- 1Organization for Research Promotion, Osaka Metropolitan University, Osaka, Japan
- 2Graduate School of Agriculture, Osaka Metropolitan University, Osaka, Japan
There is a need to develop production technology that effectively uses limited water and other resources to create a stable food supply in space. Aquaponics, which combine hydroponics and aquaculture, is expected to be an efficient system for producing crops and animal proteins. This system sustains the reuse of water and balances nutrient elements between both cultures using dissolved elements in fish excrement for plant growth. To evaluate the effect of fish density on biological production and nitrogen usage efficiency in aquaponics combining lettuce hydroponics and loach aquaculture, we investigated the growth performance of lettuce plants and loach fish. We focused on the balance of nutrient elements, especially nitrogen flow in the system. As a result, we found that lettuce grew in aquaponics with a half-strength standard solution with an optimal combination of the number of plants and fish as well as hydroponics with a standard solution. Increasing the density of loach fish and lettuce plants can increase the total biological production of fish and plants. However, it will be important to control both fish and plant densities to increase nitrogen recovery in aquaponics with a high fish density.
1 Introduction
Plant production in space has become a growing concern as the possibility of long-term manned space flights and human habitation on the moon increases. The feasibility of such missions depends heavily on the availability of crops grown in Controlled Ecological Life Support Systems (CELSS) or space farms. These crops will provide food, CO2/O2 conversion, and water purification for the crew. For long-term manned space missions and human habitation in space, space food and nutrition systems should be based on space farming, with scheduled crop production that yields high volumes and has a rapid turnover rate. The development of production technology that optimizes the use of limited water resources to create a stable food supply is also crucial for success in space. Although plant materials are an important source of nutrients, they alone are insufficient to meet the needs necessary for maintaining human health. Essential amino acids, which cannot be synthesized in the human body, are critical for maintaining physique and longevity. Richter et al. (2016) pointed out that obtaining complete nutrition from a purely plant-based diet (vegan diet) is impossible or difficult without considering the proper amounts and combinations. They reported that the most important missing nutrient is vitamin B12, and other potentially deficient nutrients include protein, essential amino acids, long-chain n-3 polyunsaturated fatty acids, other vitamins (riboflavin, vitamin D), and minerals (calcium, iron, iodine, zinc, selenium). Therefore, animal proteins must be consumed to obtain certain vitamins and amino acids necessary for human health.
Aquaponics, a biological production system that combines hydroponics and aquaculture, can provide a stable supply of plant and animal ingredients while effectively using limited water resources. In this system, nutrients dissolved in fish excretion are recycled and used to promote plant growth. Using material reuse, aquaponics reduces waste and creates a sustainable production model. Aquaponics is an efficient system for water and nutrient use (e.g., Al-Hafedh et al., 2008; Wongkiew et al., 2017). By sharing water between hydroponics and aquaculture systems, plants can use the nutrient-rich components derived from fish excrement to grow. This allows for food production while simultaneously recycling nutrients and converting waste into valuable resources.
The nitrogen balance has been the main focus of aquaponics research on Earth (e.g., Hu et al., 2015), due to its importance for plant growth (Xu et al., 2012) and the availability, concentration, and accumulation of nitrogen compounds (Goddek et al., 2016). As a biological production system combining hydroponics and aquaculture, a thorough understanding of nitrogen balance is necessary to increase system productivity. Aquaponics can produce animal protein and semi-complete nutritional food crops that are the ingredients providing the best balance of human daily nutritional requirements, as demonstrated by Islam et al. (2022) with high-nutritional sweet potato plants (Kitaya et al., 2021) and tilapia fish. Garlic plants and tilapia fish have also been successfully grown in aquaponics, promoting biosynthesis of the medicinally important metabolite ‘ajoene’ in garlic plants compared to no fish (Naznin et al., 2015).
Most studies on aquaponics conducted on Earth have used tilapia and catfish in aquaculture due to their high growth rates and edibility (e.g., Graber and Junge, 2009; Nuwansi et al., 2016). However, their heads, internal organs, and bones are inedible, which is a disadvantage. In contrast, loach has a 100% edible portion and high nutritional value, as recognized by the Japan Ministry of Education, Culture, Sports, Science and Technology (2015). Loach is also a traditional Japanese ingredient. Additionally, loaches are capable of breathing through their intestines, making them resistant to dissolved oxygen deficiency. Thus, loach was selected as the fish species to be cultured in this study.
For the perspective of aquaponics in bio-regenerative life support systems (BLSS) in space, Przybyla (2021) and Brown et al. (2021) reviewed the possibility of space aquaculture. They also emphasized the importance of a closed aquaponic system for water and material recycling as well as a balanced nutritional intake (proteins, lipids, and carbohydrates) in a long-term human stay in space.
Many studies have investigated aquaponic culture with various plant types on Earth. In this study, lettuce was selected as the food plant to be cultured, as it is a common vegetable in plant factory culture and has a wealth of physiological and ecological data on environmental responses. We are assessing the effectiveness of fish excreta-derived components as fertilizers for plant growth in aquaponics, using a combination of loach aquaculture and lettuce hydroponics for integration into CELSS in space. We are particularly examining the nitrogen compound flow within the system, as it is an essential element for plants and is abundant in fish excreta while also being harmful to fish.
This study examined the impact of fish density on the biological production rate by manipulating fish density on Earth. Furthermore, the nitrogen balance was analyzed.
2 Materials and methods
2.1 General matters
Lettuce (Lactuca sativa L., ‘crunch’) and loach (Misgurnus anguillicaudatus) were cultured for 21 days on Earth. Each culture container in six test groups described later was filled with 12 L of water and stocked with loaches (initial average weight 2.9 g/fish) in the rootzone of lettuce plants in each container. The number of loaches per container ranged from 6 to 30, depending on the density. Twenty-one-day-old lettuce seedlings (fresh weight of 2.8 ± 0.2 g/plant) were planted in the holes, spaced 5 cm apart in a grid pattern (Figure 1).
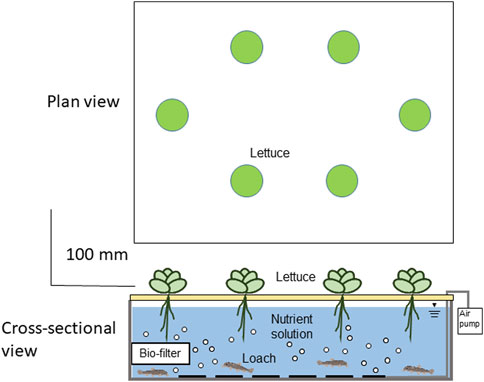
FIGURE 1. A schematic diagram of the aquaponics experiment combining lettuce hydroponics and loach aquaculture.
Six lettuce plants were grown in each test group under controlled environmental conditions, with a temperature of 25°C, relative humidity of 70%, a CO2 concentration of 700 μmol mol−1, a photosynthetic photon flux density of 200 μmol m−2 s−1, and a light period of 16 h d−1. The light source used was LEDs (LDL-B20T 50/08/10-KOD, Sanwa Seimitsu Co., Ltd.). The nutrient solution was made from dechlorinated tap water. Continuous aeration (3.5 L min−1) was provided to the nutrient solution. Loaches were fed once a day, five times a week, with commercially available goldfish feed (Sakihikari, Kyorin Co., Ltd.) equivalent to 1% of their body weight (about 0.03 g/fish) for 2 weeks before starting the experiment. The component of fish feed used are shown in Table 1.
Prior to the experiment, a biofilter consisting of non-woven fabric as a carrier for microorganisms was placed in the loach culture tank for a period of 3 weeks to facilitate the colonization of nitrite and nitrate bacteria.
Due to the inadequacy of certain fertilizer components required for lettuce growth from loach excrement alone in our preliminary experiment, we supplemented with a chemical nutrient solution. The additional medium used was a half-strength OAT House A-formula solution (OAT Agrio Co., Ltd.). The electrical conductivity of the culture medium was adjusted to about 1.2 dS m−1. The pH in the solution was adjusted to about 6.0. The chemical composition of the half-strength nutrient solution is shown in Table 2.
Fresh weight measurements of lettuce and loach, along with ammonium nitrogen, nitrite nitrogen, nitrate-nitrogen concentration, pH, and electrical conductivity of the nutrient solution, were conducted weekly. On the final day of the experiment, the dry weight and nitrogen content of lettuce and loach were determined. To measure the dry weight, the harvested lettuce and loach were dried for approximately 7 days in a constant temperature drying oven at 80°C.
For solution analysis, the ammonium nitrogen concentration was determined using a spectrophotometer (AT-2000, Central Kagaku Co., Ltd., Japan), nitrite nitrogen concentration was measured using a simple reflection photometer (RQ flux plus 10, Kanto Kagaku Co., Ltd., Japan), and nitrate-nitrogen concentration was measured using a compact nitrate ion meter (LAQUAtwin NO3-11C, Horiba, Co., Ltd., Japan). The pH was measured using a compact pH meter (LAQUAtwin pH-33B, Horiba, Co., Ltd. Japan).
To analyze the nitrogen content of loach and lettuce, three individuals of loach and the edible parts (leaves and stems) of six individuals of lettuce from each group were powdered. The nitrogen content was measured using the heated persulfate decomposition method and an absorption photometer (DR-3900, DKK-Toa Co., Ltd., Japan).
The relative growth rate (RGR), a growth index for lettuce and loach, was calculated using Eq. 1. Additionally, the nitrogen usage efficiency (NUE), which represents the ratio of the accumulated nitrogen in lettuce and loach to the input nitrogen, was calculated using Eq. 2.
In Eq. 1, W1 represents the dry weight (g) of plants and fish at time t1, and W2 represents the dry weight at time t2, where t1 and t2 represent the times of transplanting and harvesting (in days). W1 was calculated from the actual measured fresh weight of each individual at t1 by using the Dry weight/Fresh weight ratio calculated from sample individuals previously obtained. In Eq. 2, Ninput represents the amount of nitrogen (g) contained in the applied chemical fertilizer and feed, Nplant represents the amount of nitrogen (g) contained in lettuce, and Nfish represents the amount of nitrogen (g) contained in loach.
2.2 Test groups
Six test groups were established, comprising five aquaponic groups and one hydroponic group. Additionally, to determine the quantity of dissolved nitrogen released from 20 loaches, a test group was established where only loaches were cultured in the same hydroponic solution in a tank of the same size. Each aquaponic group consisted of 6 plants and 6, 12, 18, 24, and 30 loach fish (Table 1). Each individual loach was fed 0.06 g d−1. A commercial feed for goldfish (Sakihikari, Kyorin Co., Ltd.) was fed twice a day with an interval of more than 3 h during the experimental period.
2.3 Nitrogen contents
The nitrogen content (g) of each component in the system was calculated using the following procedure: The amount of nitrogen contained in the nutrient solution of each test group was calculated based on the nutrient solution composition specified by the manufacturer. The amount of nitrogen contained in the feed provided to the fish was calculated by multiplying the nitrogen content (g g−1) contained in the feed by the total feed amount (g). No leftover feed remained during the experiment. The amount of nitrogen contained in the excretion from loaches was calculated as the amount of nitrogen derived from loach emissions, which was determined by measuring the total amount (g) of dissolved nitrogen components discharged by 20 loaches over 21 days in the test group where only loaches were cultured.
2.4 Statistical analysis
Significant differences were tested using the Tukey–Kramer method and expressed as the presence or absence of significant differences at a level of p < 0.05.
3 Results and discussion
3.1 Nitrogen compound concentration in the solution
As the fish density increased, so did the amount of feed and input nitrogen. This caused an increase in the amount of excrement and an accompanying increase in ammonium nitrogen concentration in the solution, as shown in Figure 2. Nitrite nitrogen concentrations also increased with increasing fish density, but remained at low levels. The nitrate-nitrogen concentration reached its maximum at a fish density of 1.5 fish L−1. The hydroponic group, which had a fish density of 0 fish L−1, had lower ammonium nitrogen and nitrite nitrogen concentrations than the aquaponic groups. The increase in nitrite nitrogen concentration and decrease in nitrate-nitrogen concentration at high loach densities may be due to the denitrification of nitrate. The insufficient water circulation in the nutrient solution tank may have led to a local micro-partial anaerobic environment, but further details are unknown since the distribution of dissolved oxygen, especially in the biofilter, was not measured in this experiment.
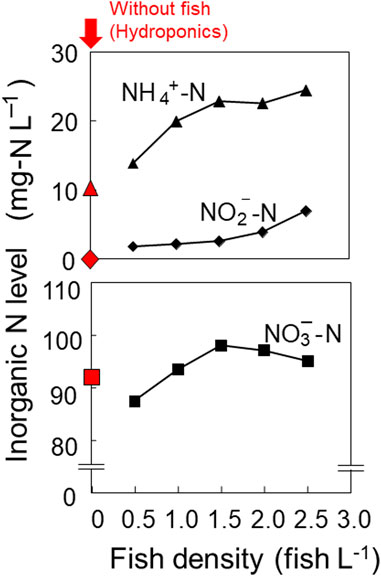
FIGURE 2. Concentrations of ammonium nitrogen, nitrite nitrogen, and nitrate nitrogen in the solution for each fish density during the experimental period.
3.2 Growth performance
On the final day of the experiment, lettuce plants showed no visible differences among the test groups (Figure 3). There was no significant difference (p < 0.05) in the lettuce biomass in the aquaponic and hydroponic groups (Table 3). Similarly, the RGR of lettuce plants did not change with increasing fish density (Figure 4), and there was no significant difference in the RGR of lettuce plants in the test groups. It has been reported that lettuce growth can be inhibited by the accumulation of nitrite in solution (Hoque et al., 2007). However, in this experiment, the nitrite level did not inhibit lettuce growth.
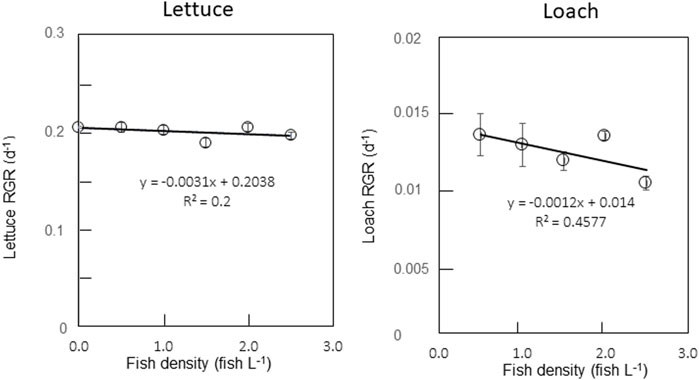
FIGURE 4. Effects of fish density on relativegrowth rates (RGRs) of lettuce plants and loach fish Error bars indicate standard errors.
On the last day of the experiment, the fresh weight of loach fish did not show any significant differences but tended to decrease with increasing fish density (Table 3). The RGR of loach also tended to decrease with increasing fish density (Figure 4). The increase in nitrite and ammonium ions in the culture solution (Figure 2) seemed to cause a slight decrease in the RGRs of loach, whereas there were no significant differences among the test groups. The accumulation of nitrite ions and ammonium ions inhibited loach growth, but the effect was not significant.
3.3 Biological production
The dry weight of the loach in each group is shown in Figure 5, which reveals an increase in loach production with increasing fish density. However, the growth rate of the loach tended to decrease as the fish density increased. Despite this, the loach population increased with the fish density, leading to an overall increase in production. In contrast, the RGR of lettuce was consistent across all fish densities, and the lettuce production was not affected by the fish density (Figure 5). Therefore, the total production of both lettuce and loach tended to increase as the fish density increased (Figure 5).
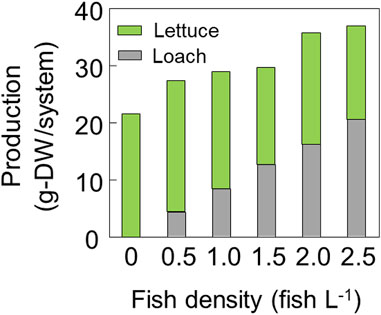
FIGURE 5. Dry mass production of lettuce plants and loach fish in the system as affected by fish density.
3.4 Nitrogen usage efficiency
Contents of nitrogen accumulated during the experimental period in lettuce plants and loach fish as affected by fish density are shown in Figure 6. The nitrogen contents in lettuce plants and loach fish tended to decrease with increasing fish density. These tendencies are partly due to the difference in the accumulated amount of biomass, but investigation of the reason requires more detailed analysis such as the type of nitrogen compounds.
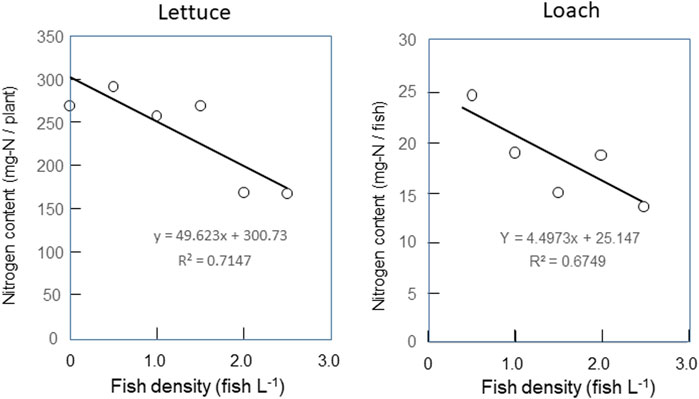
FIGURE 6. Effects of fish density on contents of nitrogen accumulated during the experimental period in lettuce plants and loach fish.
As shown in Figure 7, the NUE of lettuce decreased with increased fish density. This can be attributed to the increase in input feed and nitrogen with increasing fish density, which led to the accumulation of unused nitrogen in the nutrient solution. Despite the constant RGR of lettuce, the increase in fish density did not result in a proportional increase in nitrogen uptake by lettuce, causing a decrease in NUE. In contrast, the NUE of loaches remained constant as the amount of food ingested by loaches did not change with increasing fish density. The total NUE of loach and lettuce decreased with increasing fish density, except for the 0.5 fish L-1 group, where there was a one-to-one ratio of lettuce plant to loach fish, and the efficiency was almost similar to that of hydroponics.
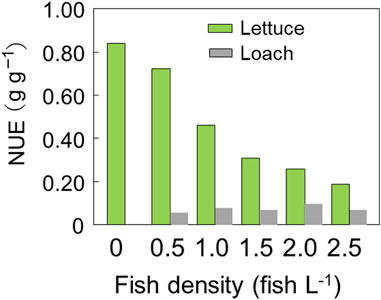
FIGURE 7. Nitrogen usage efficiency of lettuce leaves and stems and loach fish as affected by fish density.
In the nitrogen balance, approximately 70% of the nitrogen from the feed was used by growing lettuce plants, while around 25% of the nitrogen from the feed was accumulated in loach fish. In all aquaponic groups, the end-of-experiment dissolved component was less than 5% of the supply, indicating that nitrogen was fully used in the entire system. The results in this study confirmed the facts of the usefulness of aquaponics from the viewpoint of the availability, concentration, and accumulation of nitrogen compounds (Goddek et al., 2016). However, as excessive nitrogen supply can decrease usage efficiency, further investigations are needed to determine how an increased nitrogen supply in an aquaponic system could affect the system’s NUE. In addition, to find the optimal balance between plants and fish for efficiently accumulating nitrogen as a food source, a more detailed analysis, such as the type of nitrogen compounds in plants and fish is required.
In the long-term operation of aquaponics, it is also necessary to manage the mineral components other than the nitrogen compounds so that they are neither excessive nor deficient. For example, Lantovololona and Adavelo (2020) reported successful aquaponics that combined loach and lettuce, but the possibility of potassium deficiency for the lettuce growth.
In this study, we did not monitor the microbial communities. The monitoring and regulation of microbes will be important, especially for stable and safe production in the long term.
4 Conclusion
In this study, lettuce plants showed no difference in growth when a portion of the chemical fertilizer was replaced with the excretory component of loach fish. This suggests that loach excreta can be used to partially supplement chemical fertilizer. Aquaponics shows promise in reducing the environmental impact of aquaculture by enabling the simultaneous production of plants and fish, reducing the need for chemical fertilizers in lettuce hydroponics, and purifying aquaculture water.
It is essential to increase plant nitrogen absorption, for example, by increasing planting density, particularly to reduce unused nitrogen and improve nitrogen recovery in food production. Future studies should examine not only nitrogen but also other nutritional elements to model the optimal combination of loach, lettuce, and feed supply.
Due to the recent global population growth, there are concerns about ensuring a stable food supply and conserving water resources on Earth. The spin-off technology of aquaponic development in CELSS can provide valuable knowledge to meet the urgent need for developing production technology that uses water and other resources efficiently to create a stable food supply on Earth.
Data availability statement
The raw data supporting the conclusion of this article will be made available by the authors, without undue reservation.
Ethics statement
The manuscript presents research on animals that do not require ethical approval for their study.
Author contributions
YK contributed to conception and design of the study and wrote the first draft of the manuscript. TK mainly conducted the experiment with support of TS and RE. All authors contributed to the article and approved the submitted version.
Conflict of interest
The authors declare that the research was conducted in the absence of any commercial or financial relationships that could be construed as a potential conflict of interest.
Publisher’s note
All claims expressed in this article are solely those of the authors and do not necessarily represent those of their affiliated organizations, or those of the publisher, the editors and the reviewers. Any product that may be evaluated in this article, or claim that may be made by its manufacturer, is not guaranteed or endorsed by the publisher.
References
Al-Hafedh, Y., Alam, A., and Beltagi, M. S. (2008). Food production and water conservation in a recirculating aquaponic system in Saudi arabia at different ratios of fish feed to plants. J. World Aquac. Soc. 39 (4), 510–520. doi:10.1111/j.1749-7345.2008.00181.x
Brown, L., Peick, J., Pickett, M., Fanara, T., Gilchrist, S., Smiley, A., et al. (2021). Aquatic invertebrate protein sources for long-duration space travel. Life Sci. Space Res. 28, 1–10. doi:10.1016/j.lssr.2020.10.002
Goddek, S., Espinal, C. A., Delaide, B., Jijakli, M. H., Schmautz, Z., Wuertz, S., et al. (2016). Navigating towards decoupled aquaponic systems: A system dynamics design approach. Water 8, 303. doi:10.3390/w8070303
Graber, A., and Junge, R. (2009). Aquaponic systems: nutrient recycling from fish wastewater by vegetable production. Desalination 246, 147–156. doi:10.1016/j.desal.2008.03.048
Hoque, M. M., Ajwa, H. A., and Smith, R. (2007). Nitrite and ammonium toxicity on lettuce grown under hydroponics. Commun. Soil Sci. Plant Analysis 39, 207–216. doi:10.1080/00103620701759194
Hu, Z., Lee, J. W., Chandran, K., Kim, S., Brotto, A. C., and Khanal, S. K. (2015). Effect of plant species on nitrogen recovery in aquaponics. Bioresour. Technol. 188, 92–98. doi:10.1016/j.biortech.2015.01.013
Islam, A. F. M. S., Hirai, H., and Kitaya, Y. (2022). An aquaponic system with hydroponic culture of sweet potato and tilapia culture. J. Agric. Ecol. Res. Int. 23 (6), 62–72. doi:10.9734/jaeri/2022/v23i6499
Japan Ministry of Education, Culture, Sports, Science and Technology (2015). Standard tables of food composition in Japan, 2020. 8th Edn. Available at: https://www.mext.go.jp/a_menu/syokuhinseibun/mext_01110.html.
Kitaya, Y., Higashi, K., Shibuya, T., and Endo, R. (2021). Fundamental study on plant-based regenerative life-support systems with sweetpotato culture in space. Trans. JSASS Aerosp. Tech. Jpn. 19 (6), 889–891. doi:10.2322/tastj.19.889
Lantovololona, F. M. S., and Adavelo, J. M., 2021, Crop production in a loach (Misgurnus anguillicandatus) – lettuce (Lactuca saliva) raft aquaponics system, Proceedings of the the 2020 Global Conference on Aquaculture, Shanghai, China, 22–25. September 2021.
Naznin, M. T., Kitaya, Y., Shibuya, T., Hirai, H., Endo, R., and Lefsrud, M. (2015). Integrated culture of garlic and tilapia fish -source of protein and medicinal compound ajoene. ActaHortic 1098, 95–101. doi:10.17660/actahortic.2015.1098.9
Nuwansi, K. K. T., Verma, A. K., Prakash, C., Tiwari, V. K., Chandrakant, M. H., Shete, A. P., et al. (2016). Effect of water flow rate on polyculture of koi carp (Cyprinus carpio var. koi) and goldfish (Carassius auratus) with water spinach (Ipomoea aquatica) in recirculating aquaponic system. Aquac. Int. 24, 385–393. doi:10.1007/s10499-015-9932-5
Przybyla, C. (2021). Space aquaculture: prospects for raising aquatic vertebrates in a bioregenerative life-support system on a lunar base. Front. Astronomy Space Sci. 8, 1–16. doi:10.3389/fspas.2021.699097
Richter, M., Boeing, H., Grünewald-Funk, D., Heseker, H., Kroke, A., Leschik-Bonnet, E., et al. (2016). Vegan det - position of the German nutrition society (DGE). Ernaehrungs Umsch. Int. 63 (04), 92–102. doi:10.4455/eu.2016.021
Wongkiew, S., Hu, Z., Chandran, K., Lee, J. W., and Khanal, S. K. (2017). Nitrogen transformations in aquaponic systems: A review. Aquac. Eng. 76, 9–19. doi:10.1016/j.aquaeng.2017.01.004
Keywords: aquaculture, CELSS, hydroponics, plant production, space farm
Citation: Kitaya Y, Kawamoto T, Endo R and Shibuya T (2023) Effect of fish density on biological production in aquaponics combining lettuce hydroponics and loach aquaculture for controlled ecological life support systems in space. Front. Astron. Space Sci. 10:1197402. doi: 10.3389/fspas.2023.1197402
Received: 31 March 2023; Accepted: 28 August 2023;
Published: 18 September 2023.
Edited by:
Luigi Gennaro Izzo, University of Naples Federico II, ItalyReviewed by:
Jan Hendrik Bredehöft, University of Bremen, GermanySarah Milliken, University of Greenwich, United Kingdom
Copyright © 2023 Kitaya, Kawamoto, Endo and Shibuya. This is an open-access article distributed under the terms of the Creative Commons Attribution License (CC BY). The use, distribution or reproduction in other forums is permitted, provided the original author(s) and the copyright owner(s) are credited and that the original publication in this journal is cited, in accordance with accepted academic practice. No use, distribution or reproduction is permitted which does not comply with these terms.
*Correspondence: Yoshiaki Kitaya, a2l0YXlhQG9tdS5hYy5qcA==