- 1Extraterrestrial Physics, Institute of Experimental & Applied Physics, Christian-Albrechts-University Kiel, Kiel, Germany
- 2Institut de Recherche en Astrophysique et Planétologie, UPS, CNES, Université de Toulouse, Toulouse, France
- 3Swedish Institute od Space Phyics, Kiruna, Sweden
- 4Applied Physics Laboratory, Johns Hopkins University, Laurel, MD, United States
- 5Department of Physics, Faculty of Natural Sciences, Space and Atmospheric Physics Group, Imperial College, London, United Kingdom
- 6Department of Mechanical and Aerospace Engineering, Sapienza University of Rome, Rome, Italy
- 7Laboratoire d’astrophysique de Bordeaux, University Bordeaux, Pessac, France
- 8Héliosphère, Exosphéres Planétaires, Plasmas et Interfaces, Laboratoire Atmosphères, Observations Spatiales, Guyancourt, France
- 9Department of Mathematics, Physics and Electrical Engineering, Northumbria University, Newcastle upon Tyne, United Kingdom
- 10Space Physics and Planetary Sciences, Physics Institute, Faculty of Science, University of Bern, Bern, Switzerland
The discovery of a myriad of exoplanets in the past decades has revolutionized the understanding of our place in the Universe. How different are exoplants and do some of them harbor life, just like Earth? To do so, their parent stars must drive a stellar wind and carve what we call astrospheres into the surrounding interstellar medium. Astrospheres are ubiquitous in our immediate neighborhood and show similar structure to our heliosphere. Voyager 1 and 2, Ulysses, Cassini, and IBEX have shown that the interaction between interstellar medium and solar wind is much more complex and involved than previously believed. This stellar-interstellar interaction is key to understand astrospheres and the shielding they provide to the planetary systems they harbor. This article summarizes a whitepaper that was submitted to NASA’s 2023/2024 decadal survey which is being conducted by the US National Academies. It is based in parts on a proposal submitted to the European Space Agency (ESA) in response to its 2021 call for medium-class mission proposals. The whitepaper and this article propose to study the interaction described above in situ at the heliospheric boundaries and to explore the very local interstellar medium beyond. Furthermore, they highlight possible European contributions to a NASA-led Interstellar Probe (ISP).
1 Introduction
A substantial amount of work has been performed in the past decades that has shown that an interstellar probe (ISP) is today a feasible, timely, and compelling mission that would enable humanity’s journey to interstellar space. A 498-page study led by the Johns Hopkins University (JHU)/Applied Physics Laboratory (APL) provides a detailed account of the science goals and questions, the mission concept, scientific and technical implementation, as well as programmatics and concepts for the managements of such a 50-year mission (McNutt et al., 2021). The white paper reported here draws heavily from that study, as well as from white papers submitted previously to the European Space Agency (ESA) (Wimmer-Schweingruber et al., 2009; Wimmer-Schweingruber, 2013; Wimmer-Schweingruber, 2019) in response to its Cosmic Vision and Voyage 2050 calls for proposals, and the STELLA proposal submitted to ESA’s 13 December 2021 call for a medium-size mission opportunity (Barabash and Wimmer-Schweingruber, 2022). In line with the JHU/APL study, STELLA is a proposal for a substantial European contribtion to a NASA-led Interstellar Probe (ISP) that would reach 350 au after 50 years (Brandt et al., 2022). Here we highlight possible European contributions to such a NASA-led mission as well as the science questions that could be addressed by such a contribution.
All aforementioned ISP mission proposals aim at answering fundamental questions about heliophysics which can be summarized as shown in Table 1.

TABLE 1. ISP top-level science questions (McNutt et al., 2021).
In addition to answering te questions posed in Table 1, an ISP would enable planetary science with its fly-by of Jupiter and its moons after the era of the ESA’s Jupiter Icy Moons Explorer (JUICE) mission and to fly by a Kuiper-Belt Object (KBO), very similar to the successful New Horizons example. On its way to the Very Local Interstellar Medium (VLISM), ISP would allow bonus science goals from a variety of scientific disciplines (Section 2.6) and perform a fundamental measurement to test the 1/r2 law of gravitation at a hitherto unverified distance scale (Section 2.5). Thus, an ISP would serve a large scientific community while addressing the top-level questions given in Table 1. In Section 2 we discuss in more detail the science questions which could be addressed by possible European payload contributions to an ISP, some of which are shown in Table 2.
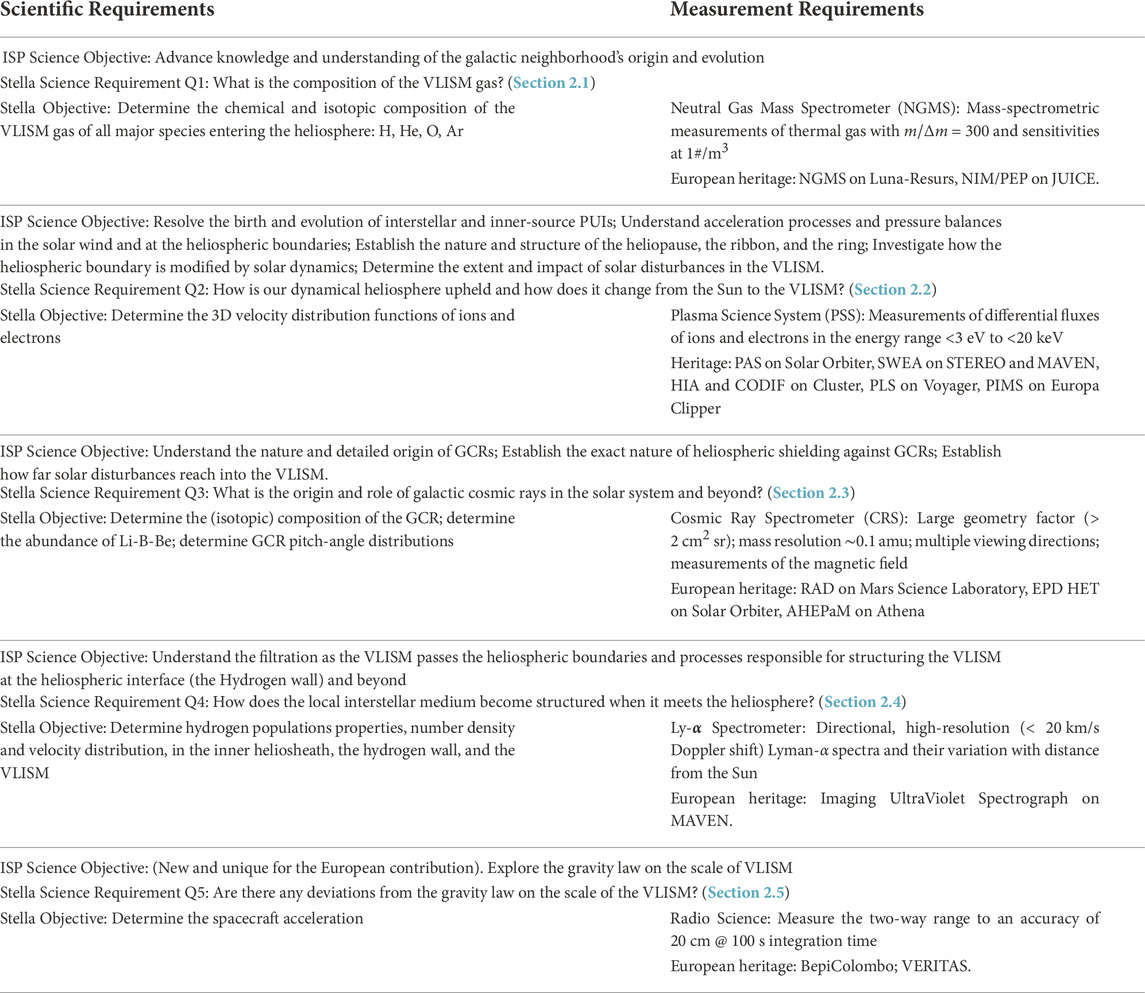
TABLE 2. Notional European contributions to the ISP science questions (McNutt et al., 2021), their measurement requirements, and European heritage. Adapted from Barabash and Wimmer-Schweingruber (2022).
2 Science goals
Today there is a growing awareness of the significance of the physics of the outer heliosphere. Its understanding helps to clarify the structure of our immediate interstellar neighborhood, contributes to the clarification of fundamental astrophysical processes like the acceleration of charged particles at a stellar wind termination shock and beyond, and also sheds light on the question to what extent interstellar-terrestrial relations are important for the environment of Earth and exoplanets.
Even with the growing knowledge about the heliosphere provided by space missions, its global shape and dimensions remain a highly contested topic (Dialynas et al., 2017; Pogorelov et al., 2017; Schwadron and Bzowski, 2018). Most critically, the total plasma pressure in the heliosheath as a function of time and the relative importance of the various plasma populations (in particular at low energies
Attempts to explain consistently the known observations are currently roiled in controversy, with no clear trend towards a consensus (McNutt et al., 2021). The many and diverse in situ and remote-sensing observations obtained to date clearly emphasize the need for a new generation of more comprehensive measurements that are required to understand the global nature of our Sun’s interaction with the local galactic environment. Science requirements informed by the now available observations drive the measurement requirements of an ISP’s in situ and remote-sensing capabilities that would allow to answer the open questions highlighted in Tables 1, 2. The latter exhibits notional, possible European contributions to the ISP science objectives and payload, but such contributions would, of course, need to undergo the usual competititve selection process. For the time being, they may be considered as an incomplete illustration of European capabilities. Other examples of European contributions are a magnetometer or a radio-plasma-wave instrument.
After the exciting in situ observations of the termination shock, heliosheath, and heliopause, both Voyagers have now entered “interstellar space” (Stone et al., 2019). “Interstellar Space” is shown in quotation marks because it is not the pristine VLISM, but rather this space continues to be influenced by the Sun and heliosphere. Because of the various competingdefinitions of VLISM we here clarify that we use the words “pristine VLISM” in the sense of the very local ISM in the vicinity of the local cloud or clouds and reserve the expressions LISM and ISM for what lies beyond. We stress that we do not expect an Interstellar Probe to reach the pristine VLISM in its lifetime, the relevant scales are simply too large even when compared with the distance that could be covered by this mission in its lifetime. We do not expect the Voyagers to last long enough to enter the undisturbed, pristine VLISM which is likely situated way beyond their current locations and remaining lifetime. Nevertheless, the Voyager results allow us to design an ISP to perform measurements of all quantities relevant to characterize and understand the VLISM. Moreover, observations by the Voyagers have underlined the importance of understanding the termination shock and how it influences the structure of the heliosheath (Richardson et al., 2022). While New Horizons is expected to reach the termination shock Stern et al. (2018), it does not carry a magnetometer (Weaver et al., 2008), which is a critical measurement to characterize the termination shock, heliosheath, and VLISM.
Although anchored in heliophysics, ISP, on its journey out of the Solar System, also offers other ground-breaking discoveries across astrophysics and planetary science. Any outward trajectory, dictated by the heliophysics objectives, offers flybys of at least one of the 130 dwarf planets or 4,000 KBOs, to understand the almost unexplored world of the outer Solar System. Remote and in situ dust observations would provide an unprecedented view of the circumsolar dust disk, critical for understanding the formation and evolution not only of our Solar System, but also to understand planetary formation in surprisingly young dust disks. Beyond the zodiacal cloud, the infrared Universe opens up to measure the poorly constrained extragalactic background light providing insight into galactic and stellar evolution since 200 My after the Big Bang. Further examples of such science opportunities are mentioned in Section 2.6.
In the following Sections 2.1, 2.2, 2.3, 2.4, 2.5 we highlight the science questions which could be addressed by a notional European payload (see Table 2) on which the white paper reported here was based. We stress that these notional contributions by no means cover all required measurements for an ISP, nor do they encompas all European capabilities.
2.1 Stella Q1: What is the composition of the VLISM gas?
Early determinations of the VLISM composition were from pickup ions in the solar wind, from anomalous cosmic rays, and from spectroscopic observations towards nearby stars. All these methods are indirect and depend on modelling for the determination of the composition of the VLISM neutral gas. Interstellar Boundary Explorer (IBEX) provided us with first direct measurements of VLISM H, He, O (Möbius et al., 2009), and Ne (Bochsler et al., 2012). However, the absolute values of their abundances in the VLISM are very uncertain because of the strong and variable photoionization in the inner Solar System (Bzowski et al., 2013) and filtration at the heliospheric boundary. Key ratios for planetary evolution studies, e.g., Ne/O and D/H, are not known with sufficient accuracy, and are limited by their small fluxes at 1 AU. Other VLISM species (C, N, Na, Mg, Al, Si, P, S, Ar, Ca, Fe) as well as the isotopic composition of He, O, Ne, have not been measured directly at all. For example, C is not able to penetrate the heliopause because it is fully ionized in the interstellar medium. This also prevents the use of indirect methods. ISP would for the first time directly measure all major neutral gas species in the VLISM.
The abundances of elements and their isotopes in the Solar System present a snapshot in time of the composition of the interstellar medium from which the Sun formed 4.57 Gyr ago. The evolution of interstellar matter that our Solar System encountered on its journey through the Galaxy can be inferred from the present state of the VLISM by measuring these elements and their isotope ratios. Measurements of the isotopic ratios of interstellar neutrals H/D, 3He/4He, and 20Ne/22Ne with high accuracy will constrain our understanding of the chemical evolution of our Galaxy. With the recent realization that the ISM is highly structured and that we are entering a new interstellar cloud now (Linsky et al., 2019), neutral gas measurements at 100s au scales will be critical for assessing the interstellar environment that the heliosphere will encounter on its interstellar trajectory.
A Neutral Gas Mass Spectrometer (NGMS, Table 2) would provide the required composition measurements of the VLISM gas, as well as gradients due to the different ionization and neutralization processes.
2.2 Stella Q2: How is our dynamical heliosphere upheld and how does it change from the sun to the VLISM?
The solar wind expands from the Sun and interacts with the neutral gas in the VLISM to form the heliosphere. In situ observations by the Voyagers and New Horizon, and remote neutral atom measurements by IBEX strongly suggest that turbulence is one of the critical processes acting at the heliospheric interface (Fraternale et al., 2022). This interaction also serves as a model for how stellar winds interact with their VLISM to form astrospheres, but is further complicated by the inflow of neutral VLISM gas. This gas is ionized in the inner heliosphere, thus creating a population of pickup ions (PUIs) which is highly non-thermal and defines the pressure balances in the heliosheath (HS) and at the heliopause (HP) against the flow of the VLISM (Rankin et al., 2019). This crucial role of PUIs in the global solar wind-VLISM interaction can not be studied with Voyager 1 and 2 because they were not equipped to measure PUIs.
While the physics of PUIs within the inner heliosphere has been previously addressed with the Solar Wind Ion Composition Spectrometer (SWICS) on Ulysses and the Advanced Composition Explorer (ACE) (Gloeckler et al., 1992; Geiss et al., 1995; Schwadron et al., 2000; Wimmer-Schweingruber and Bochsler, 2003; Allegrini et al., 2005), the small geometric factor of SWICS did not allow to reconstruct the full 3D velocity distribution function of PUIs which is critical to understand PUI evolution through acceleration and transport processes in the heliosphere. The more modern PLAsma and SupraThermal Ion Composition [PLASTIC, Galvin et al. (2008)] instrument on the Solar TERrestrial Observatory (STEREO) mission or the Proton-Alpha Sensor (PAS) and Heavy Ion Sensor (HIS) on ESA’s Solar Orbiter (Owen et al., 2020) are examples of the kind of required instruments.
PUIs experiencing a secondary charge exchange process with interstellar neutrals become Energetic Neutral Atoms (ENAs). Knowledge of PUI properties is crucial for understanding ENA images of the global heliospheric interaction. IBEX has imaged heliospheric ENAs over a solar cycle which show substantial variations and demonstrate that solar activity drives the global response of the entire heliosphere and its interaction with the VLISM (McComas et al., 2020). But how multi-scale solar wind structures propagate and evolve in the outer heliosphere; how they influence suprathermal and energetic particles; what plasma flows they cause in the heliosheath; and how locations of boundaries change because of pressure pulses, shocks, and waves in the solar wind is not known. Voyager 1 detected shocks and pressure waves beyond the heliosphere which were likely driven by transient solar events (Gurnett et al., 2013). Our understanding of the multi-component, multi-scale plasma physics in the outer boundary layers of the heliosphere and its coupling to the neutral component of the VLISM is extremely limited (See, e.g., Mostafavi et al., 2022, for a review.).
IBEX discovered the ENA ribbon, an enigmatic band of intense ENA fluxes in the sky. The ribbon is an “imprint” of the complex physical processes between charged and neutral particles controlled by the interstellar magnetic field. Understanding its origin will tell us about the fundamental physics of the heliosphere’s boundary, and thus about other astrospheres. The Interstellar Mapping and Acceleration Probe (IMAP, launch 2025) will explore ENAs in much more detail but it will not be able to investigate the micro-physical processes in this complex plasma. A launch of ISP in late summer of 2036 would let it traverse the ribbon and perform in situ particle measurements to establish its origin.
A Plasma Science System (PSS, Table 2) together with NGMS would provide the required measurements of the VLISM plasma and neutral gas populations.
2.3 Stella Q3: What is the origin and role of galactic cosmic rays in the solar system and beyond?
Galactic Cosmic Ray (GCR) anisotropies are sensitive to remote field variations and can therefore be used as an effective remote diagnostic of the field configuration of the heliosphere, and once beyond the heliopause, they provide insight into how solar disturbances can propagate deep into the VLISM (Krimigis et al., 2013; Gurnett et al., 2015; Rankin et al., 2019; Hill et al., 2020). The Voyagers’ cosmic ray instrument had limited look directions, and confounding, species-dependent anisotropies in GCR angular distributions have been reported (Rankin et al., 2019). There is no current consensus on what causes those anisotropies. With its improved instrumentation, ISP would offer a new opportunity to study the nature of GCR anisotropies, GCR shielding by the heliosphere, and the properties of the unshielded GCR spectra in the VLISM, including rare species and isotopes, that could not be observed by the Voyagers. Measurements of rare and unstable cosmic ray isotopes can be used to determine GCR source regions via spallation and direct acceleration, galactic escape rates, and solar modulation (Wiedenbeck et al., 2007). For instance, the abundance of Li, Be, and B at cosmic ray energies is comparable (same order of magnitude) to that of C, N, and O, and thus more than four to six orders of magnitude higher than their relative abundance in the Solar System. Observations of Li-Be-B can offer the capability to distinguish solar from interstellar from mixed plasmas in the HS, HP, and boundary layer(s), and VLISM. Studies of the unobserved species of GCRs in the VLISM are of importance not only to heliophysics and the nature of particle acceleration and consequences of GCRs in the heliosphere, but also to astrophysics and the nature of the Universe itself. Observations of particularly rare GCR isotopes, GCR electrons, and antimatter in the VLISM can even shed light on and further constrain models of the nature of the Big Bang and dark energy. However, because of heliospheric shielding of lower-energy GCRs, the critical observations required to answer such open questions rely on observations of these GCR species in the comparitavely unperturbed VLISM by an ISP.
A Cosmic Ray Spectrometer (CRS, Table 2) together with the PSS would provide the required measurements.
2.4 Stella Q4: How does the local interstellar medium become structured when it meets the heliosphere?
The Local Interstellar Cloud surrounding the heliosphere primarily consists of atomic hydrogen (H). Approaching the heliosphere, interstellar H atoms pile up and form a Hydrogen Wall, found to be a common feature of astrosphere-ISM interaction (Baranov and Malama, 1993; Linsky and Wood, 1996). The heliospheric Hydrogen Wall was detected remotely, however, it was never explored with in situ measurements leaving open questions about its extent, peak density, and 3D structure. Despite being critical for the global heliospheric structure, properties of interstellar H atoms far away from the heliosphere in the LIC and their variations due to ion-neutral coupling at the heliospheric boundary remain largely unknown. Measuring solar Lyman-a (121.567 nm) emission backscattered from interstellar H atoms is a powerful technique to probe interstellar H atoms. The spectral shape of the Lyman-α emission line holds key information on the spatial and velocity distribution of interstellar H and enables to infer momentum exchange between hydrogen and plasma. The study of the Lyman-α line profile in the outer heliosphere allows characterizing the distribution (bulk velocity and temperature) of the hot hydrogen population, which is created by charge exchange in the inner heliosheath. After crossing the heliopause, these same measurements will provide the hydrogen atom distribution in the outer heliosheath and the Hydrogen Wall. As the probe moves away to larger distances the pristine interstellar H population will contribute increasing amounts to the Ly-α line profile thus allowing to derive (e.g., by extrapolation) the true number density, bulk velocity, and temperature in the Local Interstellar Cloud. The combination of in situ measurements of the H and proton density with other species with different ionization states will provide key information about the ionization state and processes in the LISM.
A Ly-α spectrometer (Table 2) would provide the required measurements.
2.5 Stella Q5: Is the 1/r2 law of gravitation valid at scales of hundreds of au?
The laws of gravity are unevenly tested at different spatial scales [Fundamental Physics Roadmap Advisory Team (FPR-AT), 2010]. The most precise knowledge is obtained from Solar System dynamics, where space probes allowed the most accurate tests of general relativity to date. Violations of the 1/r2 law, associated with the Compton wavelength of the graviton, have also been tested precisely in the Solar System. But at short spatial scales (at the atomic level) or beyond the Solar System (
This science question would not require any dedicated payload, but the radio system would be required to provide precise range and range rate measurements (Table 2).
2.6 Stella bonus science opportunities B1–B5
The five science questions discussed above are examples of heliophysics and fundamental physics questions that would be addressed by an ISP. We highlight five additional, bonus science “teasers” (B1–B5) to demonstrate the breadth of ISP. Indeed, this mission would also contribute to astrophysics and planetology.
B1: Infrared emission from dust in the Solar System (Zodiacal light) obscures the extragalactic background light which is the red-shifted remnant of the emission of galaxies formed only a few hundred million years after the Big Bang (Cooray, 2016). Observing this light would constrain models of Galaxy formation, the evolution of cosmic structure, the re-ionization epoch, and allow for searches beyond the standard model (Tyson, 1995).
B2: As the solar wind interacts with planetary magnetospheres, atmospheres, or surfaces, it emits characteristic X-ray emission. An X-ray instrument would thus allow to determine the soft X-ray background in the heliosphere and solar-wind planet interactions.
B3: Acquire Ly-α absorption spectra against known stellar neighbors to map out the neutral hydrogen density structure in the local neighbourhood. The increasing distance of an interstellar probe would gradually build up this 3D information.
B4: The Solar System does not only include the Sun, planets, and asteroids, but also a large number of dwarf planets (a status to which Pluto has been demoted), Kuiper-Belt Objects (KBOs), and Trans-Neptunian Objects (TNOs). It thus comes as no surprise that there are many opportunities for a fly by of such an object. This would dramatically increase our understanding of these objects and of their diversity.
B5: As the ISP retreats from the Solar System its back-looking view of the Solar System would offer a remote observation of a known planetary system as a model for a habitable exo-planetary system.
3 Mission description
Apart from describing important science goals of an ISP, Table 2 also hints at possible European provisions of payload elements (instruments). Obviously, this would have to be subject to further refinement by a joint NASA - ESA science definition team and a competitive process. The instruments mentioned are given to illustrate European expertise and possible contributions. While these instruments have sufficient heritage and already have or will have TRL 6 by 2026, they would have to be qualified for a 50 years (or longer) mission duration. NASA and ESA would have to play a key role in this process.
One of the key European industrial and programmatic contributions proposed in the STELLA proposal to ESA is an upgrade of the European deep space communication facility that would allow the precise range and range-rate measurements of the probe to address STELLA science goal Q5 but would also provide additional downlink of ISP data and thus increase the ISP science return. The facility would be a critical augmentation of the European Deep Space Antennas (DSA) not only for ISP but also for other planned missions, e.g., to the icy giants. Less than a decade after its launch, returning data from ISP would require a receiving station with the equivalent of 4 35-m dishes or more than 16 18-m dishes, i.e., the equivalent of a 75-m dish. The architecture of the array should be studied during an early phase of the mission (0/A). European industries are among the world leaders in the field. mtex antenna technology (Germany) is the sole prime to develop a production-ready design and produce a prototype 18-m antenna for the US National Research Observatory (NRAO) Very Large Array (ngVLA) facility. Thales/Alenia (France/Italy), Schwartz Hautmont (Spain) are heavily involved in the development of the new 35-m DSA antenna.
At the other end of the communication system, i.e., on the ISP spacecraft itself, Europe could also provide the High Gain Antenna (HGA) and communication system which should supply, together with the ground segment, a telemetry rate of at least 200 bps at 350 au and would allow to address STELLA science goal Q5. It would have to be a fully redundant X-band system; optical communication would very likely pose too stringent pointing requirements on the spacecraft. Hardware for this subsystem is mature technology with ample deep space heritage. The antenna complement would include a 5-m High Gain Antenna (HGA), a 0.4-m Medium Gain Antenna (MGA) coaligned with the HGA, and fore and aft Low Gain Antennas (LGAs).
3.1 Maintaining a long-term mission
This 50-year (or longer) mission will require careful handling of mission operations and knowledge transfer:
3.1.1 Mission operations
We envision that NASA would assume the main responsibilities for ISP flight dynamics and operations, and that ESA’s primary responsibility would be to operate the European payloads, and provide a dedicated link to ISP to drastically increase ISP’s science return. ESA would also perform the special operations required by the European Radio Science experiment and could provide additional uplink. The contribution envisaged in the STELLA proposal (Barabash and Wimmer-Schweingruber, 2022) only covers operations for the first 10-year segment of the 50-year nominal life time because it is foreseen to divide the ISP mission up into 10-year segments which would be reviewed individually.
3.1.2 Knowledge transfer
The very long duration of the mission would necessitate the broad involvement of young and mid-career scientists as well as encouraging scientists who were not necessarily involved in the hardware phase to join the mission. We envision that PIs would change for the different phases of the mission.
For each 10-year segment of the mission, all project and instrument teams would be requested to include three categories of their team members:
• Senior members with extensive experience. These would typically be mentors of mid-career and junior scientists and potential PIs.
• Mid-career scientists who lead smaller teams and are in charge of important subsystems or projects.
• Junior scientists who lead individual ISP science investigations.
Through the course of the mission, scientists would flow through these categories to ensure knowledge transfer and succession. ISP as the anchor would enable an entire half-century of scientists to gain mission experience.
4 Technology developments
Of course, this 50-year (or longer) mission also requires significant, although entirely manageable, technology developments, the most noteable of which are highlighted in the following paragraphs. A more complete listing is given in McNutt et al. (2021).
4.1 Propulsion
Previous proposals have included solar sails, nuclear ion propulsion, or electric sails, which are technologically not mature for a flight in the 2030s and put complex constraints on spacecraft implementation. With the increasing availability of Super-Heavy Lift Launch Vehicles (SHLLVs), such as NASA Space Launch Systems (SLS), SpaceX Starship, or BlueOrigin vehicles, higher solar-system escape speeds are now becoming realistic using conventional chemical propulsion. For example, a launch with an SLS Block 2 followed directly by an Atlas Centaur upper stage and a Star-48 BV, all directly after launch, would put an 860-kg spacecraft on a 6-month trajectory to Jupiter. Here, a passive gravity assist would allow an escape speed twice that of the fastest solar-system escaping spacecraft, Voyager 1, of up to 7.2 au/year (McNutt et al., 2022).
4.2 Power
Nuclear power would be unavoidable. NASA is currently developing the Next-Generation Radioisotope Thermoelectric Generator (NextGen RTG) through the Radioisotope Power Systems (RPS) Program at Glenn Research Center. The RPS Program is on track to provide the first flight-qualified NextGen RTG by 2028, well before 2036 when Interstellar Probe would require units. Power supply electronics and distribution units could be reused from New Horizons and Parker Solar Probe (PSP). See Section 5.9 in (McNutt et al., 2021) for more details.
4.3 Reliability
Strategies to the ensure reliabilty of ISP for 50 years (or longer) of operations in space will need to be developed. Our knowledge of how to assess reliability has greatly improved over the past decades and has resulted in missions which have significantly exceeded their planned lifetimes, as is exemplified by the Voyagers, SOHO, Ulysses, and other missions. For instance, studies have shown that parts failures can be more accurately associated with inadequacies in design or improper handling of components or workmanship (see McNutt et al., 2021, Sec. F.2).
4.4 Management
An ISP would also pose a management challenge by necessitating a well-organized and continuous transfer of knowledge from one generation of engineers, scientists, and managers to the next as discussed in Section 3.1.
4.5 Communication
While communication across hundreds of au may be viewed as a technological challenge, the Voyagers have shown that this can be done. Current technology on Earth, paired with spacecraft communication equipment from the 1970s already allow this reliably across more than 150 au. The STELLA proposal to ESA (Barabash and Wimmer-Schweingruber, 2022) and the JHU/APL study (McNutt et al., 2021), as well as the first paragraphs of Section 3 give more details about possible improvements. Nevertheless, investments in communications would improve science return, and also benefit other outer-solar-system missions (e.g., to the giant planets and icy moons).
Investments in the fields highlighted in the previous subsections would significantly enhance humanity’s space-faring capabilities and benefit (Solar System) space science.
4.6 Technology development activities
Apart from obvious technology developments such as the development of the power sources and ensuring the powerful launcher needed for ISP mentioned above, the following technology development activities (TDAs) would be needed for an ISP:
Development and implementation of qualification procedures for missions with nominal lifetimes of 50 years and beyond. This would provide the community with knowledge of designing long-lived space equipment and be helpful for other programs such as Artemis.
Studies of using integrated X/Ka deep space transponders (IDSTs) on an ISP mission. IDSTs will be flown on NASA’s Venus Emissivity, Radio Science, InSAR, Topography, and Spectroscopy (VERITAS) mission as a contribution from the Italian Space Agency and are already at high TRL level. The possible use of Ka-band relies on the pointing accuracy of the spinning spacecraft and requires dedicated studies.
Studies of the deep space communication facility architectures, and of an upgrade of the DSA to provide a dedicated link for ISP: 4 × 35-m dishes or more than 16 18-m dishes, antenna’s location, numbers in each site. The study would also consider the use of the ESA facility for other missions to the icy giants and outer Solar System.
The required TDAs should be kicked off as early as possible to ensure completion by the end of phase 0/A of an ISP mission.
5 International context
An Interstellar Probe with varying names has been proposed to different space agencies. Apart from NASA (McNutt et al., 2021), ESA has commissioned such studies (Lyngvi et al., 2007), and China is also studying an interstellar probe which would reach 100 AU by 2049 to celebrate the 100-th anniversary of modern China. It was discussed in the 639th Academic Symposium of the renowned Xiangshan Science Conference series in October 2018. An international meeting on this topic was held at ISSI in Beijing in November 2019. Numerous mission white papers have been submitted to ESA (Wimmer-Schweingruber et al., 2009; Wimmer-Schweingruber, 2013; Wimmer-Schweingruber, 2019) and laid the ground work for the STELLA mission proposal (Barabash and Wimmer-Schweingruber, 2022). The necessarily long duration and ensuing cost of such a mission strongly suggest a coordinated NASA-ESA effort.
If an ISP is recommended in first rank order of Large Strategic Missions and/or US Congress demands such a mission, then it would be prudent for NASA to establish a Science and Technology Definition Team (STDT) in consultation with ESA.
Data availability statement
The original contributions presented in the study are included in the article/Supplementary Material, further inquiries can be directed to the corresponding author.
Author contributions
RW-S wrote the manuscript based on the inputs of his co-authors and provided information about the energetic particle environment, NA contributed information about the plasma state of the VLISM, SB led the STELLA proposal submitted to ESA in February 2022, PB provided input on US and international efforts for an ISP as well as the plasma and neutral gas measurements, TH contributed overall scientific guidance, especially about the magnetic structure of the heliosphere, LI contributed information about verifying the 1/r2 law of gravitation, BL contributed information about the plasma state of the VLISM, RM provided input on US and international efforts for an ISP, EP, and EQ contributed to the Ly-α questions, RW contributed overall scientific guidance, MW and PW contributed to the neutral gas questions.
Funding
The STELLA proposal (Barabash and Wimmer-Schweingruber, 2022) and the white paper reported here were developed using information from the JHU/APL ISP study (McNutt et al., 2021) which was supported by Task Order 80MSFC18F0139 under NASA Contract NNN06AA01C.
Acknowledgments
We thank the countless individuals who have contributed to our understanding of the heliosphere and the various studies and proposals of such a mission to explore our cosmic neighborhood.
Conflict of interest
The authors declare that the research was conducted in the absence of any commercial or financial relationships that could be construed as a potential conflict of interest.
Publisher’s note
All claims expressed in this article are solely those of the authors and do not necessarily represent those of their affiliated organizations, or those of the publisher, the editors and the reviewers. Any product that may be evaluated in this article, or claim that may be made by its manufacturer, is not guaranteed or endorsed by the publisher.
References
Allegrini, F., Schwadron, N. A., McComas, D. J., Gloeckler, G., and Geiss, J. (2005). Stability of the inner source pickup ions over the solar cycle. J. Geophys. Res. 110, A05105. doi:10.1029/2004JA010847
Barabash, S., and Wimmer-Schweingruber, R. F. (2022). Stella – europe’s contribution to interstellar probe – humanity’s journey to interstellar space. Tech. rep. Kiruna, Sweden: Swedish Institute of Space Physics.
Baranov, V. B., and Malama, Y. G. (1993). Model of the solar wind interaction with the local interstellar medium numerical solution of self-consistent problem. J. Geophys. Res. 98, 15157–15164. doi:10.1029/93JA01171
Bochsler, P., Petersen, L., Möbius, E., Schwadron, N. A., Wurz, P., Scheer, J. A., et al. (2012). Estimation of the neon/oxygen abundance ratio at the heliospheric termination shock and in the local interstellar medium from IBEX observations. Astrophys. J. Suppl. Ser. 198, 13. doi:10.1088/0067-0049/198/2/13
Brandt, P. C., Provornikova, E. A., Cocoros, A., Turner, D., DeMajistre, R., Runyon, K., et al. (2022). Interstellar probe: Humanity’s exploration of the galaxy begins. Acta Astronaut. 199, 364–373. doi:10.1016/j.actaastro.2022.07.011
Bzowski, M., Sokół, J. M., Kubiak, M. A., and Kucharek, H. (2013). Modulation of neutral interstellar He, Ne, O in the heliosphere. Survival probabilities and abundances at IBEX. Astron. Astrophys. 557, A50. doi:10.1051/0004-6361/201321700
Cooray, A. (2016). Extragalactic background light measurements and applications, 3. London, UK: Royal Society Open Science, 150555. doi:10.1098/rsos.150555
Dialynas, K., Krimigis, S. M., Mitchell, D. G., Decker, R. B., and Roelof, E. C. (2017). The bubble-like shape of the heliosphere observed by Voyager and Cassini. Nat. Astron. 1, 0115. doi:10.1038/s41550-017-0115
Fraternale, F., Adhikari, L., Fichtner, H., Kim, T. K., Kleimann, J., Oughton, S., et al. (2022). Turbulence in the outer heliosphere. Space Sci. Rev. 218, 50. doi:10.1007/s11214-022-00914-2
Fundamental Physics Roadmap Advisory Team (FPR-AT) (2010). A Roadmap for fundamental physics in space. Tech. rep. Noordwijk, Netherlands: European Space Agency, ESA. Available at: https://sci.esa.int/science-e/www/object/doc.cfm?fobjectid=47597.
Galli, A., Wurz, P., Schwadron, N. A., Kucharek, H., Möbius, E., Bzowski, M., et al. (2017). The downwind hemisphere of the heliosphere: Eight years of IBEX-lo observations. Astrophys. J. 851, 2. doi:10.3847/1538-4357/aa988f
Galvin, A. B., Kistler, L. M., Popecki, M. A., Farrugia, C. J., Simunac, K. D. C., Ellis, L., et al. (2008). The plasma and suprathermal ion composition (PLASTIC) investigation on the STEREO observatories. Space Sci. Rev. 136, 437–486. doi:10.1007/s11214-007-9296-x
Geiss, J., Gloeckler, G., Fisk, L. A., and von Steiger, R. (1995). C+ pickup ions in the heliosphere and their origin. J. Geophys. Res. 100, 23373–23378. doi:10.1029/95JA03051
Gloeckler, G., Geiss, J., Balsiger, H., Bedini, P., Cain, J. C., Fischer, J., et al. (1992). The solar wind ion composition spectrometer. Astron.& Astrophys. Suppl. 92, 267–289.
Gurnett, D. A., Kurth, W. S., Burlaga, L. F., and Ness, N. F. (2013). In situ observations of interstellar plasma with voyager 1. Science 341, 1489–1492. doi:10.1126/science.1241681
Gurnett, D. A., Kurth, W. S., Stone, E. C., Cummings, A. C., Krimigis, S. M., Decker, R. B., et al. (2015). Precursors to interstellar shocks of solar origin. Astrophys. J. 809, 121. doi:10.1088/0004-637X/809/2/121
Hill, M. E., Allen, R. C., Kollmann, P., Brown, L. E., Decker, R. B., McNutt, J., et al. (2020). Influence of solar disturbances on galactic cosmic rays in the solar wind, heliosheath, and local interstellar medium: Advanced composition explorer, new Horizons, and voyager observations. Astrophys. J. 905, 69. doi:10.3847/1538-4357/abb408
Krimigis, S. M., Decker, R. B., Roelof, E. C., Hill, M. E., Armstrong, T. P., Gloeckler, G., et al. (2013). Search for the exit: Voyager 1 at heliosphere’s border with the galaxy. Science 341, 144–147. doi:10.1126/science.1235721
Linsky, J. L., Redfield, S., and Tilipman, D. (2019). The interface between the outer heliosphere and the inner local ISM: Morphology of the local interstellar cloud, its hydrogen hole, strömgren shells, and 60Fe accretion. Astrophys. J. 886, 41. doi:10.3847/1538-4357/ab498a
Linsky, J. L., and Wood, B. E. (1996). The α centauri line of sight: D/H ratio, physical properties of local interstellar gas, and measurement of heated hydrogen (the “hydrogen wall”) near the heliopause. Astrophys. J. 463, 254–270. doi:10.1086/177238
Lyngvi, A. E., van den Berg, M. L., and Falkner, P. (2007). Study overview of the interstellar heliopause probe. Noordwijk, Netherlands: European Space Agency. Tech. Rep. SCI-A/2006114/IHP, issue 3, rev. 4. Available at: https://sci.esa.int/science-e/www/object/doc.cfm?fobjectid=40926.
McComas, D. J., Bzowski, M., Dayeh, M. A., DeMajistre, R., Funsten, H. O., Janzen, P. H., et al. (2020). Solar cycle of imaging the global heliosphere: Interstellar boundary explorer (IBEX) observations from 2009-2019. Astrophys. J. Suppl. Ser. 248, 26. doi:10.3847/1538-4365/ab8dc2
McNutt, R. L., Paul, M. V., Brandt, P. C., and Kinnison, J. D. (2021). Interstellar probe. Tech. rep. Laurel, MD: Applied Physics Laboratory, Johns Hopkins University.
McNutt, R. L., Wimmer-Schweingruber, R. F., Gruntman, M., Krimigis, S. M., Roelof, E. C., Brandt, P. C., et al. (2022). Interstellar probe - destination: Universe. Acta Astronaut. 196, 13–28. doi:10.1016/j.actaastro.2022.04.001
Möbius, E., Bochsler, P., Bzowski, M., Crew, G. B., Funsten, H. O., Fuselier, S. A., et al. (2009). Direct observations of interstellar H, He, and O by the interstellar boundary explorer. Science 326, 969–971. doi:10.1126/science.1180971
Mostafavi, P., Burlaga, L. F., Cairns, I. H., Fuselier, S. A., Fraternale, F., Gurnett, D. A., et al. (2022). Shocks in the very local interstellar medium. Space Sci. Rev. 218, 27. doi:10.1007/s11214-022-00893-4
Owen, C. J., Bruno, R., Livi, S., Louarn, P., Al Janabi, K., Allegrini, F., et al. (2020). The solar orbiter solar wind analyser (SWA) suite. Astron. Astrophys. 642, A16. doi:10.1051/0004-6361/201937259
Pogorelov, N. V., Fichtner, H., Czechowski, A., Lazarian, A., Lembege, B., le Roux, J. A., et al. (2017). Heliosheath processes and the structure of the heliopause: Modeling energetic particles, cosmic rays, and magnetic fields. Space Sci. Rev. 212, 193–248. doi:10.1007/s11214-017-0354-8
Rankin, J. S., McComas, D. J., Richardson, J. D., and Schwadron, N. A. (2019). Heliosheath properties measured from a voyager 2 to voyager 1 transient. Astrophys. J. 883, 101. doi:10.3847/1538-4357/ab3d9d
Richardson, J. D., Burlaga, L. F., Elliott, H., Kurth, W. S., Liu, Y. D., and von Steiger, R. (2022). Observations of the outer heliosphere, heliosheath, and interstellar medium. Space Sci. Rev. 218, 35. doi:10.1007/s11214-022-00899-y
Schwadron, N. A., Geiss, J., Fisk, L. A., Gloeckler, G., Zurbuchen, T. H., and von Steiger, R. (2000). Inner source distributions: Theoretical interpretation, implications, and evidence for inner source protons. J. Geophys. Res. 105, 7465–7472. doi:10.1029/1999JA000225
Schwadron, N. A., and Bzowski, M. (2018). The heliosphere is not round. Astrophys. J. 862, 11. doi:10.3847/1538-4357/aacbcf
Stern, S. A., Weaver, H. A., Spencer, J. R., and Elliott, H. A. (2018). The new Horizons kuiper belt extended mission. Space Sci. Rev. 214, 77. doi:10.1007/s11214-018-0507-4
Stone, E. C., Cummings, A. C., Heikkila, B. C., and Lal, N. (2019). Cosmic ray measurements from Voyager 2 as it crossed into interstellar space. Nat. Astron. 3, 1013–1018. doi:10.1038/s41550-019-0928-3
Tyson, J. A. (1995). “The optical extragalactic background radiation,” in Extragalactic background radiation meeting. Editors D. Calzetti, M. Livio, and C. Madau (Cambridge, NY: Cambridge University Press), 103–133.
Weaver, H. A., Gibson, W. C., Tapley, M. B., Young, L. A., and Stern, S. A. (2008). Overview of the new Horizons science payload. Space Sci. Rev. 140, 75–91. doi:10.1007/s11214-008-9376-6
Wiedenbeck, M. E., Binns, W. R., Cummings, A. C., Davis, A. J., de Nolfo, G. A., Israel, M. H., et al. (2007). An overview of the origin of galactic cosmic rays as inferred from observations of Heavy ion composition and spectra. Space Sci. Rev. 130, 415–429. doi:10.1007/s11214-007-9198-y
Wimmer-Schweingruber, R. F., and Bochsler, P. (2003). On the origin of inner-source pickup ions. Geophys. Res. Lett. 30, 1077–1080. doi:10.1029/2002GL015218
Wimmer-Schweingruber, R. F. (2013). In situ investigations of the local interstellar medium. Tech. rep. Kiel, Germany: Christian-Albrechts-University.
Wimmer-Schweingruber, R. F. (2019). In situ investigations of the local interstellar medium. Tech. rep. Kiel, Germany: Christian-Albrechts-University.
Wimmer-Schweingruber, R. F., McNutt, R., Schwadron, N. A., Frisch, P. C., Gruntman, M., Wurz, P., et al. (2009). Interstellar heliospheric probe/heliospheric boundary explorer mission—A mission to the outermost boundaries of the solar system. Exp. Astron. (Dordr). 24, 9–46. doi:10.1007/s10686-008-9134-5
Keywords: mission proposal, interstellar probe, space science, solar system, very local interstellar medium, fundamental physics
Citation: Wimmer-Schweingruber RF, André N, Barabash S, Brandt PC, Horbury TS, Iess L, Lavraud B, McNutt RL Jr, Provornikova EA, Quémerais E, Wicks R, Wieser M and Wurz P (2022) STELLA—Potential European contributions to a NASA-led interstellar probe. Front. Astron. Space Sci. 9:1063849. doi: 10.3389/fspas.2022.1063849
Received: 07 October 2022; Accepted: 26 October 2022;
Published: 17 November 2022.
Edited by:
Francesco Malara, University of Calabria, ItalyReviewed by:
Federico Fraternale, University of Alabama in Huntsville, United StatesLennard Fisk, University of Michigan, United States
Copyright © 2022 Wimmer-Schweingruber, André, Barabash, Brandt, Horbury, Iess, Lavraud, McNutt, Provornikova, Quémerais, Wicks, Wieser and Wurz. This is an open-access article distributed under the terms of the Creative Commons Attribution License (CC BY). The use, distribution or reproduction in other forums is permitted, provided the original author(s) and the copyright owner(s) are credited and that the original publication in this journal is cited, in accordance with accepted academic practice. No use, distribution or reproduction is permitted which does not comply with these terms.
*Correspondence: Robert F. Wimmer-Schweingruber, wimmer@physik.uni-kiel.de