- 1Department of Climate and Space Sciences and Engineering, University of Michigan, Ann Arbor, MI, United States
- 2NCAR/High Altitude Observatory, Boulder, CO, United States
- 3Physics and Astronomy Deparment, George Mason University, Fairfax, VA, United States
- 4National Solar Observatory, Boulder, CO, United States
- 5NASA Goddard Space Flight Center, Greenbelt, MD, United States
- 6Harvard-Smithsonian Center for Astrophysics, Cambirdge, MA, United States
- 7CONICET, Universidad de Mendoza, Mendoza, Argentina
The Need: Understanding and predicting the major phenomena taking place in the solar corona, such as flares and Coronal Mass Ejections (CMEs), the heating and evolution of the solar atmosphere, and the acceleration of the solar wind, are fundamental challenges to predict our own star. These challenges are related to the solar magnetism and to the physical properties of solar plasmas: meeting them requires two types of measurements: (A) Spectrally resolved, simultaneous observations of the entire corona in multiple spectral lines emitted by chromospheric to hot coronal plasmas at high spatial resolution and cadence for long periods of time; and (B) Coronal magnetic field measurements.
The Problem: The current fleet of space instruments suffers from three main limitations: (A) EUV narrow-band imagers provide simultaneous 2D images of the corona, but lack adequate plasma diagnostic capabilities; (B) High-resolution EUV spectrometers have the required diagnostic potential, but their narrow field of view prevents a continuous and simultaneous coverage of the entire corona. (C) No current instrument can measure the global coronal magnetic field.
The Solution: Visible to near-IR coronagraphs coupled to tunable filters combine the strengths of both EUV high resolution spectrometers and EUV imagers in one single instrument by 1) providing 2D images of the whole field of view at a single wavelength; 2) spectrally resolving individual lines near-simultaneously across the entire field of view, and 3) measuring the magnetic field through polarimetry. The proposed Coronal Solar Magnetism Observatory (COSMO) visible to near-IR coronagraph would allow the measurement of: (A) simultaneous plasma thermal structure of the whole solar corona and CMEs; (B) plasma velocity vector; and (C) coronal magnetic field. The technology behind visible/near-IR coronagraphs coupled to tunable filters is mature; ground-based implementation of such instruments would provide long-term, easily-upgradable data sets.
1 Open problems in solar physics
The solar corona has critical importance for the solar-terrestrial relationship, as it hosts a number of phenomena that directly affect the Earth. For example, flares and Coronal Mass Ejections (CMEs), the most important Space Weather events, are initiated in the corona: flare radiation influences the density of the Earth’s upper atmosphere and can perturb satellite trajectories and health; CMEs can disrupt communications, power grids, and pose health hazards to astronauts and even to airplane passengers traveling on polar routes. The solar wind shapes the structure of the Heliosphere, and the multimillion degree temperature of the corona is responsible for the high energy radiation output of the Sun, which shapes terrestrial and planetary ionospheres, is the main energy input of the Earth’s thermosphere, and affects satellite drag. In order to study these phenomena, a host of diagnostic techniques have been developed over the years to determine the main properties of solar atmospheric plasmas. Despite their availability, and the vast amount of measurements that they have allowed, our understanding of the processes regulating the solar corona and capability of predicting solar eruptions is still limited.
For example, the processes that store and release the plasma and energy involved in solar activity events are still poorly understood. This, however, is not the only limitation in our understanding of the solar atmosphere. In fact, the solar corona is heated to multimillion degree temperatures, but the mechanisms that provide the required energy to reach and maintain those temperatures are unknown; the solar wind, accelerated from the solar atmosphere, shapes the heliosphere and its characteristics, still its origin is not understood; the composition of the solar corona is subject to fractionation processes that differentiate it from that of the underlying photosphere, which have not yet been identified. Also, solar activity is strongly dependent on the 22 years solar magnetic cycle, which we are still unable to predict.
Even if we still do not understand some of the fundamental properties of the solar atmosphere, one thing is clear: all these phenomena are related to the magnetic field that pervades the solar atmosphere and organizes the plasma in structures at all scales. Several theories have been proposed on how magnetic energy is released into solar plasmas and how it accelerates them, but progress in discriminating among them has been hindered by one fundamental fact: we do not have measurements of the magnetic field in the solar corona.
Even more importantly, the lack of magnetic field measurements (due to the intrinsic difficulty of measuring it) prevents us from linking this quantity to the other properties of the solar corona, such as plasma density, temperature, dynamics and composition, so that the effects of the magnetic field on coronal plasmas are still not understood.
The lack of coronal magnetic field diagnostics has become the bottleneck in further advancing solar physics.
2 Gleaning information about the Sun: Available plasma diagnostic techniques
Understanding and forecasting the properties of activity events in the solar atmosphere needs measurements of its plasma physical properties. To this end, an array of diagnostic techniques have been developed, most of which utilize the X-ray, EUV and UV emission from the solar corona. Being optically thin, the observed radiation in this wavelength range allows the measurement of line-of-sight (LOS) averaged values for many critically important physical properties, the most important of which are:
• Electron temperature and density
• Plasma temperature distribution (Differential Emission Measure)
• Plasma elemental composition
• LOS velocity
• Ion temperature
• Non-thermal velocities
For a review of these techniques, see Phillips et al. (2008) and Del Zanna and Mason (2018). Additional techniques are available to glean information on the plasma electron velocity distribution (Del Zanna et al., 2022), wind speed (Doppler dimming in UV lines, Kohl et al., 2006), and ion abundances (Boe et al., 2020).
Measurements of the coronal magnetic field are more difficult, because of the weakness of its signatures and of the complexity of the measurements. Indirect sporadic measurements have been carried out using coronal loop seismology (De Moortel et al., 2016) and, recsently, long-duration observations of waves in the solar corona from the Coronal Magnetometer and Polarimeter (CoMP; Tomczyk et al., 2008) in the visible to near-IR wavelength range, providing maps of the plane-of-the-sky component of the coronal magnetic field at the limb (Yang et al., 2020). Components of the coronal magnetic field vector can be measured from polarimetric techniques based on the Hanle and Zeeman effects applied to visible to near-IR spectra of the off-disk solar corona (Lin et al., 2004; Casini et al., 2017). The only measurements of coronal magnetic fields on the disk come from radio measurements, sometimes in combination with EUV observations. These measurements, however, are typically able to yield magnetic field strengths in excess of ≈200 G and thus are most suitable for active regions (e.g., Brosius et al., 2006; Brosius and White 2022). The capability of measuring coronal magnetic field combining radio and EUV observations has been recently reviewed by Fleishman et al. (2022).
Recently, Si et al. (2020) and Landi et al. (2020) developed a new diagnostic technique to measure the magnetic field strength in active regions using a single Fe X line observed by Hinode/EIS at 257.26 Å. This technique has great advantages as it can measure the magnetic field both on the disk and at the limb, and can be applied to more than one solar cycle worth of measurements from Hinode/EIS. However, it does not provide any direct information on the magnetic field vector orientation, and cannot detect the weaker coronal magnetic field found in coronal holes and quiet Sun.
3 Gleaning information about the Sun: Available instruments
There are two main types of instruments currently deployed to study the solar corona in the X-ray, EUV, UV, visible and near-IR ranges: narrow-band imagers and high resolution spectrometers. Both have strengths and weaknesses, which have at the same time allowed us to make great progress in understanding the solar atmosphere and the processes that fuel its temperature structure and activity, and hindered our efforts at giving definitive answers to fundamental processes such as coronal heating and solar wind acceleration. Figure 1 summarizes their strengths and weaknesses.
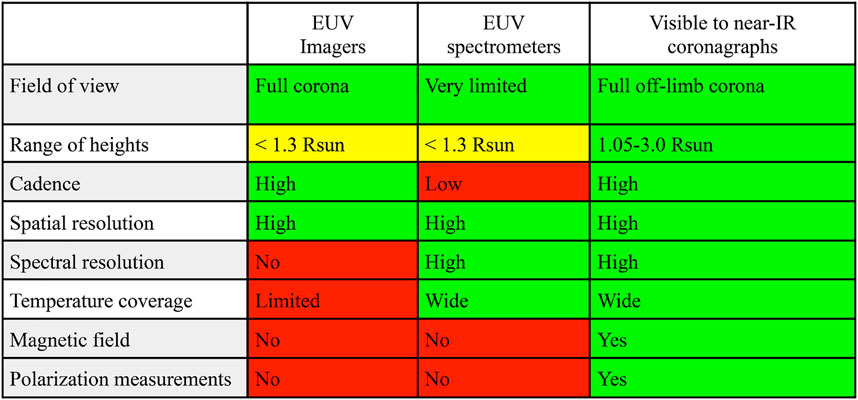
FIGURE 1. Strengths and weaknesses of available instrument types for solar coronal research. Visible to near-IR coronagraphs couple the high cadence and field of view of narrow band EUV imagers and the spectral information provided by EUV spectrometers, and further combine it with the advantages of polarized line observations.
3.1 Narrow band imagers
Narrow band imagers provide high-cadence, high resolution images of the entire corona (or, in the case of coronagraphic instruments, of the entire off-limb corona) by summing the emission in a relatively narrow wavelength range. In this way, they are able to paint a very comprehensive picture of structures in the entire solar atmosphere, and of their time evolution; in the case of soft X-ray or EUV instruments, bandpasses are centered around strong, isolated spectral lines which limit the sensitivity of the instrument to a narrow temperature range. However, several spectral lines from other ions formed at different temperatures beyond the nominal one contribute to the observed emission, so that the temperature response of each channel is wide and greatly limits the ability of the instrument to study the thermal structure of the Sun. Also, soft X-ray and EUV imagers can provide no information about plasma composition and magnetic field, and only approximate results can be obtained on plasma density and dynamical status.
3.2 High resolution spectrometers
By being able to resolve individual lines from a number of ions from different elements, and record their profiles, spectrometers provide the best diagnostic tools at our disposal, and are capable of sampling the wide range of temperatures in the solar atmosphere with the best temperature resolution. However, they also have one significant limitation: by dispersing the image of a narrow-slit, their FOV is very small, and it takes significant time to build 2D images of a portion of the solar surface, even as small as an active region, as shown in Figure 2. This results in non-simultaneous images of the FOV, and in a relatively low cadence of observations.
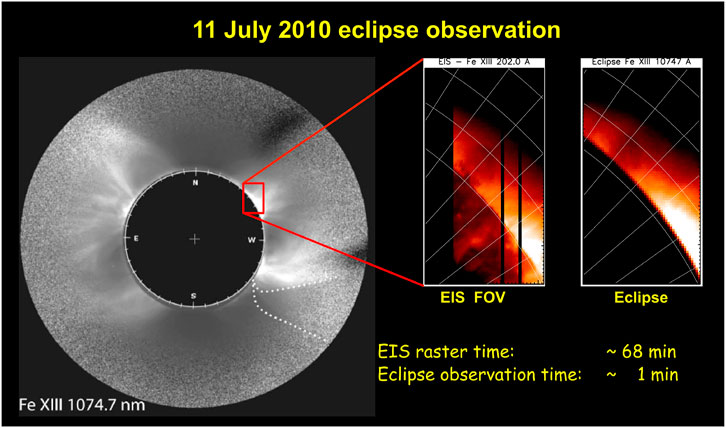
FIGURE 2. Comparison of the field of view and observation times of coordinated EIS observations during the 11 July 2010 eclipse (courtesy of Prof. S.R. Habbal). Ground based COSMO/LC integration time and cadence are comparable to eclipse ones.
3.3 Limitations of short wavelength regimes
Instruments devoted to the study of the solar corona in the UV, EUV and X-ray ranges have one more limitation: the limited range of heights above the photosphere where they can obtain a large enough signal-to-noise ratio to make meaningful observations. This is due to the mechanism generating the radiation in these wavelength ranges, which essentially consists of the excitation of coronal ions by inelastic collisions with free electrons. This mechanism causes the intensity to be proportional to the square of the local plasma density: as coronal density decreases exponentially, the brightness of this type of radiation decreases very rapidly, effectively limiting the FOV of X-ray and EUV instruments to around 0.3 solar radii above the photosphere. Although EUV imagers with larger FOV are being studied (SunCet Cubesat Mission, Mason et al., 2021), FOV limitations will still be a problem in the foreseeable future.
On the contrary, coronal radiation in the visible and near-IR is largely produced by photo-excitation from photospheric radiation, which takes over collisional excitation when this becomes inefficient. Once photoexcitation takes over, the line intensity decreases with distance proportionally to the density, and thus provides a suitable signal-to-noise ratio for much larger distances than the collisionally excited X-ray and EUV radiation. Figure 3 shows a composite image from the 1 August 2008 eclipse in the visible range superimposed to the AIA FOV, beyond which the EUV signal is too low to be detected by that instrument.
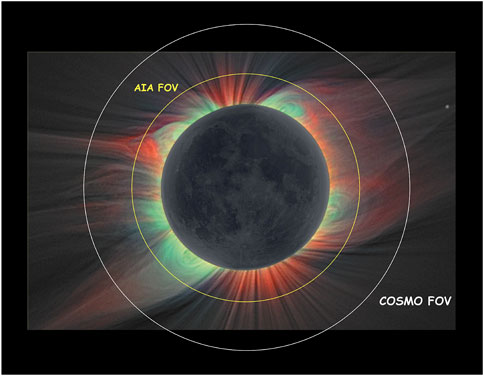
FIGURE 3. Comparison of the field of view of SDO/AIA with the COSMO one. Background eclipse image shows Fe XI (red) and Fe XIV (green) superimposed intensities from the 1 August 2008 eclipse (courtesy of Prof. S. R. Habbal).
4 Gleaning information about the Sun: What do we need and are missing?
Ground based coronagraphic observations of visible and near-IR lines emitted by the solar corona can overcome most of these limitations. First, visible and near-IR spectral lines retain the full diagnostic potential of EUV spectral lines, and through the use of tunable filters they can at the same time be resolved in wavelength and be observed near-simultaneously across the entire FOV. Second, visible/near-IR magnetic dipole lines allow the detection of the effects of the magnetic field on spectral line intensity, polarization, and profiles, allowing for systematic observations of the coronal magnetic field. Third, at large heights visible and near-IR coronal lines are radiation-dominated and thus their intensity decreases with distance from the limb much more slowly than collision-dominated EUV lines, allowing us to extend the instrument’s useful FOV to larger heights (see Figure 3). Using long time series of observations, a single ground based tunable filter coronagraph can provide several hours of continuous measurements of plasma dynamics, magnetism and thermodynamics of the entire corona; the ground based location of the instrument ensures easy maintenance, repairs and upgrades, and prolongs and enhances the operational life of the instrument.
5 A new facility: COSMO large coronagraph
The proposed Coronal Solar Magnetism Observatory (COSMO) facility comprises three telescopes, which together span the solar atmosphere: 1) a large coronagraph (LC) with a 1.5-m aperture to measure the magnetic field, temperature, density, structure and dynamics of the corona with a FOV reaching to 2 solar radii (Figure 3); 2) an instrument for monitoring magnetic fields and plasma properties of the chromosphere and prominences (ChroMag); and 3) a white light K-coronagraph (K-Cor) to measure the density structure and dynamics of the corona and CMEs. K-Cor has been operational since 2013, routinely providing images of the solar corona from 1.05 to 3 solar radii, while ChroMag is currently under construction and will observe the solar chromosphere using polarized light from emission lines.
The central instrument in the COSMO suite is a 1.5 m-aperture Large Coronagraph that will obtain daily measurements of the strength and direction of coronal magnetic fields over a 1o (±2 Rsun) FOV. The line-of-sight strength of coronal magnetic fields can be measured directly through the Zeeman effect observed in the circular polarization of coronal forbidden emission lines. The linear polarization from resonant scattering of photospheric radiation is used to measure the plane-of-sky direction of the magnetic field (azimuth). The LC aperture is driven by the need to collect sufficient photons to achieve the magnetic field sensitivity for the Zeeman measurements. The 1o FOV allows the study of typical large-scale coronal structures (see Figure 3 2). The broad wavelength range is critical to observe many visible and near-IR emission lines formed in the solar corona, which will be spectrally resolved near-simultaneously across the entire FOV by using tunable filters. These spectral lines span a wide range of temperatures and provide information on the plasma thermal structure, density and dynamical status using standard diagnostic techniques. Combination of Doppler LOS velocity with POS speed measurements from a series of images can provide an estimate of the velocity vector, which is particularly useful for CMEs.
A prototype of COSMO, the Upgraded Coronal Multi-channel Polarimeter (UCoMP), a 20-cm ground based coronagraph also working in the visible and near-IR, has been recently built as a pathfinder for COSMO LC. With a much more limited aperture, UCoMP can provide near-simultaneous, spectrally resolved images of the solar corona in coronal spectral lines from Fe X, XI, XIII (2 lines), XV. These lines allow the measurement of line intensity, centroid and width across the entire corona, for a much wider range of distances from the photosphere. Also, the Fe XIII pair of lines allows the measurement of the coronal free electron density, as well as polarimetric measurements of the magnetic field through the determination of Stokes Q and U. Although the nominal FOV reaches 2.0 solar radii, UCoMP’s small aperture does not allow the measured emission to have sufficient signal-to-noise ratios to measure Stokes V, as well as the intensities of most lines beyond 1.5 Rsun but it allows us to experiment at low cost for new filters centered on other coronal spectral lines to explore other diagnostic possibilities. An example of UCoMP observations is shown in Figure 4.
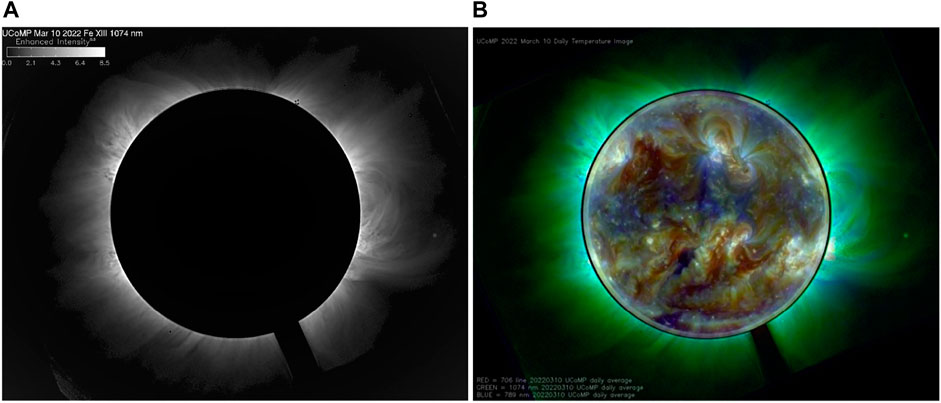
FIGURE 4. Example of UCoMP observations. (A): enhanced intensity image of the solar corona with the Fe XIII 1074 nm line, taken on 10 March 2022. (B): UCOMP daily temperature map obtained with the superposition of daily average images of Fe XV 706 nm (red), Fe XIII 1074 nm (green) and 789 nm (blue) of the solar corona; on the disk, an SDO/AIA multi-channel image has been inserted.
COSMO LC will provide the necessary observing power and necessary sensitivity to extend measurements up to 2 Rsun and spectroscopically image the region of the corona (1.5–2.0 Rsun) where the plasma becomes largely collisionless and spectral signatures of wave-particle interactions can become visible. COSMO LC will benefit from the experience gained with UCoMP, as well as from the filter experimentation the latter will enable, to open a new window in a part of the corona that has never been systematically observed. COSMO LC will provide critical observables to understand the physical processes that heat and accelerate the solar wind, and determine the first stages and the evolution of large scale coronal structures as well as CMEs.
Author contributions
The manuscript was compiled by EL with content and editorial contributions from all co-authors.
Funding
The UCoMP instrument upgrade was funded by NSF grant AGS-1408789. The work of EL is supported by NASA grants 80NSSC20K0185, 80NSSC21K0579, 80NSSC18K1553, 80NSSC18K0647, 80NSSC22K0750, and 80NSSC22K1015, and NSF grant AGS-2229138. TK is supported by the NASA ISFM program. SG, ST, JB, and GdT’s work is supported by the National Center for Atmospheric Research, which is a major facility sponsored by the National Science Foundation under Cooperative Agreement No. 1852977. JZ acknowledges the support from 80NSSC19K0082 and 80NSSC20K1274.
Acknowledgments
A modified version of this paper was submitted to the 2024 Solar and Space Physics Decadal Survey and is published in the associated issue of the Bulletin of the American Astronomical Society.
Conflict of interest
The authors declare that the research was conducted in the absence of any commercial or financial relationships that could be construed as a potential conflict of interest.
Publisher’s note
All claims expressed in this article are solely those of the authors and do not necessarily represent those of their affiliated organizations, or those of the publisher, the editors and the reviewers. Any product that may be evaluated in this article, or claim that may be made by its manufacturer, is not guaranteed or endorsed by the publisher.
References
Boe, B., Habbal, S. R., Druckmueller, M., Land, E., Kourkchi, E., Ding, A., et al. (2018). The first empirical determination of the Fe10+ and Fe13+ freeze-in distances in the solar corona. Astrophys. J. 859, 155.
Brosius, J. W., and White, S. M. (2006). Radio measurements of the height of strong coronal magnetic fields above sunspots at the solar limb. Astrophys. J. 641, L69–L72. doi:10.1086/503774
Brosius, J. W., Landi, E., Cook, J. W., Newmark, J. S., Gopalswamy, N., and Lara, A. (2022). Measurements of three-dimensional coronal magnetic fields from coordinated extreme-ultraviolet and radio observations of a solar active region sunspot. Astrophys. J. 574, 453.
Casini, R., White, S. M., and Judge, P. G. (2017). Magnetic diagnostics of the solar corona: Synthesizing optical and radio techniques. Space Sci. Rev. 210, 145–181. doi:10.1007/s11214-017-0400-6
De Moortel, I., Pascoe, D. J., Wright, A. N., and Hood, A. W. (2016). Transverse, propagating velocity perturbations in solar coronal loops. Plasma Phys. control. Fusion 58, 014001. doi:10.1088/0741-3335/58/1/014001
Del Zanna, G., and Mason, H. E. (2018). Solar UV and X-ray spectral diagnostics. Living Rev. Sol. Phys. 15, 5. doi:10.1007/s41116-018-0015-3
Del Zanna, G., Polito, V., Dudik, J., Testa, P., Mason, H. E., and Dzifkakova, E. (2022). Diagnostics of non-maxwellian electron distributions in solar active regions from Fe XII lines observed by the hinode extreme ultraviolet imaging spectrometer and interface region imaging spectrograph. Astrophys. J. 930, 61. doi:10.3847/1538-4357/ac6174
Fleishman, G. D., Martinez, O. J. C., Landi, E., and Glesener, L. (2022). What aspects of solar flares can be clarified with mm/submm observations? Frontiers.
Kohl, J. L., Noci, G., Cranmer, S. R., and Raymond, J. C. (2006). Ultraviolet spectroscopy of the extended solar corona. Astron. Astrophys. Rev. 13, 31–157. doi:10.1007/s00159-005-0026-7
Landi, E., Hutton, R., Brage, T., and Li, W. (2020). Hinode/EIS measurements of active-region magnetic fields. Astrophys. J., 87.
Lin, H., Kuhn, J. R., and Coulter, R. (2004). Coronal magnetic field measurements. Astrophys. J. 613, L177–L180. doi:10.1086/425217
Mason, J. P., Champberlin, P. C., Seaton, D., Burkepile, J., Colaninno, ., Dissauer, K., et al. (2021). SunCET: The sun coronal ejection tracker concept. J. Space Weather Space Clim. 11, 20. doi:10.1051/swsc/2021004
Phillips, K. J. H., Feldman, U., and Landi, E. (2008). “Cambridge astrophysics series,” in Ultraviolet and X-ray spectroscopy of the solar atmosphere (Cambridge University Press).
Rochus, P., Auchere, F., and Berghmans, D. (2020). The solar orbiter EUI instrument: The extreme ultraviolet imager. Astron. Astroph. 642, A8.
Seaton, D. B., Bewrghmans, D., Nicula, B., Halain, J. P., De Groof, A., Thibert, T., et al. (2013). The SWAP EUV imaging telescope Part I: Instrument overview and pre-flight testing. Sol. Phys. 286, 43–65. doi:10.1007/s11207-012-0114-6
Tomczyk, S., Card, G. L., Darnell, T., Elmore, D. F., Lull, R., Nelson, P. G., et al. (2008). An instrument to measure coronal emission line polarization. Sol. Phys. 247, 411–428. doi:10.1007/s11207-007-9103-6
Varneta, A. I., Antonucci, E., and Marocchi, D. (1994). Manifestation of magnetic reconnection in coronal streamer current sheets. Space Sci. Rev. 70, 299–302. doi:10.1007/bf00777884
Keywords: plasma diagnostics, corona, ground based instruments, imaging spectroscopy, visible radiation
Citation: Landi E, Gibson SE, Tomczyk S, Burkepile J, de Toma G, Zhang J, Schad T, Kucera TA, Reeves KK and Cremades H (2022) Coronal spectral diagnostics: The coronal solar magnetism observatory (COSMO). Front. Astron. Space Sci. 9:1059716. doi: 10.3389/fspas.2022.1059716
Received: 01 October 2022; Accepted: 31 October 2022;
Published: 24 November 2022.
Edited by:
Joseph E Borovsky, Space Science Institute, United StatesReviewed by:
Yingna Su, Purple Mountain Observatory (CAS), ChinaDebi Prasad Choudhary, California State University, United States
Copyright © 2022 Landi, Gibson, Tomczyk, Burkepile, de Toma, Zhang, Schad, Kucera, Reeves and Cremades. This is an open-access article distributed under the terms of the Creative Commons Attribution License (CC BY). The use, distribution or reproduction in other forums is permitted, provided the original author(s) and the copyright owner(s) are credited and that the original publication in this journal is cited, in accordance with accepted academic practice. No use, distribution or reproduction is permitted which does not comply with these terms.
*Correspondence: Enrico Landi, ZWxhbmRpQHVtaWNoLmVkdQ==