- National Astronomical Observatories, Chinese Academy of Sciences, Beijing, China
The spiral structure in the solar neighborhood is an important issue in astronomy. In the past few years, there has been significant progress in observation. The distances for a large number of good spiral tracers, i.e., giant molecular clouds, high-mass star-formation region masers, HII regions, O-type stars, and young open clusters, have been accurately estimated, making it possible to depict the detailed properties of nearby spiral arms. In this work, we first give an overview about the research status for the Galaxy’s spiral structure based on different types of tracers. Then the objects with distance uncertainties better than 15% and <0.5 kpc are collected and combined together to depict the spiral structure in the solar neighborhood. Five segments related with the Perseus, Local, Sagittarius-Carina, Scutum-Centaurus, and Norma arms are traced. With the large dataset, the parameters of the nearby arm segments are fitted and updated. Besides the dominant spiral arms, some substructures probably related to arm spurs or feathers are also noticed and discussed.
1 Introduction
As observers deeply embedded in the Galactic disk, mapping the spiral structure of the Milky Way and understanding its formation and evolution have long been difficult issues in astronomy. Superpositions of multiple structures along the same observed line of sight have to be solved to trace the distribution of matters in our Galaxy. Additionally, the widespread dust in the interstellar medium causes extinction, making the situation more complex. However, because we live in the Milky Way, the positions and kinematics for a large number of objects could be measured with high accuracy, making the Milky Way the only galaxy in the Universe that we can investigate in detail.
Its spiral structure is one of the fundamental characteristics of the Milky Way. It has considerable influences on some other research fields, such as the kinematics of nearby stars (e.g., Williams et al., 2013; Hunt et al., 2019; Trick et al., 2021), Galactic electron-density distribution (Taylor and Cordes, 1993; Yao et al., 2017, Han et al., 2021), Galactic dust distribution and extinction map (Drimmel and Spergel, 2001; Hottier et al., 2020), and the large-scale magnetic field of the Milky Way (e.g., Han, 2017). There have been quite a few reviews about the global properties of the Galaxy’s spiral structure, Foster and Cooper (2010), Xu et al. (2018b), and Shen and Zheng (2020) reviewed previous efforts. Although, disagreements remain in some details, a general consensus that a global spiral pattern exists in the Galactic disk has been achieved. In this work, we focus on the solar neighborhood, where the spiral structure can be better understood, because the distances of a large number of nearby objects can be measured accurately. Significant progress has been made in the past few years by taking advantage of the astrometry measurements in radio to optical bands, which enables us to reliably delineate the nearby spiral structure in unprecedented detail.
Morgan et al. (1952) and Morgan et al. (1953) first delineated three arm segments in the solar neighborhood with a sample of aggregates of high-luminosity O to A-type stars in the 1950s. The three segments are now known to be related to the Sagittarius-Carina, Local, and Perseus arms. At that time, these structures were also studied by using different methods (e.g., Thackeray, 1956; Bok, 1964; Bok et al., 1970; Georgelin and Georgelin, 1971), such as, mapping the distribution of HII regions (Courtès et al., 1970), analyzing multiple structures shown in the HI 21 cm line surveys (van de Hulst et al., 1954), or implied in the observational data of other interstellar absorption lines toward background stars (Münch, 1953). In the 1970s, Georgelin and Georgelin (1976) proposed the famous model of the Galaxy’s spiral structure consisting of four major spiral arm segments. The Sun was placed in the inter-arm region between the Perseus arm and the Sagittarius arm. Then, the picture of the Galaxy’s spiral structure was extended by taking advantage of more observational data of different types of spiral tracers e.g., HII regions (e.g., Downes et al., 1980; Caswell and Haynes, 1987; Russeil, 2003; Paladini et al., 2004), molecular clouds (e.g., Cohen et al., 1980, 1985; Hou et al., 2009; Lépine et al., 2011), neutral atomic gas (Simonson, 1970; Burton, 1973; Levine et al., 2006; Koo et al., 2017), high-mass star-formation region (HMSFR) masers (e.g., Xu et al., 2006; Reid et al., 2009), OB stars (e.g., Miller, 1972; Stothers and Frogel, 1974; de Zeeuw et al., 1999; Wright, 2020), open clusters (e.g., Becker, 1964; Becker and Fenkart, 1970; Janes et al., 1988; Dias and Lépine, 2005), and cepheids (e.g., Fernie, 1968; Majaess et al., 2009). These great efforts enhanced our understanding of the global properties of the Galaxy’s spiral structure. For the spiral structure in the solar neighborhood, Xu et al. (2013) first found that many HMSFR masers with accurate VLBI parallax measurements (Xu et al., 2006) are situated in the Local arm, indicating that the Local arm is probably a major arm segment, rather than an inter-arm spur or a branch as has been suggested for a long time. The existence of the Local arm also challenges the formation mechanism of the Galaxy’s spiral structure (Xu et al., 2016), since it would be difficult to explain its existence according to the standard density-wave theory (Yuan, 1969; Shu, 2016), owing to the narrow space between the Sagittarius arm and Perseus arm.
In the past few years, there has been significant progress in observations by taking advantage of the VLBI observations in the radio band (Reid et al., 2019; Hirota et al., 2020) and Gaia astrometry measurements in the optical band (Prusti et al., 2016). Accurate parallaxes and proper motions have been obtained for a large number of HMSFR masers (e.g., Reid et al., 2019), HII regions (e.g., Hou et al., 2021), O-type stars (e.g., Xu et al., 2021), young open clusters (OCs, e.g., Dias et al., 2021), and evolved stars (e.g., Khoperskov et al., 2020). Additionally, many giant molecular clouds (GMCs) have had accurately determined distances based on the multi-wavelength survey data from optical to infrared bands or the astrometric data of foreground/background stars (Yan et al., 2019; Chen et al., 2020). By combining the available data of different types of tracers together, it is now possible to reliably map the detailed spiral structure within about 5 kpc of the Sun.
In this work, we first give an overview about the observational status for each type of spiral tracer; the available dataset of these objects with accurate distances are collected. Then, we combine them together to give a detailed description of the spiral structure in the solar neighborhood. Conclusions and discussions follow in the last section.
2 An Overview of Spiral Tracers
Young objects (HMSFR masers, HII regions, massive OB stars, and young open clusters, etc.) and GMCs are known as good tracers for the Galaxy’s spiral structure (hereafter gas arms). In addition, the spiral structure is imprinted by the distribution of old and evolved stars (hereafter stellar arms). The gas arms and stellar arms in a galaxy are not necessarily coincident with each other. Discovering a large number of spiral tracers widely spread through the Galactic disk and measuring their distances as accurately as possible are the key to settle the disputes on the spiral structure of our Milky Way Galaxy. In the following, the observational status about GMCs, HMSFR masers, HII regions, OB stars, young OCs and evolved stars are discussed, respectively.
2.1 Giant Molecular Clouds
Giant molecular clouds are the vast assemblies of molecular gas with masses from ∼103M⊙ to ∼107M⊙ (e.g., Murray, 2011). They are believed to primarily form in spiral arms (Dobbs and Baba, 2014) and are the nurseries of most young stars in a galaxy. In the Milky Way, GMCs have long been known as good tracers of spiral arms (e.g., Myers et al., 1986; Grabelsky et al., 1988; Hou and Han, 2014). From the wealthy dataset of Galactic CO surveys (see Heyer and Dame, 2015, for a review), a large number of isolated molecular clouds have been identified by different methods (e.g., García et al., 2014; Rice et al., 2016; Miville-Deschênes et al., 2017; Yan et al., 2020; Duarte-Cabral et al., 2021). For the majority of them, only kinematic distances are known, which depend on the adopted Galaxy rotation curve, the solution of the kinematic distance ambiguity, and deviation from the hypothetic circular rotation. For instance, Duarte-Cabral et al. (2021) compiled a large catalogue of 10,663 molecular clouds in the inner Galaxy. They estimated the kinematic distances for 10,300 clouds after solving the distance ambiguities through different methods. In addition to determining the distances of molecular clouds and then mapping their distribution in the Galactic disk, the other two methods have been used to reveal the Galaxy’s spiral structure with CO surveys: 1) Deconvolution of the survey data cube (e.g., Pohl et al., 2008; Nakanishi and Sofue, 2016); 2) Modeling the observed longitude-velocity (l − v) maps of CO (e.g., Bissantz et al., 2003; Rodriguez-Fernandez and Combes, 2008; Baba et al., 2010; Pettitt et al., 2014, 2015; Li et al., 2021). A detailed review about these two methods can be found in Xu et al. (2018b). As discussed in Xu et al. (2018b), although there have been many efforts, the spiral structure traced by molecular gas is still unclear, primarily due to the large uncertainties of distances. There has been noticeable progress in the past few years, as accurate distances were estimated for a large number of molecular clouds.
With the CO data of the Milky Way Imaging Scroll Painting survey1, the Gaia DR2 parallax, and G-band extinction (Brown et al., 2018), Yan et al. (2019) proposed a background-eliminated extinction-parallax method to estimate the distances of molecular clouds. The distance uncertainties of 11 clouds were ≲ 10%. With the same method, Yan et al. (2020) determined the distances for 28 local molecular clouds (d < 1.5 kpc, here d is the distance to the Sun) in the first Galactic quadrant. The distances for 76 molecular clouds were measured in the second Galactic quadrant by Yan et al. (2021). Based on a sample of over 32 million stars with color excesses and Gaia distances, Chen et al. (2019b) constructed new three-dimensional dust reddening maps of the Milky Way. With the maps and the sample of stars, Chen et al. (2020) identified 567 dust/molecular clouds, and estimated their distances by using a dust model fitting algorithm. The typical distance uncertainty was less than 5%. These clouds were within ∼ 3 kpc of the Sun. Based on the near-infrared photometry data from the Two Micron All Sky Survey and the Vista Variables in the Via Lactea Survey, Chen et al. (2019a) tracked the extinction of red clump stars versus distance profiles of the sightlines towards a sample of molecular clouds from Rice et al. (2016). Distances of 169 GMCs in the fourth Galactic quadrant were obtained.
We collected the data of GMCs from the above references. A total of 475 GMCs with masses > 104M⊙ were obtained. To reliably depict the local spiral structure, only the GMCs with distance uncertainties better than 15% were adopted. For the distant clouds, we also required that their distance uncertainties were < 0.5 kpc. In total, 427 GMCs with masses from 104M⊙ to 2.45 × 106M⊙ were left. Their projected distributions on the Galactic disk are shown in Figure 1A. According to the trigonometric parallax data of HMSFR masers (see Section 2.2), Reid et al. (2019) obtained an updated model of the Galaxy’s spiral arms, which is plotted in Figure 1 to make a comparison with the GMC distribution. It is shown that most of these GMCs were distributed within about 3 kpc of the Sun, in the Perseus, Local, and Sagittarius-Carina arms. Some distant GMCs in the fourth Galactic quadrant were probably associated with the Centaurus arm and the Norma arm. Along spiral arms, the distribution of GMCs presents some substructures, especially in the regions within about 2 kpc of the Sun. The accuracies of distances ensure that they are probably true features, but their nature (arm spurs or feathers) and properties have not been well studied.
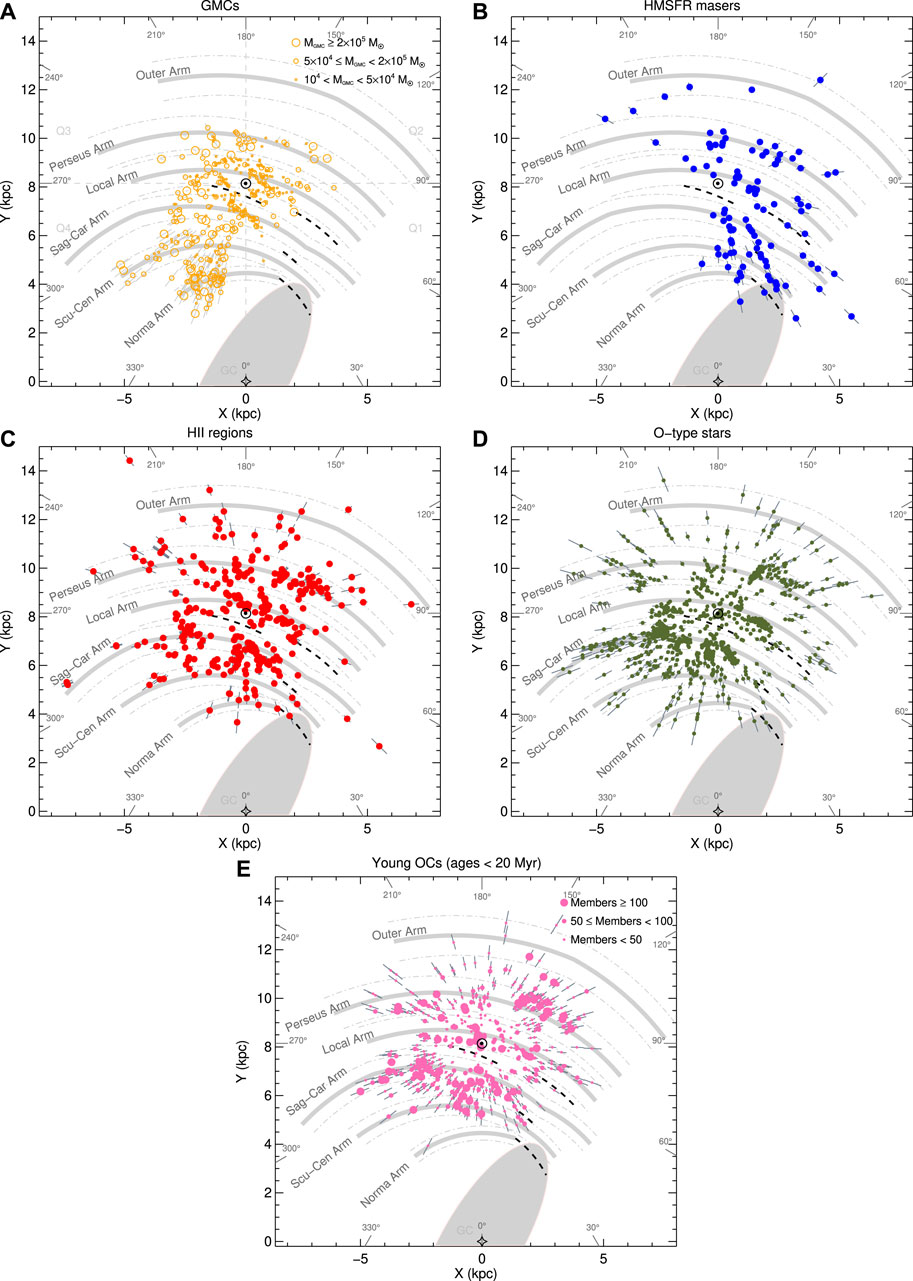
FIGURE 1. Distributions of (A) GMCs, (B) HMSFR masers, (C) HII regions, (D) O-type stars, and (E) young OCs in the solar neighborhood. All of the plotted objects have distance uncertainties better than 15% and <0.5 kpc as determined by the trigonometric or photometric method. The symbol sizes of GMCs are proportional to their masses. For HMSFR masers, HII regions, and O-type stars, an equal size of the dots is adopted. The symbol sizes of young OCs are proportional to the number of cluster member stars. The position uncertainty for each data point is shown by an underlying gray line segment. The thick gray curved lines indicate the best fitted spiral arm model given by Reid et al. (2019), the dotted lines denote the arm widths enclosing 90% of the HMSFR masers. The black dashed lines indicate the four spurs or spur-like structures proposed in literature (see Section 3.3). In each plot, the segments of spiral arms are labelled; the Sun is at (0.0, 8.15) kpc (Reid et al., 2019), while the Galactic center is at (0.0, 0.0) kpc; and the Galactic long bar is indicated by a shaded ellipse (Wegg et al., 2015).
2.2 High-Mass Star-forming Region Masers
The early stage of massive star formation is accompanied by the maser emission from molecular species such as OH, CH3OH, and H2O (Fish, 2007). The maser spots are compact and bright, and hence are optimal targets for radio interferometric observations. The trigonometric annual parallax measurement with Very Long Baseline Interferometry (VLBI) is the most accurate method for deriving the distances of astronomical objects. In 2006, a pioneer study on measuring the trigonometric parallax of molecular masers was made by Xu et al. (2006), who found a distance of W3(OH) in the Perseus arm to be 1.95 ± 0.04 kpc. This work opened a new era to accurately reveal the Galaxy’s spiral structure through VLBI measurements. Since then, nearly 200 HMSFRs have had measured trigonometric parallaxes (typical accuracy of about ± 0.02 mas) and proper motions, primarily by the Bar And Spiral Structure Legacy (BeSSeL) Survey using the VLBA (Reid et al., 2019) and the Japanese VLBI Exploration of Radio Astrometry (VERA) project (Hirota et al., 2020). Some sources were observed by the European VLBI Network and the Australian Long Baseline Array (e.g., Rygl et al., 2010; Krishnan et al., 2017). Based on the data of 199 HMSFRs, parameters of spiral arms in about one third of the Galactic disk were updated by Reid et al. (2019), which is adopted in Figure 1 to make a comparison with the data distribution.
To depict the local spiral arms with high confidence, only the HMSFRs (Reid et al., 2019; Hirota et al., 2020) with uncertainties of trigonometric distances better than 15% were kept as for GMCs. For the distant sources, we also required that their distance uncertainties were < 0.5 kpc. Then, 111 HMSFRs remained. Their distribution is given in Figure 1B. These HMSFRs are located in six arm segments, i.e., the Outer, Perseus, Local, Sagittarius, Scutum, and Norma arms. Some of the HMSFRs are probably related to spur-like structures in the inter-arm regions (Reid et al., 2019). A prominent one is the spur branching the Sagittarius arm and the Local arm near l ∼ 50◦ (see Figure 1), which was firstly identified by Xu et al. (2016).
As shown in Figure 1B, the HMSFRs with parallax measurements are distributed in the regions covering about one-third of the entire Galactic disk. There are a lack of observational data for many Galaxy areas, especially in the longitude range of ∼ 240◦—360◦. Other spiral tracers (e.g., GMCs, HII regions, massive OB stars, and young OCs) could be good complementary data, which can help us to better depict the properties of spiral arms in the solar neighborhood.
2.3 HII Regions
HII regions are the regions of ionized gas surrounding recently formed O or early B-type stars or star clusters. They have been widely detected in the Galactic disk, from the Galactic center (GC) region (Shahzamanian et al., 2019) to the far outer Galaxy (Galactocentric distances > 16 kpc, Anderson et al., 2015). As indicators of the early evolutionary stage of massive stars, HII regions have long been known as one of the primary tracers of spiral arms, and helped us to construct the commonly used picture of the Galaxy’s spiral structure (e.g., Georgelin and Georgelin, 1976; Caswell and Haynes, 1987; Russeil, 2003; Hou et al., 2009; Hou and Han, 2014). However, for the majority of known HII regions, only kinematic distances are available, which sometimes have large uncertainties. It is currently the major obstacle for using HII regions to accurately delineate the spiral arms. There are about 400 HII regions with measured spectra-photometric (Russeil, 2003; Moisés et al., 2011; Foster and Brunt, 2015) or trigonometric distances (e.g., Xu et al., 2006; Honma et al., 2012). They are distributed within about 5 kpc of the Sun (Hou and Han, 2014). The sample size and the accuracies of distances have yet to be improved.
In a recent work (Hou et al., 2021), we carried out a cross-match between the WISE HII regions (Anderson et al., 2014) and the known O or early B-type stars (Chen et al., 2019a; Xu et al., 2021). The ionizing stars of 315 HII regions were identified. The trigonometric parallaxes for these OB stars from Gaia Early Data release 3 (Gaia EDR3, Brown et al., 2021) were used to estimate the distances of HII regions. In combination with the HII regions with known spectra-photometric/trigonometric distances, we obtained a sample of 448 HII regions with accurately determined distances, i.e., distance uncertainties were better than 15% and less than 0.5 kpc. Their distribution in the Galactic disk resembled GMCs and HMSFR masers as presented in Figure 1C.
These HII regions are scattered primarily in the Perseus, Local, Sagittarius-Carina, and Scutum-Centaurus arms. The data distribution in the Local arm and Centaurus arm deviate from the modeled spiral arms given by Reid et al. (2019) in the third and fourth Galactic quadrants, where the HMSFRs with trigonometric measurements are largely absent. Hence, the extension of the Local arm and the position of the Centaurus arm given by Reid et al. (2019) need to be updated. In these arm segments, we also noticed that the HII regions are not uniformly distributed, but present some substructures. The Sagittarius-Carina arm is not continuous in the direction of l ∼315◦–340◦. A similar property is found for the Perseus arm in the longitude range of ∼150◦– 160◦. These properties are consistent with the features illustrated by GMCs (Figure 1A). Although the number of HII regions with accurate distances have largely increased, there is still a lack of accurate distances for many Galactic HII regions. From Gaia EDR3 or their future data release, it is expected to identify more ionizing stars and determine the distances of HII regions as accurately as possible.
2.4 O and Early B-Type Stars
The massive and bright O and early B-type (OB) stars are born in dense molecular clouds. Many of them are not randomly distributed, but concentrated in loose groups, named as “aggregates” (Morgan et al., 1953) or OB associations (de Zeeuw et al., 1999; Wright, 2020). In the early 1950s, substantial progress on tracing the arm segments in the solar neighborhood was first made by Morgan et al. (1952) and Morgan et al. (1953). Three segments of spiral arms (i.e., the Sagittarius, Local, and Perseus arms) appeared in the distribution of their 27 aggregates of O-A stars and additional eight distant stars. After that, some follow-up studies identified more OB stars or OB associations, and determined their spectra-photometric distances (e.g., Walborn, 1971; Miller, 1972; Stothers and Frogel, 1974; Reed and Reed, 2000). However, the picture of Morgan did not expand significantly. With the Hipparcos catalog, de Zeeuw et al. (1999) estimated the trigonometric distances for some OB associations within ∼1 kpc from the Sun, primarily in the Local arm. At present, the known OB associations are limited within about ∼2 kpc from the Sun (Wright, 2020). To extend the nearby arm segments traced by OB stars or OB associations, higher accuracies of astrometry measurements than the Hipparcos (typical parallax error ∼1 mas, de Zeeuw et al., 1999) are needed.
The Gaia satellite (Prusti et al., 2016), launched in 2013, will ultimately achieve parallax accuracy comparable to that of VLBI for approximately 109 stars. Many OB stars with accurate distances can be derived from Gaia. By taking advantage of Gaia Data release 2, Xu et al. (2018a) depicted the spiral structure within ∼3 kpc of the Sun. About 2,800 O-B2 stars with formal parallax uncertainties better than 10% were extracted from the Catalog of Galactic OB stars (Reed, 2003). The spiral structure demonstrated by the Gaia OB stars agrees well with that illustrated by the VLBI HMSFR masers. These OB stars also extend the arm segments traced by HMSFR masers into the fourth Galactic quadrant. Chen et al. (2019a) identified 6,858 candidates of O- and early B-type stars. Together with the known spectroscopically confirmed O-B2 stars from literature, a sample of 14,880 OB stars/candidates with Gaia DR2 parallax uncertainties better than 20% was obtained, and used to delineate the arm segments in the solar neighborhood. Recently, Gaia published its Early Data release 3, the parallax accuracies have been improved significantly, to be 0.02–0.07 mas for G < 17. In a recent work, Xu et al. (2021) compiled the largest sample of spectroscopically confirmed O-B2 stars (Skiff, 2014) available to date with astrometric measurements of Gaia EDR3, including 14,414 O-B2 stars. In total, 9,750 of them have parallax uncertainties better than 10%. With this sample, the spiral structure within ∼5 kpc of the Sun are delineated in detail.
In this work, the sample consisting of about 1,090 O-type stars given by Xu et al. (2021) is adopted. Their distribution in the Galactic disk is shown in Figure 1D. As discussed in Xu et al. (2021), the distribution of O-type stars in spiral arms are clumped. The Sagittarius-Carina arm traced by O-type stars seems to be not continuous in the direction of l ∼315◦–340◦. A gap of O-type stars in the range of l ∼150◦–160◦ in the Perseus arm is also noticed. These properties are consistent with the results shown by using GMCs and HII regions.
2.5 Young Open Clusters
An open cluster is a group of stars that formed in a giant molecular cloud. In comparison to individual stars, it is possible to estimate more accurate values of distance, proper motions, and radial velocity for an OC, as it has many member stars. In our Galaxy, star formation occurs mainly in spiral arms. Hence, the majority of young OCs are believed to be born in spiral arms, and too young to migrate far from their birth locations. It is accepted that young OCs (e.g., < 20 Myr) can be used as good tracers for the nearby spiral arm segments. In comparison, older OCs have more scattered distribution.
Becker (1963, 1964) first used 156 OCs to study the spiral structure in the solar neighborhood. They suggested that the distribution of clusters with the earliest spectral type between O and B2 follows three spiral arm segments. In comparison, the distribution of the clusters with the earliest spectral type between B3 and F does not present arm-like structures and seems to be random. The picture was extended by Becker and Fenkart (1970) and Fenkart and Binggeli (1979). Meanwhile, a different point of view was raised by Janes and Adler (1982) and Lynga (1982) with a large sample of about 400 open clusters. They suggested that the observed nonuniform distribution of OCs is affected by the interstellar obscuration, and dominated by the locations of dust clouds rather than by the spiral structure. Janes et al. (1988) mentioned that the young clusters define three complexes, but their association to a spiral structure is not obvious. After that, the number of Galactic open clusters gradually increased (e.g., see Mermilliod, 1995; Dias et al., 2002; Kharchenko et al., 2013; Schmeja et al., 2014; Scholz et al., 2015). Meanwhile, the spiral structure of the Milky Way was better uncovered by multi-wavelength observations (e.g., Georgelin and Georgelin, 1976; Caswell and Haynes, 1987; Dame et al., 2001; Russeil, 2003), especially in the radio band, where the dust obscuration has neglectable influence on the results. There has been a general consensus that the nonuniform distribution of nearby OCs is related to spiral arms (e.g., Dias & Le´pine, 2005; Carraro et al., 2005; Moitinho et al., 2006; Vázquez et al., 2008; Moitinho, 2010; Camargo et al., 2013; Bobylev and Bajkova, 2014; Dias et al., 2019).
Before the data release of Gaia, there were more than 3,000 known OCs (e.g., see, Dias et al., 2002; Kharchenko et al., 2013; Dias et al., 2014; Schmeja et al., 2014; Scholz et al., 2015). Many of them have determined mean proper motions and membership probabilities (Sampedro et al., 2017; Dias et al., 2018). Since the publication of Gaia DR2 (Brown et al., 2018), on the one hand, the parameters of known OCs have been updated (Cantat-Gaudin et al., 2018; Soubiran et al., 2018; Bossini et al., 2019; Monteiro and Dias, 2019; Cantat-Gaudin and Anders, 2020; Cantat-Gaudin et al., 2020; Dias et al., 2021; Tarricq et al., 2021). On the other hand, a large number of new OCs and candidates have been identified (e.g., see Castro-Ginard et al., 2018; Cantat-Gaudin et al., 2019; Castro-Ginard et al., 2019; Sim et al., 2019; Liu and Pang, 2019; Hao et al., 2020; Castro-Ginard et al., 2020; Ferreira et al., 2020; He et al., 2021a; Hunt and Reffert, 2021; Ferreira et al., 2021). With OCs, the nearby spiral arms were studied by Cantat-Gaudin et al. (2018), Dias et al. (2019), Cantat-Gaudin et al. (2020), Tarricq et al. (2021), and Ferreira et al. (2021) in the past few years. Especially, Monteiro et al. (2021) studied the spiral arms traced by young OCs with the updated OC catalogue of Dias et al. (2021), and determined the spiral pattern rotation speed of the Galaxy, the corotation radius, and the statistic properties of OC parameters. Poggio et al. (2021) studied the spiral structure in the solar neighborhood with samples of young upper main sequence stars, classical cepheids, and open clusters. The open clusters used by Poggio et al. (2021) is from Cantat-Gaudin et al. (2020). Hao et al. (2021) compiled a catalogue of more than 3,700 OCs from the references above, and re-calculated the parameters (parallaxes, mean proper motions, radial velocities) based on the latest Gaia EDR3 (Brown et al., 2021). The ages of these OCs were either collected from references or estimated by their analysis.
In this work, we adopted the OC sample provided by Hao et al. (2021), in which the OC parameters are based on the latest Gaia EDR3. There are 627 young OCs (ages < 20 Myr) in their catalogue. The distribution of young OCs in the Galactic disk is shown in Figure 1E. The distribution of young OCs resembles that of GMCs, HMSFR masers, HII regions, and O-type stars. When the OCs are weighted with their number of member stars, one can notice that the OCs with more member stars are more inclined to be located in spiral arms.
2.6 Evolved Stars
As the velocity dispersion of gas is smaller than that of the old stars, the gas response to any perturbations in the stellar disk is highly amplified (Dobbs and Baba, 2014), making the gas arms easier to identify than the stellar arms traced by old and evolved stars. For our Milky Way, unlike the gas arms well depicted by GMCs, HMSFR masers, HII regions, young OB stars, or young OCs, the spiral arms traced by old stars are still not clear. The properties of stellar arms are important to better constrain the formation and evolution of gas arms.
It is commonly suggested that the spiral structure traced by old stars is dominated by two major spiral arms (the Scutum-Centaurus arm and Perseus arm) based on analyzing the arm tangencies (Churchwell et al., 2009). In observations, spiral arm tangencies are indicated by the local maxima in the integrated number count of old stars or in the integrated emission in the near-infrared and/or far-infrared bands against the Galactic longitude, and have only been clearly identified for the Scutum-Centaurus arm (Drimmel, 2000; Drimmel and Spergel, 2001; Churchwell et al., 2009). Clear indications of tangencies corresponding to the stellar Sagittarius-Carina arm and Norma arm have not been found from observational data. As the Sun is located inside the Perseus arm, the observed line of sight does not penetrate its tangency. The method of analyzing tangency points cannot be applied to this arm. However, the Perseus arm is also suggested to be a major stellar arm based on symmetry. Indeed, many grand-design spiral galaxies with two well-defined spiral arms are observed in the Universe (e.g., Willett et al., 2013). In comparison, arm tangencies for the gaseous Sagittarius-Carina arm, Scutum-Centaurus arm, and Norma arm could be identified from the wealth of survey data of radio recombination lines, HII regions, CO lines, dense molecular clumps, and HI 21-cm line, etc. (see Hou and Han, 2015).
By taking advantage of the data quality and large sky coverage of Gaia, it is possible to map the nearby spiral structure traced by evolved stars. Miyachi et al. (2019) studied the surface density distribution of stars aged ∼1 Gyr, and identified a marginal arm-like overdensity in the longitude range of 90◦⩽l⩽190◦. The overdensity of stars is close to the Local arm defined by HMSFRs. By analyzing the Gaia DR2 data, Kounkel and Covey (2019) identified a number of clusters, associations, and moving groups distributed within ∼1 kpc of the Sun, many of them appear to be filamentary or string-like. The youngest strings (< 100 Myr) are orthogonal to the Local arm. The older ones are suggested to be the remnants of several other arm-like structures which cannot be traced by dust and gas any more. With a new method of analyzing the six-dimensional phase-space data of Gaia DR2 sources, Khoperskov et al. (2020) identified six prominent stellar density structures in the guiding coordinate space2, corresponding to a physical spatial coverage of about 5 kpc from the Sun. Four of these structures were suggested to correspond to the Scutum-Centaurus, Sagittarius, Local, and Perseus arms, while the remaining two may be associated with the main resonances of the Milky Way bar and the outer Lindblad resonance beyond the solar circle. While Hunt et al. (2020) presented a different point of view. They suggested that the stellar density structures identified by Khoperskov et al. (2020) are known kinematic moving groups, rather than a coherent structure in physical space such as spiral arms. In addition, it has also been shown that very different bar and spiral arm models can be tuned to explain the observed features of Gaia data (e.g., Hunt et al., 2019; Monari et al., 2019; Eilers et al., 2020; Khoperskov et al., 2020; Chiba et al., 2021; Trick et al., 2021), making the situation more difficult to handle.
In the past few years, progress in mapping the stellar arms in the solar neighborhood has been made, but there are no conclusive observational results. As the properties of nearby stellar arms are not clear, we do not incorporate them into our analysis/discussions in the following. But we emphasize that determination of the properties of stellar arms in our Galaxy definitely deserves more attention.
3 Properties of Spiral Structure in the Solar Neighborhood
In order to better reveal the properties of spiral structure in the solar neighborhood, we combine the data of good tracers of gas arms, i.e., GMCs, HMSFR masers, HII regions, O-type stars, and young OCs. It is probably the most wide spread dataset of spiral tracers with accurate distances available to date. Especially as the data distributions of different types of tracers are complementary to each other in the sky coverage. As shown in Figure 1 and Figure 2, although some substructures seem to exist in spiral arms or inter-arm regions, the distributions of these objects in general follow the dominant spiral arms identified by previous works (e.g., Georgelin and Georgelin, 1976; Russeil, 2003; Hou and Han, 2014; Reid et al., 2019). Five segments of spiral arms are delineated by the combined dataset. They are related to the Perseus, Local, Sagittarius-Carina, Scutum-Centaurus, and Norma arms from the outer Galaxy to the GC direction. Additionally, there are about 30 sources possibly scattered in the Outer arm defined by HMSFR masers. However, the aggregation of sources is not obvious. We will not discuss the Outer arm in this work.
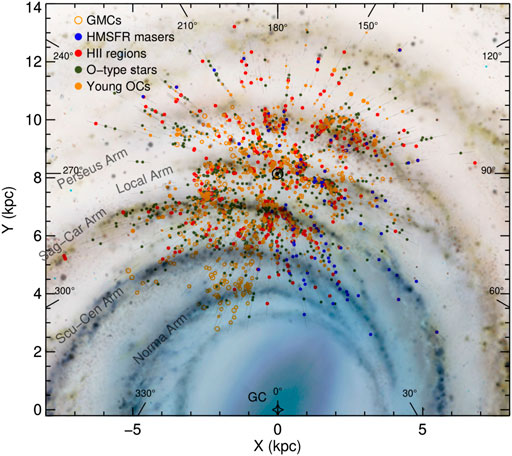
FIGURE 2. Distributions of the combined dataset of GMCs (yellow circles), HMSFR masers (blue dots), HII regions (red dots), O-type stars (green dots), and young OCs (yellow dots) are overlaid on a new concept map of the Milky Way, which is credited by: Xing-Wu Zheng and Mark Reid BeSSeL/NJU/CFA based on the spiral arm model of Reid et al. (2019).
3.1 Fitting Model to Tracer Distributions
From the combined dataset of spiral tracers (Figure 1 and Figure 2), some noticeable features are the deviations of tracer distributions from the modeled spiral arms given by Reid et al. (2019) in the third and fourth Galactic quadrants, where the HMSFRs with trigonometric measurements are largely absent. For instance, many O-type stars and young OCs in the longitude range of l ∼ 210◦–260◦ deviate from the modeled Perseus arm towards the GC direction. A similar feature is found for the Local arm in the longitude range of l ∼ 260◦–280◦. In the fourth quadrant, many GMCs, HII regions, and O-type stars near l ∼ 300◦–350◦ deviate slightly from the modeled Centaurus arm of Reid et al. (2019) towards the anti-GC direction. Hence, with the combined dataset, it would be helpful to update the parameters of spiral arms in the solar neighborhood.
The spiral arms observed in spiral galaxies (e.g., Seigar and James, 1998; Yu et al., 2018) and those predicted by the density-wave theory (e.g., Lin and Shu, 1964; Shu, 2016) are approximately in a logarithmic form, which is characterized by a constant pitch angle. A simple and pure logarithmic form of spiral arm was commonly adopted in previous works about the Galaxy’s spiral structure (e.g., Russeil, 2003; Vallée, 2008; Hou et al., 2009; Reid et al., 2009). On the other hand, it is found that the spiral arms in galaxies do not follow logarithmic spirals perfectly, but seem to be kinked in nature (e.g., Kennicutt, 1981; Kendall et al., 2011; Honig and Reid, 2015; Díaz-García et al., 2019, also see the Whirlpool galaxy M 51). The observed spiral arms can be better described by segments of logarithmic form with different pitch angles. In theories, tidal interactions can result in noticeable kinks along spiral arms in a galaxy (Dobbs and Baba, 2014). In simulation, D’Onghia et al. (2013) found that the perturbers can produce segments, and these segments are joined at kinks to form a spiral arm. For our Milky Way Galaxy, signs of kinked spiral arms were also noticed (e.g., Taylor and Cordes, 1993; Hou and Han, 2014), as some of the spiral arms cannot be well fitted by pure logarithmic spirals (e.g., the Sagittarius-Carina arm). With pure logarithmic spirals, it is also difficult to reproduce the observed l − v maps of CO and HI for the outer Milky Way (e.g., Pettitt et al., 2014). Following Reid et al. (2019), in this work we adopted a form of kinked logarithmic spiral to fit an arm, which does not necessarily have a constant pitch angle.
We allowed one or two “kinks” in an arm, which means that two or more segments with different pitch angles are used to describe a single spiral arm. For the ith spiral arm, the form is described as:
here, R is the Galactocentric radius at a Galactocentric azimuth angle β. Following Reid et al. (2019), β is defined as 0◦ toward the Sun and increases in the direction of Galactic rotation. Ri,kink and βi,kink are the corresponding values of R and β at the “kink” position for the ith arm. ψi is the pitch angle, which may have an abrupt change at the “kink” position. To be consistent with Reid et al. (2019) and compare the results with their models, a Markov chain Monte Carlo (MCMC) approach was adopted in this work to estimate the arm parameters. The best-fitted arm parameters are listed in Table 1, and the model is shown in Figure 3. We also compared it with the observed l–υ diagram of CO (Dame et al., 2001) as given in Figure 4. In the fitting with the combined dataset, an equal weight is adopted, although the sky coverage and sample size for different types of tracers are not the same. Different treatments of weighting parameters were tested, e.g., scaling according to the sample size of different types of tracers, but the differences of the fitted arm positions were found to be small.
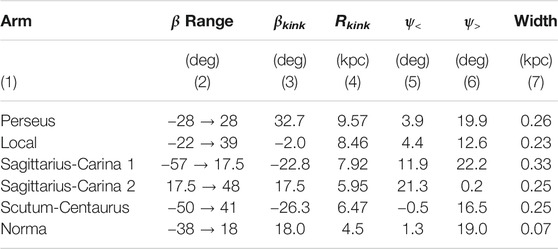
TABLE 1. Parameters of the best-fitted model of spiral arms (see Equation 1) with the combined dataset of GMCs, HMSFR masers, HII regions, O-type stars, and young OCs. For the ith spiral arm, β range in column (2) gives the range of the arm segment as shown in Figure 3, β is the Galactocentric azimuth angle, which is defined as 0◦ toward the Sun and increases in the Galactic rotation direction. βkink and Rkink in columns (3) and (4) are the corresponding values of β and R at the “kink” position, here R is the Galactocentric radius. The pitch angle is ψ< for β < βkink, and ψ> for β > βkink. The fitted arm width is listed in column (7). The Sagittarius-Carina arm consists of three segments, hence divided into two parts and listed below separately.
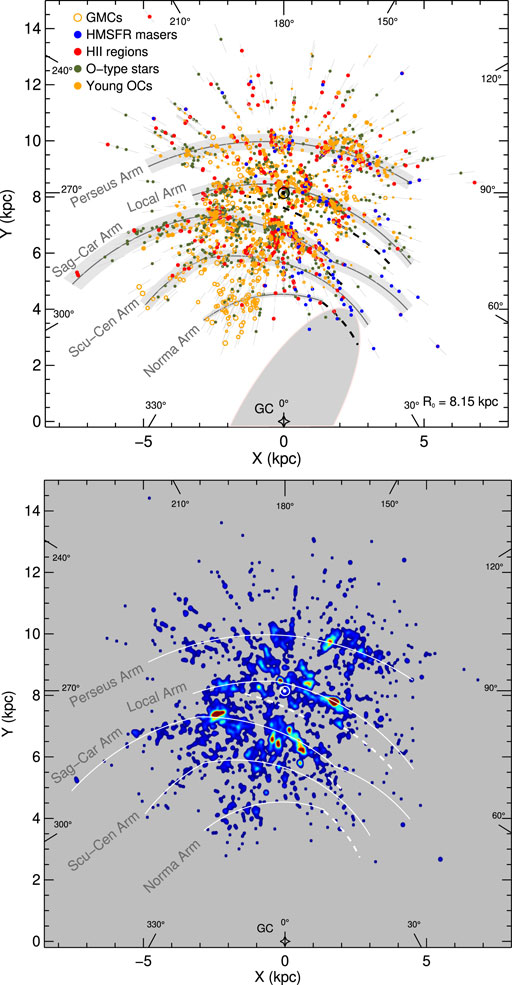
FIGURE 3. Upper: Distributions of the combined dataset of GMCs, HMSFR masers, HII regions, O-type stars, and young OCs in the Galactic disk. For all the plotted sources, their distance uncertainties are better than 15% and smaller than 0.5 kpc. The positional uncertainty for each data point is shown by an underlying gray line segment. The curved solid lines indicate the best fitted spiral arm model given by this work (Table 1). The shaded areas around spiral arms denote the fitted arm widths. The four dashed lines are the spurs or spur-like structures proposed in literature. Lower: Similar to the Upper panel, but a “density” distribution is calculated according to the distribution of the sources.
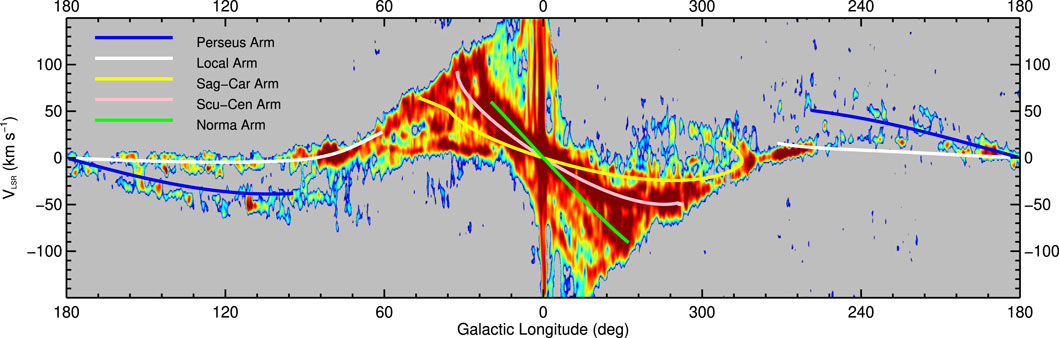
FIGURE 4. The best fitted spiral arm model give by this work (Table 1) is overlaid on the l − v diagram of 12CO (1–0) of Dame et al. (2001). The spiral arm segments are indicated by different colors. To convert the position of spiral arms into the l − v diagram, the fitted “universal” form of the Galaxy rotation curve given by Reid et al. (2019) was adopted. The distance of the Sun to the GC, R0 is taken as 8.15 kpc. The circular orbital speed at the Sun Θ0 is 236 km s−1 (Reid et al., 2019).
In comparison with the best-fitted model of Reid et al. (2019), the extension of the Perseus arm obtained in this work spirals slightly inward in the third Galactic quadrant. In this work, the Local arm was best fit with two segments with different pitch angles, and spiraled inward to the inner Galaxy regions in the third to fourth Galactic quadrants to match the observational data. The Centaurus arm in the fourth quadrant was also fit with two segments in this work. Their positions were slightly different from those of Reid et al. (2019) near l ∼ 300◦–350◦ but matched the distributions of the collected GMCs, HII regions, O-type stars, and young OCs. For the Sagittarius-Carina arm and the Norma arm, our fitted arm positions were, in general, consistent with that of Reid et al. (2019).
To evaluate that how well the different types of tracers fit the spiral arms, we calculated the percentages of tracers falling into our best-fitted spiral arms. The arm widths given in Table 1 were adopted to denote the spiral arm regions. In the Norma arm, the known HMSFR masers, HII regions, O-type stars, and young OCs are still rare. This arm was not used in the calculation. Additionally, the Outer arm was also omitted. The percentages of tracers falling into spiral arms were estimated to be 44%, 53%, 58%, 65%, and 53% for the GMCs, HMSFR masers, HII regions, O-type stars, and young OCs, respectively. It seems that the GMCs are less confined to the spiral arms than the other types of tracers. In comparison, if the objects are randomly distributed in the solar neighborhood, about 33% of them are expected to be in the spiral arms.
3.2 Spiral Arms in the Solar Neighborhood
By taking advantage of the combined dataset of different types of tracers, segments of spiral arms in the solar neighborhood have been delineated and fitted. Now we discuss their properties in detail.
Perseus arm: The Perseus arm is a dominant arm in our Galaxy as indicated by high-mass star-formation activity (HMSFR masers, HII regions, O-type stars, young OCs), molecular gas, and HI gas (e.g., Morgan et al., 1953; Caswell and Haynes, 1987; Russeil, 2003; Hou and Han, 2014; Reid et al., 2019). Additionally, the Perseus arm has been suggested as one of the two dominant stellar arms of the Milky Way (Drimmel, 2000; Drimmel and Spergel, 2001; Churchwell et al., 2009). As shown in Figure 3, different types of spiral tracers (GMCs, HMSFR masers, HII regions, O-type stars, and young OCs) are mixed together. Their distributions are, in general, consistent with each other. The depicted Perseus arm is as long as ∼12 kpc, starts near (X,Y) = (4.5, 8) kpc, and extends to the third Galactic quadrant near (X,Y) = (−6, 9) kpc. In this arm, the spiral tracers are not evenly distributed but tend to cluster. There are two obvious aggregation areas of sources, one is in the longitude range of l ∼ 100◦–150◦, the other is in l ∼ 170◦–190◦, indicating the active star-formation areas in the Perseus arm. Outside these two regions, some sites of GMCs or star-formation are scattered and interspersed with regions showing low star-formation activity and/or low number density of GMCs. Outside the segment shown in Figure 3, the extension of the Perseus arm to the first or fourth Galactic quadrant could be explored in the l − υ diagram of CO and HI survey data, but have not been accurately depicted (Xu et al., 2018b). Reid et al. (2019) has suggested that the Perseus arm may be not a dominant arm as measured by high-mass star-formation activity over most of its length. More spiral tracers with accurate distances are needed in order to reliably trace this arm to distant Galaxy regions.
Local arm: The Local arm was thought to be a “spur” or secondary spiral feature for a long time (e.g., Georgelin and Georgelin, 1976; Amaral and Lepine, 1997; Russeil, 2003), until the density of HMSFRs in the Local arm was found to be comparable to that of the Sagittarius arm and Perseus arm (Xu et al., 2013, 2016). The Local arm traced by HMSFR masers stretches for > 6 kpc, which is larger and more prominent than previously thought. Hence, it is suggested to be a dominant arm segment. As shown in Figure 3, there are a large number of spiral tracers (GMCs, HMSFR masers, HII regions, O-type stars, and young OCs) located in this arm, which present complex substructures. There are several areas where sources are accumulated. One is near (X,Y) = (1.5, 7.7) kpc in the first Galactic quadrant, another is near (X,Y) = (−2, 8.2) kpc in the fourth quadrant. A filament-like structure appears in the region from (X,Y) = (2, 7.5) kpc to (X,Y) = (0.8, 9) kpc, and spirals outward toward the anti-GC direction with respect to the fitted arm center. Interestingly, a substructure near l ∼ 100◦–150◦ and with d ∼ 0.6 kpc is indicated by many GMCs, but without associated HMSFR masers, HII regions, O-type stars, or young OCs, at least shown by the collected dataset. For the majority of sources in this arm, their distance uncertainties are less than 10%, hence, these substructures are believed to be true features. In general, the depicted Local arm could be as long as ∼9 kpc, starts near (X,Y) = (4.5, 6) kpc, and extends to the third and even fourth quadrant near (X,Y) = (−3, 8) kpc. Outside the delineated segment, the extension of the Local arm is still not clear. The Local arm seems to gradually spiral inward in the fourth Galactic quadrant, becoming very close to the Carina arm. It is indicating that the Local arm is possibly an arm branch located between the Perseus arm and the Sagittarius-Carina arm. More observational data are needed to uncover its nature.
Sagittarius-Carina arm: This arm can be clearly traced by GMCs (Grabelsky et al., 1988) and massive star-formation regions (Russeil, 2003; Urquhart et al., 2014; Hou and Han, 2014). There are a large number of sources in this arm. The Sagittarius-Carina arm in Figure 3 starts near (X,Y) = (4.5, 4) kpc, extends to the fourth quadrant near (X,Y) = (−7, 5) kpc, as long as ∼19 kpc. It is found that this arm cannot be well fitted by a single pitch angle model, especially in the longitude range of 20◦–40◦ (e.g., Taylor and Cordes, 1993; Russeil, 2003; Hou and Han, 2014; Reid et al., 2019). Three major aggregation areas of sources are noticed. One is near l ∼ 30◦, showing an elongated structure, which is probably a true feature as it is not only traced by HMSFR masers, but also by HII regions, O-type stars, or young OCs. The other two areas are near l ∼ 340◦–360◦ and close to the tangent region of the Carina arm (l ∼ 280◦–290◦). The distribution of sources in this arm is very consistent with the model given by Reid et al. (2019). Outside the long segment traced in Figure 3, the extension of the Sagittarius-Carina arm could be well delineated by the distribution of GMCs or HII regions with kinematic distances, or indicated by the features shown in the l − υ diagrams of CO and HI.
Scutum-Centaurus arm: Similar to the Sagittarius-Carina arm, the Scutum-Centaurus arm has also been traced by many GMCs and massive star-formation regions. In addition, it is also suggested to be one of the two dominant stellar spiral arms of the Milky Way, as the Centaurus arm tangent was clearly shown by the evolved stars surveyed by Spitzer (Drimmel, 2000; Drimmel and Spergel, 2001; Churchwell et al., 2009). The collected GMCs, HII regions, O-type stars, and young OCs enrich the sample of spiral tracers in this arm, especially in the fourth Galactic quadrant. As shown in Figure 3, the spiral tracers in this arm seem to be more evenly distributed than that of the Perseus arm, Local arm, and Sagittarius-Carina arm. The traced segment of the Scutum-Centaurus arm starts near (X,Y) = (2.5, 4) kpc, and extends to the fourth Galactic quadrant near (X,Y) = (−5, 4.5) kpc, as long as ∼8 kpc.
Norma arm: This arm is distant (≳ 4 kpc) from the Sun, and most likely starts near the near end of the Galactic bar. Previously, the Norma arm has not been clearly traced by GMCs or massive star-formation regions. Mainly because the majority of spiral tracers possibly associated with this arm only have kinematic distances. With the collected dataset, it seems that only a segment of the Norma arm can be roughly traced by GMCs in the fourth Galactic quadrant. The number of HMSFR masers, HII regions, O-type stars, or young OCs related to this arm is still very limited.
3.3 Substructures in the Spiral Arms or Inter-arm Regions
Besides the extended structure of major spiral arms which probably wrap fully around the Milky Way, substructures named as branches, spurs, and features are often observed in spiral galaxies (Elmegreen, 1980; Dobbs and Bonnell, 2006). As discussed in La Vigne et al. (2006) and Dobbs and Baba (2014), there are no formal definitions of these different types of substructures in observations and/or numerical simulations. Different definitions have been adopted (Chakrabarti et al., 2003; Dobbs and Bonnell, 2006). The initial definitions based on observations are as follows: 1) Arm branches are in general the structures located between two major spiral arms, and/or where one arm bifurcates into two; branches may extend from one arm to another (Elmegreen, 1980; La Vigne et al., 2006; Dobbs and Baba, 2014). 2) Spurs are shorter features than branches, and are indicated by strings of star formation sites in the inter-arm regions. They jut out from spiral arms into the inter-arm regions at larger pitch angles than the arm itself (Weaver, 1970; Elmegreen, 1980). Two or more spurs are commonly found to be close or parallel to one another. 3) Feathers are also short features, but are indicated by thin dust lanes or extinction features that cut across spiral arms and have large pitch angles. Outside the luminous arms, these extinction features become mostly undetectable (Lynds, 1970). Arm branches, spurs, and features typically extend away from the trailing side of spiral arms (e.g., M 51, Dobbs and Baba, 2014).
As shown in Figure 3, the objects in spiral arms are not uniformly distributed, resulting in the patchy and/or bifurcate appearance of spiral arms. Additionally, about 40% of the collected sources that are distributed in the inter-arm regions, also present some structural features. These substructures may be related to arm branches, spurs, and/or feathers as found in some nearby face-on spiral galaxies (e.g., M 51, M 101, Dobbs and Baba, 2014; Xu et al., 2018b). Our knowledge about these substructures in our Galaxy is very limited.
In our Galaxy, several spurs and/or spur-like structures have been classified from observations. In the direction of l ∼ 90◦–210◦, a structural feature named the Orion spur (e.g., Figure 1 of Humphreys, 1970; Schmidt-Kaler, 1975; Kolesnik and Vedenicheva, 1979) or Cepheus spur (Pantaleoni González et al., 2021) was discussed. This feature is suggested to be located between the Local arm and the Perseus arm, which may even extend to the first Galactic quadrant (Aasi et al., 2016). However, the name of the Orion spur was also used to indicate the Local arm in some literature (Amaral and Lepine, 1997; Carraro, 2014; Eker et al., 2014), which brings up a question: if the Orion spur discussed in the early days (e.g., Humphreys, 1970) exists or not? It will be helpful to reinvestigate this question with modern observational data. Recently, Xu et al. (2016) identified a spur near the direction of l ∼ 50◦ traced by five HMSFRs with VLBI parallax measurements, bridging the Local arm to the Sagittarius arm and having a pitch angle of ∼18◦ (∼13◦ given by a recent analysis of Reid et al., 2019). The existence of this spur is also supported by the CO features shown in the l–υ diagram. By analyzing the distribution and peculiar motions of HMSFR G352.630–1.067 and five O-type stars, a possible spur-like structure is proposed by Chen X. et al. (2019), which extends outward from the Sagittarius arm. Reid et al. (2019) mentioned that the Norma arm in the first Galactic quadrant displays a spur-like structure, which starts at (X, Y) = (3, 2) kpc near the end of the Galactic bar and extends to about (X, Y) = (2, 5) kpc at a Galactic azimuth angle of ∼ 18◦. This structure has a large pitch angle of ∼ 20◦. In addition, a spur-like structure bridging the Scutum arm and the Sagittarius arm is also mentioned in Reid et al. (2019), which is indicated by the distribution and proper motions of six HMSFRs and has a large pitch angle of ∼ 20◦. These proposed spurs or spur-like structures are plotted in Figure 1 and Figure 3. It seems that some GMCs, HII regions, O-type stars, and young OCs are coincident with these structures in positions.
Except the Orion spur mentioned in some early literature, the known spurs or spur-like structures identified in the past few years are nearly all based on the astrometric data of HMSFR masers. In comparison to HMSFR masers, the GMCs, HII regions, and especially O-type stars and young OCs with accurate distances have covered a much wider Galactic range. Hence, it is expected that more substructures could be identified. To identify the substructures in the inter-arm regions or spiral arms, radial velocities and/or proper motions for the sources would be helpful, which are still not available for many of them. Additionally, the properties of these substructures will help us to better understand the formation mechanisms of the Galaxy’s spiral structure. As the formation of these features is different for different spiral arm models (Dobbs and Baba, 2014).
3.4 Formation Mechanisms of the Galaxy’s Spiral Structure
Besides accurately mapping the spiral structure, understanding its formation mechanism is another difficult issue. Different mechanisms have been proposed, e.g., the quasi-stationary density wave theory (Lin and Shu, 1964, 1966), localized instabilities, perturbations, or noise-induced kinematic spirals (Sellwood and Carlberg, 1984), dynamically tidal interactions (Toomre and Toomre, 1972), or a combination of some of them (Dobbs and Baba, 2014). Although many efforts have been dedicated to elaborate plausible hypotheses concerning the origin of the dominant spiral arms of the Galaxy, it is still not conclusive for now. One way is to analyze the kinematic properties of stars in the vicinity of the Sun (e.g., Williams et al., 2013; Faure et al., 2014; Liu et al., 2017; Kawata et al., 2018). However, it has been shown that very different bar and spiral arm models can be tuned to look like the local Gaia data (Hunt et al., 2019), or convincingly explain all observed features at once (e.g., Monari et al., 2019; Eilers et al., 2020; Khoperskov et al., 2020; Chiba et al., 2021; Trick et al., 2021). The other method is comparing the relative positions of gas arms and stellar arms (e.g., Roberts, 1969; Dobbs and Pringle, 2010; Dobbs and Baba, 2014; Hou and Han, 2015; Monguió et al., 2015; Shu, 2016; He et al., 2021b), which can be used to verify the predictions of different theories. Observational evidence for the spatial offsets between the gas arms and stellar arms have been noticed for the tangent regions (e.g., Hou and Han, 2015). However, for other regions in the Galactic disk, it is not clear whether the systematic spatial offsets or age pattern exist or not (Monguió et al., 2015; Vallée, 2018; He et al., 2021b). More tests based on observations are needed.
In addition, the properties of the Local arm make the situation more complex. Its existence induces some challenge to the density wave theory applied to our Galaxy (Xu et al., 2013, 2016). Before 2017, no specific mechanism for the origin of the Local arm had been proposed. Lépine et al. (2017) first interpreted the Local arm as an outcome of the spiral corotation resonance, which traps arm tracers and the Sun inside it (also see Michtchenko et al., 2018). Their modeled corotation zone looks consistent with the banana-like structure of the Local arm shown by the distributions of GMCs, HII regions, O-type Stars, and young OCs in Figure 3. In the context of other mechanisms, e.g., localized instabilities, perturbations, or noise-induced kinematic spirals, the properties of the Local arm may be easily interpreted. The Milky Way has been suggested to be quite different from a pure grand design spiral, but probably resembles a multi-armed galaxy M 101, due to the existence of the Local arm and the many possible spurs noticed from observational data (Xu et al., 2018b). Typically, the localized instabilities are associated with flocculent or multi-armed galaxies (Dobbs and Baba, 2014).
4 Conclusions and Discussions
In this work, the spiral structure in the solar neighborhood is discussed based on the largest dataset available to date, which consists of different types of good spiral tracers. They are GMCs, HMSFR masers, HII regions, O-type stars, and young OCs. All the collected data have accurate distances with uncertainties < 15% and < 0.5 kpc. With the dataset, we update the parameters of spiral arm segments in the solar neighborhood, and discuss their properties. The spiral structure traced by GMCs, HMSFR masers, HII regions, O-type stars, and young OCs are, in general, consistent with each other. Five segments of dominant spiral arms in the solar neighborhood are depicted, they are the Perseus, Local, Sagittarius-Carina, Scutum-Centaurus, and Norma arms. However, the extensions of these arm segments to distant Galaxy regions have not been reliably traced. In the spiral arms and inter-arm regions, the distributions of spiral tracers present complex substructures, which are probably true features as the distance uncertainties of the tracers are small. At least five spurs or spur-like features have been identified in the literature by taking advantage of the astrometric data of HMSFR masers, but more substructures remain to be uncovered with the updated dataset of different types of good spiral tracers. In comparison to the gas arms traced by GMCs and star-formation activity, the properties of stellar arms indicated by evolved stars are still inconclusive.
There has been significant progress in understanding the Galaxy’s spiral structure in the past few years, which is heavily dependent on the developments of astrometric observations by the VLBI in the radio band and the Gaia satellite in the optical band. The VLBI observations have the advantage of measuring the spiral tracers in distant Galaxy regions with high accuracies (as high as 0.006 mas, typically about ±0.2 mas, Reid et al., 2019), and almost not affected by dust extinction. BeSSeL is planned to extend to the southern sky (Reid et al., 2019), which will provide parallax and proper motion measurements for many HMSFRs in the third and fourth Galactic quadrants, where the data of such kinds of measurements are largely absent at present. In the near future, the SKA is expected to open a new era for the trigonometric measurements of a large number of HMSFRs and hence for investigations into the Galaxy’s global spiral structure. On the other hand, the Gaia EDR3 was released at the end of 2020, the parallax uncertainties have been significantly improved to be 0.02–0.03 mas for a G band magnitude less than 15, and 0.07 mas for G = 17. The full Gaia DR3 is expected in 2022. Gaia is still committing itself to improving the accuracies of parallaxes and proper motions for a large number of stars. Although the stars measured by Gaia suffered from dust extinction, so that distant objects cannot be measured, the Gaia data have the advantage of revealing detailed structures/substructures and kinematic properties in the solar neighborhood, at least for the regions within about 5 kpc of the Sun. In the solar neighborhood, the segments of dominant spiral arms have been well traced as discussed in the main text. However, the properties of substructures in the spiral arms or inter-arm regions, and the properties of stellar arms traced by evolved stars are still far from conclusive, which may be deserving of more attention.
Author Contributions
LGH conceived the original idea, and responsible for the data collection, interpretation and analysis of the data, and writing of the manuscript.
Funding
This work is supported by the National Key R and D Program of China (NO. 2017YFA0402701) and the National Natural Science Foundation (NNSF) of China No. 11988101, 11933011, 11833009. This work is also supported by the Youth Innovation Promotion Association, Chinese Academy of Sciences.
Conflict of Interest
The author declares that the research was conducted in the absence of any commercial or financial relationships that could be construed as a potential conflict of interest.
Publisher’s Note
All claims expressed in this article are solely those of the authors and do not necessarily represent those of their affiliated organizations, or those of the publisher, the editors and the reviewers. Any product that may be evaluated in this article, or claim that may be made by its manufacturer, is not guaranteed or endorsed by the publisher.
Acknowledgments
The author thanks the anonymous referees for constructive comments and suggestions that significantly improved this work, and Prof. Y. Xu for helpful suggestions. The author would also like to thank Dr C. J. Hao for kindly providing the data of young OCs, and Dr X. Y. Gao for carefully reading the manuscript. This work has made use of data from the European Space Agency (ESA) mission Gaia (https://www.cosmos.esa.int/gaia), processed by the Gaia Data Processing and Analysis Consortium (DPAC, https://www. cosmos. esa.int/web/gaia/dpac/consortium). Funding for the DPAC has been provided by national institutions, in particular the institutions participating in the Gaia Multilateral Agreement.
Footnotes
1http://www.radioast.nsdc.cn/mwisp.php
2The “guiding coordinate space” is defined as (please see Khoperskov et al., 2020, for a detail): Xg = −Rgsin(ϕ), Yg = −Rgcos(ϕ), here, Rg = Lz/VLSR is the guiding radius, Lz = R × Vϕ is the instantaneous angular momentum of the star, R is the Galactocentric distance, ϕ is the azimuthal angle around the Galactic center clockwise from the direction towards the Sun, Vϕ is the azimuthal velocity in the Galactic plane.
References
Aasi, J., Abbott, B. P., Abbott, R., Abbott, T. D., Abernathy, M. R., Acernese, F., et al. (2016). Search of the Orion spur for Continuous Gravitational Waves Using a Loosely Coherent Algorithm on Data from LIGO Interferometers. Phys. Rev. D 93, 042006. doi:10.1103/PhysRevD.93.042006
Amaral, L. H., and Lepine, J. R. D. (1997). A Self-Consistent Model of the Spiral Structure of the Galaxy. Monthly Notices R. Astronomical Soc. 286, 885–894. doi:10.1093/mnras/286.4.885
Anderson, L. D., Armentrout, W. P., Johnstone, B. M., Bania, T. M., Balser, D. S., Wenger, T. V., et al. (2015). Finding Distant Galactic HII Regions. ApJS 221, 26. doi:10.1088/0067-0049/221/2/26
Anderson, L. D., Bania, T. M., Balser, D. S., Cunningham, V., Wenger, T. V., Johnstone, B. M., et al. (2014). The WISE Catalog of Galactic H II Regions. ApJS 212, 1. doi:10.1088/0067-0049/212/1/1
Becker, W. (1963). Die räumliche Verteilung von 156 galaktischen Sternhaufen in Abhängigkeit von ihrem Alter. Mit 7 Textabbildungen. Z. Astrophysik 57, 117.
Becker, W., and Fenkart, R. (1970). “Galactic Clusters and HII Regions,” in The Spiral Structure of Our Galaxy. Editors W. Becker, and G. I. Kontopoulos, 38, 205–208. doi:10.1007/978-94-010-3275-9_35
Becker, W. (1964). “Space Distribution of 156 Galactic star Clusters and of 61 HII Regions,” in The Galaxy and the Magellanic Clouds. Editor F. J. Kerr, 20, 16–18. doi:10.1017/s0074180900052141
Bissantz, N., Englmaier, P., and Gerhard, O. (2003). Gas Dynamics in the Milky Way: Second Pattern Speed and Large-Scale Morphology. Monthly Notices R. Astronomical Soc. 340, 949–968. doi:10.1046/j.1365-8711.2003.06358.x
Bobylev, V. V., and Bajkova, A. T. (2014). The Milky Way Spiral Structure Parameters from Data on Masers and Selected Open Clusters. Monthly Notices R. Astronomical Soc. 437, 1549–1553. doi:10.1093/mnras/stt1987
Bok, B. J. (1964). “An Outline of the Spiral Structure of the Southern Milky Way,” in The Galaxy and the Magellanic Clouds. Editor F. J. Kerr, 20, 147–155. doi:10.1017/s0074180900052475
Bok, B. J., Hine, A. A., and Miller, E. W. (1970). “A Progress Report on the Carina Spiral Feature,” in The Spiral Structure of Our Galaxy. Editors W. Becker, and G. I. Kontopoulos, 38, 246–261. doi:10.1007/978-94-010-3275-9_44
Bossini, D., Vallenari, A., Bragaglia, A., Cantat-Gaudin, T., Sordo, R., Balaguer-Núñez, L., et al. (2019). Age Determination for 269 Gaia DR2 Open Clusters. A and A 623, A108. doi:10.1051/0004-6361/201834693
Brown, A. G. A., Vallenari, A., Prusti, T., de Bruijne, J. H. J., Babusiaux, C., Bailer-Jones, C. A. L., et al. (2018). Gaia Data Release 2. Summary of the Contents and Survey Properties. Astron. Astrophysics 616, A1. doi:10.1051/0004-6361/201833051
Brown, A. G. A., Vallenari, A., Prusti, T., de Bruijne, J. H. J., Babusiaux, C., Biermann, M., et al. (2021). Gaia Early Data Release 2. Summary of the Contents and Survey Properties. Astron. Astrophysics 649, A1. doi:10.1051/0004-6361/202039657
Burton, W. B. (1973). The Kinematics of Galactic Spiral Structure. Pasp 85, 679–703. doi:10.1086/129529
Camargo, D., Bica, E., and Bonatto, C. (2013). Towards a Census of the Galactic Anticentre star Clusters - III. Tracing the Spiral Structure in the Outer Disc. Monthly Notices R. Astronomical Soc. 432, 3349–3360. doi:10.1093/mnras/stt703
Cantat-Gaudin, T., Anders, F., Castro-Ginard, A., Jordi, C., Romero-Gómez, M., Soubiran, C., et al. (2020). Painting a Portrait of the Galactic Disc with its Stellar Clusters. A&A 640, A1. doi:10.1051/0004-6361/202038192
Cantat-Gaudin, T., and Anders, F. (2020). Clusters and Mirages: Cataloguing Stellar Aggregates in the Milky Way. A&A 633, A99. doi:10.1051/0004-6361/201936691
Cantat-Gaudin, T., Jordi, C., Vallenari, A., Bragaglia, A., Balaguer-Núñez, L., Soubiran, C., et al. (2018). A Gaia DR2 View of the Open Cluster Population in the Milky Way. A&A 618, A93. doi:10.1051/0004-6361/201833476
Cantat-Gaudin, T., Krone-Martins, A., Sedaghat, N., Farahi, A., de Souza, R. S., Skalidis, R., et al. (2019). Gaia DR2 Unravels Incompleteness of Nearby Cluster Population: New Open Clusters in the Direction of Perseus. A&A 624, A126. doi:10.1051/0004-6361/201834453
Carraro, G. (2014). “The Milky Way Thin Disk Structure as Revealed by Stars and Young Open Clusters,” in Setting the Scene for Gaia and LAMOST. Editors S. Feltzing, G. Zhao, N. A. Walton, and P. Whitelock, 9, 7–16. doi:10.1017/S1743921313006157
Carraro, G., Vázquez, R. A., Moitinho, A., and Baume, G. (2005). Detection of a Young Stellar Population in the Background of Open Clusters in the Third Galactic Quadrant. ApJ 630, L153–L156. doi:10.1086/491787
Castro-Ginard, A., Jordi, C., Luri, X., Álvarez Cid-Fuentes, J., Casamiquela, L., Anders, F., et al. (2020). Hunting for Open Clusters in Gaia DR2: 582 New Open Clusters in the Galactic Disc. A&A 635, A45. doi:10.1051/0004-6361/201937386
Castro-Ginard, A., Jordi, C., Luri, X., Cantat-Gaudin, T., and Balaguer-Núñez, L. (2019). Hunting for Open Clusters in Gaia DR2: the Galactic Anticentre. A&A 627, A35. doi:10.1051/0004-6361/201935531
Castro-Ginard, A., Jordi, C., Luri, X., Julbe, F., Morvan, M., Balaguer-Núñez, L., et al. (2018). A New Method for Unveiling Open Clusters in Gaia. A&A 618, A59. doi:10.1051/0004-6361/201833390
Caswell, J. L., and Haynes, R. F. (1987). Southern HII Regions : an Extensive Study of Radio Recombination Lineemission. Astron. Astrophysics 171, 261–276.
Chakrabarti, S., Laughlin, G., and Shu, F. H. (2003). Branch, Spur, and Feather Formation in Spiral Galaxies. ApJ 596, 220–239. doi:10.1086/377578
Chen, B.-Q., Huang, Y., Hou, L.-G., Tian, H., Li, G.-X., Yuan, H.-B., et al. (2019a). The Galactic Spiral Structure as Revealed by O- and Early B-type Stars. Monthly Notices R. Astronomical Soc. 487, 1400–1409. doi:10.1093/mnras/stz1357
Chen, B.-Q., Huang, Y., Yuan, H.-B., Wang, C., Fan, D.-W., Xiang, M.-S., et al. (2019b). Three-dimensional Interstellar Dust Reddening Maps of the Galactic Plane. Monthly Notices R. Astronomical Soc. 483, 4277–4289. doi:10.1093/mnras/sty3341
Chen, B.-Q., Li, G.-X., Yuan, H.-B., Huang, Y., Tian, Z.-J., Wang, H.-F., et al. (2020). A Large Catalogue of Molecular Clouds with Accurate Distances within 4 Kpc of the Galactic Disc. Monthly Notices R. Astronomical Soc. 493, 351–361. doi:10.1093/mnras/staa235
Chen, X., Li, J.-J., Zhang, B., Ellingsen, S. P., Xu, Y., Ren, Z.-Y., et al. (2019). High-mass Star Formation in the Nearby Region G352.630-1.067. I. Parallax. ApJ 871, 198. doi:10.3847/1538-4357/aaf862
Chiba, R., Friske, J. K. S., and Schönrich, R. (2020). Resonance Sweeping by a Decelerating Galactic Bar. Monthly Notices R. Astronomical Soc. 500, 4710–4729. doi:10.1093/mnras/staa3585
Churchwell, E., Babler, B. L., Meade, M. R., Whitney, B. A., Benjamin, R., Indebetouw, R., et al. (2009). TheSpitzer/GLIMPSE Surveys: A New View of the Milky Way. Publ. Astron. Soc. Pac. 121, 213–230. doi:10.1086/597811
Cohen, R. S., Cong, H., Dame, T. M., and Thaddeus, P. (1980). Molecular Clouds and Galactic Spiral Structure. ApJ 239, L53–L56. doi:10.1086/183290
Cohen, R. S., Grabelsky, D. A., May, J., Alvarez, H., Bronfman, L., and Thaddeus, P. (1985). Molecular Clouds in the Carina Arm. ApJ 290, L15–L20. doi:10.1086/184433
Courtès, G., Georgelin, Y. P., Georgelin, Y. M., and Monnet, G. (1970). “A New Interpretation of the Galactic Structure from HII Regions,” in The Spiral Structure of Our Galaxy. Editors W. Becker, and G. I. Kontopoulos, 38, 209–212. doi:10.1007/978-94-010-3275-9_36
Dame, T. M., Hartmann, D., and Thaddeus, P. (2001). The Milky Way in Molecular Clouds: A New Complete CO Survey. ApJ 547, 792–813. doi:10.1086/318388
de Zeeuw, P. T., Hoogerwerf, R., de Bruijne, J. H. J., Brown, A. G. A., and Blaauw, A. (1999). A [ITAL]Hipparcos[/ITAL] Census of the Nearby OB Associations. Astronomical J. 117, 354–399. doi:10.1086/300682
Dias, W. S., Alessi, B. S., Moitinho, A., and Lépine, J. R. D. (2002). New Catalogue of Optically Visible Open Clusters and Candidates. A&A 389, 871–873. doi:10.1051/0004-6361:20020668
Dias, W. S., and Lépine, J. R. D. (2005). Direct Determination of the Spiral Pattern Rotation Speed of the Galaxy. ApJ 629, 825–831. doi:10.1086/431456
Dias, W. S., Monteiro, H., and Assafin, M. (2018). Update of Membership and Mean Proper Motion of Open Clusters from UCAC5 Catalogue. Monthly Notices R. Astronomical Soc. 478, 5184–5189. doi:10.1093/mnras/sty1456
Dias, W. S., Monteiro, H., Caetano, T. C., Lépine, J. R. D., Assafin, M., and Oliveira, A. F. (2014). Proper Motions of the Optically Visible Open Clusters Based on the UCAC4 Catalog. A&A 564, A79. doi:10.1051/0004-6361/201323226
Dias, W. S., Monteiro, H., Lépine, J. R. D., and Barros, D. A. (2019). The Spiral Pattern Rotation Speed of the Galaxy and the Corotation Radius with Gaia DR2. Monthly Notices R. Astronomical Soc. 486, 5726–5736. doi:10.1093/mnras/stz1196
Dias, W. S., Monteiro, H., Moitinho, A., Lépine, J. R. D., Carraro, G., Paunzen, E., et al. (2021). Updated Parameters of 1743 Open Clusters Based on Gaia DR2. Monthly Notices R. Astronomical Soc. 504, 356–371. doi:10.1093/mnras/stab770
Díaz-García, S., Salo, H., Knapen, J. H., and Herrera-Endoqui, M. (2019). The Shapes of Spiral Arms in the S4G Survey and Their Connection with Stellar Bars. A&A 631, A94. doi:10.1051/0004-6361/201936000
Dobbs, C., and Baba, J. (2014). Dawes Review 4: Spiral Structures in Disc Galaxies. Publ. Astron. Soc. Aust. 31, e035. doi:10.1017/pasa.2014.31
Dobbs, C. L., and Bonnell, I. A. (2006). Spurs and Feathering in Spiral Galaxies. Monthly Notices R. Astronomical Soc. 367, 873–878. doi:10.1111/j.1365-2966.2006.10146.x
Dobbs, C. L., and Pringle, J. E. (2010). Age Distributions of star Clusters in Spiral and Barred Galaxies as a Test for Theories of Spiral Structure. Monthly Notices R. Astronomical Soc. 409, 396–404. doi:10.1111/j.1365-2966.2010.17323.x
D’Onghia, E., Vogelsberger, M., and Hernquist, L. (2013). Self-perpetuating Spiral Arms in Disk Galaxies. Astrophysical J. 766, 34. doi:10.1088/0004-637X/766/1/34
Downes, D., Wilson, T. L., Bieging, J., and Wink, J. (1980). H110alpha and H2CO Survey of Galactic Radio Sources. Astron. Astrophysics, Suppl. Ser. 40, 379–394.
Drimmel, R. (2000). Evidence for a Two-Armed Spiral in the Milky Way. Astron. Astrophysics 358, L13–L16.
Drimmel, R., and Spergel, D. N. (2001). Three‐dimensional Structure of the Milky Way Disk: The Distribution of Stars and Dust beyond 0.35R⊙. ApJ 556, 181–202. doi:10.1086/321556
Duarte-Cabral, A., Colombo, D., Urquhart, J. S., Ginsburg, A., Russeil, D., Schuller, F., et al. (2021). The SEDIGISM Survey: Molecular Clouds in the Inner Galaxy. Monthly Notices R. Astronomical Soc. 500, 3027–3049. doi:10.1093/mnras/staa2480
Eilers, A.-C., Hogg, D. W., Rix, H.-W., Frankel, N., Hunt, J. A. S., Fouvry, J.-B., et al. (2020). The Strength of the Dynamical Spiral Perturbation in the Galactic Disk. ApJ 900, 186. doi:10.3847/1538-4357/abac0b
Eker, Z., Bilir, S., Soydugan, F., Gökçe, E. Y., Soydugan, E., Tüysüz, M., et al. (2014). The Catalogue of Stellar Parameters from the Detached Double-Lined Eclipsing Binaries in the Milky Way. Publ. Astron. Soc. Aust. 31, e024. doi:10.1017/pasa.2014.17
Elmegreen, D. M. (1980). Properties of Spurs in Spiral Galaxies. ApJ 242, 528–532. doi:10.1086/158486
Faure, C., Siebert, A., and Famaey, B. (2014). Radial and Vertical Flows Induced by Galactic Spiral Arms: Likely Contributors to Our 'wobbly Galaxy'. Monthly Notices R. Astronomical Soc. 440, 2564–2575. doi:10.1093/mnras/stu428
Fenkart, R. P., and Binggeli, B. (1979). A Catalogue of Galactic Clusters Observed in Three Colours. Astron. Astrophysics, Suppl. Ser. 35, 271–275.
Fernie, J. D. (1968). Classical Cepheids and Galactic Structure. Astronomical J. 73, 995. doi:10.1086/110758
Ferreira, F. A., Corradi, W. J. B., Maia, F. F. S., Angelo, M. S., and Santos, J. F. C. (2020). Discovery and Astrophysical Properties of Galactic Open Clusters in Dense Stellar fields Using Gaia DR2. Monthly Notices R. Astronomical Soc. 496, 2021–2038. doi:10.1093/mnras/staa1684
Ferreira, F. A., Corradi, W. J. B., Maia, F. F. S., Angelo, M. S., and Santos, J. F. C. (2021). New star Clusters Discovered towards the Galactic Bulge Direction Using Gaia DR2. Monthly Notices R. Astronomical Soc. 502, L90–L94. doi:10.1093/mnrasl/slab011
Fish, V. L. (2007). “Masers and star Formation,” in Astrophysical Masers and Their Environments. Editors J. M. Chapman, and W. A. Baan, 3, 71–80. doi:10.1017/s1743921307012604
Foster, T., and Brunt, C. M. (2015). A CGPS Look at the Spiral Structure of the Outer Milky Way. I. Distances and Velocities to Star-forming Regions. Aj 150, 147. doi:10.1088/0004-6256/150/5/147
Foster, T., and Cooper, B. (2010). “Structure and Dynamics of the Milky Way: The Evolving Picture,” in The Dynamic Interstellar Medium: A Celebration of the Canadian Galactic Plane Survey. Editors R. Kothes, T. L. Landecker, and A. G. Willis, 438, 16.
García, P., Bronfman, L., Nyman, L. Å., Dame, T. M., and Luna, A. (2014). Giant Molecular Clouds and Massive Star Formation in the Southern Milky Way. ApJS 212, 2. doi:10.1088/0067-0049/212/1/2
Georgelin, Y. M., and Georgelin, Y. P. (1976). The Spiral Structure of Our Galaxy Determined from H II Regions. Astron. Astrophysics 49, 57–79.
Georgelin, Y. P., and Georgelin, Y. M. (1971). Spiral Structure of Our Galaxy from H N Regions. Astron. Astrophysics 12, 482.
Grabelsky, D. A., Cohen, R. S., Bronfman, L., and Thaddeus, P. (1988). Molecular Clouds in the Carina Arm - the Largest Objects, Associated Regions of star Formation, and the Carina Arm in the Galaxy. ApJ 331, 181. doi:10.1086/166548
Han, J. L. (2017). Observing Interstellar and Intergalactic Magnetic Fields. Annu. Rev. Astron. Astrophys. 55, 111–157. doi:10.1146/annurev-astro-091916-055221
Hao, C. J., Xu, Y., Hou, L. G., Bian, S. B., Li, J. J., Wu, Z. Y., et al. (2021). Evolution of the Local Spiral Structure of the Milky Way Revealed by Open Clusters. arXiv [Epub ahead of print]. doi:10.1051/0004-6361/202140608
Hao, C., Xu, Y., Wu, Z., He, Z., and Bian, S. (2020). Sixteen Open Clusters Discovered with Sample-Based Clustering Search of Gaia DR2. Pasp 132, 034502. doi:10.1088/1538-3873/ab694d
He, Z.-H., Xu, Y., Hao, C.-J., Wu, Z.-Y., and Li, J.-J. (2021a). A Catalogue of 74 New Open Clusters Found in Gaia Data-Release 2. Res. Astron. Astrophys. 21, 093. doi:10.1088/1674-4527/21/4/93
He, Z.-H., Xu, Y., and Hou, L.-G. (2021b). Search for Age Pattern across Spiral Arms of the Milky Way. Res. Astron. Astrophys. 21, 009. doi:10.1088/1674-4527/21/1/9
Heyer, M., and Dame, T. M. (2015). Molecular Clouds in the Milky Way. Annu. Rev. Astron. Astrophys. 53, 583–629. doi:10.1146/annurev-astro-082214-122324
Hirota, T., Nagayama, T., Honma, M., Adachi, Y., Burns, R. A., Chibueze, J. O., et al. (2020). The First VERA Astrometry Catalog. Publications Astronomical Soc. Jpn. 72, 50. doi:10.1093/pasj/psaa018
Honig, Z. N., and Reid, M. J. (2015). Characteristics of Spiral Arms in Late-type Galaxies. ApJ 800, 53. doi:10.1088/0004-637X/800/1/53
Honma, M., Nagayama, T., Ando, K., Bushimata, T., Choi, Y. K., Handa, T., et al. (2012). Fundamental Parameters of the Milky Way Galaxy Based on VLBI Astrometry. Publ. Astron. Soc. Jpn. 64, 136. doi:10.1093/pasj/64.6.136
Hottier, C., Babusiaux, C., and Arenou, F. (2020). FEDReD. A&A 641, A79. doi:10.1051/0004-6361/202037573
Hou, L. G., and Han, J. L. (2015). Offset between Stellar Spiral Arms and Gas Arms of the Milky Way. Mon. Not. R. Astron. Soc. 454, 626–636. doi:10.1093/mnras/stv1904
Hou, L. G., Han, J. L., and Shi, W. B. (2009). The Spiral Structure of Our Milky Way Galaxy. A&A 499, 473–482. doi:10.1051/0004-6361/200809692
Hou, L. G., and Han, J. L. (2014). The Observed Spiral Structure of the Milky Way. A&A 569, A125. doi:10.1051/0004-6361/201424039
Humphreys, R. M. (1970). The Space Distribution and Kinematics of Supergiants. Astronomical J. 75, 602–623. doi:10.1086/110995
Hunt, E. L., and Reffert, S. (2021). Improving the Open Cluster Census. A&A 646, A104. doi:10.1051/0004-6361/202039341
Hunt, J. A. S., Bub, M. W., Bovy, J., Mackereth, J. T., Trick, W. H., and Kawata, D. (2019). Signatures of Resonance and Phase Mixing in the Galactic Disc. Monthly Notices R. Astronomical Soc. 490, 1026–1043. doi:10.1093/mnras/stz2667
Hunt, J. A. S., Johnston, K. V., Pettitt, A. R., Cunningham, E. C., Kawata, D., and Hogg, D. W. (2020). The Power of Coordinate Transformations in Dynamical Interpretations of Galactic Structure. Monthly Notices R. Astronomical Soc. 497, 818–828. doi:10.1093/mnras/staa1987
Janes, K., and Adler, D. (1982). Open Clusters and Galactic Structure. ApJS 49, 425–446. doi:10.1086/190805
Janes, K. A., Tilley, C., and Lynga, G. (1988). Properties of the Open Cluster System. Astronomical J. 95, 771. doi:10.1086/114676
Junichi, B., Takayuki, R. S., and Keiichi, W. (2010). On the Interpretation of Thel-vFeatures in the Milky Way Galaxy. Publ. Astron. Soc. Jpn. 62, 1413–1422. doi:10.1093/pasj/62.6.1413
Kawata, D., Baba, J., Ciucă, I., Cropper, M., Grand, R. J. J., Hunt, J. A. S., et al. (2018). Radial Distribution of Stellar Motions in Gaia DR2. Monthly Notices R. Astronomical Soc. 479, L108–L112. doi:10.1093/mnrasl/sly107
Kendall, S., Kennicutt, R. C., and Clarke, C. (2011). Spiral Structure in Nearby Galaxies - I. Sample, Data Analysis and Overview of Results. Monthly Notices R. Astronomical Soc. 414, 538–564. doi:10.1111/j.1365-2966.2011.18422.x
Kennicutt, R. C. J. (1981). The Shapes of Spiral Arms along the Hubble Sequence. Astronomical J. 86, 1847–1858. doi:10.1086/113064
Kharchenko, N. V., Piskunov, A. E., Schilbach, E., Röser, S., and Scholz, R.-D. (2013). Global Survey of star Clusters in the Milky Way. A&A 558, A53. doi:10.1051/0004-6361/201322302
Khoperskov, S., Gerhard, O., Di Matteo, P., Haywood, M., Katz, D., Khrapov, S., et al. (2020). Hic Sunt Dracones: Cartography of the Milky Way Spiral Arms and Bar Resonances with Gaia Data Release 2. A&A 634, L8. doi:10.1051/0004-6361/201936645
Kolesnik, L. N., and Vedenicheva, I. P. (1979). The Displacement of Spiral Arms of the Galaxy from the Galactic Plane. Astron. Astrophysics 76, 124–126.
Koo, B.-C., Park, G., Kim, W.-T., Lee, M. G., Balser, D. S., and Wenger, T. V. (2017). Tracing the Spiral Structure of the Outer Milky Way with Dense Atomic Hydrogen Gas. Pasp 129, 094102. doi:10.1088/1538-3873/aa7c08
Kounkel, M., and Covey, K. (2019). Untangling the Galaxy. I. Local Structure and Star Formation History of the Milky Way. Aj 158, 122. doi:10.3847/1538-3881/ab339a
Krishnan, V., Ellingsen, S. P., Reid, M. J., Bignall, H. E., McCallum, J., Phillips, C. J., et al. (2017). Parallaxes of 6.7-GHz Methanol Masers towards the G 305.2 High-Mass star Formation Region. Mon. Not. R. Astron. Soc. 465, 1095–1105. doi:10.1093/mnras/stw2850
La Vigne, M. A., Vogel, S. N., and Ostriker, E. C. (2006). AHubble Space TelescopeArchival Survey of Feathers in Spiral Galaxies. ApJ 650, 818–834. doi:10.1086/506589
Lépine, J. R. D., Michtchenko, T. A., Barros, D. A., and Vieira, R. S. S. (2017). The Dynamical Origin of the Local Arm and the Sun's Trapped Orbit. ApJ 843, 48. doi:10.3847/1538-4357/aa72e5
Lépine, J. R. D., Roman-Lopes, A., Abraham, Z., Junqueira, T. C., and Mishurov, Y. N. (2011). The Spiral Structure of the Galaxy Revealed by CS Sources and Evidence for the 4:1 Resonance. Monthly Notices R. Astronomical Soc. 414, 1607–1616. doi:10.1111/j.1365-2966.2011.18492.x
Levine, E. S., Blitz, L., and Heiles, C. (2006). The Spiral Structure of the Outer Milky Way in Hydrogen. Science 312, 1773–1777. doi:10.1126/science.1128455
Li, Z., Shen, J., Gerhard, O., and Clarke, J. P. (2021). Gas Dynamics in the Galaxy: Total Mass Distribution and the Bar Pattern Speed. arXiv e-prints, arXiv:2103.10342
Lin, C. C., and Shu, F. H. (1964). On the Spiral Structure of Disk Galaxies. ApJ 140, 646. doi:10.1086/147955
Lin, C. C., and Shu, F. H. (1966). On the Spiral Structure of Disk Galaxies, II. Outline of a Theory of Density Waves. Proc. Natl. Acad. Sci. 55, 229–234. doi:10.1073/pnas.55.2.229
Liu, C., Wang, Y.-G., Shen, J., Li, Z.-Y., Qin, Y.-J., Hou, Y., et al. (2017). The Local Spiral Arm in the LAMOST- Gaia Common Stars?. ApJ 835, L18. doi:10.3847/2041-8213/835/1/L18
Liu, L., and Pang, X. (2019). A Catalog of Newly Identified Star Clusters in Gaia DR2. ApJS 245, 32. doi:10.3847/1538-4365/ab530a10.3847/1538-4365/ab530a
Lynds, B. T. (1970). “The Distribution of Dark Nebulae in Late-type Spirals,” in The Spiral Structure of Our Galaxy. Editors W. Becker, and G. I. Kontopoulos, 38, 26–34. doi:10.1007/978-94-010-3275-9_5
Majaess, D. J., Turner, D. G., and Lane, D. J. (2009). Characteristics of the Galaxy According to Cepheids. Monthly Notices R. Astronomical Soc. 398, 263–270. doi:10.1111/j.1365-2966.2009.15096.x
Mermilliod, J.-C. (1995). “The Database for Galactic Open Clusters (BDA),” in Information & On-Line Data in Astronomy. Astrophysics and Space Science Library, Vol 203. Editors D. Egret, and M. A. Albrecht (Dordrecht: Springer), 203, 127–138. doi:10.1007/978-94-011-0397-8_12
Michtchenko, T. A., Lépine, J. R. D., Barros, D. A., and Vieira, R. S. S. (2018). Combined Dynamical Effects of the Bar and Spiral Arms in a Galaxy Model. Application to the Solar Neighbourhood. A&A 615, A10. doi:10.1051/0004-6361/201833035
Miller, E. W. (1972). Faint O-B2 Stars in the Vela, Car, Centaurus and Crux Sections of Thesouthern Milky Way. Astronomical J. 77, 216–229. doi:10.1086/111271
Miville-Deschênes, M.-A., Murray, N., and Lee, E. J. (2017). Physical Properties of Molecular Clouds for the Entire Milky Way Disk. ApJ 834, 57. doi:10.3847/1538-4357/834/1/57
Miyachi, Y., Sakai, N., Kawata, D., Baba, J., Honma, M., Matsunaga, N., et al. (2019). Stellar Overdensity in the Local Arm in Gaia DR2. ApJ 882, 48. doi:10.3847/1538-4357/ab2f86
Moisés, A. P., Damineli, A., Figuerêdo, E., Blum, R. D., Conti, P. S., and Barbosa, C. L. (2011). Spectrophotometric Distances to Galactic H II Regions. Monthly Notices R. Astronomical Soc. 411, 705–760. doi:10.1111/j.1365-2966.2010.17713.x
Moitinho, A. (2010). “Observational Properties of the Open Cluster System of the Milky Way and what They Tell Us about Our Galaxy,” in Star Clusters: Basic Galactic Building Blocks throughout Time and Space. Editors R. de Grijs, and J. R. D. Lépine, 5, 106–116. doi:10.1017/S1743921309990949
Moitinho, A., Vázquez, R. A., Carraro, G., Baume, G., Giorgi, E. E., and Lyra, W. (2006). Spiral Structure of the Third Galactic Quadrant and the Solution to the Canis Major Debate. Monthly Notices R. Astronomical Soc. Lett. 368, L77–L81. doi:10.1111/j.1745-3933.2006.00163.x
Monari, G., Famaey, B., Siebert, A., Wegg, C., and Gerhard, O. (2019). Signatures of the Resonances of a Large Galactic Bar in Local Velocity Space. A&A 626, A41. doi:10.1051/0004-6361/201834820
Monguió, M., Grosbøl, P., and Figueras, F. (2015). First Detection of the Field star Overdensity in the Perseus Arm. A&A 577, A142. doi:10.1051/0004-6361/201424896
Monteiro, H., Barros, D. A., Dias, W. S., and Lépine, J. R. D. (2021). The Distribution of Open Clusters in the Galaxy. Front. Astron. Space Sci. 8, 62. doi:10.3389/fspas.2021.656474
Monteiro, H., and Dias, W. S. (2019). Distances and Ages from Isochrone Fits of 150 Open Clusters Using Gaia DR2 Data. Monthly Notices R. Astronomical Soc. 487, 2385–2406. doi:10.1093/mnras/stz1455
Morgan, W. W., Sharpless, S., and Osterbrock, D. (1952). Some Features of Galactic Structure in the Neighborhood of the Sun. Astronomical J. 57, 3. doi:10.1086/106673
Morgan, W. W., Whitford, A. E., and Code, A. D. (1953). Studies in Galactic Structure. I. A Preliminary Determination of the Space Distribution of the Blue Giants. ApJ 118, 318. doi:10.1086/145754
Münch, G. (1953). Galactic Structure and the Distribution of Interstellar Gas. Pasp 65, 179. doi:10.1086/126574
Murray, N. (2011). Star Formation Efficiencies and Lifetimes of Giant Molecular Clouds in the Milky Way. ApJ 729, 133. doi:10.1088/0004-637x/729/2/133
Myers, P. C., Dame, T. M., Thaddeus, P., Cohen, R. S., Silverberg, R. F., Dwek, E., et al. (1986). Molecular Clouds and star Formation in the Inner Galaxy - A Comparison of CO, H II, and Far-Infrared Surveys. ApJ 301, 398. doi:10.1086/163909
Nakanishi, H., and Sofue, Y. (2016). Three-dimensional Distribution of the ISM in the Milky Way Galaxy. III. The Total Neutral Gas Disk. Publ. Astron. Soc. Jpn. 68, 5. doi:10.1093/pasj/psv108
Paladini, R., Davies, R. D., and DeZotti, G. (2004). Spatial Distribution of Galactic HII Regions. Monthly Notices R. Astronomical Soc. 347, 237–245. doi:10.1111/j.1365-2966.2004.07210.x
Pantaleoni González, M., Maíz Apellániz, J., Barbá, R. H., and Reed, B. C. (2021). The Alma Catalogue of OB Stars - II. A Cross-Match with Gaia DR2 and an Updated Map of the Solar Neighbourhood. Monthly Notices R. Astronomical Soc. 504, 2968–2982. doi:10.1093/mnras/stab688
Pettitt, A. R., Dobbs, C. L., Acreman, D. M., and Bate, M. R. (2015). The Morphology of the Milky Way - II. Reconstructing CO Maps from Disc Galaxies with Live Stellar Distributions. Monthly Notices R. Astronomical Soc. 449, 3911–3926. doi:10.1093/mnras/stv600
Pettitt, A. R., Dobbs, C. L., Acreman, D. M., and Price, D. J. (2014). The Morphology of the Milky Way - I. Reconstructing CO Maps from Simulations in Fixed Potentials. Monthly Notices R. Astronomical Soc. 444, 919–941. doi:10.1093/mnras/stu1075
Poggio, E., Drimmel, R., Cantat-Gaudin, T., Ramos, P., Ripepi, V., Zari, E., et al. (2021). Galactic Spiral Structure Revealed by Gaia EDR3. arXiv e-prints, arXiv:2103.01970
Pohl, M., Englmaier, P., and Bissantz, N. (2008). Three‐Dimensional Distribution of Molecular Gas in the Barred Milky Way. ApJ 677, 283–291. doi:10.1086/529004
Prusti, T., de Bruijne, J. H. J., Brown, A. G. A., Vallenari, A., Babusiaux, C., Bailer-Jones, C. A. L., et al. (2016). The Gaia mission. Astron. Astrophysics 595, A1. doi:10.1051/0004-6361/201629272
Reed, B. C., and Reed, L. G. (2000). BVRPhotometry of Northern Hemisphere Luminous Stars. V. 123 Stars in the First Two Galactic Quadrants and the Distribution of O-B2 Supergiants1. Publ. Astron. Soc. Pac. 112, 409–416. doi:10.1086/316524
Reid, M. J., Menten, K. M., Brunthaler, A., Zheng, X. W., Dame, T. M., Xu, Y., et al. (2019). Trigonometric Parallaxes of High-Mass Star-forming Regions: Our View of the Milky Way. ApJ 885, 131. doi:10.3847/1538-4357/ab4a11
Reid, M. J., Menten, K. M., Zheng, X. W., Brunthaler, A., Moscadelli, L., Xu, Y., et al. (2009). Trigonometric Parallaxes of Massive Star-Forming Regions. VI. Galactic Structure, Fundamental Parameters, and Noncircular Motions. ApJ 700, 137–148. doi:10.1088/0004-637X/700/1/137
Rice, T. S., Goodman, A. A., Bergin, E. A., Beaumont, C., and Dame, T. M. (2016). A Uniform Catalog of Molecular Clouds in the Milky Way. ApJ 822, 52. doi:10.3847/0004-637X/822/1/52
Roberts, W. W. (1969). Large-Scale Shock Formation in Spiral Galaxies and its Implications on Star Formation. ApJ 158, 123. doi:10.1086/150177
Rodriguez-Fernandez, N. J., and Combes, F. (2008). Gas Flow Models in the Milky Way Embedded Bars. A&A 489, 115–133. doi:10.1051/0004-6361:200809644
Russeil, D. (2003). Star-forming Complexes and the Spiral Structure of Our Galaxy. A&A 397, 133–146. doi:10.1051/0004-6361:20021504
Rygl, K. L. J., Brunthaler, A., Reid, M. J., Menten, K. M., van Langevelde, H. J., and Xu, Y. (2010). Trigonometric Parallaxes of 6.7 GHz Methanol Masers. A&A 511, A2. doi:10.1051/0004-6361/200913135
Sampedro, L., Dias, W. S., Alfaro, E. J., Monteiro, H., and Molino, A. (2017). A Multimembership Catalogue for 1876 Open Clusters Using UCAC4 Data. Monthly Notices R. Astronomical Soc. 470, 3937–3945. doi:10.1093/mnras/stx1485
Schmeja, S., Kharchenko, N. V., Piskunov, A. E., Röser, S., Schilbach, E., Froebrich, D., et al. (2014). Global Survey of star Clusters in the Milky Way. A&A 568, A51. doi:10.1051/0004-6361/201322720
Schmidt-Kaler, T. (1975). The Spiral Structure of Our Galaxy- A Review of Current Studies. Vistas Astron. 19, 69IN575IN777–74IN676IN889. doi:10.1016/0083-6656(75)90007-0
Scholz, R.-D., Kharchenko, N. V., Piskunov, A. E., Röser, S., and Schilbach, E. (2015). Global Survey of star Clusters in the Milky Way. A&A 581, A39. doi:10.1051/0004-6361/201526312
Seigar, M. S., and James, P. A. (1998). The Structure of Spiral Galaxies - II. Near-Infrared Properties of Spiral Arms. Monthly Notices R. Astronomical Soc. 299, 685–698. doi:10.1046/j.1365-8711.1998.01779.x
Sellwood, J. A., and Carlberg, R. G. (1984). Spiral Instabilities Provoked by Accretion and star Formation. ApJ 282, 61–74. doi:10.1086/162176
Shahzamanian, B., Schödel, R., Nogueras-Lara, F., Dong, H., Gallego-Cano, E., Gallego-Calvente, A. T., et al. (2019). First Results from a Large-Scale Proper Motion Study of the Galactic centre. A&A 632, A116. doi:10.1051/0004-6361/201936579
Shen, J., and Zheng, X.-W. (2020). The Bar and Spiral Arms in the Milky Way: Structure and Kinematics. Res. Astron. Astrophys. 20, 159. doi:10.1088/1674-4527/20/10/159
Shu, F. H. (2016). Six Decades of Spiral Density Wave Theory. Annu. Rev. Astron. Astrophys. 54, 667–724. doi:10.1146/annurev-astro-081915-023426
Sim, G., Lee, S. H., Ann, H. B., and Kim, S. (2019). 207 New Open Star Clusters within 1 Kpc from Gaia Data Release 2. J. Korean Astronomical Soc. 52, 145–158. doi:10.5303/JKAS.2019.52.5.145
Skiff, B. A. (2014). VizieR Online Data Catalog: Catalogue of Stellar Spectral Classifications (Skiff, 2009- ). VizieR Online Data Catalog, B/mk
Soubiran, C., Cantat-Gaudin, T., Romero-Gómez, M., Casamiquela, L., Jordi, C., Vallenari, A., et al. (2018). Open Cluster Kinematics with Gaia DR2. A&A 619, A155. doi:10.1051/0004-6361/201834020
Stothers, R., and Frogel, J. A. (1974). The Local Complex of 0 and B Stars. I. Distribution of Stars and Interstellar Dust. Astronomical J. 79, 456. doi:10.1086/111565
Tarricq, Y., Soubiran, C., Casamiquela, L., Cantat-Gaudin, T., Chemin, L., Anders, F., et al. (2021). 3D Kinematics and Age Distribution of the Open Cluster Population. A&A 647, A19. doi:10.1051/0004-6361/202039388
Taylor, J. H., and Cordes, J. M. (1993). Pulsar Distances and the Galactic Distribution of Free Electrons. ApJ 411, 674. doi:10.1086/172870
Thackeray, A. D. (1956). Spectroscopic Indications of an Internal Spiral Arm in the Galaxy. Nature 178, 1458. doi:10.1038/1781458a0
Trick, W. H., Fragkoudi, F., Hunt, J. A. S., Mackereth, J. T., and White, S. D. M. (2020). Identifying Resonances of the Galactic Bar in Gaia DR2: I. Clues from Action Space. Monthly Notices R. Astronomical Soc. 500, 2645–2665. doi:10.1093/mnras/staa3317
Urquhart, J. S., Figura, C. C., Moore, T. J. T., Hoare, M. G., Lumsden, S. L., Mottram, J. C., et al. (2014). The RMS Survey: Galactic Distribution of Massive star Formation★. Monthly Notices R. Astronomical Soc. 437, 1791–1807. doi:10.1093/mnras/stt2006
Vallée, J. P. (2008). New Velocimetry and Revised Cartography of the Spiral Arms in the Milky Way-A Consistent Symbiosis. Astronomical J. 135, 1301–1310. doi:10.1088/0004-6256/135/4/1301
Vallée, J. P. (2018). Offsets of Masers with Respect to the Middle of the Perseus Arm and the Corotation Radius in the Milky Way. ApJ 863, 52. doi:10.3847/1538-4357/aad054
van de Hulst, H. C., Muller, C. A., and Oort, J. H. (1954). The Spiral Structure of the Outer Part of the Galactic System Derived from the Hydrogen Emission at 21 Cm Wavelength. Bull. Astronomical Institutes Neth. 12, 117.
Vázquez, R. A., May, J., Carraro, G., Bronfman, L., Moitinho, A., and Baume, G. (2008). Spiral Structure in the Outer Galactic Disk. I. The Third Galactic Quadrant. ApJ 672, 930–939. doi:10.1086/524003
Walborn, N. R. (1971). Some Spectroscopic Characteristics of the OB Stars: an Investigation of the Space Distribution of Certain OB Stars and the Reference Frame of the Classification. ApJS 23, 257. doi:10.1086/190239
Weaver, H. F. (1970). “Some Characteristics of Interstellar Gas in the Galaxy,” in Interstellar Gas Dynamics. Editor H. J. Habing, 39, 22–50. doi:10.1007/978-94-010-3329-9_3
Wegg, C., Gerhard, O., and Portail, M. (2015). The Structure of the Milky Way's Bar outside the Bulge. Monthly Notices R. Astronomical Soc. 450, 4050–4069. doi:10.1093/mnras/stv745
Willett, K. W., Lintott, C. J., Bamford, S. P., Masters, K. L., Simmons, B. D., Casteels, K. R. V., et al. (2013). Galaxy Zoo 2: Detailed Morphological Classifications for 304 122 Galaxies from the Sloan Digital Sky Survey. Monthly Notices R. Astronomical Soc. 435, 2835–2860. doi:10.1093/mnras/stt1458
Williams, M. E. K., Steinmetz, M., Binney, J., Siebert, A., Enke, H., Famaey, B., et al. (2013). The Wobbly Galaxy: Kinematics north and South with RAVE Red-Clump Giants. Monthly Notices R. Astronomical Soc. 436, 101–121. doi:10.1093/mnras/stt1522
Wright, N. J. (2020). OB Associations and Their Origins. New Astron. Rev. 90, 101549. doi:10.1016/j.newar.2020.101549
Xu, Y., Bian, S. B., Reid, M. J., Li, J. J., Zhang, B., Yan, Q. Z., et al. (2018a). A Comparison of the Local Spiral Structure from Gaia DR2 and VLBI Maser Parallaxes. A&A 616, L15. doi:10.1051/0004-6361/201833407
Xu, Y., Hou, L.-G., and Wu, Y.-W. (2018b). The Spiral Structure of the Milky Way. Res. Astron. Astrophys. 18, 146. doi:10.1088/1674-4527/18/12/146
Xu, Y., Hou, L. G., Bian, S. B., Hao, C. J., Liu, D. J., Li, J. J., et al. (2021). Local Spiral Structure Based on the Gaia EDR3 Parallaxes. A&A 645, L8. doi:10.1051/0004-6361/202040103
Xu, Y., Li, J. J., Reid, M. J., Menten, K. M., Zheng, X. W., Brunthaler, A., et al. (2013). On the Nature of the Local Spiral Arm of the Milky Way. ApJ 769, 15. doi:10.1088/0004-637X/769/1/15
Xu, Y., Reid, M., Dame, T., Menten, K., Sakai, N., Li, J., et al. (2016). The Local Spiral Structure of the Milky Way. Sci. Adv. 2, e1600878. doi:10.1126/sciadv.1600878
Xu, Y., Reid, M. J., Zheng, X. W., and Menten, K. M. (2006). The Distance to the Perseus Spiral Arm in the Milky Way. Science 311, 54–57. doi:10.1126/science.1120914
Yan, Q.-Z., Yang, J., Su, Y., Sun, Y., and Wang, C. (2020). Distances and Statistics of Local Molecular Clouds in the First Galactic Quadrant. ApJ 898, 80. doi:10.3847/1538-4357/ab9f9c
Yan, Q.-Z., Yang, J., Sun, Y., Su, Y., and Xu, Y. (2019). Molecular Cloud Distances Based on the MWISP CO Survey and Gaia DR2. ApJ 885, 19. doi:10.3847/1538-4357/ab458e
Yan, Q.-Z., Yang, J., Sun, Y., Su, Y., Xu, Y., Wang, H., et al. (2021). Distances to Molecular Clouds in the Second Galactic Quadrant. A&A 645, A129. doi:10.1051/0004-6361/202039768
Yao, J. M., Manchester, R. N., and Wang, N. (2017). A New Electron-Density Model for Estimation of Pulsar and FRB Distances. ApJ 835, 29. doi:10.3847/1538-4357/835/1/29
Yu, S.-Y., Ho, L. C., Barth, A. J., and Li, Z.-Y. (2018). The Carnegie-Irvine Galaxy Survey. VI. Quantifying Spiral Structure. ApJ 862, 13. doi:10.3847/1538-4357/aacb25
Keywords: Galaxy: solar neighborhood, Galaxy: structure, Galaxy: disk, Galaxy: spiral structure, HII regions, stars: massive, open clusters and associations: general, molecular clouds
Citation: Hou LG (2021) The Spiral Structure in the Solar Neighborhood. Front. Astron. Space Sci. 8:671670. doi: 10.3389/fspas.2021.671670
Received: 24 February 2021; Accepted: 04 June 2021;
Published: 06 August 2021.
Edited by:
Jacques R. D. Lepine, University of São Paulo, BrazilReviewed by:
J. Allyn Smith, Austin Peay State University, United StatesClare Dobbs, University of Exeter, United Kingdom
Copyright © 2021 Hou. This is an open-access article distributed under the terms of the Creative Commons Attribution License (CC BY). The use, distribution or reproduction in other forums is permitted, provided the original author(s) and the copyright owner(s) are credited and that the original publication in this journal is cited, in accordance with accepted academic practice. No use, distribution or reproduction is permitted which does not comply with these terms.
*Correspondence: L. G. Hou, bGdob3VAbmFvLmNhcy5jbg==