- 1Changchun Observatory, National Astronomical Observatories, Chinese Academy of Sciences, Changchun, China
- 2University of Chinese Academy of Sciences, Beijing, China
- 3National Astronomical Observatories, Chinese Academy of Sciences, Beijing, China
- 4Key Laboratory of Space Object and Debris Observation, Chinese Academy of Sciences, Nanjing, China
- 5Yunnan Observatories, Chinese Academy of Sciences, Kunming, China
- 6Key Laboratory for the Structure and Evolution of Celestial Objects, Chinese Academy of Sciences, Kunming, China
In the era of multi-messenger astronomy, gamma-ray bursts (GRBs) with known redshifts, especially high-redshift GRBs, are a powerful tool for studying the structure and evolution of the early Universe. We review the background, the history, and the application of measurement methods of GRB redshifts in astronomy. Based on different observation targets, two measurement methods are mainly introduced. One is on GRB afterglow, the other is on GRB host galaxy. There are various processing methods belonging to measurement methods based on afterglow, including spectral measurement method of afterglow and afterglow spectral energy distribution fitting method with improved methods. There are also numerous measurement methods based on host galaxy, such as spectral measurement method of host galaxy, template matching method of host galaxy, some automatic spectroscopic redshift measurement methods, and machine learning methods. We subsequently introduce the principles, effects, and performance of these methods. We enumerate several detection and measurement instruments, which have been used in observation. The characteristics, advantages, disadvantages, and applicability of the GRB redshift measurement methods are summarized and analyzed. Furthermore, we provide a data set of 611 GRBs with measured redshift. The data set has been collected since 1997. Analysis and statistics are presented based on this data set. We summarize the characteristics of GRBs such as location, time, and accuracy. Finally, we introduce Space-based multi-band astronomical Variable Objects Monitor (SVOM) mission dedicated to searching high redshift GRBs. We also introduce the application prospect of various redshift measurement methods in SVOM mission.
1 Introduction
In the era of multi-messenger astronomy, the redshifts of gamma-ray bursts (GRBs) have been widely used in many research fields of astronomy because they can be observed up to z ≈ 8–9 (Salvaterra et al., 2009; Cucchiara et al., 2011). We note that their redshifts distribution has a peak at z ∼ 2 (Coward et al., 2013) which approximately corresponds to the distance of the farthest SNe-Ia ever detected (Rodney et al., 2015). Therefore, today we can state, without fear of contradiction, that GRBs have become an important tool for the study of cosmology as a whole (Salvaterra, 2015). At present, GRB redshift shows its importance in the study of cosmological parameters (Amati et al., 2008; Amati and Della Valle, 2013; Izzo et al., 2015; Moresco et al., 2022), dark energy with GRBs (Muccino et al., 2021), core-collapse supernovae (SNe) and GRB connection (Woosley and Bloom, 2006; Kelly et al., 2008), SNe emerging from the lightcurve afterglows (Della Valle et al., 2006; Melandri et al., 2019), GRBs hosts (Modjaz et al., 2008; Fruchter et al., 2006; Savaglio et al., 2009).
Redshifts are used to derive GRB parameters, such as Ep,i, Eiso, etc. At present, the correlations of GRB parameters are often used to measure cosmological parameters ΩM and ΩΛ. The results are high-precision and independent by parameters of other cosmological detectors such as SN Ia. Amati and Della Valle (2013) have measured ΩM with Ep,i − Eiso correlation and obtained (for a flat Universe) that the ΩM is in the range 0.14–0.57. The same analysis carried out on a larger sample, found ΩM ∼ 0.3 (Amati and Della Valle, 2013), which is consistent with
GRBs are suitable for studying the possible evolution of the equation from the state of the Universe when we consider the intermediate redshift case. The parameter w(z) of dark energy will be affected by GRB redshift. According to GRB Hubble diagram, the evolution of w(z) has different piecewise formulae in different redshift intervals. When z ≤ 1.2, Muccino et al. (2021) found that w = −1, and the deviation of w(z) was within 1σ. With the increasing of z, the value of w(z) is better determined, but w(z) seems to have a strong deviation more than 2σ.
The GRB redshifts and light curves of afterglows are helpful to study the relationship between SN and GRB. By studying the GRB and SN at different redshifts, it is found that not all SNe connect with GRBs (Woosley and Bloom, 2006). It is predicted that SN associated with GRB has basically no optical flux at z > 1.2. For example, the GRB 050525A has the redshift of spectrum z = 0.606. The shape and fluxes of the light curve confirmed that SN 2005nc was formed in the late afterglow of GRB 050525A (Della Valle et al., 2006). GRB 171010A and SN 2017htp (Melandri et al., 2019) have the same redshift at z = 0.33. This is another example that SN is connected with GRB.
GRB redshifts are helpful to study the physical characteristics of the host population. The spectra of GRB host galaxies with different redshifts are studied, and the characteristics of chemical abundance are obtained (Modjaz et al., 2008). Long GRBs (LGRBs) are more concentrated in the bright regions of the host galaxy than Core Collapse SNe (Fruchter et al., 2006) and seem to share the same locations as SNe-Ic (Kelly et al., 2008). In addition, the host galaxies of LGRBs are much dimmer and more irregular than those of core-collapse supernovae. The results show that LGRBs are related to the massive stars, and may be confine to chemical evolution. Most of the high redshift LGRB hosts are irregular with no bulges (Kelly et al., 2008). The optical-near infrared (NIR) photometry and spectroscopy are used to deduce the star mass, star formation rates (SFRs), dust extinction, and metal abundance of GRB host galaxy (Savaglio et al., 2009).
Some GRB detection instruments are working or being built, such as Gamma-Ray Burst Optical and Near-Infrared Detector (GROND) (Greiner et al., 2008), Swift (Gehrels et al., 2004), Fermi Gamma Ray Space Telescope (Atwood et al., 2009; GLAST Facility Science Team et al., 1999), Space-based multi-band astronomical Variable Objects Monitor (SVOM) (Wei et al., 2016) and so on.
In Section 2, we introduce the history of GRB redshift measurement. The redshift measurements of GRB 970228 and GRB 970508 (the first and second GRBs with known redshift) are emphatically reviewed. In Section 3, we mainly summarize two kinds of common measurement methods of GRB redshift, including the measurement methods based on afterglow and the measurement methods based on host galaxy. The principles, advantages, disadvantages of different methods are introduced. In Section 4, we gather 611 GRBs with redshifts since 1997. We make a statistical analysis of the data, and summarize the characteristics of GRBs, such as location, time and accuracy. We verify the application of different methods introduced above. The advantages and disadvantages are also been presented. In Section 5, we present the summary in this review. We prospect a future space mission SVOM and new GRB redshift measurement methods.
2 The history of GRB redshift measurement
The GRB redshift measurement began in 1997. On 28 February 1997, BeppoSAX satellites detected GRB 970228 and its X-ray afterglow (Costa et al., 1997; Sahu et al., 1997). The optical afterglow of GRB 970228 was also observed to obtain photometric and spectroscopic data by William Herschel Telescope (WHT), Bologna University Telescope (BUT), Rome Astro Physical Observatory (ROA), and other telescopes (Van Paradijs et al., 1997; Galama et al., 1997). The observed photometric data and images are used for theoretical interpretation and modeling analysis (Wijers et al., 1997). Moreover, an extended nebula region was found and determined as the host of GRB 970228 (Galama et al., 1997; Fruchter et al., 1999). Keck II telescope with a low-resolution spectrometer was used to observe host galaxy of GRB 970228 several times. There are two strong emission lines, which are identified as [O II] λ3727 and [O III] λ5007, and a weak emission line [Ne III] λ3869 in the spectrum of the host galaxy. However, the low resolution of the instrument makes it impossible to distinguish the double peaks of [O II] λ3727. The strong OH line in the night sky also interferes with the measurement of Hβ λ4861, [O III] λ4959 and the higher Balmer line. Despite the influence of the above factors, the redshift of GRB 970228 can still be derived as z = 0.695 ± 0.003 by the spectral measurement method of host galaxy. The redshift confirms GRBs lie at cosmological distance (Bloom et al., 2001a).
On 8 May 1997, BeppoSAX satellite detected GRB 970508 (Metzger et al., 1997b; Sahu et al., 1997). Subsequently, its multi-waveband afterglow was observed, and relatively complete afterglow data were derived (Reichart, 1998). Metzger et al. (1997b) estimated the redshift range by spectral measurement from optical afterglow. Spectrum of optical afterglow OT J065349+79163 were obtained using Keck II 10-m telescope with low-resolution imaging spectrometer. The spectrum contains eight strong absorption lines of Fe II, Mg II and Mg I systems. By calculating the independent redshift for each absorption line, and considering the interference of gas clouds, a strict lower limit for the redshift of the source was set as z ≥ 0.835. An approximate upper limit (z ≤ 2.3) was set for the characteristic that no obvious Lyman-α absorption line in the spectrum. To sum up, the redshift range of GRB 970508 is 0.835 ≤ z ≤ 2.3. Reichart (1998) used the photometric data of GRB 970508 optical afterglow to fit the optical spectral energy distribution SED, and used χ2 fitting to get the redshift
Subsequently, more and more GRBs with redshifts have been measured, such as GRB 970828 at z = 0.9579 (Djorgovski et al., 2001), GRB 971214 at z = 3.42 (Kulkarni et al., 1998), GRB 980326 at z ∼ 1 (Bloom et al., 1999), etc. After the Swift satellite was launched in 2004, the number of measured GRBs redshifts greatly increased (Lin, 2009). High redshift GRBs can be detected. At present, GRB redshift ranges from z = 0.0085 (GRB 980425) (Galama et al., 1998) to z ∼ 9.4 (GRB 090429B) (Cucchiara et al., 2011). Especially, a few GRBs with z ≥ 6 have been detected, such as GRB 080913 at z = 6.7 (Greiner et al., 2009a), GRB 090423 at z ∼ 8.2 (Tanvir et al., 2009), GRB 120521C at z ∼ 6 (Laskar et al., 2014), GRB 120923A at z ∼7.8 (Tanvir et al., 2018). High-redshift GRBs are considered as a new and powerful tool to explore the early Universe (Wei et al., 2015). As LGRBs are related to the death of massive stars. They can be born in the interstellar matter (ISM) of primordial galaxies, and unique probes in the re-ionization era (Heintz et al., 2019; Tanvir et al., 2019; Saccardi et al., 2023). In principle, GRB afterglow can be detected at z ∼ 20 (Ciardi and Loeb, 2000; Gou et al., 2004). The early Universe with z = 6–20 is the crucial period in cosmology at present (Wei et al., 2015).
3 Measurement methods of GRB redshift
Based on different observation targets, two types of measurement methods are mainly introduced. One is on GRB afterglow, the other is on GRB host galaxy. Besides, we also introduce a pseudo-redshift estimation method without redshift measurement.
Based on the principles and characteristics of different measurement methods summarized in Section 3.1–3.3, we summarize the applications of different measurement methods. We also present a comparison of the advantages and disadvantages of each method in Table 1.
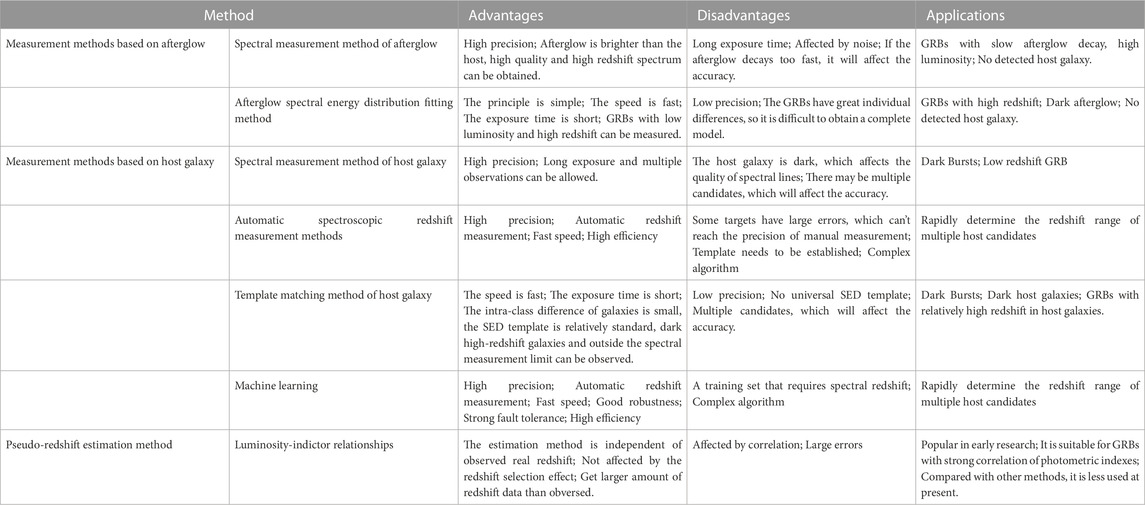
TABLE 1. Advantages, disadvantages and applicability of different measurement methods of GRB redshift
3.1 Measurement methods based on afterglow
The GRB prompt emission duration is extremely short, usually between a few milliseconds and a few minutes. GRB afterglow is occurring later than prompt emission and lasts longer time, so is beneficial for target tracking and observation. Among them, X-ray and optical afterglow emission can last for several days to several weeks, and radio afterglow radiation can last for several years (Zhang, 2007). At present, the afterglows used to measure GRB redshifts are generally in optical, NIR and ultraviolet bands. In the BeppoSAX era, GRB redshifts were measured by the emission lines of X-ray afterglow (Antonelli et al., 2000). In principle, Swift/XRT can be used to measure the redshift with Fe line emission (Burrows et al., 2003). However, Swift never measured the redshift by using the X-ray emission line in observation.
The advantage of afterglow measurement is that afterglow is usually brighter than the host galaxy (Curran et al., 2008). The spectrum and photometry data of afterglow can be obtained. Compared with galaxy optical lines, many prominent absorption lines in the afterglow are bluer. Thus, GRB afterglow at higher redshift can be measured in optical spectroscopy of afterglows. For example, Lyman-α absorption line in GRB afterglow is one of the most important features for redshifts measurement in the case of z > 2. In the case of z ∼ 3, there are many prominent interstellar absorption lines of Mg II, Mg I, Ca II, Fe II often seen in optical spectrum. These absorption lines have rest wavelengths in the 2,000–4,000
3.1.1 Spectral measurement method of afterglow
GRB Redshift measurement usually relies on optical and NIR spectroscopy in the medium and large telescopes (Tanvir and Jakobsson, 2007). The observation spectrum is matched with laboratory spectrum. Usually, GRB optical afterglow do not show emission lines.1 We can compare one observed line of the spectrum to the line in the laboratory spectrum, and the weighted average operation is performed to derive spectroscopic redshift value of the target. The method was applied in GRB 060714 (z = 2.711) (Fynbo et al., 2009; Jakobsson et al., 2006a), GRB 080210 (z = 2.6419) (Fynbo et al., 2009), GRB 210905A (z = 6.312) (Rossi et al., 2022).
The accuracy of the measured redshift is high, up to about 10–4. However, the signal-to-noise ratio of absorption line can affect the accuracy of spectroscopic redshift. The optical afterglow is too fast to have a sufficient bright spectrum. Due to some optical afterglow decaying in a short time, and the brightness is insufficient. Thus, the spectrum with strong absorption may not be obtained.
Spectral measurement method of afterglow is suitable for relatively bright GRBs with slow delay. In fact, as long as one GRB has bright afterglow, when a clear spectrum can be obtained, the very high redshift of target can be measured. For example, GRB 090423 has a high redshift of z ∼ 8 (Salvaterra et al., 2009; Tanvir et al., 2009; Oates and Cummings, 2009). Due to the bright near-infrared afterglow and no counterpart at optical wavelengths, Tanvir et al. (2009) used the ISAAC spectroscopy and VLT spectroscopy by spectral measurement method of afterglow, as well as the photometric data to derive the redshift of
The common instruments for measuring afterglow spectrum include Keck, VLT, GTC and so on. Due to the limitation of the atmosphere, the occurrence time and the position of the source in the sky, in a few cases, the afterglow spectrum can be obtained by using ground near-ultraviolet equipment such as VLT-UVES(Fiore et al., 2005; Vreeswijk et al., 2007). Ultraviolet afterglow can also be detected by Swift/UVOT (Kuin et al., 2009) to measure redshift.
3.1.2 Afterglow spectral energy distribution fitting method (photometric redshift measurement of afterglow)
We used spectral energy distribution (SED) fitting method of GRB afterglow to obtain photometric redshift. The medium and small aperture telescopes are used to perform photometric observations of the afterglow in multi-bands. Then SED fitting method is used to estimate the photometric redshift of target. Some templates are necessary for SED fitting method. The synthetic template is compared with the observed data, so that it can be reasonably extrapolated to the unmeasured redshift range. When χ2 is the smallest, the redshift of target is considered (Bolzonella et al., 2000). X-ray and optical afterglows are usually used, and the SED shape depends on cooling frequency (vc), optical frequency (vo) and X-ray frequency (vx) (Japelj et al., 2015; Zaninoni et al., 2013). The SED includes two prominent features, Lyman-α and Lyman Break at 121.5 (1 + z) nm and 91.2 (1 + z) nm respectively, and GRB redshift measurement mainly depends on them (Krühler et al., 2011b).
When vc < vo or vc > vx, the SED can be described with a single power-law as Eq. 1
Where v is the observed frequency, βO,X the spectral index and F0 the normalization.
And, when vo < vc < vx, the SED can be described with a broken power-law as Eq. 2
Where Δβ = βX − βO.
At present, Hyperz, New Hyperz, etc., are usually used to fit SED (Cucchiara et al., 2011; Afonso et al., 2011; Zaninoni et al., 2013; Rossi et al., 2008). The dust extinction laws in the Milky Way (MW), Small Magellanic Clouds (SMC) and Large Magellanic Clouds (LMC) are added to the synthetic template. This method was applied in GRB 050814 (z = 5.3 ± 0.3) (Jakobsson et al., 2006b), GRB 070802
In order to make the characteristics of the synthetic template approach to the real afterglow, some improved SED fitting methods are proposed. Curran et al. (2008) simulated the absorption effect caused by Lyman forest and host extinction, and used both a genetic algorithm-based routine and Monte Carlo error analysis to simultaneously fit the parameters of interest. Japelj et al. (2015) corrected the MW extinction laws and photoelectric absorption. Littlejohns et al. (2014) increased the host dust extinction and the neutral hydrogen effect along the line of sight. Krühler et al. (2011b) considered several systematic uncertainties, including the uncertainty in the dust redding properties, the opacity of the Lyman-α forest, and the strength of the Damped Lyman-α Absorber (DLA) associated with the GRB. All the above methods are reliably to derive the GRB photometric redshift. The cases are GRB 050904 (z = 6.61 ± 0.14) (Curran et al., 2008), GRB 100905A
Compared to spectroscopic redshift, the accuracy of photometric redshift is lower. Greiner et al. (2008) proposed that the error of GRB photometric redshift is in Δz ∼ 0.3–0.5. Krühler et al. (2011b) proposed that the best result is
Each GRB has certain individual differences. Thus, a standard template is difficult to meet the characteristics of all GRBs. However, photometric measurement is suitable for relatively optical-NIR GRB afterglow.
Some common telescopes for photometric measurements are GROND, Robotic Optical Transient Search Experiment (ROTSE-III), Rapid Eye Mount telescope (REM). In addition, Swift/UVOT are also perform for the photometric measurement. When Lyman-α and Lyman-break pass into the filter ranges, accurate GRB photometric redshift can be derived. In other words, with different redshifts, Lyman features enter different filters. For example, redshift sensitivity to Lyman-α cutoff between UVW2 band (central wavelength λc = 188 nm) and UVM2 band (λc = 217 nm) is z ∼ 1.3, between UVM2 band and UVW1 band (λc = 251 nm) is z ∼ 1.7, between UVM1 band and U band (λc = 345 nm) is z ∼ 2.3, between U band and B band (λc = 439 nm) is z ∼ 3.2, between B band and V band (λc = 544 nm) is z ∼ 3.5 (Krühler et al., 2011b; Roming et al., 2005). 2 Lyman-break falls from g’ band (λc = 468.6 nm) to z’ band (λc = 893.1 nm) at 3.5 < z < 6.5, and from z’ band to J band (λc = 1.25 μm) at z ∼ 8. Afterglows are only detected in H band (λc = 1.65 μm) or Ks band (λc = 2.16 μm) at z ∼ 10 (Krühler et al., 2011b; Greiner et al., 2008; Skrutskie et al., 2006; Abazajian et al., 2009). 3
It appears that targets with different redshift ranges can be measured using different filter bands. The measurable redshift range of Swift/UVOT is 0.5 < z < 3.5 (Kuin et al., 2009), covering the 170–600 nm wavelength, and the measurable redshift range of GROND is 3.5 ≤ z ≤ 13 (Greiner et al., 2008), covering the 360–2,300 nm wavelength. Therefore, multiple devices are often used for combined measurement.
3.2 Measurement methods based on host galaxy
Host galaxy provides important information on the GRB environment. GRB host has the individual differences. For example, the host of GRB 970228 is essentially a face-on late-type blue dwarf galaxy (Bloom et al., 2002), GRB 970508 is in a compact, elongated and blue galaxy (Bloom et al., 2002), GRB 980425 is suspected to be in a nearby spiral galaxy (Conselice et al., 2005), the host galaxy of GRB 981226 appears to have a doubly nucleated morphology (Bloom et al., 2002), GRB 000418 is in a compact galaxy (Bloom et al., 2002).
By the statistical analysis, GRB hosts, which have been observed, are usually young, blue and low-redshift, in addition to low mass and low metallicity (Savaglio et al., 2009) (Perley et al., 2016b). But there are also a few GRBs, especially Dark Bursts, found in massive, metal-rich and high-redshift galaxies (Krühler et al., 2012; Perley et al., 2016b). Due to the correlation between mass and metallicity, GRB host galaxies have lower luminosity and smaller sizes than typical galaxies (Le Floc’h et al., 2003; Fruchter et al., 2006). Bloom et al. (2002) found that the hosts are compact and blue with high central surface brightness, when GRBs are found nearby their host centers. Svensson et al. (2010) found that GRB hosts are always fainter than those of SN counterparts, due to the low metallicity of the GRB host. Moreover, GRB hosts are irregular, small, low mass with more actively star forming. Perley et al. (2016b) found that NIR luminosities of hosts are rapidly increase in low redshift range (0.5 < z < 1.5). However, they are almost unchanged in mid-high redshift (1.5 < z < 5). The low-redshift hosts are fainter than tracers with star formation uniformly, and the mid-high redshift hosts are similar to tracers. At low redshift, there is almost no GRB in massive galaxies.
Short GRBs (SGRBs) originate from the mergers of compact objects, such as binary neutron stars mergers and neutron star-black holes mergers (Fruchter et al., 2006; Nugent et al., 2022). About 84% of SGRB hosts are star-forming galaxies (Nugent et al., 2022). The hosts of SGRB have SFRs consistent with their stellar masses, which is about 1.44 M⊙yr−1. They have usually a wide age range
LGRBs are usually found in extremely blue galaxies with strong emission lines, indicating that this is an apparent abundance of young and massive stars (Fruchter et al., 2006). LGRB hosts are mainly irregular galaxies with low mass, and they have relatively higher redshift median than SGRB hosts (Lyman et al., 2017). Only a few LGRBs are found in spiral galaxies (Fruchter et al., 2006; Conselice et al., 2005). LGRB hosts with low redshifts have higher concentration and lower luminosity than high redshift hosts (Lyman et al., 2017). LGRBs are centrally concentrated, strongly biased towards the brighter regions in hosts. It is found that LGRB hosts are fainter than SFR-weighted field galaxy populations at the same redshift.
The advantage of host galaxy redshift measurement is that hosts have constant luminosity. Unlike the rapidly fading afterglow, the GRB host galaxy can be detected by increasing the exposure time, so that we can obtain more accurate spectrum and photometric data. Host galaxy can be detected many times in a long-time range, reducing the measurement error. At low redshift, Lyman-break may not enter into the photometric bands of UVOT, so the spectral features of afterglow are not clear. The host galaxy measurement is more suitable for low redshift GRB. For “Dark Burst”, where optical afterglow is hardly observed (O’Connor et al., 2022), measurement methods based on host galaxy are important tools to derive redshift.
Some GRBs host galaxies are failed to be detected. It is because some GRBs were kick out from the host galaxies before the bursts (Bloom et al., 2002), or the GRBs have a high redshift and the host galaxy are too dark to be detected. Several candidate galaxies are near the GRB position, so it is difficulty to identify host. In addition, due to the influence of “Redshift Desert”, the lack of strong emission lines affects the measurement of GRB with z = 1–2 (Fiore et al., 2007).
3.2.1 Spectral measurement method of host galaxy
The principle of host galaxy spectral measurement is the same as the normal galaxy. By comparing the observed lines with spectral lines in the laboratory, the spectroscopic redshift of GRB can be derived. GRB hosts are usually star-forming galaxies, and they show the strong emission lines (Jakobsson et al., 2012; Krühler et al., 2015), such as [O II] λ3727, Hβ [OIII] λ5007 and Hα, in addition to weak emission lines [Ne III] [NIII], etc.Krühler et al. (2015) build a comprehensive sample of GRB host emission line spectrum. In the spectra, the superposition fitting of the Gaussian function to the data is effective to get the fluxes of strong emission lines. For weak emission lines, it is necessary to tie the Gaussian centroid and linewidth to those of strong lines. The method was applied in GRB 050714B (z = 2.4383) (Krühler et al., 2015), GRB 060923B (z = 1.5094) (Krühler et al., 2015), GRB 080905A (z = 0.1218) (Rowlinson et al., 2010), GRB 101224A (z = 0.4536) (O’Connor et al., 2022), GRB 180805B (z = 0.6609) (O’Connor et al., 2022), GRB 211211A (z = 0.0763) (Rastinejad et al., 2022).
Spectral measurement method of host galaxy has high accuracy. GRB host has constant luminosity. Unlike the rapidly fading afterglow, they can be observed with a high-precision spectrometer for long exposure time. However, some host galaxies are faint, so that we cannot detected the spectral lines with high signal-to-noise ratio. Moreover, some GRB host galaxies at high redshift are not detected. Therefore, spectral measurement method of host galaxy is suitable for low redshift GRBs. We can also use this method to detect “Dark Burst” redshift. Some large telescopes, such as VLT/X-shooter, Keck, Magellan, are used for this method.
3.2.2 Automatic spectroscopic redshift measurement methods
Some GRB host galaxies are nearby galaxies. They are blue, low mass and low metallicity. Almost all the observation techniques and data processing techniques for studying other distant galaxies can be used to GRB host galaxies (Antonelli et al., 2000). At present, astronomers manually compare the observed spectral lines to the spectral lines in the laboratory. This way is inefficient.
By Automatic spectroscopic redshift measurement methods, we can automatically measure, identify and verify spectral lines, and use template matching method to derive redshift. There are many methods we can consider adopting. They are cross-correlation (Tonry and Davis, 1979), PCAZ (Glazebrook et al., 1998), pseudo-triangle (Qiu et al., 2002), wavelet technique to measure spectral lines and identify Balmer jump points (Luo and Zhao, 2001), density estimation (Duan et al., 2005), Hough transform (Zhou et al., 2000), Bayesian convolution network (Xu et al., 2006a), similarity measurement (Liu et al., 2008), nonlinear dimension reduction (Xu et al., 2006b), Parzen window (Tu et al., 2012), multi-resolution fusion distance method (Pan et al., 2016).
Compared with the number of normal galaxies, the number of GRB hosts is much less, and they are spread over the sky and randomly distributed. Therefore, it is hard to observe one GRB host from astronomy sky surveys. But automated algorithms still have some advantages. Automated algorithms may improve efficiency compared to traditional processing methods. It was presented that the automatic spectroscopic redshift measurement methods have high accuracy. The 97% goal can get the same result as manual processing (Zhu et al., 2005). These methods have fast processing speed, and can automatically process low signal-noise-ratio spectrum (Pan et al., 2016; Zhu et al., 2005). When GRB host is identified, the automatic algorithm can rapidly and accurately measure the redshift. Moreover, the automatic algorithm can quickly determine the redshift interval of each candidate and make a rough classification when the GRBs have multiple candidate hosts. Thus, one or more unqualified candidates can be quickly eliminated and the observation cost can be reduced.
3.2.3 Template matching method of host galaxy
Template matching method of host galaxy can measure the photometric redshift of target by SED fitting. Compared with GRB afterglow, the physical model of host galaxy is more complex, which should have considered isochron, star spectrum, initial mass function (IMF), star formation history, and chemical evolution (Johnson et al., 2021). At present, some popular codes for SED fitting are GALAXEV (Bruzual and Charlot, 2003), PGase (Maraston, 2005), FSPS (Conroy et al., 2009), BPASS (Eldridge et al., 2017). We can compare the template with the observation data. When the likelihood function is minimum, the photometric redshift is derived. Some Matching methods are Bayes (Salim et al., 2007), Hyperz (Bolzonella et al., 2000), EAZY (Acquaviva et al., 2015), CIGALE (Burgarella et al., 2005), MAGPHYS (Cunha et al., 2008), MCMC (Acquaviva et al., 2012), GalMC (Acquaviva et al., 2011), BEAGLE (Chevallard and Charlot, 2016), BAGPIPES (Carnall et al., 2018), LePHARE (Gupta et al., 2021), Prospector (Johnson et al., 2021). The method was applied in GRB 000214
At present, there is no universal SED template due to the diverse features of galaxies (Gupta et al., 2022). Due to the uncertainty of photometric measurement, the accuracy of template matching method of host galaxy is relatively low. However, some GRB host galaxy are faint, so absorption lines and emission lines cannot be distinguished in some galaxy spectra. Photometric measurement is particularly useful for observing faint and relatively high redshift galaxies.
3.2.4 Machine learning
As long as the host galaxy can be detected, the redshift measurement methods of ordinary galaxies can be applied to the GRB afterglow or host galaxy. Besides template matching method, training set methods are also very common. Training set methods usually use machine learning technology to estimate redshift, which is based on statistical theory. These methods need a training data set, which contains samples of data or images with both spectroscopic and photometric redshifts. Redshift without spectroscopic measurement can be estimated by building a fitting model based on spectrum and photometric data in the training data set. Some typical methods are Polynomial Function Fitting (Connolly et al., 1995), Bayesian model (Benítez, 2000), Support Vector Machine (Wadadekar, 2005), K-Nearest Neighbor (Beck et al., 2016), Kernel Regression (Wang et al., 2008), Random Forest (Carrasco and Brunner, 2013), Active Learning (Han et al., 2015), Artificial Neural Network (Firth et al., 2003) and so on. The latest methods are Ensemble Learning (Cunha and Humphrey, 2022), Deep Capsule Network (Dey et al., 2022) and so on.
Compared to traditional processing methods, machine learning has the advantage of high efficiency. But the sample size of GRB hosts is far smaller than that of the normal galaxies. However, machine learning methods can still be useful in combination with other redshift estimation methods to improve the accuracy and speed of the analysis.
3.3 Pseudo-redshift estimation method
Besides the methods mentioned in section 3.1 and 3.2, pseudo-redshift can also be estimated according to the luminosity-indictor relationships, which contain some GRB observed features such as spectral peak energy, spectral complexity, light variation, afterglow correlation, etc (Tan et al., 2013). This redshift estimation method was popularly used in the Compton BATSE era (Lloyd-Ronning et al., 2002). The advantage of this method is that it is completely independent of real redshift measurements in section 3.1 and 3.2, so Pseudo-redshift estimation cannot be affected by redshift selection effects such as redshift desert. Moreover, it can provide a larger amount of redshift data. However, the accuracy of the estimation depends on the tightness of the correlations between the observed features and redshift, and it may not be reliable for all GRBs (Guo, 2020). The method was applied in GRB 080514B (z = 1.76 ± 0.30), and its photometric redshift is
4 Statistical characteristics of GRB redshifts measured by different methods
The purpose of this paper is to explore various measurement methods of GRB redshift, so as to help astronomers choose accurate and effective measurement methods and apply them to future exploration. Therefore, in addition to the theoretical study of the algorithm, it is necessary to perform statistics on GRB redshift and show the applicable scope of these measurement methods in practical application.
We collect 611 GRBs with redshifts measured since 1997, as shown in Supplementary Table S2. The afterglow zspec indicates spectroscopic redshift measured by spectral measurement method of afterglow. The afterglow zphot indicates photometric redshift measured by Afterglow SED fitting method. The host zspec indicates measurement by spectral measurement method of host galaxy. The host zphot indicates measurement by template matching method of host galaxy.
581 GRBs have accurately measured redshifts, with a range from 0.0085 to 9.4. 30 GRBs redshift only have an upper or lower limit. Some GRBs have been measured by several methods or the same method several times, and these GRBs have multiple redshift values. In a few cases, afterglow zphot and host zphot may have large differences in several measurements. One example is GRB 191031D. The photometric redshift methods select the redshift with the minimum χ2. Although, χ2 values of the afterglow zphot measurement and host zphot measurement may be similar, the redshift values of each result can have large differences.
The distribution of GRB redshifts is shown in Figure 1. The mean value is
As shown in Figure 2, GRB is randomly distributed on the celestial sphere. When RA < 180°, −90° < DEC < 0°, there are 151 GRBs; when RA < 180°, 0° < DEC < 90°, there are 160 GRBs; when RA > 180°, −90° < DEC < 0°, there are 125 GRBs; when RA > 180°, 0° < DEC < 90°, there are 175 GRBs. In addition, afterglow zspec, afterglow zphot, host zspec and host zphot GRBs are also randomly distributed on the celestial sphere respectly.
As shown in Figures 3, 4, GRBs are less distributed in the low galactic latitudes. It is very difficult to find GRBs in galactic disk, due to the serious dust extinction, reddening and dense star field in galactic disk. In the high galactic latitudes, there are few transients such as GRB. There is no obvious correlation between galactic latitude and redshift. GRB with the highest redshift (GRB 090429B) is b = 73.72°.
As shown in Figure 5, except for the low galactic latitude, GRB is uniform distributed on the galactic celestial sphere, which proves that GRB is isotropic.
Comparison of different measurement methods of redshift is shown in Figure 6. It can be seen that the redshift distribution measured in different years is similar. While the dark host galaxy and the detection limit of current equipment, it is difficult to detect the host galaxy with high redshift. With the increase of redshift, the errors of all methods are relatively increased. The accuracy of spectral measurement is usually higher than that of photometric measurement. At present, the highest redshift of GRB 090429B belongs to afterglow zphot, as the afterglow is relatively bright. As GRB afterglow is relatively brighter than host. In order to improve the redshift measurement accuracy, we can combine above methods and take all the advantages of the various methods.
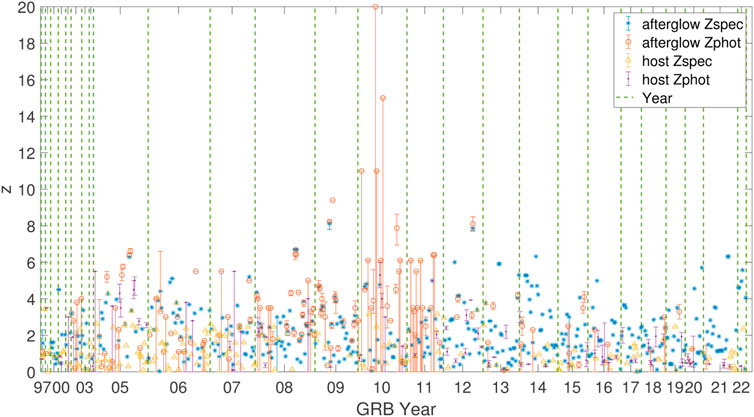
FIGURE 6. Comparison of different measurement methods of redshift. The horizontal axis represents the years of the GRBs, and the vertical axis represents the redshift. GRBs redshifts with known errors are described by error bars. For the GRBs with a certainly range limitation, the bottoms of error bars are connected to the zero-value point.
5 Summary and prospect
We introduce the research history and scientific significance of GRB redshift. Two types of measurement methods for GRB redshift are mainly summarized. The two types are the measurement methods based on afterglow and the measurement methods based on host galaxy. We have also outlined the principles, methods, and processes involved in GRB redshift measurement, and summarized the applications of different redshift measurement methods. Finally, we summarized and discussed the distribution of 611 GRBs with redshift measured by various methods.
SVOM mission is dedicated to GRB detection especially for high redshifts thanks to its gamma-ray detector payload, ECLAIRs, is more sensitive in softer the energy range of 4–150 keV (Wei et al., 2016). After ECLAIRs is triggered, SVOM can take follow-up observation quickly with its payload telescope Visible-band Telescope (VT), which can detect GRB at z > 6.5, and narrow-field ground-based robotic follow-up telescope (F-GFT and C-GFT) in multiple bands. The F-GFT will take broadband optical/infrared photometry with r and J simultaneous bands, operating in 0.4–1.7 μm. While C-GFT has SDSS g, r, i bands to operate photometry in 0.4–0.95 μm (Wei et al., 2016). The two ground telescopes, which have characteristics of high availability, can perform follow-up observations with, good sensitivity, fast pointing speed, low read-out time, high-precision localization and so on. GFTS can measure redshift from
Author contributions
CL and CW are responsible for the construction of the overall framework of the article. JM is responsible for literature collection, data collection, theoretical guidance and writing guidance. ML is responsible for literature collection, data collection, experiment design, data analysis and writing. ZK is responsible for theoretical and writing guidance. ZL is responsible for literature collection and theoretical guidance. SD and BN are responsible for data collection. PJ is responsible for writing guidance. In addition, All authors contributed to the article and approved the submitted version.
Funding
This work is supported by the National Natural Science Foundation of China (Grant No. U2031129, U1931133, 12273080, 12203078, 11673062). This work is also supported by the SVOM project, a mission in the Strategic Priority Program on Space Science of CAS. This work is also supported by Yunnan Revitalization Talent Support Program (YunLin Scholar Award).
Acknowledgments
We appreciate all reviewers and editor for their careful review. The comments are very valuable for us to complete the major revision and improve the quality of the manuscript. We appreciate Prof. Jianyan Wei's useful discussion and helps on improving paper.
Conflict of interest
The authors declare that the research was conducted in the absence of any commercial or financial relationships that could be construed as a potential conflict of interest.
Publisher’s note
All claims expressed in this article are solely those of the authors and do not necessarily represent those of their affiliated organizations, or those of the publisher, the editors and the reviewers. Any product that may be evaluated in this article, or claim that may be made by its manufacturer, is not guaranteed or endorsed by the publisher.
Supplementary material
The Supplementary Material for this article can be found online at: https://www.frontiersin.org/articles/10.3389/fspas.2023.1124317/full#supplementary-material
Footnotes
1The point is quoted from anonymous reviewers’ comments.
2The bands please see https://swift.gsfc.nasa.gov/about_swift/uvot_desc.html
3g’r’i’z’ filters please see https://classic.sdss.org/dr5/
References
Abazajian, K. N., Adelman-McCarthy, J. K., Agüeros, M. A., Allam, S. S., Allende Prieto, C., An, D., et al. (2009). The seventh data release of the Sloan digital sky survey. Astrophysical J. Suppl.182, 543–558. doi:10.1088/0067-0049/182/2/543
Acquaviva, V., Gawiser, E., and Guaita, L. (2012). SED fitting with MCMC: Methodology and application to large galaxy surveys. Spectr. Energy Distribution Galaxies - SED 2011284, 42–45. doi:10.1017/S1743921312008691
Acquaviva, V., Gawiser, E., and Guaita, L. (2011). Spectral energy distribution fitting with Markov chain Monte Carlo: Methodology and application to z = 3.1 lyα-emitting galaxies. Astrophysical J.737, 47. doi:10.1088/0004-637X/737/2/47
Acquaviva, V., Raichoor, A., and Gawiser, E. (2015). Simultaneous estimation of photometric redshifts and SED parameters: Improved techniques and a realistic error budget. Astrophysical J.804, 8. doi:10.1088/0004-637X/804/1/8
Afonso, P., Greiner, J., Pian, E., Covino, S., Malesani, D., Küpcü Yoldaş, A., et al. (2011). GRB 050502B optical afterglow: A jet-break at high redshift. Astronomy Astrophysics526, A154. doi:10.1051/0004-6361/200913965
Agüí Fernández, J. F., Thöne, C. C., Kann, D. A., de Ugarte Postigo, A., Selsing, J., Schady, P., et al. (2021). Grb 160410A: The first chemical study of the interstellar medium of a short GRB. arXiv e-prints , arXiv:2109.13838.
Amati, L., and Della Valle, M. (2013). Measuring cosmological parameters with gamma ray bursts. Int. J. Mod. Phys. D22, 1330028. doi:10.1142/S0218271813300280
Amati, L., Guidorzi, C., Frontera, F., Della Valle, M., Finelli, F., Landi, R., et al. (2008). Measuring the cosmological parameters with the Ep,i-Eiso correlation of gamma-ray bursts. Mon. Notices R. Astronomical Soc.391, 577–584. doi:10.1111/j.1365-2966.2008.13943.x
Andersen, M. I., Hjorth, J., Pedersen, H., Jensen, B. L., Hunt, L. K., Gorosabel, J., et al. (2000). VLT identification of the optical afterglow of the gamma-ray burst GRB 000131 at z=4.50. Astronomy Astrophysics364, L54–L61. doi:10.48550/arXiv.astro-ph/0010322
Antonelli, L. A., Piro, L., Vietri, M., Costa, E., Soffitta, P., Feroci, M., et al. (2000). Discovery of a redshifted iron K line in the X-ray afterglow of GRB 000214. Astrophysical J.545, L39–L42. doi:10.1086/317328
Atwood, W. B., Abdo, A. A., Ackermann, M., Althouse, W., Anderson, B., Axelsson, M., et al. (2009). The large area telescope on the Fermi gamma-ray space telescope mission. Astrophysical J.697, 1071–1102. doi:10.1088/0004-637X/697/2/1071
Atwood, W. B., Baldini, L., Bregeon, J., Bruel, P., Chekhtman, A., Cohen-Tanugi, J., et al. (2013). New fermi-LAT event reconstruction reveals more high-energy gamma rays from gamma-ray bursts. Astrophysical J.774, 76. doi:10.1088/0004-637X/774/1/76
Barthelmy, S. D., Chincarini, G., Burrows, D. N., Gehrels, N., Covino, S., Moretti, A., et al. (2005). An origin for short γ-ray bursts unassociated with current star formation. Nature438, 994–996. doi:10.1038/nature04392
Basa, S., Cuby, J. G., Savaglio, S., Boissier, S., Clément, B., Flores, H., et al. (2012). Constraining the nature of the most distant gamma-ray burst host galaxies. Astronomy Astrophysics542, A103. doi:10.1051/0004-6361/201218882
Beck, R., Dobos, L., Budavári, T., Szalay, A. S., and Csabai, I. (2016). Photometric redshifts for the SDSS data release 12. Mon. Notices R. Astronomical Soc.460, 1371–1381. doi:10.1093/mnras/stw1009
Bedient, J., Pearce, A., Otero, S., and Carvajal, J. (2001). V445 puppis. International Astronomical Union Circular, Available at: http://www.cbat.eps.harvard.edu/iauc/07500/07586.html.
Bellm, E. C., Hurley, K., Pal’shin, V., Yamaoka, K., Bandstra, M. S., Boggs, S. E., et al. (2008). Observations of the prompt gamma-ray emission of GRB 070125. Astrophysical J.688, 491–498. doi:10.1086/592136
Benítez, N. (2000). Bayesian photometric redshift estimation. Astrophysical J.536, 571–583. doi:10.1086/308947
Berger, E. (2010). A short gamma-ray burst “No-host” problem? Investigating large progenitor offsets for short GRBs with optical afterglows. Astrophysical J.722, 1946–1961. doi:10.1088/0004-637X/722/2/1946
Berger, E., Fox, D. B., Kulkarni, S. R., Frail, D. A., and Djorgovski, S. G. (2007a). The ERO host galaxy of GRB 020127: Implications for the metallicity of GRB progenitors. Astrophysical J.660, 504–508. doi:10.1086/513007
Berger, E., Fox, D. B., Price, P. A., Nakar, E., Gal-Yam, A., Holz, D. E., et al. (2007b). A new population of high-redshift short-duration gamma-ray bursts. Astrophysical J.664, 1000–1010. doi:10.1086/518762
Berger, E., Kulkarni, S. R., Fox, D. B., Soderberg, A. M., Harrison, F. A., Nakar, E., et al. (2005). Afterglows, redshifts, and properties ofSwiftGamma-ray bursts. Astrophysical J.634, 501–508. doi:10.1086/491667
Berger, E., Penprase, B. E., Cenko, S. B., Kulkarni, S. R., Fox, D. B., Steidel, C. C., et al. (2006). Spectroscopy of GRB 050505 at z = 4.275: A logN(H I) = 22.1 DLA host galaxy and the nature of the progenitor. Astrophysical J.642, 979–988. doi:10.1086/501162
Bloom, J. S., Djorgovski, S. G., Kulkarni, S. R., and Frail, D. A. (1998). The host galaxy of GRB 970508. Astrophysical J.507, L25–L28. doi:10.1086/311682
Bloom, J. S., Djorgovski, S. G., and Kulkarni, S. R. (2001a). The redshift and the ordinary host galaxy of GRB 970228. Astrophysical J.554, 678–683. doi:10.1086/321398
Bloom, J. S., Frail, D. A., and Sari, R. (2001b). The prompt energy release of gamma-ray bursts using a cosmological [ITAL]k[/ITAL]-Correction. Astronomical J.121, 2879–2888. doi:10.1086/321093
Bloom, J. S., Kulkarni, S. R., Djorgovski, S. G., Eichelberger, A. C., Côté, P., Blakeslee, J. P., et al. (1999). The unusual afterglow of the γ-ray burst of 26 March 1998 as evidence for a supernova connection. Nature401, 453–456. doi:10.1038/46744
Bloom, J. S., Kulkarni, S. R., and Djorgovski, S. G. (2002). The observed offset distribution of gamma-ray bursts from their host galaxies: A robust clue to the nature of the progenitors. Astronomical J.123, 1111–1148. doi:10.1086/338893
Bloom, J. S., Perley, D. A., Chen, H. W., Butler, N., Prochaska, J. X., Kocevski, D., et al. (2007). A putative early-type host galaxy for GRB 060502B: Implications for the progenitors of short-duration hard-spectrum bursts. Astrophysical J.654, 878–884. doi:10.1086/509114
Bolmer, J., Greiner, J., Krühler, T., Schady, P., Ledoux, C., Tanvir, N. R., et al. (2018). Dust reddening and extinction curves toward gamma-ray bursts at z > 4. Astronomy Astrophysics609, A62. doi:10.1051/0004-6361/201731255
Bolzonella, M., Miralles, J. M., and Pelló, R. (2000). Photometric redshifts based on standard SED fitting procedures. Astronomy Astrophysics363, 476–492. doi:10.48550/arXiv.astro-ph/0003380
Bruzual, G., and Charlot, S. (2003). Stellar population synthesis at the resolution of 2003. Mon. Notices R. Astronomical Soc.344, 1000–1028. doi:10.1046/j.1365-8711.2003.06897.x
Burgarella, D., Buat, V., and Iglesias-Páramo, J. (2005). Star formation and dust attenuation properties in galaxies from a statistical ultraviolet-to-far-infrared analysis. Mon. Notices R. Astronomical Soc.360, 1413–1425. doi:10.1111/j.1365-2966.2005.09131.x
Burrows, D. N., Hill, J. E., Nousek, J. A., Wells, A. A., Short, A. T., Ambrosi, R. M., et al. (2003). Swift x-ray telescope (XRT). Soc. Photo-Optical Instrum. Eng. (SPIE) Conf. Ser.4851, 1320–1325. doi:10.1117/12.461279
Butler, N. R. (2007). On the early-time X-ray spectra of Swift afterglows. I. Evidence for anomalous soft X-ray emission. Astrophysical J.656, 1001–1018. doi:10.1086/510548
Carnall, A. C., McLure, R. J., Dunlop, J. S., and Davé, R. (2018). Inferring the star formation histories of massive quiescent galaxies with BAGPIPES: Evidence for multiple quenching mechanisms. Mon. Notices R. Astronomical Soc.480, 4379–4401. doi:10.1093/mnras/sty2169
Carrasco, K. M., and Brunner, R. J. (2013). Tpz: Photometric redshift PDFs and ancillary information by using prediction trees and random forests. Mon. Notices R. Astronomical Soc.432, 1483–1501. doi:10.1093/mnras/stt574
Castro-Tirado, A. J., Møller, P., García-Segura, G., Gorosabel, J., Pérez, E., de Ugarte Postigo, A., et al. (2010). Grb 021004: Tomography of a gamma-ray burst progenitor and its host galaxy. Astronomy Astrophysics517, A61. doi:10.1051/0004-6361/200913966
Chen, H.-W. (2012). Near-infrared spectroscopy of gamma-ray burst host galaxies at: Insights into host galaxy dynamics and interpretations of afterglow absorption spectra: NIR spectra of two z ≳ 1.5 GRB hosts. Mon. Notices R. Astronomical Soc.419, 3039–3047. doi:10.1111/j.1365-2966.2011.19944.x
Chevallard, J., and Charlot, S. (2016). Modelling and interpreting spectral energy distributions of galaxies with BEAGLE. Mon. Notices R. Astronomical Soc.462, 1415–1443. doi:10.1093/mnras/stw1756
Chornock, R., Berger, E., Fox, D. B., Lunnan, R., Drout, M. R., Fong, W., et al. (2013). GRB 130606A as a probe of the intergalactic medium and the interstellar medium in a star-forming galaxy in the first gyr after the big bang. Astrophysical J.774, 26. doi:10.1088/0004-637X/774/1/26
Ciardi, B., and Loeb, A. (2000). Expected number and flux distribution of gamma-ray burst afterglows with high redshifts. Astrophysical J.540, 687–696. doi:10.1086/309384
Connolly, A. J., Csabai, I., Szalay, A. S., Koo, D. C., Kron, R. G., and Munn, J. A. (1995). Slicing through multicolor space: Galaxy redshifts from broadband photometry. Astronomical J.110, 2655. doi:10.1086/117720
Conroy, C., Gunn, J. E., and White, M. (2009). The propagation of uncertainties in stellar population synthesis modeling. I. The relevance of uncertain aspects of stellar evolution and the initial mass function to the derived physical properties of galaxies. Astrophysical J.699, 486–506. doi:10.1088/0004-637X/699/1/486
Conselice, C. J., Vreeswijk, P. M., Fruchter, A. S., Levan, A., Kouveliotou, C., Fynbo, J. P. U., et al. (2005). Gamma-ray burst-selected high-redshift galaxies: Comparison to field galaxy populations to z ˜3. Astrophysical J.633, 29–40. doi:10.1086/432829
Costa, E., Frontera, F., Heise, J., Feroci, M., Zand, J., Fiore, F., et al. (1997). Discovery of an X-ray afterglow associated with the γ-ray burst of 28 February 1997. Nature387, 783–785. doi:10.1038/42885
Coward, D. M., Guetta, D., Burman, R. R., and Imerito, A. (2008). Where are the missing gamma-ray burst redshifts?Mon. Notices R. Astronomical Soc.386, 111–116. doi:10.1111/j.1365-2966.2008.13006.x
Coward, D. M., Howell, E. J., Branchesi, M., Stratta, G., Guetta, D., Gendre, B., et al. (2013). The Swift gamma-ray burst redshift distribution: Selection biases and optical brightness evolution at high z?Mon. Notices R. Astronomical Soc.432, 2141–2149. doi:10.1093/mnras/stt537
Cucchiara, A., Levan, A. J., Fox, D. B., Tanvir, N. R., Ukwatta, T. N., Berger, E., et al. (2011). A photometric redshift of z ˜9.4 for GRB 090429B. Astrophysical J.736, 7. doi:10.1088/0004-637X/736/1/7
Cucchiara, A., Prochaska, J. X., Perley, D., Cenko, S. B., Werk, J., Cardwell, A., et al. (2013). Gemini spectroscopy of the short-hard gamma-ray burst GRB 130603B afterglow and host galaxy. Astrophysical J.777, 94. doi:10.1088/0004-637X/777/2/94
Cunha, E. D., Charlot, S., and Elbaz, D. (2008). A simple model to interpret the ultraviolet, optical and infrared emission from galaxies. Mon. Notices R. Astronomical Soc.388, 1595–1617. doi:10.1111/j.1365-2966.2008.13535.x
Cunha, P. A. C., and Humphrey, A. (2022). Photometric redshift-aided classification using ensemble learning. Astronomy Astrophysics666, A87. doi:10.1051/0004-6361/202243135
Curran, P. A., Wijers, R. A. M. J., Heemskerk, M. H. M., Starling, R. L. C., Wiersema, K., and van der Horst, A. J. (2008). Robust photometric redshift determinations of gamma-ray burst afterglows at z ≳ 2. Astronomy Astrophysics490, 1047–1053. doi:10.1051/0004-6361:200810545
Dainotti, M. G., Young, S., Li, L., Levine, D., Kalinowski, K. K., Kann, D. A., et al. (2022). The optical two- and three-dimensional fundamental plane correlations for nearly 180 gamma-ray burst afterglows with Swift/UVOT, RATIR, and the subaru telescope. Astrophysical J. Suppl. Ser.261, 25. doi:10.3847/1538-4365/ac7c64
D’Avanzo, P., Malesani, D., Covino, S., Piranomonte, S., Grazian, A., Fugazza, D., et al. (2009). The optical afterglows and host galaxies of three short/hard gamma-ray bursts. Astronomy Astrophysics498, 711–721. doi:10.1051/0004-6361/200811294
Della Valle, M., Malesani, D., Bloom, J. S., Benetti, S., Chincarini, G., D’Avanzo, P., et al. (2006). Hypernova signatures in the late rebrightening of GRB 050525A. Astrophysical J.642, L103–L106. doi:10.1086/504636
Dey, B., Andrews, B. H., Newman, J. A., Mao, Y.-Y., Rau, M. M., and Zhou, R. (2022). Photometric redshifts from SDSS images with an interpretable deep capsule network. Mon. Notices R. Astronomical Soc.515, 5285–5305. doi:10.1093/mnras/stac2105
Dimple, K., MisraGhosh, A., Arun, K. G., Gupta, R., Kumar, A., Resmi, L., et al. (2022). Grb 210217A: A short or a long GRB?Astrophysics Astronomy43, 39. doi:10.1007/s12036-022-09822-x
Djorgovski, S. G., Frail, D. A., Kulkarni, S. R., Bloom, J. S., Odewahn, S. C., and Diercks, A. (2001). The afterglow and the host galaxy of the dark burst GRB 970828. Astrophysical J.562, 654–663. doi:10.1086/323845
Djorgovski, S. G., Kulkarni, S. R., Bloom, J. S., Goodrich, R., Frail, D. A., Piro, L., et al. (1998). Spectroscopy of the host galaxy of the gamma-ray burst 980703. Astrophysical J.508, L17–L20. doi:10.1086/311729
Duan, F. Q., Wu, F. C., Luo, A. L., and Zhao, Y. H. (2005). Density estimation based model matching method for redshift determination. Spectrosc. Spectr. Analysis2005, 1895–1898. doi:10.3321/j.issn:1000-0593.2005.11.042
Eldridge, J. J., Stanway, E. R., Xiao, L., McClelland, L. A. S., Taylor, G., Ng, M., et al. (2017). Binary population and spectral synthesis version 2.1: Construction, observational verification, and new results. Astronomical Soc. Aust.34, e058. doi:10.1017/pasa.2017.51
Ferrero, P., Klose, S., Kann, D. A., Savaglio, S., Schulze, S., Palazzi, E., et al. (2009). Grb 060605: Multi-wavelength analysis of the first GRB observed using integral field spectroscopy. Astronomy Astrophysics497, 729–741. doi:10.1051/0004-6361/200809980
Fiore, F., D’Elia, V., Lazzati, D., Perna, R., Sbordone, L., Stratta, G., et al. (2005). A flash in the dark: UVES very large telescope high-resolution spectroscopy of gamma-ray burst afterglows. Astrophysical J.624, 853–867. doi:10.1086/429385
Fiore, F., Guetta, D., Piranomonte, S., D’Elia, V., and Antonelli, L. A. (2007). Selection effects shaping the gamma ray burst redshift distributions. Astronomy Astrophysics470, 515–522. doi:10.1051/0004-6361:20077157
Firth, A. E., Lahav, O., and Somerville, R. S. (2003). Estimating photometric redshifts with artificial neural networks. Mon. Notice R. Astronomical Soc.339, 1195–1202. doi:10.1046/j.1365-8711.2003.06271.x
Fong, W., Berger, E., Chornock, R., Margutti, R., Levan, A. J., Tanvir, N. R., et al. (2013). Demographics of the galaxies hosting short-duration gamma-ray bursts. Astrophysical J.769, 56. doi:10.1088/0004-637X/769/1/56
Fong, W., Berger, E., Chornock, R., Tanvir, N. R., Levan, A. J., Fruchter, A. S., et al. (2011). The optical afterglow and z = 0.92 early-type host galaxy of the short GRB 100117A. Astrophysical J.730, 26. doi:10.1088/0004-637X/730/1/26
Fong, W., Laskar, T., Rastinejad, J., Escorial, A. R., Schroeder, G., Barnes, J., et al. (2021). The broadband counterpart of the short GRB 200522A at z = 0.5536: A luminous kilonova or a collimated outflow with a reverse shock?Astrophysical J.906, 127. doi:10.3847/1538-4357/abc74a
Fong, W., Margutti, R., Chornock, R., Berger, E., Shappee, B. J., Levan, A. J., et al. (2016). The afterglow and early-type host galaxy of the short GRB 150101B at z = 0.1343. Astrophysical J.833, 151. doi:10.3847/1538-4357/833/2/151
Fong, W., Nugent, A. E., Dong, Y., Berger, E., Paterson, K., Chornock, R., et al. (2022). Short GRB host galaxies. I. Photometric and spectroscopic catalogs, host associations, and galactocentric offsets. Astrophysical J.940, 56. doi:10.3847/1538-4357/ac91d0
Fruchter, A., Bergeron, L., and Pian, E. (1997). Grb 970508. International Astronomical Union Circular. Available at: http://www.cbat.eps.harvard.edu/iauc/06600/06674.html.
Fruchter, A. S., Levan, A. J., Strolger, L., Vreeswijk, P. M., Thorsett, S. E., Bersier, D., et al. (2006). Long γ-ray bursts and core-collapse supernovae have different environments. Nature441, 463–468. doi:10.1038/nature04787
Fruchter, A. S., Pian, E., Thorsett, S. E., Bergeron, L. E., González, R. A., Metzger, M., et al. (1999). The fading optical counterpart of GRB 970228, 6 Months and 1 Year later. Astrophysical J.516, 683–692. doi:10.1086/307136
Fynbo, J. P. U., Jakobsson, P., Prochaska, J. X., Malesani, D., Ledoux, C., de Ugarte Postigo, A., et al. (2009). Low-resolution spectroscopy of gamma-ray burst optical afterglows: Biases in the Swift sample and characterization of the absorbers. Astrophysical J. Suppl.185, 526–573. doi:10.1088/0067-0049/185/2/526
Fynbo, J. P. U., Starling, R. L. C., Ledoux, C., Wiersema, K., Thöne, C. C., Sollerman, J., et al. (2006). Probing cosmic chemical evolution with gamma-ray bursts: GRB 060206 at z = 4.048. Astronomy Astrophysics451, L47–L50. doi:10.1051/0004-6361:20065056
Galama, T., Groot, P. J., Vanparadijs, J., Kouveliotou, C., Robinson, C. R., Fishman, G. J., et al. (1997). The decay of optical emission from the γ-ray burst GRB 970228. Nature387, 479–481. doi:10.1038/387479a0
Galama, T. J., Vreeswijk, P. M., Van Paradijs, J., Kouveliotou, C., Augusteijn, T., Böhnhardt, H., et al. (1998). An unusual supernova in the error box of the γ-ray burst of 25 April 1998. Nature395, 670–672. doi:10.1038/27150
Gehrels, N., Chincarini, G., Giommi, P., Mason, K. O., Nousek, J. A., Wells, A. A., et al. (2004). TheSwiftGamma-ray burst mission. Astrophysical J.611, 1005–1020. doi:10.1086/422091
GLAST Facility Science Team Gehrels, N., and Michelson, P. (1999). Glast: The next-generation high energy gamma-ray astronomy mission. Astropart. Phys.11, 277–282. doi:10.1016/S0927-6505(99)00066-3
Glazebrook, K., Offer, A. R., and Deeley, K. (1998). Automatic redshift determination by use of principal component analysis. I. Fundamentals. Astrophysical J.492, 98–109. doi:10.1086/305039
Goldstein, A., Hamburg, R., Wood, J., Hui, C. M., Cleveland, W. H., Kocevski, D., et al. (2019). Updates to the Fermi GBM targeted sub-threshold Search in preparation for the third observing run of LIGO/virgo. arXiv e-prints , arXiv:1903.12597.
Gorosabel, J., Christensen, L., Hjorth, J., Fynbo, J. U., Pedersen, H., Jensen, B. L., et al. (2003). A multi-colour study of the dark GRB 000210 host galaxy and its environment. Astronomy Astrophysics400, 127–136. doi:10.1051/0004-6361:20021907
Gorosabel, J., Fynbo, J. P. U., Fruchter, A., Levan, A., Hjorth, J., Nugent, P., et al. (2005). A possible bright blue supernova in the afterglow of GRB 020305. Astronomy Astrophysics437, 411–418. doi:10.1051/0004-6361:20052783
Götz, D., Covino, S., Hascoët, R., Fernandez-Soto, A., Daigne, F., Mochkovitch, R., et al. (2011). A detailed spectral study of GRB 041219A and its host galaxy. Mon. Notices R. Astronomical Soc.413, 2173–2183. doi:10.1111/j.1365-2966.2011.18290.x
Gou, L. J., Mészáros, P., Abel, T., and Zhang, B. (2004). Detectability of long gamma-ray burst afterglows from very high redshifts. Astrophysical J.604, 508–520. doi:10.1086/382061
Graham, J. F., Fruchter, A. S., Levan, A. J., Melandri, A., Kewley, L. J., Levesque, E. M., et al. (2009). Grb 070714B—discovery of the highest spectroscopically confirmed short burst redshift. Astrophysical J.698, 1620–1629. doi:10.1088/0004-637X/698/2/1620
Greiner, J., Bornemann, W., Clemens, C., Deuter, M., Hasinger, G., Honsberg, M., et al. (2008). GROND—A 7-channel imager. Astronomical Soc. Pac.120, 405–424. doi:10.1086/587032
Greiner, J., Fox, D. B., Schady, P., Krühler, T., Trenti, M., Cikota, A., et al. (2015). GAMMA-RAY BURSTS TRACE UV METRICS OF STAR FORMATION OVER 3 <z< 5. Astrophysical J.809, 76. doi:10.1088/0004-637X/809/1/76
Greiner, J., Krühler, T., Fynbo, J. P. U., Rossi, A., Schwarz, R., Klose, S., et al. (2009a). Grb 080913 at redshift 6.7. Astrophysical J.693, 1610–1620. doi:10.1088/0004-637X/693/2/1610
Greiner, J., Krühler, T., McBreen, S., Ajello, M., Giannios, D., Schwarz, R., et al. (2009b). A strong optical flare before the rising afterglow of GRB 080129. Astrophysical J.693, 1912–1919. doi:10.1088/0004-637X/693/2/1912
Guo, Q. (2020). Study on the jet structure and correlation of short gamma-ray bursts. University of Science and Technology of China. doctor. doi:10.27517/d.cnki.gzkju.2020.001139
Gupta, R., Oates, S. R., Pandey, S. B., Castro-Tirado, A. J., Joshi, J. C., Hu, Y. D., et al. (2021). Grb 140102A: Insight into prompt spectral evolution and early optical afterglow emission. Mon. Notices R. Astronomical Soc.505, 4086–4105. doi:10.1093/mnras/stab1573
Gupta, R., Pandey, S. B., Kumar, A., Aryan, A., Ror, A. K., Sharma, S., et al. (2022). Photometric studies on the host galaxies of gamma-ray bursts using 3.6m Devasthal optical telescope. J. Astrophysics Astronomy43, 82. doi:10.1007/s12036-022-09865-0
Guziy, S., Gorosabel, J., Castro-Tirado, A. J., de Ugarte Postigo, A., Jelínek, M., Pérez Ramírez, M. D., et al. (2005). The search for the host galaxy of the gamma-ray burst GRB 000214. Astronomy Astrophysics441, 975–979. doi:10.1051/0004-6361:20042419
Haislip, J. B., Nysewander, M. C., Reichart, D. E., Levan, A., Tanvir, N., Cenko, S. B., et al. (2006). A photometric redshift of z = 6.39 ± 0.12 for GRB 050904. Nature440, 181–183. doi:10.1038/nature04552
Han, B., Zhang, Y., and Zhao, Y. (2015). “Active learning applied for photometric redshift estimation of quasars,” in IAU 29th General Assembly in 2015. Available at: https://ui.adsabs.harvard.edu/abs/2015IAUGA.2256851H/abstract.
Heintz, K. E., Bolmer, J., Ledoux, C., Noterdaeme, P., Krogager, J. K., Fynbo, J. P. U., et al. (2019). New constraints on the physical conditions in H2-bearing GRB-host damped Lyman-α absorbers. Astronomy Astrophysics629, A131. doi:10.1051/0004-6361/201936250
Hjorth, J., Malesani, D., Jakobsson, P., Jaunsen, A. O., Fynbo, J. P. U., Gorosabel, J., et al. (2012). The optically unbiased gamma-ray burst host (TOUGH) survey. I. Survey design and catalogs. Astrophysical J.756, 187. doi:10.1088/0004-637X/756/2/187
Hjorth, J., Møller, P., Gorosabel, J., Fynbo, J. P. U., Toft, S., Jaunsen, A. O., et al. (2003). Very high column density and small reddening toward GRB 020124 at z=3.20. Astrophysical J.597, 699–705. doi:10.1086/378493
Hunt, L., Palazzi, E., Rossi, A., Savaglio, S., Cresci, G., Klose, S., et al. (2011). The extremely red host galaxy of GRB 080207. Astrophysical J. Lett.736, L36. doi:10.1088/2041-8205/736/2/L36
Izzo, L., Muccino, M., Zaninoni, E., Amati, L., and Della Valle, M. (2015). New measurements of Ωm from gamma-ray bursts. Astronomy Astrophysics582, A115. doi:10.1051/0004-6361/201526461
Jakobsson, P., Fynbo, J. P. U., Ledoux, C., Vreeswijk, P., Kann, D. A., Hjorth, J., et al. (2006a). H I column densities ofz> 2Swiftgamma-ray bursts. Astronomy Astrophysics460, L13–L17. doi:10.1051/0004-6361:20066405
Jakobsson, P., Hjorth, J., Malesani, D., Chapman, R., Fynbo, J. P. U., Tanvir, N. R., et al. (2012). The optically unbiased GRB host (TOUGH) survey. III. Redshift distribution. Astrophysical J.752, 62. doi:10.1088/0004-637X/752/1/62
Jakobsson, P., Levan, A., Fynbo, J. P. U., Priddey, R., Hjorth, J., Tanvir, N., et al. (2006b). A mean redshift of 2.8 forSwiftgamma-ray bursts. Astronomy Astrophysics447, 897–903. doi:10.1051/0004-6361:20054287
Jakobsson, P., Levan, A., Fynbo, J. P. U., Priddey, R., Hjorth, J., Tanvir, N., et al. (2006c). GRB 050814 at z = 5.3 and the redshift distribution of Swift GRBs. Am. Inst. Phys. Conf. Ser.836, 552–557. doi:10.1063/1.2207953
Japelj, J., Covino, S., Gomboc, A., Vergani, S. D., Goldoni, P., Selsing, J., et al. (2015). Spectrophotometric analysis of gamma-ray burst afterglow extinction curves with X-Shooter. Astronomy Astrophysics579, A74. doi:10.1051/0004-6361/201525665
Jaunsen, A. O., Andersen, M. I., Hjorth, J., Fynbo, J. P. U., Holland, S. T., Thomsen, B., et al. (2003). An HST study of three very faint GRB host galaxies. Astronomy Astrophysics402, 125–132. doi:10.1051/0004-6361:20030154
Jaunsen, A. O., Rol, E., Watson, D. J., Malesani, D., Fynbo, J. P. U., Milvang-Jensen, B., et al. (2008). Grb 070306: A highly extinguished afterglow. Astrophysical J.681, 453–461. doi:10.1086/588602
Johnson, B. D., Leja, J., Conroy, C., and Speagle, J. S. (2021). Stellar population inference with prospector. Astrophysical J. Suppl. Ser.254, 22. doi:10.3847/1538-4365/abef67
Kelly, P. L., Kirshner, R. P., and Pahre, M. (2008). Long γ-ray bursts and type ic core-collapse supernovae have similar locations in hosts. Astrophysical J.687, 1201–1207. doi:10.1086/591925
Klose, S., Schmidl, S., Kann, D. A., Nicuesa Guelbenzu, A., Schulze, S., Greiner, J., et al. (2019). Four GRB supernovae at redshifts between 0.4 and 0.8 the bursts GRB 071112C, 111228A, 120714B, and 130831A. Astronomy Astrophysics622, A138. doi:10.1051/0004-6361/201832728
Krühler, T., Greiner, J., Schady, P., Savaglio, S., Afonso, P. M. J., Clemens, C., et al. (2011a). The SEDs and host galaxies of the dustiest GRB afterglows. Astronomy Astrophysics534, A108. doi:10.1051/0004-6361/201117428
Krühler, T., Küpcü Yoldaş, A., Greiner, J., Clemens, C., McBreen, S., Primak, N., et al. (2008). The 2175 Å dust feature in a gamma-ray burst afterglow at redshift 2.45. Astrophysical J.685, 376–383. doi:10.1086/590240
Krühler, T., Malesani, D., Fynbo, J. P. U., Hartoog, O. E., Hjorth, J., Jakobsson, P., et al. (2015). GRB hosts through cosmic time. VLT/X-Shooter emission-line spectroscopy of 96 γ-ray-burst-selected galaxies at 0.1 < z < 3.6. Astronomy Astrophysics581, A125. doi:10.1051/0004-6361/201425561
Krühler, T., Malesani, D., Milvang-Jensen, B., Fynbo, J. P. U., Hjorth, J., Jakobsson, P., et al. (2012). The optically unbiased GRB host (TOUGH) survey. V. VLT/X-shooter emission-line redshifts for Swift GRBs at z ˜2. Astrophysical J.758, 46. doi:10.1088/0004-637X/758/1/46
Krühler, T., Schady, P., Greiner, J., Afonso, P., Bottacini, E., Clemens, C., et al. (2011b). Photometric redshifts for gamma-ray burst afterglows from GROND and Swift/UVOT. Astronomy Astrophysics526, A153. doi:10.1051/0004-6361/201015327
Kuin, N. P. M., Landsman, W., Page, M. J., Schady, P., Still, M., Breeveld, A. A., et al. (2009). Grb 081203A: Swift UVOT captures the earliest ultraviolet spectrum of a gamma-ray burst. Mon. Notices R. Astronomical Soc.395, L21–L24. doi:10.1111/j.1745-3933.2009.00632.x
Kulkarni, S. R., Djorgovski, S. G., Ramaprakash, A. N., Goodrich, R., Bloom, J. S., Adelberger, K. L., et al. (1998). Identification of a host galaxy at redshift z = 3.42 for the γ-ray burst of 14 December 1997. Nature393, 35–39. doi:10.1038/29927
Laskar, T., Berger, E., Tanvir, N., Zauderer, B. A., Margutti, R., Levan, A., et al. (2014). GRB 120521C at z ˜6 and the properties of high-redshift γ-ray bursts. Astrophysical J.781, 1. doi:10.1088/0004-637X/781/1/1
Le Floc’h, E., Duc, P. A., Mirabel, I. F., Sanders, D. B., Bosch, G., Diaz, R. J., et al. (2003). Are the hosts of gamma-ray bursts sub-luminous and blue galaxies?Astronomy Astrophysics400, 499–510. doi:10.1051/0004-6361:20030001
Le Floc’h, E., Duc, P. A., Mirabel, I. F., Sanders, D. B., Bosch, G., Rodrigues, I., et al. (2002). Very large telescope and [ITAL]Hubble space telescope[/ITAL] observations of the host galaxy of GRB 990705. Astrophysical J.581, L81–L84. doi:10.1086/346072
Leibler, C. N., and Berger, E. (2010). The stellar ages and masses of short gamma-ray burst host galaxies: Investigating the progenitor delay time distribution and the role of mass and star formation in the short gamma-ray burst rate. Astrophysical J.725, 1202–1214. doi:10.1088/0004-637X/725/1/1202
Levan, A. J., Tanvir, N. R., Starling, R. L. C., Wiersema, K., Page, K. L., Perley, D. A., et al. (2014). A new population of ultra-long duration gamma-ray bursts. Astrophysical J.781, 13. doi:10.1088/0004-637X/781/1/13
Li, L., Liang, E. W., Tang, Q. W., Chen, J. M., Xi, S. Q., Lü, H. J., et al. (2012). A comprehensive study of gamma-ray burst optical emission. I. Flares and early shallow-decay component. Astrophysical J.758, 27. doi:10.1088/0004-637X/758/1/27
Li, L., Wang, Y., Shao, L., Wu, X. F., Huang, Y. F., Zhang, B., et al. (2018). A large catalog of multiwavelength GRB afterglows. I. Color evolution and its physical implication. Astrophysical J. Suppl. Ser.234, 26. doi:10.3847/1538-4365/aaa02a
Li, L., Wu, X. F., Huang, Y. F., Wang, X. G., Tang, Q. W., Liang, Y. F., et al. (2015). A correlated study of optical and X-ray afterglows of GRBs. Astrophysical J.805, 13. doi:10.1088/0004-637X/805/1/13
Lin, Y. Q. (2009). Research paper: Comparison between Swift and pre-Swift gamma-ray bursts. Res. Astronomy Astrophysics9, 682–686. doi:10.1088/1674-4527/9/6/006
Littlejohns, O. M., Butler, N. R., Cucchiara, A., Watson, A. M., Kutyrev, A. S., Lee, W. H., et al. (2014). Identifying high-redshift gamma-ray bursts with RATIR. Astronomical J.148, 2. doi:10.1088/0004-6256/148/1/2
Liu, R., Qiao, X. J., and Duan, F. Q. (2008). Redshift estimation of galaxy spectra based on similarity measure. Spectrosc. Spectr. Analysis28, 235–238. doi:10.3964/j.issn.1000-0593.2008.01.057
Lloyd-Ronning, N. M., Fryer, C. L., and Ramirez-Ruiz, E. (2002). Cosmological aspects of gamma-ray bursts: Luminosity evolution and an estimate of the star formation rate at high redshifts. Astrophysical J.574, 554–565. doi:10.1086/341059
Luo, A. L., and Zhao, Y. H. (2001). Steps towards a fully automated classification and redshift-measurement pipeline for LAMOST spectra. I. Continuum level and wavelength estimation for galaxies. Chin. J. Astronomy Astrophysics1, 563–572. doi:10.1088/1009-9271/1/6/563
Lyman, J. D., Levan, A. J., Tanvir, N. R., Fynbo, J. P. U., McGuire, J. T. W., Perley, D. A., et al. (2017). The host galaxies and explosion sites of long-duration gamma ray bursts: Hubble Space Telescope near-infrared imaging. Mon. Notices R. Astronomical Soc.467, stx220–1817. doi:10.1093/mnras/stx220
Maraston, C. (2005). Evolutionary population synthesis: Models, analysis of the ingredients and application to high-z galaxies. Mon. Notices R. Astronomical Soc.362, 799–825. doi:10.1111/j.1365-2966.2005.09270.x
Melandri, A., Malesani, D. B., Izzo, L., Japelj, J., Vergani, S. D., Schady, P., et al. (2019). GRB 171010A/SN 2017htp: A GRB-SN at z = 0.33. Mon. Notices R. Astronomical Soc.490, 5366–5374. doi:10.1093/mnras/stz2900
Metzger, M. R., Cohen, J. G., Chaffee, F. H., and Blandford, R. D. (1997a). Grb 970508. International Astronomical Union Circular. Available at: http://www.cbat.eps.harvard.edu/iauc/06600/06676.html.
Metzger, M. R., Djorgovski, S. G., Kulkarni, S. R., Steidel, C. C., Adelberger, K. L., Frail, D. A., et al. (1997b). Spectral constraints on the redshift of the optical counterpart to the γ-ray burst of 8 May 1997. Nature387, 878–880. doi:10.1038/43132
Mirabel, I. F., and Rodrigues, I. (2003). Formation of a black hole in the dark. Science300, 1119–1120. doi:10.1126/science.1083451
Modjaz, M., Kewley, L., Kirshner, R. P., Stanek, K. Z., Challis, P., Garnavich, P. M., et al. (2008). Measured metallicities at the sites of nearby broad-lined type ic supernovae and implications for the supernovae gamma-ray burst connection. Astronomical J.135, 1136–1150. doi:10.1088/0004-6256/135/4/1136
Moresco, M., Amati, L., Amendola, L., Birrer, S., Blakeslee, J. P., Cantiello, M., et al. (2022). Unveiling the Universe with emerging cosmological probes. Living Rev. Relativ.25, 6. doi:10.1007/s41114-022-00040-z
Muccino, M., Izzo, L., Luongo, O., Boshkayev, K., Amati, L., Della Valle, M., et al. (2021). Tracing dark energy history with gamma-ray bursts. Astrophysical J.908, 181. doi:10.3847/1538-4357/abd254
Nakagawa, Y. E., Yoshida, A., Sugita, S., Tanaka, K., Ishikawa, N., Tamagawa, T., et al. (2006). An optically dark GRB observed by HETE-2: Grb 051022. Publ. Astronomical Soc. Jpn.58, L35–L39. doi:10.1093/pasj/58.4.L35
Nugent, A. E., Fong, W.-F., Dong, Y., Leja, J., Berger, E., Zevin, M., et al. (2022). Short GRB host galaxies. II. A legacy sample of redshifts, stellar population properties, and implications for their neutron star merger origins. Astrophysical J.940, 57. doi:10.3847/1538-4357/ac91d1
Nugent, A. E., Fong, W., Dong, Y., Palmese, A., Leja, J., Escorial, A. R., et al. (2020). The distant, galaxy cluster environment of the short GRB 161104A at z ∼ 0.8 and a comparison to the short GRB host population. Astrophysical J.904, 52. doi:10.3847/1538-4357/abc24a
Oates, S. R., and Cummings, J. R. (2009). GRB090426 Swift/UVOT observations. GRB Coord. Netw.9265, 1.
O’Connor, B., Troja, E., Dichiara, S., Beniamini, P., Cenko, S. B., Kouveliotou, C., et al. (2022). A deep survey of short GRB host galaxies over z 0-2: Implications for offsets, redshifts, and environments. Mon. Notices R. Astronomical Soc.515, 4890–4928. doi:10.1093/mnras/stac1982
Östlin, G., Zackrisson, E., Sollerman, J., Mattila, S., and Hayes, M. (2008). Constraining the mass of the GRB 030329 progenitor. Mon. Notices R. Astronomical Soc.387, 1227–1236. doi:10.1111/j.1365-2966.2008.13319.x
Pan, J. C., Luo, A. L., Wei, P., Jiang, B., Li, Y. B., and Zheng, Q. (2016). A new redshift measuring method for low-quality galaxy spectra based on multi resolution fusion distance. Spectrosc. Spectr. Analysis36, 1521–1525. doi:10.3964/j.issn.1000-0593(2016)05-1521-05
Paterson, K., Fong, W., Nugent, A., Escorial, A. R., Leja, J., Laskar, T., et al. (2020). Discovery of the optical afterglow and host galaxy of short GRB 181123B at z = 1.754: Implications for delay time distributions. Astrophysical J. Lett.898, L32. doi:10.3847/2041-8213/aba4b0
Penprase, B. E., Berger, E., Fox, D. B., Kulkarni, S. R., Kadish, S., Kerber, L., et al. (2006). Spectroscopy of GRB 051111 at z = 1.54948: Kinematics and elemental abundances of the GRB environment and host galaxy. Astrophysical J.646, 358–368. doi:10.1086/504678
Perley, D. A., Bloom, J. S., Butler, N. R., Pollack, L. K., Holtzman, J., Blake, C. H., et al. (2008a). The troublesome broadband evolution of GRB 061126: Does a gray burst imply gray dust?Astrophysical J.672, 449–464. doi:10.1086/523929
Perley, D. A., Bloom, J. S., Klein, C. R., Covino, S., Minezaki, T., Woźniak, P., et al. (2010). Evidence for supernova-synthesized dust from the rising afterglow of GRB 071025 at z ∼ 5. Mon. Notices R. Astronomical Soc.406, 2473–2487. doi:10.1111/j.1365-2966.2010.16772.x
Perley, D. A., Cenko, S. B., Bloom, J. S., Chen, H. W., Butler, N. R., Kocevski, D., et al. (2009). The host galaxies of Swift dark gamma-ray bursts: Observational constraints on highly obscured and very high redshift GRBs. Astronomical J.138, 1690–1708. doi:10.1088/0004-6256/138/6/1690
Perley, D. A., Krühler, T., Schady, P., Michałowski, M. J., Thöne, C. C., Petry, D., et al. (2017). A revised host galaxy association for GRB 020819B: A high-redshift dusty starburst, not a low-redshift gas-poor spiral. Mon. Notices R. Astronomical Soc. Lett.465, L89–L93. doi:10.1093/mnrasl/slw221
Perley, D. A., Krühler, T., Schulze, S., de Ugarte Postigo, A., Hjorth, J., Berger, E., et al. (2016a). THESWIFTGAMMA-RAY burst host galaxy legacy survey. I. Sample selection and redshift distribution. Astrophysical J.817, 7. doi:10.3847/0004-637X/817/1/7
Perley, D. A., Levan, A. J., Tanvir, N. R., Cenko, S. B., Bloom, J. S., Hjorth, J., et al. (2013). A population of massive, luminous galaxies hosting heavily dust-obscured gamma-ray bursts: Implications for the use of GRBs as tracers of cosmic star formation. Astrophysical J.778, 128. doi:10.1088/0004-637X/778/2/128
Perley, D. A., Li, W., Chornock, R., Prochaska, J. X., Butler, N. R., Chandra, P., et al. (2008b). Grb 071003: Broadband follow-up observations of a very bright gamma-ray burst in a galactic halo. Astrophysical J.688, 470–490. doi:10.1086/591961
Perley, D. A., Modjaz, M., Morgan, A. N., Cenko, S. B., Bloom, J. S., Butler, N. R., et al. (2012). The luminous infrared host galaxy of short-duration GRB 100206A. Astrophysical J.758, 122. doi:10.1088/0004-637X/758/2/122
Perley, D. A., and Perley, R. A. (2013). Radio constraints on heavily obscured star formation within dark gamma-ray burst host galaxies. Astrophysical J.778, 172. doi:10.1088/0004-637X/778/2/172
Perley, D. A., Tanvir, N. R., Hjorth, J., Laskar, T., Berger, E., Chary, R., et al. (2016b). The Swift GRB host galaxy legacy survey. II. Rest-Frame near-IR luminosity distribution and evidence for a near-solar metallicity threshold. Astrophysical J.817, 8. doi:10.3847/0004-637X/817/1/8
Piro, L., Frail, D. A., Gorosabel, J., Garmire, G., Soffitta, P., Amati, L., et al. (2002). The bright gamma-ray burst of 2000 february 10: A case study of an optically dark gamma-ray burst. Astrophysical J.577, 680–690. doi:10.1086/342226
Pisani, G. B., Ruffini, R., Aimuratov, Y., Bianco, C. L., Karlica, M., Kovacevic, M., et al. (2018). The first ICRANet catalog of binary-driven hypernovae. Eur. Phys. J. Web Conf.168, 04002. doi:10.1051/epjconf/201816804002
Price, P. A., Berger, E., Kulkarni, S. R., Djorgovski, S. G., Fox, D. W., Mahabal, A., et al. (2002). The unusually long duration gamma-ray burst GRB 000911: Discovery of the afterglow and host galaxy. Astrophysical J.573, 85–91. doi:10.1086/340585
Qiu, B., Hu, Z. Y., and Zhao, Y. H. (2002). A pseudo triangle technique for redshift identification of celestial spectra. Spectrosc. Spectr. Analysis22, 695–698. doi:10.3321/j.issn:1000-0593.2002.04.051
Rastinejad, J. C., Gompertz, B. P., Levan, A. J., Fong, W., Nicholl, M., Lamb, G. P., et al. (2022). A kilonova following a long-duration gamma-ray burst at 350 mc. arXiv e-prints , arXiv:2204.10864.
Rau, A., Salvato, M., and Greiner, J. (2005). The host of GRB/XRF 030528 - an actively star forming galaxy at z = 0.782. Astronomy Astrophysics444, 425–430. doi:10.1051/0004-6361:20053773
Reichart, D. E. (1998). The redshift of GRB 970508. Astrophysical J.495, L99–L101. doi:10.1086/311222
Rodney, S. A., Riess, A. G., Scolnic, D. M., Jones, D. O., Hemmati, S., Molino, A., et al. (2015). Two SNe ia at redshift ∼2: Improved classification and redshift determination with medium-band infrared imaging. Astronomical J.150, 156. doi:10.1088/0004-6256/150/5/156
Roming, P. W. A., Kennedy, T. E., Mason, K. O., Nousek, J. A., Ahr, L., Bingham, R. E., et al. (2005). The Swift ultra-violet/optical telescope. Space Sci. Rev.120, 95–142. doi:10.1007/s11214-005-5095-4
Roming, P. W. A., Schady, P., Fox, D. B., Zhang, B., Liang, E., Mason, K. O., et al. (2006a). Very early optical afterglows of gamma-ray bursts: Evidence for relative paucity of detection. Astrophysical J.652, 1416–1422. doi:10.1086/508481
Roming, P. W. A., Vanden Berk, D., Pal’shin, V., Pagani, C., Norris, J., Kumar, P., et al. (2006b). Grb 060313: A new paradigm for short-hard bursts?Astrophysical J.651, 985–993. doi:10.1086/508054
Rossi, A., de Ugarte Postigo, A., Ferrero, P., Kann, D. A., Klose, S., Schulze, S., et al. (2008). A photometric redshift of
Rossi, A., Frederiks, D. D., Kann, D. A., De Pasquale, M., Pian, E., Lamb, G., et al. (2022). A blast from the infant Universe: The very high-z GRB 210905A. Astronomy Astrophysics665, A125. doi:10.1051/0004-6361/202243225
Rossi, A., Piranomonte, S., Savaglio, S., Palazzi, E., Michałowski, M. J., Klose, S., et al. (2014). A quiescent galaxy at the position of the long GRB 050219A. Astronomy Astrophysics572, A47. doi:10.1051/0004-6361/201423865
Rowlinson, A., Wiersema, K., Levan, A. J., Tanvir, N. R., O’Brien, P. T., Rol, E., et al. (2010). Discovery of the afterglow and host galaxy of the low-redshift short GRB 080905A. Mon. Notices R. Astronomical Soc.408, 383–391. doi:10.1111/j.1365-2966.2010.17115.x
Saccardi, A., Vergani, S. D., De Cia, A., D’Elia, V., Heintz, K. E., Izzo, L., et al. (2023). Dissecting the interstellar medium of a z = 6.3 galaxy. X-shooter spectroscopy and HST imaging of the afterglow and environment of the Swift GRB 210905A. Astronomy Astrophysics671, A84. doi:10.1051/0004-6361/202244205
Sahu, K. C., Livio, M., Petro, L., Bond, H. E., Macchetto, F. D., Galama, T. J., et al. (1997). Observations of GRB 970228 and GRB 970508 and the neutron star merger model. Astrophysical J. Lett.489, L127–L131. doi:10.1086/316786
Salim, S., Rich, R. M., Charlot, S., Brinchmann, J., Johnson, B. D., Schiminovich, D., et al. (2007). UV star formation rates in the local universe. Astrophysical J. Suppl. Ser.173, 267–292. doi:10.1086/519218
Salvaterra, R., Campana, S., Vergani, S. D., Covino, S., D’Avanzo, P., Fugazza, D., et al. (2012). A complete sample of bright Swift long gamma-ray bursts. I. Sample presentation, luminosity function and evolution. Astrophysical J.749, 68. doi:10.1088/0004-637X/749/1/68
Salvaterra, R., Della Valle, M., Campana, S., Chincarini, G., Covino, S., D’Avanzo, P., et al. (2009). GRB090423 at a redshift of z˜8.1. Nature461, 1258–1260. doi:10.1038/nature08445
Salvaterra, R. (2015). High redshift gamma-ray bursts. J. High Energy Astrophysics7, 35–43. doi:10.1016/j.jheap.2015.03.001
Sánchez-Ramírez, R., Gorosabel, J., de Ugarte Postigo, A., Castro-Tirado, A. J., Thöne, C. C., Fynbo, J. P. U., et al. (2013). “Grb 100316A: An explosion at a high redshift galaxy,” in Highlights of Spanish astrophysics VII. Editors J. C. Guirado, L. M. Lara, V. Quilis, and J. Gorgas, 463.
Savaglio, S., Glazebrook, K., and Le Borgne, D. (2009). The galaxy population hosting gamma-ray bursts. Astrophysical J.691, 182–211. doi:10.1088/0004-637X/691/1/182
Schulze, S., Chapman, R., Hjorth, J., Levan, A. J., Jakobsson, P., Björnsson, G., et al. (2015). The optically unbiased GRB host (TOUGH) survey. VII. The host galaxy luminosity function: Probing the relationship between GRBs and star formation to redshift ∼ 6. Astrophysical J.808, 73. doi:10.1088/0004-637X/808/1/73
Selsing, J., Krühler, T., Malesani, D., D’Avanzo, P., Schulze, S., Vergani, S. D., et al. (2018). The host galaxy of the short GRB 111117A at z = 2.211. Impact on the short GRB redshift distribution and progenitor channels. Astronomy Astrophysics616, A48. doi:10.1051/0004-6361/201731475
Selsing, J., Malesani, D., Goldoni, P., Fynbo, J. P. U., Krühler, T., Antonelli, L. A., et al. (2019). The X-shooter GRB afterglow legacy sample (XS-GRB). Astronomy Astrophysics623, A92. doi:10.1051/0004-6361/201832835
Si, S. K., Qi, Y. Q., Xue, F. X., Liu, Y. J., Wu, X., Yi, S. X., et al. (2018). The three-parameter correlations about the optical plateaus of gamma-ray bursts. Astrophysical J.863, 50. doi:10.3847/1538-4357/aad08a
Skrutskie, M. F., Cutri, R. M., Stiening, R., Weinberg, M. D., Schneider, S., Carpenter, J. M., et al. (2006). The two micron all sky survey (2MASS). Astronomical J.131, 1163–1183. doi:10.1086/498708
Smith, K. L., Ridden-Harper, R., Fausnaugh, M., Daylan, T., Omodei, N., Racusin, J., et al. (2021). Grb 191016A: A long gamma-ray burst detected by tess. Astrophysical J.911, 43. doi:10.3847/1538-4357/abe6a2
Soderberg, A. M., Berger, E., Kasliwal, M., Frail, D. A., Price, P. A., Schmidt, B. P., et al. (2006). The afterglow, energetics, and host galaxy of the short-hard gamma-ray burst 051221a. Astrophysical J.650, 261–271. doi:10.1086/506429
Stanway, E. R., Levan, A. J., Tanvir, N., Wiersema, K., van der Horst, A., Mundell, C. G., et al. (2015). Grb 080517: A local, low-luminosity gamma-ray burst in a dusty galaxy at z = 0.09. Mon. Notices R. Astronomical Soc.446, 3911–3925. doi:10.1093/mnras/stu2286
Stratta, G., Basa, S., Butler, N., Atteia, J. L., Gendre, B., Pélangeon, A., et al. (2007). X-ray flashes or soft gamma-ray bursts?. The case of the likely distant XRF 040912. Astronomy Astrophysics461, 485–492. doi:10.1051/0004-6361:20065831
Svensson, K. M., Levan, A. J., Tanvir, N. R., Fruchter, A. S., and Strolger, L. G. (2010). The host galaxies of core-collapse supernovae and gamma-ray bursts. Mon. Notices R. Astronomical Soc.405, 57–76. doi:10.1111/j.1365-2966.2010.16442.x
Tan, W. W., Cao, X. F., and Yu, Y. W. (2013). Determining the luminosity function of Swift long gamma-ray bursts with pseudo-redshifts. Astrophysical J. Lett.772, L8. doi:10.1088/2041-8205/772/1/L8
Tanvir, N. R., Fox, D. B., Levan, A. J., Berger, E., Wiersema, K., Fynbo, J. P. U., et al. (2009). A γ-ray burst at a redshift of z˜8.2. Nature461, 1254–1257. doi:10.1038/nature08459
Tanvir, N. R., Fynbo, J. P. U., de Ugarte Postigo, A., Japelj, J., Wiersema, K., Malesani, D., et al. (2019). The fraction of ionizing radiation from massive stars that escapes to the intergalactic medium. Mon. Notices R. Astronomical Soc.483, 5380–5408. doi:10.1093/mnras/sty3460
Tanvir, N. R., and Jakobsson, P. (2007). Observations of GRBs at high redshift. Philosophical Trans. R. Soc. Lond. Ser. A365, 1377–1384. doi:10.1098/rsta.2006.1992
Tanvir, N. R., Laskar, T., Levan, A. J., Perley, D. A., Zabl, J., Fynbo, J. P. U., et al. (2018). The properties of GRB 120923A at a spectroscopic redshift of z ≈ 7.8. Astrophysical J.865, 107. doi:10.3847/1538-4357/aadba9
Tanvir, N. R., Levan, A. J., Rol, E., Starling, R. L. C., Gorosabel, J., Priddey, R. S., et al. (2008). The extreme, red afterglow of GRB 060923A: Distance or dust?Mon. Notices R. Astronomical Soc.388, 1743–1750. doi:10.1111/j.1365-2966.2008.13496.x
Thöne, C. C., Greiner, J., Savaglio, S., and Jehin, E. (2007). ISM studies of GRB 030329 with high-resolution spectroscopy. Astrophysical J.671, 628–636. doi:10.1086/522558
Tinney, C., Stathakis, R., Cannon, R., Galama, T., Wieringa, M., Frail, D. A., et al. (1998). Grb 980425. IAU Circ.6896, 3.
Tonry, J., and Davis, M. (1979). A survey of galaxy redshifts. I. Data reduction techniques. Astronomical J.84, 1511–1525. doi:10.1086/112569
Tsvetkova, A., Frederiks, D., Golenetskii, S., Lysenko, A., Oleynik, P., Pal’shin, V., et al. (2017). The konus-wind catalog of gamma-ray bursts with known redshifts. I. Bursts detected in the triggered mode. Astrophysical J.850, 161. doi:10.3847/1538-4357/aa96af
Tu, L. P., Luo, A. L., Jiang, B., Wei, P., Zhao, Y. H., and Liu, R. (2012). A novel method to determine the redshifts of active galaxies based on wavelet transform. Spectrosc. Spectr. Analysis32, 2858–2862. doi:10.3964/j.issn.1000-0593(2012)10-2858-05
Van Paradijs, J., Groot, P. J., Galama, T., Kouveliotou, C., Strom, R. G., Telting, J., et al. (1997). Transient optical emission from the error box of the γ-ray burst of 28 February 1997. Nature386, 686–689. doi:10.1038/386686a0
Volnova, A. A., Pozanenko, A. S., Gorosabel, J., Perley, D. A., Frederiks, D. D., Kann, D. A., et al. (2014). Grb 051008: A long, spectrally hard dust-obscured GRB in a lyman-break galaxy at z ≈ 2.8. Mon. Notices R. Astronomical Soc.442, 2586–2599. doi:10.1093/mnras/stu999
Vreeswijk, P. M., Ledoux, C., Raassen, A. J. J., Smette, A., De Cia, A., Woźniak, P. R., et al. (2013). Time-dependent excitation and ionization modelling of absorption-line variability due to GRB 080310. Astronomy Astrophysics549, A22. doi:10.1051/0004-6361/201219652
Vreeswijk, P. M., Ledoux, C., Smette, A., Ellison, S. L., Jaunsen, A. O., Andersen, M. I., et al. (2007). Rapid-response mode VLT/UVES spectroscopy of GRB 060418: Conclusive evidence for UV pumping from the time evolution of Fe II and Ni II excited- and metastable-level populations. Astronomy Astrophysics468, 83–96. doi:10.1051/0004-6361:20066780
Wadadekar, Y. (2005). Estimating photometric redshifts using Support vector machines. Astronomical Soc. Pac.117, 79–85. doi:10.1086/427710
Wang, D., Zhang, Y. X., Liu, C., and Zhao, Y. H. (2008). Two novel approaches for photometric redshift estimation based on SDSS and 2MASS. Chin. J. Astronomy Astrophysics8, 119–126. doi:10.1088/1009-9271/8/1/13
Wang, J., Qiu, Y.-L., and Wei, J.-Y. (2020). A pilot study of catching high-z GRBs and exploring circumburst environment in the forthcoming SVOM era. Res. Astronomy Astrophysics20, 124. doi:10.1088/1674-4527/20/8/124
Wei, J., Cordier, B., Antier, S., Antilogus, P., Atteia, J. L., Bajat, A., et al. (2016). The Deep and transient universe in the SVOM era: New challenges and opportunities - scientific prospects of the SVOM mission. arXiv e-prints , arXiv:1610.06892.
Wei, J. J., Wu, X. F., Wang, F. Y., Liu, Z., Dai, Z. G., and Zhang, B. (2015). “High redshift gamma-ray bursts as a probe of the early universe and first stars,” in The 3rd einstein probe science workshop. SCIENTIA SINICA physica, mechanica & astronomica). Editor X. F. Wu, 1–30.
Wijers, R. A. M. J., Rees, M. J., and Meszaros, P. (1997). Shocked by GRB 970228: The afterglow of a cosmological fireball. Mon. Notices R. Astronomical Soc.288, L51–L56. doi:10.1093/mnras/288.4.L51
Woosley, S. E., and Bloom, J. S. (2006). The supernova gamma-ray burst connection. Annu. Rev. Astronomy Astrophysics44, 507–556. doi:10.1146/annurev.astro.43.072103.150558
Xie, L., Wang, X. G., Zheng, W., Filippenko, A. V., Qin, S. M., Li, L., et al. (2020). Early optical observations of GRB 150910A: Bright jet optical afterglow and X-ray dipole radiation from a magnetar central engine. Astrophysical J.896, 4. doi:10.3847/1538-4357/ab8d2a
Xu, X., Luo, A. L., Wu, F. C., and Zhao, Y. H. (2006a). Using neural networks based template matching method to obtain redshifts of normal galaxies. Spectrosc. Spectr. Analysis25, 996–1001. doi:10.3321/j.issn:1000-0593.2005.06.050
Xu, X., Wu, F. C., Hu, Z. Y., and Luo, A. L. (2006b). A novel method for the determination of redshifts of normal galaxies by non-linear dimensionality reduction. Spectrosc. Spectr. Analysis26, 182–186. doi:10.3321/j.issn:1000-0593.2006.01.049
Yuan, H. Y., Lü, H. J., Li, Y., Zhang, B. B., Sun, H., Rice, J., et al. (2022). Probing the progenitor of high-z short-duration GRB 201221D and its possible bulk acceleration in prompt emission. Res. Astronomy Astrophysics22, 075011. doi:10.1088/1674-4527/ac712d
Yue, C., Hu, Q., Zhang, F. W., Liang, Y. F., Jin, Z. P., Zou, Y. C., et al. (2018). How special is GRB 170817A?Astrophysical J. Lett.853, L10. doi:10.3847/2041-8213/aaa66c
Zaninoni, E., Bernardini, M. G., Margutti, R., Oates, S., and Chincarini, G. (2013). Gamma-ray burst optical light-curve zoo: Comparison with X-ray observations. Astronomy Astrophysics557, A12. doi:10.1051/0004-6361/201321221
Zhang, B. (2007). Gamma-ray burst afterglows. Adv. Space Res.40, 1186–1198. doi:10.1016/j.asr.2007.01.016
Zhou, H., Huang, L. Y., and Luo, M. L. (2000). A stratified approach for quasar recognition based on hough transform and neural network. J. Electron. Inf. Technol.2000, 529–535. doi:10.1007/BF02948846
Keywords: gamma-ray bursts, redshift, measurement methods, afterglow, host galaxy
Citation: Li M, Kang Z, Wu C, Liu C, Mao J, Li Z, Deng S, Niu B and Jiang P (2023) Measurement methods for gamma-ray bursts redshifts. Front. Astron. Space Sci. 10:1124317. doi: 10.3389/fspas.2023.1124317
Received: 15 December 2022; Accepted: 12 June 2023;
Published: 05 July 2023.
Edited by:
Matthew Parry, University of Otago, New ZealandReviewed by:
Massimo Della Valle, Astronomical Observatory of Capodimonte (INAF), ItalyNial Tanvir, University of Leicester, United Kingdom
Copyright © 2023 Li, Kang, Wu, Liu, Mao, Li, Deng, Niu, and Jiang. This is an open-access article distributed under the terms of the Creative Commons Attribution License (CC BY). The use, distribution or reproduction in other forums is permitted, provided the original author(s) and the copyright owner(s) are credited and that the original publication in this journal is cited, in accordance with accepted academic practice. No use, distribution or reproduction is permitted which does not comply with these terms.
*Correspondence: Chao Wu, Y3d1QG5hby5jYXMuY24=; Chengzhi Liu, bGN6QGNoby5hYy5jbg==