (2014). Adaptive optics images. III. 87 kepler objects of interest. AJ 148, 78. doi:10.1088/0004-6256/148/5/78
Espinoza, N., Brahm, R., Henning, T., Jordán, A., Dorn, C., Rojas, F., et al. (2019). HD 213885b: a transiting 1-day-period super-Earth with an Earth-like composition around a bright (V = 7.9) star unveiled by TESS. Mon. Not. R. Astron. Soc. 491 (2), 2982–2999. doi:10.1093/mnras/stz3150
Fabrycky, D., and Tremaine, S. (2007). Shrinking binary and planetary orbits by kozai cycles with tidal friction. ApJ 669, 1298–1315. doi:10.1086/521702
Fressin, F., Torres, G., Charbonneau, D., Bryson, S. T., Christiansen, J., Dressing, C. D., et al. (2013). The false positive rate of kepler and the occurrence of planets. ApJ 766, 81. doi:10.1088/0004-637X/766/2/81
Fulton, B. J., Petigura, E. A., Howard, A. W., Isaacson, H., Marcy, G. W., Cargile, P. A., et al. (2017). The California-kepler survey. III. a gap in the radius distribution of small planets. AJ 154, 109. doi:10.3847/1538-3881/aa80eb
Gaidos, E., Mann, A. W., Kraus, A. L., and Ireland, M. (2016). They are small worlds after all: revised properties of kepler M dwarf stars and their planets. Mon. Not. Roy. Astron. Soc. 457, 2877–2899. doi:10.1093/mnras/stw097
Hirsch, L. A., Ciardi, D. R., Howard, A. W., Everett, M. E., Furlan, E., Saylors, M., et al. (2017). Assessing the effect of stellar companions from high-resolution imaging of kepler objects of interest. AJ 153, 117. doi:10.3847/1538-3881/153/3/117
Horch, E. P., Howell, S. B., Everett, M. E., and Ciardi, D. R. (2014). Most sub-arcsecond companions of kepler exoplanet candidate host stars are gravitationally bound. ApJ 795, 60. doi:10.1088/0004-637X/795/1/60
Horch, E. P., Howell, S. B., Everett, M. E., and Ciardi, D. R. (2012). Observations of binary stars with the differential speckle survey instrument. IV. Observations of kepler, CoRoT, and hipparcos stars from the gemini north telescope. AJ 144, 165. doi:10.1088/0004-6256/144/6/165
Howell, S. B., Everett, M. E., Sherry, W., Horch, E., and Ciardi, D. R. (2011). Speckle camera observations for the NASA kepler mission follow-up Program. AJ 142, 19. doi:10.1088/0004-6256/142/1/19
Jang-Condell, H., Mugrauer, M., and Schmidt, T. (2008). Disk truncation and planet formation in γ cephei. ApJ 683, L191. doi:10.1086/591791
Jensen-Clem, R., Duev, D. A., Riddle, R., Salama, M., Baranec, C., Law, N. M., et al. (2018). The performance of the robo-AO laser guide star adaptive optics system at the Kitt peak 2.1 m telescope. AJ 155, 32. doi:10.3847/1538-3881/aa9be6
Jones, M. I., Brahm, R., Espinoza, N., Wang, S., Shporer, A., Henning, T., et al. (2019). HD 2685 b: a hot Jupiter orbiting an early F-type star detected by TESS. Astron. Astrophys. 625, A16. doi:10.1051/0004-6361/201834640
Katz, B., Dong, S., and Malhotra, R. (2011). Long-term cycling of kozai-lidov cycles: extreme eccentricities and inclinations excited by a distant eccentric perturber. Phys. Rev. Lett. 107, 181101. doi:10.1103/PhysRevLett.107.181101
Kraus, A. L., Ireland, M. J., Hillenbrand, L. A., and Martinache, F. (2012). The role of multiplicity in disk evolution and planet formation. ApJ 745, 19. doi:10.1088/0004-637X/745/1/19
Kraus, A. L., Ireland, M. J., Huber, D., Mann, A. W., and Dupuy, T. J. (2016). The impact of stellar multiplicity on planetary systems. I. The ruinous influence of close binary companions. AJ 152, 8. doi:10.3847/0004-6256/152/1/8
Law, N. M., Morton, T., Baranec, C., Riddle, R., Ravichandran, G., Ziegler, C., et al. (2014). Robotic laser adaptive optics imaging of 715 kepler exoplanet candidates using robo-AO. ApJ 791, 35. doi:10.1088/0004-637X/791/1/35
Lillo-Box, J., Barrado, D., and Bouy, H. (2014). High-resolution imaging of Kepler planet host candidates. a comprehensive comparison of different techniques. Astron. Astrophys. 566, A103. doi:10.1051/0004-6361/201423497
Lillo-Box, J., Barrado, D., and Bouy, H. (2012). Multiplicity in transiting planet-host stars. a lucky imaging study of kepler candidates. Astron. Astrophys. 546, A10. doi:10.1051/0004-6361/201219631
Marcy, G. W., Isaacson, H., Howard, A. W., Rowe, J. F., Jenkins, J. M., Bryson, S. T., et al. (2014). Masses, radii, and orbits of small kepler planets: the transition from gaseous to rocky planets. ApJs 210, 20. doi:10.1088/0067-0049/210/2/20
Mathur, S., Huber, D., Batalha, N. M., Ciardi, D. R., Bastien, F. A., Bieryla, A., et al. (2017). Revised stellar properties of kepler targets for the Q1-17 (DR25) transit detection run. ApJs 229, 30. doi:10.3847/1538-4365/229/2/30
Morton, T. D., Bryson, S. T., Coughlin, J. L., Rowe, J. F., Ravichandran, G., Petigura, E. A., et al. (2016). False positive probabilities for all kepler objects of interest: 1284 newly validated planets and 428 likely false positives. ApJ 822, 86. doi:10.3847/0004-637X/822/2/86
Morton, T. D., and Johnson, J. A. (2011). On the low false positive probabilities of kepler planet candidates. ApJ 738, 170. doi:10.1088/0004-637X/738/2/170
Naoz, S., Farr, W. M., and Rasio, F. A. (2012). On the formation of hot jupiters in stellar binaries. ApJ 754, L36. doi:10.1088/2041-8205/754/2/L36
Ngo, H., Knutson, H. A., Hinkley, S., Crepp, J. R., Bechter, E. B., Batygin, K., et al. (2015). Friends of hot jupiters. II. no correspondence between hot-jupiter spin-orbit misalignment and the incidence of directly imaged stellar companions. ApJ 800, 138. doi:10.1088/0004-637X/800/2/138
Quinn, S. N., Becker, J. C., Rodriguez, J. E., Hadden, S., Huang, C. X., Morton, T. D., et al. (2019). Near-resonance in a system of sub-Neptunes from TESS. AJ 158 (5), 177. doi:10.3847/1538-3881/ab3f2b
Quintana, E. V., Adams, F. C., Lissauer, J. J., and Chambers, J. E. (2007). Terrestrial planet formation around individual stars within binary star systems. ApJ 660, 807–822. doi:10.1086/512542
Raghavan, D., McAlister, H. A., Henry, T. J., Latham, D. W., Marcy, G. W., Mason, B. D., et al. (2010). A survey of stellar families: multiplicity of solar-type stars. ApJs 190, 1–42. doi:10.1088/0067-0049/190/1/1
Ricker, G. R., Winn, J. N., Vanderspek, R., Latham, D. W., Bakos, G. Á., Bean, J. L., et al. (2014). “Transiting exoplanet survey satellite (TESS),” In Space telescopes and instrumentation 2014: optical, infrared, and millimeter wave, Bellingham, WA, August 28, 2014 (Bellingham, WA: Society of Photo-Optical Instrumentation Engineers (SPIE)), Vol. 9143, 914320. doi:10.1117/12.2063489
Rodriguez, J. E., Quinn, S. N., Huang, C. X., Vanderburg, A., Penev, K., Brahm, R., et al. (2019). An eccentric massive jupiter orbiting a subgiant on a 9.5-day period discovered in the transiting exoplanet survey satellite full frame images. AJ 157, 191. doi:10.3847/1538-3881/ab11d9
Roell, T., Neuhäuser, R., Seifahrt, A., and Mugrauer, M. (2012). Extrasolar planets in stellar multiple systems. A&A 542, A92. doi:10.1051/0004-6361/201118051
Rogers, L. A. (2015). Most 1.6 earth-radius planets are not rocky. ApJ 801, 41. doi:10.1088/0004-637X/801/1/41
Rowe, J. F., Bryson, S. T., Marcy, G. W., Lissauer, J. J., Jontof-Hutter, D., Mullally, F., et al. (2014). Validation of kepler’s multiple planet candidates. III. light curve analysis and announcement of hundreds of new multi-planet systems. ApJ 784, 45. doi:10.1088/0004-637X/784/1/45
Stassun, K. G., Oelkers, R. J., Paegert, M., Torres, G., Pepper, J., De Lee, N., et al. (2019). The revised TESS input catalog and candidate target list. AJ 158 (4), 138. doi:10.3847/1538-3881/aade86
Tokovinin, A. (2018). Ten years of speckle interferometry at SOAR. PASP 130, 035002. doi:10.1088/1538-3873/aaa7d9
Van Eylen, V., Agentoft, C., Lundkvist, M. S., Kjeldsen, H., Owen, J. E., Fulton, B. J., et al. (2018). An asteroseismic view of the radius valley: stripped cores, not born rocky. MNRAS 479, 4786–4795. doi:10.1093/mnras/sty1783
Vanderburg, A., Huang, C. X., Rodriguez, J. E., Becker, J. C., Ricker, G. R., Vanderspek, R. K., et al. (2019). TESS spots a compact system of super-earths around the naked-eye star HR 858. Astrophys. J. Lett. 881 (1), L19. doi:10.3847/2041-8213/ab322d
Wizinowich, P. L., Le Mignant, D., Bouchez, A. H., Campbell, R. D., Chin, J. C. Y., Contos, A. R., et al. (2006). The W. M. Keck observatory laser guide star adaptive optics system: overview. Publ. Astron. Soc. Pac. 118, 297–309. doi:10.1086/499290
Xie, J.-W., Wu, Y., and Lithwick, Y. (2014). Frequency of close companions among kepler planets—a transit time variation study. ApJ 789, 165. doi:10.1088/0004-637X/789/2/165
Ziegler, C., Law, N. M., Baranec, C., Howard, W., Morton, T., Riddle, R., et al. (2018a). Robo-AO kepler survey. V. the effect of physically associated stellar companions on planetary systems. AJ 156, 83. doi:10.3847/1538-3881/aace59
Ziegler, C., Law, N. M., Baranec, C., Morton, T., Riddle, R., De Lee, N., et al. (2018b). Measuring the recoverability of close binaries in Gaia DR2 with the robo-AO kepler survey. AJ 156, 259. doi:10.3847/1538-3881/aad80a
Ziegler, C., Law, N. M., Baranec, C., Riddle, R., Duev, D. A., Howard, W., et al. (2018c). Robo-AO kepler survey. IV. the effect of nearby stars on 3857 planetary candidate systems. AJ 155 (4), 161. doi:10.3847/1538-3881/aab042
Ziegler, C., Law, N. M., Morton, T., Baranec, C., Riddle, R., Atkinson, D., et al. (2017). Robo-AO kepler planetary candidate survey. III. adaptive optics imaging of 1629 kepler exoplanet candidate host stars. AJ 153, 66. doi:10.3847/1538-3881/153/2/66
Keywords: exoplanets, adaptive optics, speckle interferometry, binary stars, high-resolution imaging
Citation: Ziegler C, Law N, Baranec C, Riddle R and Tokovinin A (2021) Robo-AO and SOAR High-Resolution Surveys of Exoplanet Hosting Stars. Front. Astron. Space Sci. 8:625230. doi: 10.3389/fspas.2021.625230
Received: 02 November 2020; Accepted: 08 January 2021;
Published: 29 March 2021.
Edited by:
Steve B. Howell, National Aeronautics and Space Administration (NASA), United StatesReviewed by:
Luca Fossati, Austrian Academy of Sciences, AustriaJohn Livingston, The University of Tokyo, Japan
Copyright © 2021 Ziegler, Law, Baranec, Riddle and Tokovinin. This is an open-access article distributed under the terms of the Creative Commons Attribution License (CC BY). The use, distribution or reproduction in other forums is permitted, provided the original author(s) and the copyright owner(s) are credited and that the original publication in this journal is cited, in accordance with accepted academic practice. No use, distribution or reproduction is permitted which does not comply with these terms.
*Correspondence: Carl Ziegler, Y2FybC56aWVnbGVyQHNmYXN1LmVkdQ==