Prospect for measurements of (γ, n) reaction cross-sections of p-nuclei at ELI-NP
- 1Extreme Light Infrastructure-Nuclear Physics (ELI-NP), Horia Hulubei National Institute for Physics and Nuclear Engineering (IFIN-HH), Măgurele, Romania
- 2Department of Physics, Faculty of Science, Istanbul University, Istanbul, Türkiye
The gamma beam system under construction at the ELI-NP facility in Romania is projected to give the nuclear physics community access to an experimental system providing a high-intensity, narrow bandwidth photon beam at variable energy. With high-efficiency detector systems in place, the experimental programme will have a strong potential for in-depth studies of rare stable isotopes originating from the astrophysical p-process. In particular, the neutron detection systems are already implemented through a dedicated 3He long neutron counter array, called ELIGANT-TN, that is completed and in use. In this mini-review, we will give a summary of the current status of existing (γ, n) cross-section data, as well as the methods to obtain them, and highlight the future potential to expand and improve such data using the ELI-NP instrumentation and beam-lines.
1 Introduction
The nucleosynthesis of elements above iron and their isotopes mainly proceeds by neutron capture in the s- and r-processes. However, there are 35 stable proton-rich isotopes which these processes cannot produce. These so-called p-nuclei are believed to be produced by proton capture and γ dissociation processes in proton-rich stellar environments in the p-process. More specifically, the p-process is a combination of (p, γ), (γ, p), (γ, α), and (γ, n) reactions complemented by β+ decay and (n, γ) reactions. Many p-nuclei are underproduced in astrophysical model calculations and while these reactions take place at high temperatures and the ground state cross-sections themselves have a negligible contribution in stellar environmentes (Mohr, 2004; Utsunomiya et al., 2006a; Rauscher, 2012; Rauscher, 2013; Rauscher, 2014); experimental data on the γ dissociation cross-sections are needed to improve and develop theoretical models that can be implemented in calculations of the stellar reaction rates. Since the abundances of p-nuclei are low, high-intensity γ-ray beams are required for the (γ, n) measurements on enriched targets. The Extreme Light Infrastructure–Nuclear Physics (ELI-NP) facility is projected to have the capability of producing brilliant γ-ray beams suitable for such studies (Filipescu et al., 2015; Gales et al., 2016; Gales et al., 2018; Tanaka et al., 2020), with a higher energy resolution,
2 Review of cross-section data and methods
2.1 Measurement methods and beams
The generation of γ-ray beams dates back to Baldwin and Klaiber (1947), who generated a photon beam via the Bremsstrahlung process from a 100-MeV betatron accelerator. The Bremsstrahlung process, where electrons or other charged particles emit photons while decelerating in a dense matter, has remained one of the primary methods for producing the beams necessary for photodisintegration reaction studies throughout the decades. For a complete historical overview and a thorough discussion about current photonuclear topics, a review of the status and history of the field of photonuclear physics was recently published by Zilges et al. (2022). Here, we will focus on the aspects of (γ, n) cross-section measurements.
As the Bremsstrahlung beams are generated by slowing down, typically, electrons, the beams produced have some drawbacks regarding photonuclear cross-section measurements. While easy to produce, the energy spectra are extending as a modified power law from the lowest energy to the electron end-point energies. This property generates the risk of significant systematic uncertainties from the unfolding procedures necessary to have cross-sections at fixed energies. One method to overcome this problem is using high-energy positron beams (Colgate and Gilbert, 1953). While the generation becomes slightly more complex, the beam quality is improved by the annihilation peak of the positrons, giving rise to a quasi-monoenergetic beam on top of the Bremsstrahlung background. This method was developed in the two laboratories in Livermore (Fultz et al., 1962) and Saclay (Beil et al., 1969). There has been a longstanding discussion about discrepant results between Livermore and Saclay, both using the same method to generate the beams. The discrepancy is illustrated in Figure 1 and discussed in detail by Varlamov et al. (2017) and in the recent review by Kawano et al. (2020).
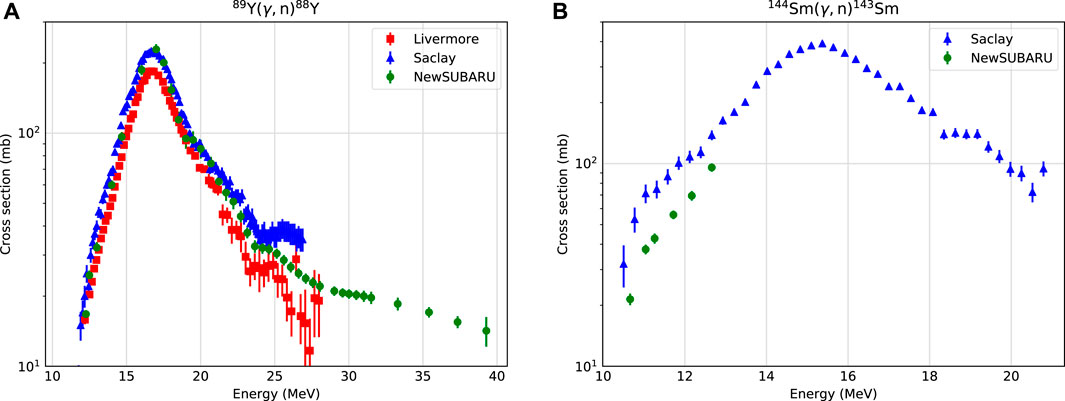
FIGURE 1. (A) Cross-section data for the (γ, n) reaction on 89Y from Livermore (Berman et al., 1967), Saclay (Lepretre et al., 1971) and NewSubaru Kawano et al. (2020). (B) Cross-section data for the (γ, n) reaction on 144Sm from Saclay (Carlos et al., 1974) and NewSubaru (Filipescu et al., 2014).
To generate monoenergetic γ-ray beams, the method of laser Compton backscattering (LCS) was introduced by Milburn (1963) and Arutyunian and Tumanian (1963). Here, a relativistic electron beam of a given energy collides with laser photons of a given frequency, and the backscattered photons will take a significant part of the electron kinetic energy with an energy-dependent angular distribution. Selecting specific angles through a collimator, the photon beams will have a well-defined energy and a narrow bandwidth, significantly reducing systematic measurement uncertainties, illustrated in Figure 1. This beam type will be the primary source of γ rays at ELI-NP.
Finally, another method under development is based on accelerating the primary electrons using laser wakefield acceleration from high-power lasers, a technology that will be the second main pillar of the ELI-NP facility via the 100 TW, 1 PW, and 10 PW beam lines (Tanaka et al., 2020). Such a measurement on p-process nuclei, namely the 92Mo(γ,n)91m,gMo isomeric ratios and the 92Mo (γ, 3n)89Mo reaction was recently reported by Wu et al. (2023).
Mechanisms of neutron detection are based on indirect methods since neutrons do not carry any charge. These mechanisms are based on neutron scattering or neutron absorption by detection media leading to the production of secondary particles, which can produce ionization around the interaction point. Typically neutron detectors to study (γ, n) reactions rely on the neutron capture process due to their almost 4π angular coverage and high efficiency. The neutron capture cross-section is usually higher for the thermal energy neutron range. Therefore, neutron counters are typically embedded in moderator material. The first neutron counters developed of this type represented boron neutron detectors surrounded by a water tank. More modern and nowadays systems commonly exploit 3He or BF3 gas-filled counters placed inside a paraffin or high-density polyethylene matrix. Different quenching gas, for example, CO2 or Ne, are often used to enhance the counting characteristics. However, due to the moderation process, precise information on the individual neutron energy is lost. This problem is solved by designing the system with flat neutron detection efficiency in a broad neutron energy range (Utsunomiya et al., 2017). Another method of neutron counting is based on (semi)activation techniques. The total number of emitted neutrons can be estimated by counting γ-rays emitted by the daughter nucleus. However, the method requires information on the absolute γ-ray transition intensities, thus, representing an additional source of systematic errors. It also limits the possible cases that can be measured to isotopes with levels with strong γ-ray emission either following β decay or population of isomeric states.
2.1.1 ELI-NP gamma beam
The main beam-line, still under construction, of interest at the ELI-NP facility for these measurements is the high-brilliance, low-bandwidth γ-ray beam produced by Compton backscattering of a laser off an electron beam. This system will have an electron storage ring coupled to an optical cavity. The energy of the circulating electrons will be steplessly varied in the range of 234–742 MeV, and photons from two different lasers can cover γ-ray energies in the range of 1–19.5 MeV. The beam is expected to be almost 100% polarized with a bandwidth less than 0.5%, with a peak spectral density of more than 5,000 s−1 eV−1 and a beam intensity at 10 MeV of 2.5 × 108 s−1. The characteristics of this type of measurement at ELI-NP put specific requirements on the beam properties compared to other types of γ-beam experiments. In these cases, the ELI-NP γ beam must operate up to the maximum energy above the neutron separation thresholds. The energy variability and bandwidth are the most critical properties of the ELI-NP beam for this type of measurements.
2.1.2 ELIGANT-TN
For the ELI-NP measurements, the primary neutron counting system planned is the ELI Gamma Above Neutron Threshold (ELIGANT) setups, in particular, the ELIGANT Thermal Neutron (ELIGANT-TN) high-efficiency neutron detector system in the flat efficiency configuration (Camera et al., 2016; Utsunomiya et al., 2017). The instrument consists of 28 tubes filled with 3He at a pressure of 12 bar arranged in a pattern of three rings, containing 4, 8, and 16 detectors, respectively, embedded in a polyethylene matrix and shielded from external neutrons using thin sheets of cadmium with an additional moderator outside. The efficiency of the neutron counter is around 38% over the complete, predicted neutron energy range. This feature is essential as it means the energy of the emitted neutrons will not bias the measured (γ, n) cross-section. However, although the detector works with neutrons moderated to thermal energies, the average neutron energy can provide additional information about the reaction and would be obtained from the ring-ratio method (Berman and Fultz, 1975). The instrument will use a fully digital data acquisition system based on CAEN v1725 digitizers. The preliminary performance of the system, in terms of efficiency and average neutron energy measurements with the ring ratio, has been reported by Söderström et al. (2021), using a plutonium-beryllium neutron source, and by Clisu et al. (2023) via in-beam characterization at a 9 MV Tandem accelerator.
2.2 Overview of existing data
This section will overview the existing photonuclear data of the p-process nuclei. We will limit the discussion to pure (γ, n) data and not include, except in exceptional cases, measurements of, for example, isomeric ratios and higher order channels like (γ, 2n). Table 1 summarises the most recent data sets for an accessible overview of the current state-of-the-art.
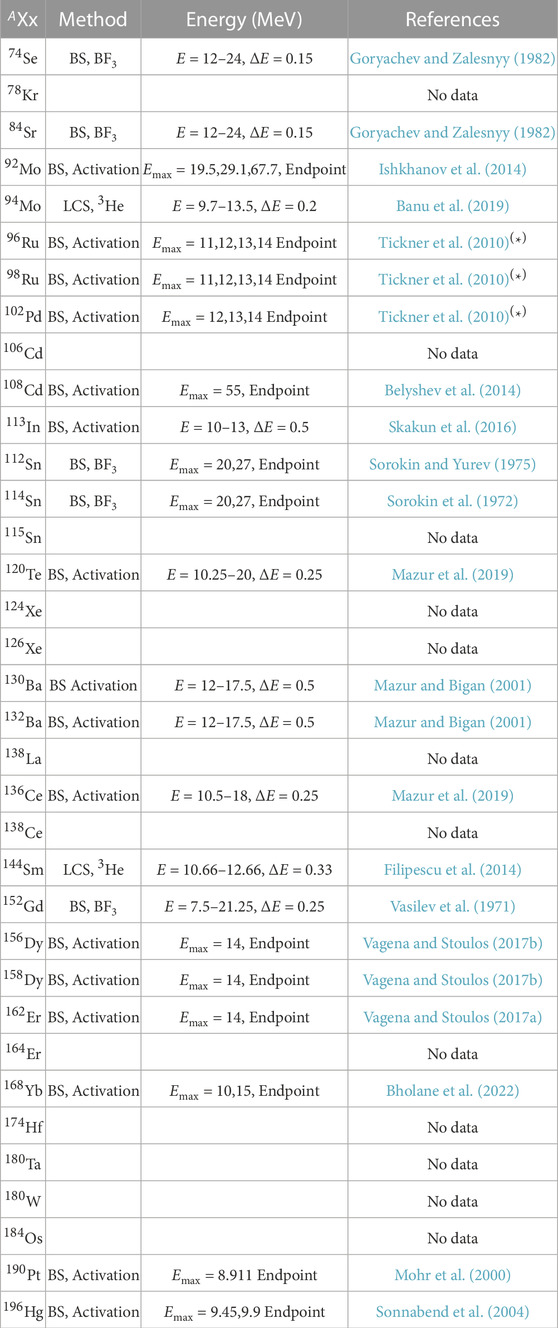
TABLE 1. Summary of the most recent measurements for each p-process isotope. For a discussion about additional work on the different nuclei see the text. Beam and detection methods are labelled as Bremsstrahlung (BS), laser Compton Backscattering (LCS) and either indirect measurements using activation or direct counting with BF3 or 3He counters. The energy range and energy step are given for each reference, except for integrated Bremsstrahlung measurements, where the end-points are given. (*) Skakun et al. (2013) have performed a newer measurement with more points and smaller ΔE. However the cross-sections are not reported in the citation. Thus, we list the values by Tickner et al. (2010) in this table.
The lightest p-process nuclei 74Se and 84Sr isotopes have been measured once using Bremsstrahlung from the Betatron at Saratov State University in Russia with a BF3 counter setup by Goryachev and Zalesnyy (1982). In the case of 92Mo, this is one of the most well-studied p-process nuclei, where cross-section, integrated cross-section, and cross-section ratio measurements have been performed. The first data set on the photoneutron cross-section curve and integrated cross-section have been measured by residual activity method using the Tohoku University 25 MeV betatron Bremsstrahlung gamma rays by Mutsuro et al. (1959). The second data set is by Ishkhanov et al. (1970) using Bremsstrahlung from the Betatron at Moscow State University, in the range of 12.2 MeV and 26.8 MeV, using BF3 detectors. The third data set for the cross-section of 92Mo and also 94Mo were obtained by the positron annihilation in-flight of monochromatic positions from the 60 MeV linear accelerator at Saclay in by Beil et al. (1974) and Carlos et al. (1974) using their gadolinium-loaded liquid scintillator tank setup in the range of 12.53–29.47 MeV. In a recent measurement, the cross sections for the (γ, n) reaction have again been measured using Bremsstrahlung photons from an electron accelerator and determined using the gamma-activation method (Ishkhanov et al., 2014) at the end-point energies of 19.5, 29.1, and 67.7 MeV at Moscow State University.
The 94Mo(γ,n) reaction has been investigated with quasi-monochromatic LCS photon beams both at TERAS in Japan (Utsunomiya et al., 2013), and HIγS facility of the Triangle Universities Nuclear Laboratory (TUNL) by Banu et al. (2019) in the range of 9.9–11.78 MeV and 9.7–13.5 MeV, respectively. The data obtained data all cases (Beil et al., 1974; Utsunomiya et al., 2013; Banu et al., 2019) are in good agreement below 10.8 MeV photon energies. However, above that, energy results of Banu et al. (2019) start to deviate from the Beil et al. (1974) and Utsunomiya et al. (2013) data when the contribution to the measured cross sections from neutrons emitted from excited states that γ decay to the ground state in 93Mo. Note that neither Utsunomiya et al. (2013) or Banu et al. (2019) had a flat-efficiency neutron detector but relied on the ring-ratio method (Utsunomiya et al., 2013) or Geant4 simulations of the possible branching channels (Banu et al., 2019) to infer the efficiency. Thus, the flat-efficiency ELIGANT-TN detector could provide an interesting complementary data set also here.
Cross sections have been measured for (γ,n) reactions of 96Ru, 98Ru, and 102Pd by Tickner et al. (2010) and Skakun et al. (2013). The first measurement used Bremsstrahlung radiation with end-point energies of 11–14 MeV using the activation technique in the Australian Radiation Protection and Nuclear Safety Agency in Melbourne, Australia. The second measurement was performed to determine the integral cross-section using Bremsstrahlung beams at the electron linear accelerator of Kharkiv Institute of Physics and Technology (KIPT) and the Microtron of Uzhgorod National University in Ukraine with different end-points in the range of 10.85–14 MeV (Skakun et al., 2013). The cross-section of 108Cd has been measured with the induced-activity method by using a beam of Bremsstrahlung radiation with end-point energy of 55 MeV at the racetrack microtron RM-55 by Belyshev et al. (2014) at the Lebedev Physical Institute in Moscow. The cross-section of the of 113In(γ,n)112mIn reaction has been measured in the Bremsstrahlung end-point energy range between 10 and 13 MeV by activation using the 197Au(γ,n)196Au reaction cross section as a reference standard by Skakun et al. (2016) at KIPT and the Uzhgorod National University.
For the 112Sn and 114Sn, the only direct experimental data available is from Bremsstrahlung experiments from Moscow State University by Sorokin and Yurev (1975) and Sorokin et al. (1972), respectively, using BF3 detectors. In the 114Sn case, the target had a relatively low isotopic purity with only 65.1%. Additionally, these data sets have been evaluated by Varlamov et al. (2010) extracting cross-section curves based on the theoretical modelling of cross-section ratios. However, model-independent direct measurements would be desirable.
The population of isomeric states in the isotopes 130Ba and 132Ba has been measured twice using Bremsstrahlung beams and the activation method, once at the Joint Institute for Nuclear Research (JINR) in Dubna (Belov et al., 1996b; Thiep et al., 2012) and once at Uzhgorod National University (Mazur and Bigan, 2001), with consistent results. However, no exclusive cross-section that populates ground-state to ground-state data can be found. One case highlighted in the work by Camera et al. (2016) is 138La, with a natural abundance of 0.089%. This isotope has been out of reach from experimental measurements with previous facilities and is systematically problematic in p-process calculations as it is consistently underproduced in all p-process scenarios. Here, the destruction cross-section, 138La (γ, n)137La is of fundamental importance as it determines the balance between the 139La (γ, n)138La production, whose ground state cross section is known (Utsunomiya et al., 2006b), and destruction. The activation method was used for 136Ce with Bremsstrahlung beams at Uzhgorod National University by Mazur et al. (2019) providing data in the energy range 10.5–18 MeV. For 138Ce, no absolute (γ, n) cross sections are available, only isomeric ratios (Belov et al., 1996a; Palvanov and Razhabov, 1999; Goryachev and Zalesnyy, 2001; Thiep et al., 2009; Mazur et al., 2016) from JINR in Russia, KIPT and Uzhgorod National University in Ukraine, and the SB-50 Betatron of the National University of Uzbekistan; and an estimation of the total cross-section from Dietrich and Berman (1988) used by Belov et al. (1996a).
One of the nuclei that have recent LCS measurements available is 144Sm, which was remeasured by Filipescu et al. (2014) using LCS and a 3He neutron detector. In this measurement, a systematic reduction of the cross-section with 20% was found compared to the previous data set by Carlos et al. (1974) from Saclay, as shown in Figure 1, based on positron annihilation in-flight with a gadolinium-loaded scintillator tank, and highlights the importance of also remeasuring existing data with new methods.
For the remaining heavy nuclei, the data is increasingly sparse. The 152Gd isotope has been measured once using Bremsstrahlung from the Betatron at Saratov State University in Russia with 12 proportional counters by Goryachev and Zalesnyy (1982). There is only one integrated data point each available for 156Dy, 158Dy (Vagena and Stoulos, 2017b), and 162Er Vagena and Stoulos (2017a), in the energy range 9.1–14 MeV obtained via Bremsstrahlung at the University Hospital in Larissa, Greece. A similar situation occurs for 168Yb, where two integrated Bremsstrahlung cross sections were measured at 10 MeV and 15 MeV end-point energies by Bholane et al. (2022) at Dr. Vikhe Patil Memorial Hospital, Ahmednagar, India, and in the energy range 9.1–14 MeV by (Vagena and Stoulos, 2018), using the activation technique. Also, in this case, systematic measurements would be desirable.
The isotope 180Ta, identified in the studies by Camera et al. (2016) as one of the flagship cases for studies with ELIGANT-TN at ELI-NP, is unique in this case due to the extreme rarity of the element and the existence of a long-lived isomeric state with a significantly longer lifetime than the ground state. Thus, in the 179−181Ta network, there are eight different production and destruction reactions, in addition to the thermalization equilibrium between 180mTa and 180gTa, see for example the discussion by Belic et al. (1999), Belic et al. (2002); Schlegel et al. (2016). Of these, the 181Ta (γ, n)180Ta (Utsunomiya et al., 2003) and the 180mTa (n, γ)181Ta (Wisshak et al., 2001) are measured, as well as the isomeric ratios in the 181Ta (γ, n)180Ta reaction (Goko et al., 2006). Measurements of 180mTa (γ, n)181Ta is challenging due to the natural abundance of the target being only 0.012% and, thus, very high-intensity γ-ray beams with well-defined energies to reduce the uncertainties related to subtraction of, for example, Bremsstrahlung spectra and high-efficiency neutron detector systems are critical. However, also here it is important to note that this ground-state cross-section will only provide a lower limit of the astrophysical rate due to the dominating contributions of thermally excited states (Mohr et al., 2007; Hayakawa et al., 2010a; Hayakawa et al., 2010b) and the main impact will be constraining theoretical models.
For 180W, only the isomeric ratios have been measured by Demekhina et al. (2002) at the synchrotron facility using the activation technique at the Yerevan Physics Institute in Armenia. For 190Pt, the stellar reaction rates have been simulated by a superposition of Bremsstrahlung measurements at different energies by Vogt et al. (2001) and the threshold reaction cross-section has been measured from a Bremsstrahlung spectrum at the Superconducting-DArmstadt-LINear-ACcelerator (S-DALINAC) using the activation techniqe with high-purity germanium detectors by Mohr et al. (2000). For 196Hg, isomeric ratios have been measured by Thiep et al. (2019) with Bremsstrahlung at JINR, and the threshold reaction cross-section has been measured by the same method as 190Pt at the S-DALINAC by Sonnabend et al. (2004).
3 Conclusion
We have presented an overview of the existing nuclear data on (γ, n) reactions for p-process nuclei in the context of the upcoming γ-ray beam at ELI-NP. Currently, the data is sparse, and several existing data sets suffer from inconsistencies or lack of independent verification. This implies that the planned ELI-NP measurement programme will be able to significantly contribute to the body of photonuclear data on the p-process nuclei. While not the focus of this article, in addition to (γ, n) reactions a dedicated scientific program for charged-particle reactions like (γ, p) and (γ, α) is also in preparation (Tesileanu et al., 2020) that will provide an even broader understanding of these rare isotopes.
Author contributions
All authors listed have made a substantial, direct, and intellectual contribution to the work and approved it for publication.
Funding
P-AS acknowledges the support from the Ministry of Research, Innovation and Digitization, CNCS—UEFISCDI, project number PN-III-P4-PCE-2021-0595, within PNCDI III. AK and DT acknowledge the support of the Romanian Ministry of Research and Innovation under research contract PN 23 21 01 06.
Acknowledgments
The authors would like to thank Prof. D. L. Balabanski at ELI-NP for proofreading of the manuscript.
Conflict of interest
The authors declare that the research was conducted in the absence of any commercial or financial relationships that could be construed as a potential conflict of interest.
Publisher’s note
All claims expressed in this article are solely those of the authors and do not necessarily represent those of their affiliated organizations, or those of the publisher, the editors and the reviewers. Any product that may be evaluated in this article, or claim that may be made by its manufacturer, is not guaranteed or endorsed by the publisher.
References
Amano, S., Horikawa, K., Ishihara, K., Miyamoto, S., Hayakawa, T., Shizuma, T., et al. (2009). Several-MeV γ-ray generation at NewSUBARU by laser Compton backscattering. Nucl. Instrum. Methods Phys. Res. A 602, 337–341. doi:10.1016/j.nima.2009.01.010
Arutyunian, F., and Tumanian, V. (1963). The Compton effect on relativistic electrons and the possibility of obtaining high energy beams. Phys. Lett. 4, 176–178. doi:10.1016/0031-9163(63)90351-2
Baldwin, G. C., and Klaiber, G. S. (1947). Photo-fission in heavy elements. Phys. Rev. 71, 3–10. doi:10.1103/physrev.71.3
Banu, A., Meekins, E. G., Silano, J. A., Karwowski, H. J., and Goriely, S. (2019). Photoneutron reaction cross section measurements on 94Mo and 90Zr relevant to the p-process nucleosynthesis. Phys. Rev. C 99, 025802. doi:10.1103/physrevc.99.025802
Beil, H., Bergere, R., Carlos, P., Lepretre, A., de Miniac, A., and Veyssiere, A. (1974). A study of the photoneutron contribution to the giant dipole resonance in doubly even Mo isotopes. Nucl. Phys. A 227, 427–449. doi:10.1016/0375-9474(74)90769-6
Beil, H., Bergere, R., and Veyssiere, A. (1969). Systeme de detection de photoneutrons utilisant un scintillateur a grandes dimensions permettant l’etude simultanee des reactions (γ, xn). Nucl. Instrum. Methods 67, 293–304. doi:10.1016/0029-554x(69)90462-5
Belic, D., Arlandini, C., Besserer, J., de Boer, J., Carroll, J. J., Enders, J., et al. (2002). Photo-induced depopulation of the 180Tam isomer via low-lying intermediate states: structure and astrophysical implications. Phys. Rev. C 65, 035801. doi:10.1103/physrevc.65.035801
Belic, D., Arlandini, C., Besserer, J., de Boer, J., Carroll, J. J., Enders, J., et al. (1999). Photoactivation of 180Tam and its implications for the nucleosynthesis of nature’s rarest naturally occurring isotope. Phys. Rev. Lett. 83, 5242–5245. doi:10.1103/physrevlett.83.5242
Belov, A. G., Gangrsky, Y. P., Tonchev, A. P., and Balabanov, N. P. (1996a). Excitation of isomeric 1h11/2 states in the reactions (γ, n). Phys. At. Nucl. 59, 553.
Belov, A. G., Gangrsky, Y. P., Tonchev, A. P., Balabanov, N. P., and Khristov, K. G. (1996b). Measurement of isomeric ratios in the reactions (γ, n) on barium isotopes in the giant-dipole-resonance region. Phys. At. Nucl. 59, 367.
Belyshev, S. S., Ishkhanov, B. S., Kuznetsov, A. A., Orlin, V. N., Stopani, K. A., Khankin, V. V., et al. (2014). Photodisintegration of cadmium isotopes. Phys. At. Nucl. 77, 809–816. doi:10.1134/s1063778814060039
Berman, B. L., Caldwell, J. T., Harvey, R. R., Kelly, M. A., Bramblett, R. L., and Fultz, S. C. (1967). Photoneutron cross sections for Zr90, Zr91, Zr92, Zr94, and Y89. Phys. Rev. 162, 1098.
Berman, B. L., and Fultz, S. C. (1975). Measurements of the giant dipole resonance with monoenergetic photons. Rev. Mod. Phys. 47, 713–761. doi:10.1103/revmodphys.47.713
Bholane, G., Ganesapandy, T., Phatangare, A., Attar, F., Dahiwale, S., Suryanarayana, S., et al. (2022). Activation cross section measurements and estimation of photon and neutron induced nuclear reactions for ytterbium isotopes with covariance analysis. Radiat. Phys. Chem. 195, 110066. doi:10.1016/j.radphyschem.2022.110066
Camera, F., Utsunomiya, H., Varlamov, V., Filipescu, D., Baran, V., Bracco, A., et al. (2016). Gamma above the neutron threshold experiments at ELI-NP. Rom. Rep. Phys. 68, S539.
Carlos, P., Beil, H., Bergére, R., Leprêtre, A., De Miniac, A., and Veyssière, A. (1974). The giant dipole resonance in the transition region of the samarium isotopes. Nucl. Phys. A 225, 171–188. doi:10.1016/0375-9474(74)90373-x
Clisu, C., Gheorghe, I., Filipescu, D., Renstrøm, T., Aciksoz, E., Boromiza, M., et al. (2023). Cross section measurements of low-energy charged particle induced reactions using moderated neutron counter arrays. EPJ Web Conf. 284, 01015. doi:10.1051/epjconf/202328401015
Colgate, S. A., and Gilbert, F. C. (1953). Electron-positron annihilation in flight. Phys. Rev. 89, 790–792. doi:10.1103/physrev.89.790
Demekhina, N. A., Danagulyan, A. S., and Karapetyan, G. S. (2002). Analysis of isomeric ratios in (γ, n) and (γ, p) reactions around giant-resonance energies n) and (γ, p) reactions around giant-resonance energies. Phys. At. Nucl. 65, 365–370. doi:10.1134/1.1451955
Dietrich, S. S., and Berman, B. L. (1988). Atlas of photoneutron cross sections obtained with monoenergetic photons. At. Data Nucl. Data Tables 38, 199–338. doi:10.1016/0092-640x(88)90033-2
Filipescu, D., Anzalone, A., Balabanski, D. L., Belyshev, S. S., Camera, F., Cognata, M. L., et al. (2015). Perspectives for photonuclear research at the extreme light infrastructure - nuclear physics (ELI-NP) facility. Eur. Phys. J. A51, 185. doi:10.1140/epja/i2015-15185-9
Filipescu, D. M., Gheorghe, I., Utsunomiya, H., Goriely, S., Renstrøm, T., Nyhus, H.-T., et al. (2014). Photoneutron cross sections for samarium isotopes: toward a unified understanding of (γ, n) and (n, γ) reactions in the rare earth region. Phys. Rev. C 90, 064616. doi:10.1103/physrevc.90.064616
Fultz, S. C., Bramblett, R. L., Caldwell, J. T., and Kerr, N. A. (1962). Photoneutron cross-section measurements on gold using nearly monochromatic photons. Phys. Rev. 127, 1273–1279. doi:10.1103/physrev.127.1273
Gales, S., Balabanski, D. L., Negoita, F., Tesileanu, O., Ur, C. A., Ursescu, D., et al. (2016). New frontiers in nuclear physics with highpower lasers and brilliant monochromatic gamma beams. Phys. Scr. 91, 093004. doi:10.1088/0031-8949/91/9/093004
Gales, S., Tanaka, K. A., Balabanski, D. L., Negoita, F., Stutman, D., Tesileanu, O., et al. (2018). The extreme light infrastructure–nuclear physics (ELI-NP) facility: new horizons in physics with 10 PW ultra-intense lasers and 20 MeV brilliant gamma beams. Rep. Prog. Phys. 81, 094301. doi:10.1088/1361-6633/aacfe8
Goko, S., Utsunomiya, H., Goriely, S., Makinaga, A., Kaihori, T., Hohara, S., et al. (2006). Partial photoneutron cross sections for the isomeric state 180Tam. Phys. Rev. Lett. 96, 192501. doi:10.1103/physrevlett.96.192501
Goryachev, A. M., and Zalesnyy, G. N. (2001). Isomeric ratios in crossing (nγ) and (γn) reactions. Bull. Rus. Acad. Sci. Phys. 65, 121.
Goryachev, A. M., and Zalesnyy, G. N. (1982). The studying of the photoneutron reactions cross sections in the region of the giant dipole resonance in zinc, germanium, selenium, and strontium isotopes. Voprosy Teoreticheskoy i Yadernoy Fiziki 121 Internal scientific collection, published by Saratov University.
Hayakawa, T., Kajino, T., Chiba, S., and Mathews, G. J. (2010a). New estimate for the time-dependent thermal nucleosynthesis of 180Tam. Phys. Rev. C 81, 052801(R). doi:10.1103/physrevc.81.052801
Hayakawa, T., Mohr, P., Kajino, T., Chiba, S., and Mathews, G. J. (2010b). Reanalysis of the (J = 5) state at 592 keV in 180Ta and its role in the ν-process nucleosynthesis of 180Ta in supernovae. Phys. Rev. C 82, 058801. doi:10.1103/physrevc.82.058801
Ishkhanov, B. S., Kapitonov, I. M., Kuznetsov, A. A., Orlin, V. N., and Yoon, H. D. (2014). Photonuclear reactions on molybdenum isotopes. Phys. At. Nucl. 77, 1362–1370. doi:10.1134/s106377881410007x
Ishkhanov, B. S., Kapitonov, I. M., Lazutin, E. V., Sopov, V. S., Piskarev, I. M., and Shevchenko, G. (1970). Intermediate structure of photoneutron reactions cross sections for Mo isotopes. Sov. J. Nucl. Phys. 11, 394.
Kawano, T., Cho, Y., Dimitriou, P., Filipescu, D., Iwamoto, N., Plujko, V., et al. (2020). IAEA photonuclear data library 2019. Nucl. Data Sheets 163, 109–162. doi:10.1016/j.nds.2019.12.002
Lepretre, A., Beil, H., Bergere, R., Carlos, P., Veyssiere, A., and Sugawara, M. (1971). The giant dipole states in the A = 90 mass region. Nucl. Phys. A 175, 609–628. doi:10.1016/0375-9474(71)90454-4
Litvinenko, V. N., Burnham, B., Emamian, M., Hower, N., Madey, J. M. J., Morcombe, P., et al. (1997). Gamma-ray production in a storage ring free-electron laser. Phys. Rev. Lett. 78, 4569–4572. doi:10.1103/physrevlett.78.4569
Mazur, V. M., and Bigan, Z. M. (2001). Cross section of excitation of isomeric states for 129mBa, 131mBa, 135mBa isotopes in (γ, n) reactions. Ukr. J. Phys. 46, 529.
Mazur, V. M., Bigan, Z. M., Derechkey, P. S., and Symochko, D. M. (2016). Excitation of 11/2− isomeric state in 137Ce in (γ, n) reaction within 10-22MeV energy range. Int. J. Mod. Phys. E 25, 1650070. doi:10.1142/s0218301316500701
Mazur, V. M., Bigan, Z. M., Derechkey, P. S., Zheltonozhsky, V. A., and Savrasov, A. M. (2019). Study of the cross sections for (γ,n)-reactions on р-nuclei 120Te, 136Cе in the giant E1 resonance energy region n)-reactions on p-nuclei 120Te, 136Ce in the giant E1 resonance energy region. Nucl. Phys. At. Energy 20, 228–234. doi:10.15407/jnpae2019.03.228
Milburn, R. H. (1963). Electron scattering by an intense polarized photon field. Phys. Rev. Lett. 10, 75–77. doi:10.1103/physrevlett.10.75
Mohr, P., Käppler, F., and Gallino, R. (2007). Survival of nature’s rarest isotope 180Ta under stellar conditions. Phys. Rev. C 75, 012802. (R). doi:10.1103/physrevc.75.012802
Mohr, P. (2004). Photon-induced reactions in stars and in the laboratory: A critical comparison. AIP Conf. Proc. 704, 532.
Mohr, P., Vogt, K., Babilon, M., Enders, J., Hartmann, T., Hutter, C., et al. (2000). Experimental simulation of a stellar photon bath by bremsstrahlung: the astrophysical γ-process. Phys. Lett. B 488, 127–130. doi:10.1016/s0370-2693(00)00862-5
Mutsuro, N., Ohnuki, Y., Sato, K., and Kimura, M. (1959). Photoneutron cross sections forAg107, Mo92 and Zr90. J. Phys. Soc. Jpn. 14, 1649–1653. doi:10.1143/jpsj.14.1649
Palvanov, S. R., and Razhabov, O. (1999). Isomer yield ratios of photonuclear reactions at eγmax 25 and 30 MeV. At. Energy 87, 533–536. doi:10.1007/bf02673215
Rauscher, T. (2014). Challenges in nucleosynthesis of trans-iron elements. AIP Adv. 4, 041012. doi:10.1063/1.4868239
Rauscher, T. (2013). General properties of astrophysical reaction rates in explosive nucleosynthesis. J. Phys. Conf. Ser. 420, 012138. doi:10.1088/1742-6596/420/1/012138
Rauscher, T. (2012). Sensitivity of astrophysical reaction rates to nuclear uncertainties. Astrophys. J. Suppl. Ser. 201, 26. doi:10.1088/0067-0049/201/2/26
Schlegel, Ch., von Neumann-Cosel, P., de Boer, J., Gerl, J., Kaspar, M., Kozhoukharov, I., et al. (2016). Depopulation of the Jπ = 9− isomer in 180Ta to the Jπ = 1+ ground state by Coulomb excitation. Eur. Phys. J. A 10, 135–138. doi:10.1007/s100500170124
Skakun, Y., Semisalov, I., Kasilov, V., Popov, V., Kochetov, S., Avramenko, N., et al. (2013). “Possibilities to investigate astrophysical photonuclear reactions in Ukraine,” in International conference on current problems in nuclear physics and atomic energy (NPAE-Kyiv2012) (I. M. Vyshnevskyi), 570.
Skakun, Y., Semisalov, I., Kasilov, V., Popov, V., Kochetov, S., Maslyuk, V., et al. (2016). Reaction rates of the 113In(γ, n)112mIn and 115In(γ, n)114mIn. J. Phys. Conf. Ser. 665, 012040. doi:10.1088/1742-6596/665/1/012040
Söderström, P.-A., Matei, C., Capponi, L., Açıksöz, E., Balabanski, D. L., and Mitu, I. O. (2021). Characterization of a plutonium-beryllium neutron source. Appl. Radiat. Isot. 167, 109441. doi:10.1016/j.apradiso.2020.109441
Sonnabend, K., Vogt, K., Galaviz, D., Müller, S., and Zilges, A. (2004). Systematic study of (γ, n) reaction rates for Z ≤ 78 isotopes. Phys. Rev. C 70, 035802. doi:10.1103/physrevc.70.035802
Sorokin, Y., Krushchev, V., and Yurev, B. (1972). Photoneutron cross sections for tin isotopes with A = 114, 116, 117, 119, 122 and 124. Bull. Russ. Acad. Sci. Phys. 36, 170.
Sorokin, Y., and Yurev, B. (1975). Photoneutron cross sections for 112Sn, 118Sn, and 120Sn. Sov. J. Nucl. Phys. 20, 123.
Tanaka, K. A., Spohr, K. M., Balabanski, D. L., Balascuta, S., Capponi, L., Cernaianu, M. O., et al. (2020). Current status and highlights of the ELI-NP research program. Matter Radiat. Extrem. 5, 024402. doi:10.1063/1.5093535
Tesileanu, O., Gai, M., Anzalone, A., Balan, C., Bihalowicz, J. S., Cwiok, M., et al. (2020). Charged particle detection at ELI-NP. Rom. Rep. Phys. 68, S699.
Thiep, T. D., An, T. T., Cuong, P. V., Hue, B. M., Belov, A., and Mitrofanov, S. (2019). Isomeric yield ratios of 195m,gHg and 197m,gHg in the 196Hg(γ, n) and 198Hg(γ, n) reactions induced by bremsstrahlung energy within giant dipole resonance region. Nucl. Instrum. Methods Phys. Res. B 457, 4–9. doi:10.1016/j.nimb.2019.07.022
Thiep, T. D., An, T. T., Cuong, P. V., Khai, N. T., and Vinh, N. T. (2012). The isomeric ratios in photonuclear reactions of natural barium induced by bremsstrahlungs with endpoint energies in the giant dipole resonance region. J. Radioanal. Nucl. Chem. 292, 89–96. doi:10.1007/s10967-011-1371-9
Thiep, T. D., An, T. T., Khai, N. T., Vinh, N. T., Cuong, P. V., Belov, A. G., et al. (2009). The isomeric ratios in some photonuclear reactions (γ, n), (γ, p), (γ, 2n), and (γ, np) induced by bremsstrahlungs with end-point energies in the Giant Dipole Resonance region n), (γ, p), (γ, 2n), and (γ, np) induced by bremsstrahlungs with end-point energies in the Giant Dipole Resonance region. Phys. Part. Nucl. Lett. 6, 126–133. doi:10.1134/s1547477109020058
Tickner, J., Bencardino, R., and Roach, G. (2010). Measurement of activation yields for platinum group elements using Bremsstrahlung radiation with end-point energies in the range 11-14MeV. Nucl. Instrum. Methods Phys. Res. B 268, 99–105. doi:10.1016/j.nimb.2009.10.179
Toyokawa, H., Goko, S., Hohara, S., Kaihori, T., Kaneko, F., Kuroda, R., et al. (2009). Recent progress in generation and application of AIST laser-Compton gamma-ray beam. Nucl. Instrum. Methods Phys. Res. A 608, S41–S43. doi:10.1016/j.nima.2009.05.062
Utsunomiya, H., Akimune, H., Goko, S., Ohta, M., Ueda, H., Yamagata, T., et al. (2003). Cross section measurements of the 181Ta(γ,n)180Ta reaction near neutron threshold and the p-process nucleosynthesis. Phys. Rev. C 67, 015807. doi:10.1103/physrevc.67.015807
Utsunomiya, H., Gheorghe, I., Filipescu, D. M., Glodariu, T., Belyshev, S., Stopani, K., et al. (2017). Direct neutron-multiplicity sorting with a flat-efficiency detector. Nucl. Instrum. Methods Phys. Res. A 871, 135–141. doi:10.1016/j.nima.2017.08.001
Utsunomiya, H., Goriely, S., Kondo, T., Iwamoto, C., Akimune, H., Yamagata, T., et al. (2013). Photoneutron cross sections for Mo isotopes: A step toward a unified understanding of (γ, n) and (n, γ) reactions. Phys. Rev. C 88, 015805. doi:10.1103/physrevc.88.015805
Utsunomiya, H., Makinaga, A., Goko, S., Kaihori, T., Akimune, H., Yamagata, T., et al. (2006b). Photoneutron cross section measurements on the N = 82 nuclei 139La and 141 Pr: implications for p-process nucleosynthesis. Phys. Rev. C 74, 025806. doi:10.1103/physrevc.74.025806
Utsunomiya, H., Mohr, P., Zilges, A., and Rayet, M. (2006a). Direct determination of photodisintegration cross sections and the p-process. Nucl. Phys. A 777, 459–478. doi:10.1016/j.nuclphysa.2004.06.025
Vagena, E., and Stoulos, S. (2017a). Average cross section measurement for 162Er (γ, n) reaction compared with theoretical calculations using TALYS. Nucl. Phys. A 957, 259–273. doi:10.1016/j.nuclphysa.2016.09.007
Vagena, E., and Stoulos, S. (2017b). Photodisintegration average cross sections of dysprosium p-nuclei near (γ,n) reaction threshold n) reaction threshold. Eur. Phys. J. A 53, 85. doi:10.1140/epja/i2017-12278-5
Vagena, E., and Stoulos, S. (2018). Ytterbium (γ, n) average cross-sections data near to photodisintegration reaction threshold. Eur. Phys. J. A 54, 153. doi:10.1140/epja/i2018-12583-5
Varlamov, V., Ishkhanov, B., and Orlin, V. (2017). Experimental and evaluated photoneutron cross sections for 197Au. Phys. Rev. C 96, 044606. doi:10.1103/physrevc.96.044606
Varlamov, V. V., Ishkhanov, B. S., Orlin, V. N., and Chetvertkova, V. A. (2010). Evaluated cross sections of the σ(γ, nX) and σ(γ, 2nX) reactions on 112,114,116,117,118,119,120,122,124Sn isotopes. Bull. Russ. Acad. Sci. Phys. 74, 833–841. doi:10.3103/s1062873810060225
Vasilev, O. V., Semenov, V. A., and Semenko, S. F. (1971). Giant dipole resonance in transition region nuclei with N approximately 90. Sov. J. Nucl. Phys. 13, 259.
Vogt, K., Mohr, P., Babilon, M., Enders, J., Hartmann, T., Hutter, C., et al. (2001). Measurement of the (γ, n) reaction rates of the nuclides 190Pt, 192Pt, and 198Pt in the astrophysical γ process. Phys. Rev. C 63, 055802.
Wisshak, K., Voss, F., Arlandini, C., Becvář, F., Straniero, O., Gallino, R., et al. (2001). Neutron capture on 180Tam: clue for an s-process origin of nature’s rarest isotope. Phys. Rev. Lett. 87, 251102. doi:10.1103/physrevlett.87.251102
Wu, D., Lan, H. Y., Zhang, J. Y., Liu, J. X., Lu, H. G., Lv, J. F., et al. (2023). New measurements of 92Mo(γ, n) and (γ, 3n) reactions using laser-driven bremsstrahlung γ-ray. Front. Phys. 11, 1178257. doi:10.3389/fphy.2023.1178257
Keywords: p-process, nucleosynthesis, photonuclear reaction, neutron detection, nuclear cross section
Citation: Söderström P-A, Kuşoğlu A and Testov D (2023) Prospect for measurements of (γ, n) reaction cross-sections of p-nuclei at ELI-NP. Front. Astron. Space Sci. 10:1248834. doi: 10.3389/fspas.2023.1248834
Received: 27 June 2023; Accepted: 16 August 2023;
Published: 31 August 2023.
Edited by:
Denise Piatti, University of Padua, ItalyCopyright © 2023 Söderström, Kuşoğlu and Testov. This is an open-access article distributed under the terms of the Creative Commons Attribution License (CC BY). The use, distribution or reproduction in other forums is permitted, provided the original author(s) and the copyright owner(s) are credited and that the original publication in this journal is cited, in accordance with accepted academic practice. No use, distribution or reproduction is permitted which does not comply with these terms.
*Correspondence: P.-A. Söderström, par.anders@eli-np.ro