- South African Astronomical Observatory, Cape Town, South Africa
High-precision photometry from TESS has revealed over 500 stars, located between the δ Scuti and β Cephei instability strips, which pulsate with high frequencies. Models do not predict high pulsation frequencies in these stars. These anomalous variables may be identified with the historical “Maia” variables. From the projected rotational velocities, it is shown that the rotation rates of Maia variables are no different from main sequence or SPB stars in the same effective temperature range. Some Maia stars pulsate at frequencies typical of roAp stars. It is shown that Maia stars should be considered an extension of δ Scuti variables to effective temperatures as high as 18,000 K, rather than as a separate class. The TESS data show a continuous sequence of low-frequency pulsating stars linking the γ Doradus and SPB variables, which is not predicted by the models. There are, in fact, no well-defined instability strips at all among upper main sequence stars, which means that arbitrary choices of effective temperature and frequency ranges need to be made in order to assign a particular variability class. It seems that a mixture of driving mechanisms is present in which convection may play a very important role.
1 Introduction
Pulsating stars with high frequencies (i.e., ν ≳ 5 d−1) lying between the δ Scuti and β Cephei instability strips have historically been called Maia variables. According to the models, stars in this region of the H–R diagram should not pulsate, though these anomalous pulsating stars have been suspected for many decades. Struve (1955) reported short-period variations in the star Maia, a member of the Pleiades cluster, but later disclaimed the variability (Struve et al., 1957). It is now known from K2 space photometry that Maia itself is a rotational variable with a 10-day period (White et al., 2017) and no sign of high frequencies. However, possible rapid far-ultraviolet flux variations have been reported in this star (Monier, 2021).
McNamara (1985), Lehmann et al. (1995), Percy and Wilson (2000) and Kallinger et al. (2004) found evidence for the Maia group using ground-based photometry. From CoRoT space photometry, Degroote et al. (2009) discovered several low-amplitude late B-type pulsators with a very broad range of frequencies and low amplitudes characteristic of Maia variables. Mowlavi et al. (2013) found that many rapidly rotating mid-B stars in the open cluster NGC 3766 pulsate with frequencies as high as 10 d−1. Mowlavi et al. (2016) also found that the majority of these stars obey a period–luminosity relation. They suggested the name “FaRPB” for these variables, but their properties are similar to what have been historically known as Maia variables.
Several late-B stars qualifying as Maia variables have been detected in the Kepler field (Balona et al., 2015; 2016). Using TESS data, Balona and Ozuyar (2020) identified 131 Maia candidates. More recently (Gaia Collaboration et al., 2022; Gaia Collaboration et al., 2023) found a population of Maia stars using Gaia photometry. The A0Vnne star HD 42477, which is hotter than the blue edge of the δ Sct variables, shows a single p-mode pulsation coupled to lower frequency g modes. Kurtz et al. (2023) argue that the anomalous pulsation in this star cannot be explained by temperature uncertainties, a binary companion, or contamination by background pulsators.
From TESS observations, Balona and Ozuyar (2020) found that stars pulsating at frequencies higher than about 5 d−1 are found all along the main sequence, including the region between the δ Sct and β Cep stars. In the H–R diagram, the distribution of β Cep variables merges smoothly with the distribution of the Maia stars which, in turn, merges smoothly with the δ Sct stars. The same occurs for the low-frequency pulsators (ν ≲ 5 d−1). SPB stars are found which are cooler than the predicted red edge (Sharma et al., 2022). The sequence of low-frequency pulsators continues into the A-star region where they merge with the γ Dor variables. These anomalous stars have been called “hot γ Dor variables” (Balona et al., 2016).
In an attempt to understand these anomalous pulsating stars, it is often assumed that their location outside the predicted instability region is a result of rapid rotation. When observed equator-on, rapid rotation lowers the apparent effective temperature due to equatorial gravity darkening, shifting the star to cooler temperatures in the H–R diagram. Some hot Maia variables could, for example, be understood as rapidly-rotating β Cep stars. The presence of SPB stars cooler than the predicted red edge could likewise be explained in this way.
Gravito-inertial modes in moderate to fast rotators may have frequencies higher than normal. Some Maia variables could be understood as rapidly-rotating SPB stars, for example. In fact, Salmon et al. (2014) concluded that their models could reproduce the observations of Mowlavi et al. (2013, 2016). Saio et al. (2017) examined pulsation models of rapidly-rotating main sequence B stars and calculated the properties of prograde sectoral g and retrograde r modes excited by the κ mechanism at the Fe opacity peak. They found that the period-luminosity relation described by Mowlavi et al. (2016) can be explained by prograde sectoral g modes in rapidly rotating stars.
Daszyńska-Daszkiewicz et al. (2017) considered three hypothesis for the Maia variables: rapidly rotating stars with underestimated masses, rapidly rotating stars with non-standard opacities and slowly rotating stars with non-standard opacities. While there are indications that one or more of these hypotheses might be able to explain the observations, no definite conclusions can be made at this stage.
If rapid rotation is indeed an important characteristic of Maia variables, the projected rotational velocities for these stars should be significantly higher compared to non-pulsating main sequence stars in the same effective temperature range. Such a test was performed by Balona and Ozuyar (2020) using 41 Maia stars, but no significant difference in rotation rate between Maia stars and main sequence stars could be found. However, it could be argued that the sample of Maia stars is too small for a definitive conclusion.
In this paper, we use TESS data from Sectors 1–65 to identify many new Maia candidates. Over 500 Maia stars as well as many other pulsating A and B stars have been identified. Full details of how the search was conducted, how estimates of effective temperature were obtained and the sources of projected rotational velocities are described in Balona (2022a).
The large number of variable stars allows an examination of the relationships between the β Cep, SPB, δ Sct and γ Dor stars each of which are characterized by different pulsation mechanisms. It is show that the concept of distinct instability strips is not valid. These groups are not isolated, but blend into each other in a way which cannot be explained by uncertainties in effective temperatures or rapid rotation. The Maia variables are best described as an extension of the δ Sct stars into the B star region. These results are strongly at variance with current concepts.
2 New Maia variables
The TESS mission has been observing the whole sky and obtaining light curves for thousands of stars with 2-min cadence. This wide-band photometry has been corrected for long-term drifts using pre-search data conditioning (PDC, Jenkins et al., 2010). The author has been engaged in a project to classify the variability of stars hotter than about 6,000 K. Results of the classification of over 120,000 stars, together with their effective temperatures, Teff, luminosities, log L/L⊙, and projected rotational velocities, v sin i, are reported in Balona (2022a). The values of log L/L⊙, are from Gaia DR3 parallaxes (Gaia Collaboration et al., 2016; Gaia Collaboration et al., 2018). The classification was extended to TESS sector 65.
Many stars were detected which normally would be classified as δ Sct variables, but with effective temperatures in the range 10,000–18,000 K. These were classified as Maia variables if significant frequency peaks higher than νmin = 5 d−1 were detected. Frequency peaks are deemed to be significant if the signal-to-noise ratio in the periodogram amplitude, S/N > 4.7. The choice of νmin is arbitrary, but guided by the fact that in this temperature range only the low-frequency SPB variables, which have frequencies not much higher than about 3 d−1, are expected. Models do not predict δ Sct pulsations for main sequence stars hotter than about 9,000 K. The extension to 10,000 K is purely to minimize inclusion of δ Sct stars with erroneous values of effective temperature. For the same reason, the high-temperature limit for Maia variables was chosen to minimize inclusion of β Cep stars.
The full list of 519 Maia stars (and other variables) is to be found in Balona (2022a). In the present work, only stars within the main-sequence band are considered. This band is defined by −0.5 < Δ log L/L⊙ < 1.5, where Δ log L/L⊙ is the luminosity above the zero-age main sequence. Out of the 519 Maia variables observed by TESS, 446 stars are located within this luminosity band. If the value of νmin chosen to separate the SPB from the Maia class is raised to 10 d−1, this number is reduced from 446 to 373 stars. Clearly, the adopted value of νmin is not too important since most Maia variables have frequencies higher than 10 d−1.
It is difficult to distinguish between β Cep and SPB stars. Some bright, well studied β Cep stars have frequencies lower than 4 d−1, which is within the range predicted for SPB variables. In order to properly define the two variability classes, the frequency limit for SPB stars hotter than 18,000 K was lowered from νmin = 5 d−1 to νmin = 3 d−1. This means that the SPB classification depends not only on the range of observed frequencies, but also on whether the effective temperature is higher or lower than 18,000 K (Balona and Ozuyar, 2020; Balona, 2022a). Sharma et al. (2022) encountered the same difficulty, but used νmin = 2.4 d−1.
There are quite a number of Maia stars where a few low-amplitude peaks with high frequencies are present, in addition to peaks with lower frequencies. High frequencies can arise as a result of harmonics or combinations. In this investigation, harmonics and combination frequencies of the form ν = n1ν1 + n2ν2 were constructed from all frequency pairs ν1, ν2, where n1, n2 are positive or negative integers between −7 and +7. If the resulting frequency ν matches an observed frequency peak within the expected frequency uncertainty, it is not considered an independent frequency.
There are 59 Maia stars in which significant independent frequency peaks extending higher than 60 d−1 are present (Table 1). This number corresponds to about 11 percent of the Maia variables. As described in Balona (2022a), the notation MAIAU assigned to these stars does not indicate a separate variability class, but is just a convenient way of identifying Maia stars with high frequencies. These stars would probably be mistaken for roAp stars with δ Sct frequencies, except that they are significantly hotter and chemically normal. The roAp stars themselves can no longer be considered a separate class (Balona, 2022b). In the classification system described by Balona (2022a), δ Sct stars with independent frequencies higher than 60 d−1 are classified as DSCTU. There are 1344 DSCTU stars out of 11,721 δ Sct stars (also 11 percent of the population).
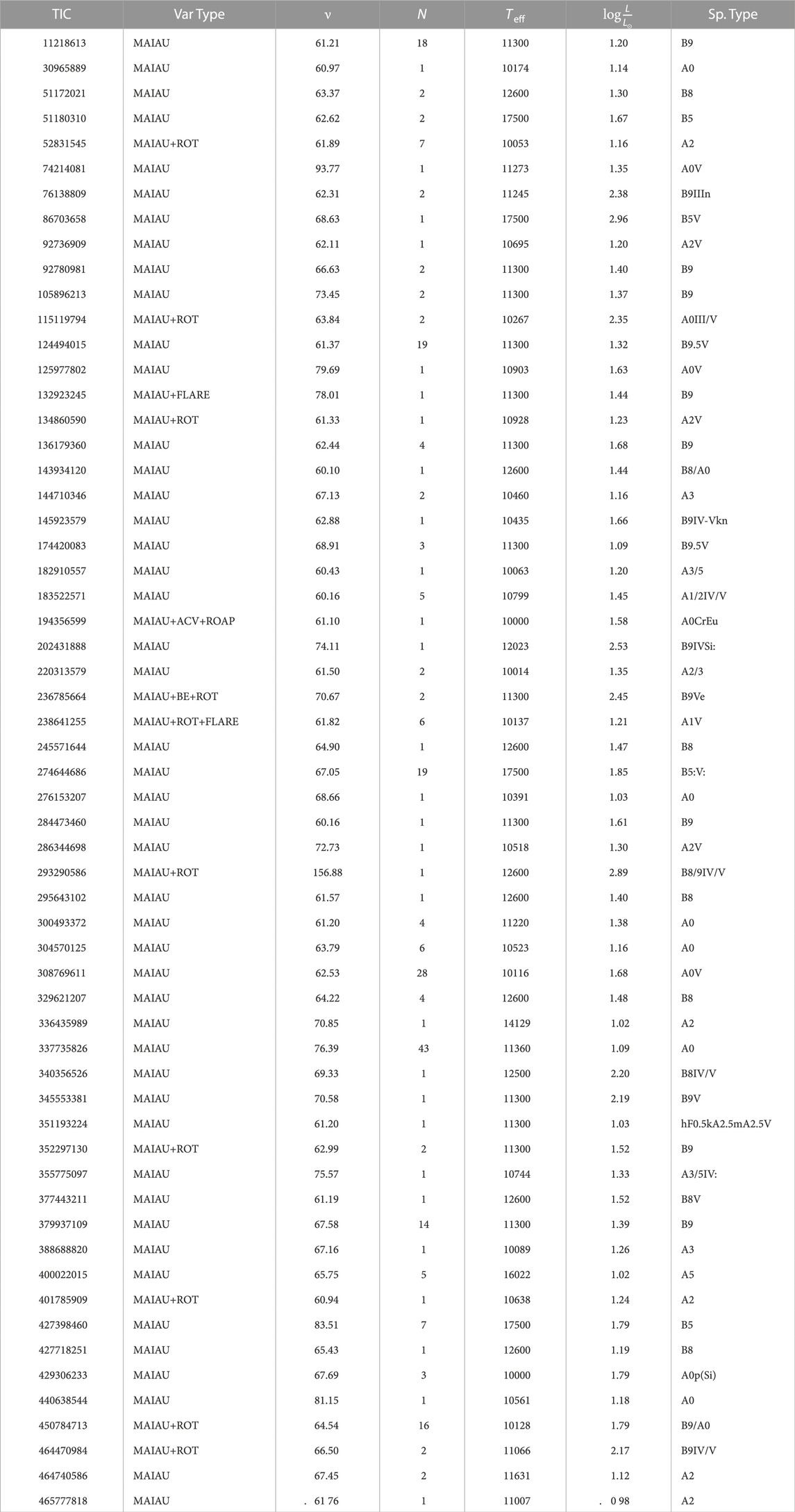
TABLE 1. List of Maia variables with high frequencies. These are classified as MAIAU if νmax >60 d−1. The column labeled ν is the frequency (in d−1) of the most prominent peak above 60 d−1. The number of independent frequency peaks greater than 60 d−1, N, is shown. The effective temperature, Teff (in K), the luminosity,
3 Rotation
As already mentioned, rotation affects the apparent location of the star in the H–R diagram due to equatorial gravity darkening and introduces new gravito-inertial modes with moderately high frequencies (Salmon et al., 2014). It is often assumed that anomalous high frequencies in late B stars are a result of rapid rotation. While this idea is reasonable and provides a way of avoiding a confrontation with theoretical predictions, it needs to be tested by observations. All that needs to be done is to compare the projected rotational velocities, v sin i, for Maia stars with those of non-pulsating main sequence stars or SPB stars in the same effective temperature range.
The distribution of v sin i for 145 Maia stars and for 6,538 main sequence stars in the temperature range 10,000 < Teff < 18,000 is shown in Figure 1. Also shown in the same figure is a comparison of v sin i for Maia stars with 323 SPB stars in the same temperature range. The conclusion is clear: Maia stars have rotation rates similar to those of normal main sequence stars or to the SPB variables. The high frequencies in Maia stars have nothing to do with rapid rotation.
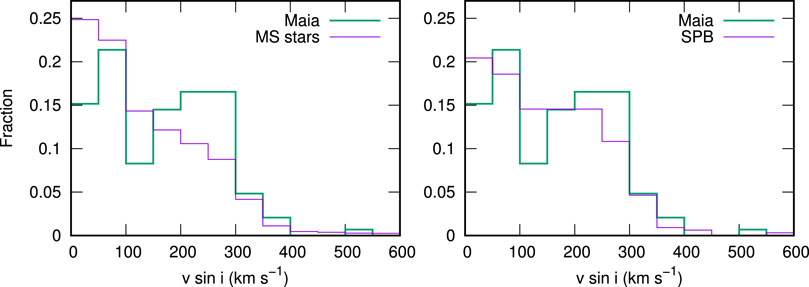
FIGURE 1. Top panel: the distribution of projected rotational velocity, v sin i, for Maia variables (heavy green histogram) and for main sequence stars in the same temperature range. The bottom panel shows the distributions for Maia and SPB stars in the same temperature range.
Another problem is the presence of low-frequency pulsations in stars cooler than predicted for SPB variables (i.e., Teff ≲ 11,000 K). Sharma et al. (2022) suggested that gravity darkening due to rapid rotation might explain the existence of these stars. To test this idea, we examined the v sin i distribution of 60 low-frequency pulsators with 9,000 < Teff < 11,000 K and compared it to the v sin i distribution of 1,217 main sequence stars in the same effective temperature range. No obvious difference could be found between the two distributions, but the sample is rather small. For SPB stars, the mean is ⟨v sin i⟩ = 159 ± 12 km s−1 and for main sequence stars ⟨v sin i⟩ = 118 ± 2 km s−1. In any case the rotation rate of these cool SPB variables cannot be called rapid. It can be concluded that rapid rotation is not the correct explanation for these anomalous cool SPB stars.
4 Distribution of Maia stars
Early results from TESS photometry (Balona and Ozuyar, 2020) seem to show a concentration of hot B stars in the H–R diagram, which can be identified as β Cep variables, and a larger concentration of cooler stars identified as δ Sct variables. The models predict that pulsating stars should be confined to well-defined instability strips, but the observations show a different picture with many pulsating stars in locations where they should not exist. While uncertainties in effective temperature and luminosity as well as rapid rotation would obviously contribute to such a spread, the spread appears to be larger than can be explained in this way. To illustrate this point, Figure 2 shows the number density of δ Sct, Maia and β Cep as well as γ Dor and SPB variables as a function of effective temperature. This figure was constructed by counting the number of main sequence stars of a particular variability class within a small range of effective temperature (0.02 dex in log Teff).
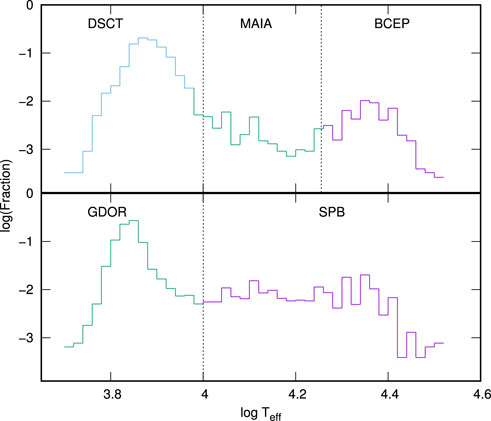
FIGURE 2. The distribution of δ Sct, Maia and β Cep variables (top panel) and the γ Dor and SPB stars (bottom panel).
The figure illustrates the problem: there are no distinct instability regions among stars in the upper main sequence. The typical uncertainty in Teff of early-A and late-B stars is less than 1,000 K even if the temperature estimate is based on spectral type alone. This translates to an error of about 0.05 dex in log Teff, which is only about twice the bin size used in Figure 2. Clearly, errors in effective temperature alone cannot explain the spread.
The figure also appears to suggest that Maia stars are not a separate class, but simply an extension of the δ Sct variables to much hotter effective temperatures. It is possible that high frequencies in a Maia star might arise in a binary system with a B-type primary and a normal δ Sct companion. Because the primary will be more luminous, the effective temperature would tend to reflect that of the B star rather than the δ Sct variable. In such a system, the observed pulsation amplitude of the δ Sct companion would be considerably reduced owing to the greater luminosity of the primary. This idea can be tested by comparing the amplitude distribution in Maia stars with that of δ Sct variables.
Figure 3 shows the distribution of peak amplitudes for δ Sct and Maia stars as well as β Cep variables. It is apparent that the amplitude distributions of Maia and δ Sct stars are the same, showing that Maia stars cannot be explained as binary δ Sct stars with B-type primaries. It is also quite interesting to note that the amplitude distribution in β Cep stars is rather different from the δ Sct or Maia variables. This supports the notion that Maia stars and δ Sct stars belong to the same population, but different from the population of β Cep variables.
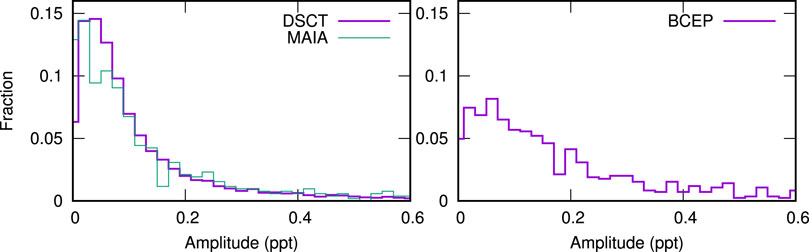
FIGURE 3. The left panel shows the amplitude distribution in δ Sct and Maia stars. The right panel shows the amplitude distribution in β Cep stars. Amplitudes are in parts per thousand (ppt).
5 Conclusion
From TESS light curves in sectors 1–65, 519 stars have been identified as Maia variables. These are defined as main sequence stars with 10,000 < Teff < 18,000 K with frequencies greater than 5 d−1. In this temperature range, high-frequency pulsations are not predicted to occur. It is generally supposed that the high frequencies in these anomalous stars are probably a result of rapid rotation (Salmon et al., 2014). By comparing the projected rotational velocities, v sin i, of Maia variables with those of main sequence stars or with SPB variables in the same temperature range, it is shown that Maia stars are not rapidly rotating (Figure 1).
It is also demonstrated that the instability strips of δ Sct and Maia variables merge to form one single instability strip extending to the cool end of the β Cep variables. This merger cannot be explained by uncertainties in effective temperatures or by assuming that Maia stars are binary systems with B-type primaries and δ Sct companions. Indeed, as Figure 2 shows, there is no clear separation between any variability group among early-type stars, as already pointed out in Balona and Ozuyar (2020).
Figure 2 also shows a continuous sequence of low-frequency pulsators from γ Dor variables (Teff ≈ 7,000 K) to the hottest SPB stars (Teff > 25,000 K). The instability strip defined by the majority of γ Dor stars actually lies within the δ Sct instability strip (Balona, 2018). The distinction between these two types of pulsating star arose from the fact that low frequencies in δ Sct stars are generally undetectable from ground-based photometry. There would have been no need to create a separate γ Dor class if this was not the case.
It is also concluded that there exists a sequence of low-frequency pulsating stars beyond the cool edge of the SPB instability strip. This sequence of anomalous pulsators eventually merges with the γ Dor stars. These cool SPB stars (or hot γ Dor stars) appear to have rotation rates similar to normal stars and are not rapidly rotating, as suggested by Sharma et al. (2022). The distinction between SPB and β Cep stars is also arbitrary. In fact, the definition of SPB stars with Teff > 18,000 K had to be modified to ensure that stars previously defined as β Cep variables many decades ago need not be reclassified as SPB (Balona and Ozuyar, 2020; Sharma et al., 2022).
The main conclusion of this paper is to re-emphasize the fact that our current ideas regarding pulsational driving in stars of the upper main sequence need to be revised. It is no longer possible to identify separate variability groups with their own distinct driving mechanisms. One can consider SPB stars as simply Maia stars in which low frequencies are preferentially selected, just as one can consider γ Dor stars as δ Sct stars in which low frequencies are preferentially selected. The difference between β Cep and SPB variables in the same temperature range may also be a mode selection effect.
It appears that in each star there are multiple driving mechanisms which compete with each other. The traditional opacity mechanisms are still dominant in the δ Sct and β Cep stars, which accounts for the peaks in the number density at around 7,500 K and 23,000 K respectively. However, we do not know the origin of the driving mechanism which must also be present in order to account for the Maia stars, the cool SPB stars and the merging of different variability groups. The discovery that starspots are pervasive along the entire main sequence (Balona, 2022c) is relevant. This seems to imply that surface convection present in all A and B stars. Including convection in the models may assist in resolving these problems, but convection may not be the only factor. A better understanding of the upper stellar layers of A and B stars is clearly required.
Data availability statement
The raw data supporting the conclusions of this article will be made available by the authors, without undue reservation.
Author contributions
LB: Conceptualization, Data curation, Formal Analysis, Investigation, Software, Writing–original draft.
Funding
The author declares financial support was received for the research, authorship, and publication of this article from the National Research Foundation of South Africa.
Acknowledgments
Funding for the TESS mission is provided by the NASA Explorer Program. Funding for the TESS Asteroseismic Science Operations Centre is provided by the Danish National Research Foundation (Grant agreement no.: DNRF106), ESA PRODEX (PEA 4000119301) and Stellar Astrophysics Centre (SAC) at Aarhus University. This work has made use of data from the European Space Agency (ESA) mission Gaia, processed by the Gaia Data Processing and Analysis Consortium (DPAC). Funding for the DPAC has been provided by national institutions, in particular the institutions participating in the Gaia Multilateral Agreement. This research has made use of the SIMBAD database, operated at CDS, Strasbourg, France. Data were obtained from the Mikulski Archive for Space Telescopes (MAST). STScI is operated by the Association of Universities for Research in Astronomy, Inc., under NASA contract NAS5-2655.
Conflict of interest
The author declares that the research was conducted in the absence of any commercial or financial relationships that could be construed as a potential conflict of interest.
Publisher’s note
All claims expressed in this article are solely those of the authors and do not necessarily represent those of their affiliated organizations, or those of the publisher, the editors and the reviewers. Any product that may be evaluated in this article, or claim that may be made by its manufacturer, is not guaranteed or endorsed by the publisher.
References
Balona, L. A., Baran, A. S., Daszyńska-Daszkiewicz, J., and De Cat, P. (2015). Analysis of kepler B stars: rotational modulation and maia variables. MNRAS 451, 1445–1459. doi:10.1093/mnras/stv1017
Balona, L. A., Engelbrecht, C. A., Joshi, Y. C., Joshi, S., Sharma, K., Semenko, E., et al. (2016). The hot γ Doradus and Maia stars. MNRAS 460, 1318–1327. doi:10.1093/mnras/stw1038
Balona, L. A. (2018). Gaia luminosities of pulsating A-F stars in the Kepler field. MNRAS 479, 183–191. doi:10.1093/mnras/sty1511
Balona, L. A. (2022a). Identification and classification of TESS variable stars. arXiv e-prints, arXiv:2212.10776. Available at: https://arxiv.org/pdf/2212.10776.pdf (Accessed August 16, 2023).
Balona, L. A., and Ozuyar, D. (2020). Pulsation among TESS A and B stars and the Maia variables. MNRAS 493, 5871–5879. doi:10.1093/mnras/staa670
Balona, L. A. (2022b). Rapidly oscillating TESS A-F main-sequence stars: are the roap stars a distinct class? MNRAS 510, 5743–5759. doi:10.1093/mnras/stac011
Balona, L. A. (2022c). Rotation of hot normal, peculiar and Be stars from space photometry. MNRAS 516, 3641–3649. doi:10.1093/mnras/stac2515
Daszyńska-Daszkiewicz, J., Walczak, P., and Pamyatnykh, A. (2017). “On possible explanations of pulsations in Maia stars,” in European Physical Journal Web of Conferences vol. 160 of European Physical Journal Web of Conferences, Açores Islands, Portugal, July 11-25, 2016.03013
Degroote, P., Aerts, C., Ollivier, M., Miglio, A., Debosscher, J., Cuypers, J., et al. (2009). CoRoT’s view of newly discovered B-star pulsators: results for 358 candidate b pulsators from the initial run’s exoplanet field data. A&A 506, 471–489. doi:10.1051/0004-6361/200911884
Gaia Collaboration Brown, A. G. A., Vallenari, A., Prusti, T., de Bruijne, J. H. J., Babusiaux, C., Bailer-Jones, C. A. L., et al. (2018). Gaia Data Release 2. Summary of the contents and survey properties. A&A 616, A1. doi:10.1051/0004-6361/201833051
Gaia Collaboration De Ridder, J., Ripepi, V., Aerts, C., Palaversa, L., Eyer, L., Holl, B., et al. (2022). Gaia data release 3: pulsations in main sequence obaf-type stars. arXiv e-prints, arXiv:2206.06075. Available at: https://arxiv.org/abs/2206.06075 (Accessed August 16, 2022).
Gaia Collaboration De Ridder, J., Ripepi, V., Aerts, C., Palaversa, L., and Eyer, L. (2023). Gaia Data Release 3. Pulsations in main sequence OBAF-type stars. A&A 674, A36. doi:10.1051/0004-6361/202243767
Gaia Collaboration Prusti, T., de Bruijne, J. H. J., Brown, A. G. A., Vallenari, A., and Babusiaux, C. (2016). The Gaia mission. A&A 595, A1. doi:10.1051/0004-6361/201629272
Jenkins, J. M., Caldwell, D. A., Chandrasekaran, H., Twicken, J. D., Bryson, S. T., Quintana, E. V., et al. (2010). Overview of the kepler science processing pipeline. ApJ 713, L87–L91. doi:10.1088/2041-8205/713/2/L87
Kallinger, T., Iliev, I., Lehmann, H., and Weiss, W. W. (2004). “The puzzling Maia candidate star α Draconis,” in The A-star puzzle. Editors J. Zverko, J. Ziznovsky, S. J. Adelman, and W. W. Weiss (Cambridge, Massachusetts: Astrophysics Data System), 848–852. vol. 224 of IAU Symposium.
Kurtz, D. W., Jayaraman, R., Sowicka, P., Handler, G., Saio, H., Labadie-Bartz, J., et al. (2023). HD 42477: coupled r modes, g modes, and a p mode in an a0vnne star. MNRAS 521, 4765–4774. doi:10.1093/mnras/stad858
Lehmann, H., Scholz, G., Hildebrandt, G., Klose, S., Panov, K. P., Reimann, H.-G., et al. (1995). Variability investigations of possible Maia stars. A&A 300, 783.
McNamara, B. J. (1985). Maia variables and upper-main-sequence phenomena. ApJ 289, 213–219. doi:10.1086/162881
Monier, R. (2021). The rapid far-ultraviolet variability of Maia recorded by IUE. Res. Notes Am. Astronomical Soc. 5, 81. doi:10.3847/2515-5172/abf43b
Mowlavi, N., Barblan, F., Saesen, S., and Eyer, L. (2013). Stellar variability in open clusters. I. A new class of variable stars in NGC 3766. A&A 554, A108. doi:10.1051/0004-6361/201321065
Mowlavi, N., Saesen, S., Semaan, T., Eggenberger, P., Barblan, F., Eyer, L., et al. (2016). Stellar variability in open clusters. II. Discovery of a new period-luminosity relation in a class of fast-rotating pulsating stars in NGC 3766. A&A 595, L1. doi:10.1051/0004-6361/201629175
Percy, J. R., and Wilson, J. B. (2000). Another search for Maia variable stars. PASP 112, 846–851. doi:10.1086/316577
Saio, H., Ekström, S., Mowlavi, N., Georgy, C., Saesen, S., Eggenberger, P., et al. (2017). Period-luminosity relations of fast-rotating B-type stars in the young open cluster NGC 3766. MNRAS 467, 3864–3873. doi:10.1093/mnras/stx346
Salmon, S. J. A. J., Montalbán, J., Reese, D. R., Dupret, M.-A., and Eggenberger, P. (2014). The puzzling new class of variable stars in NGC 3766: old friend pulsators? A&A 569, A18. doi:10.1051/0004-6361/20132-3259
Sharma, A. N., Bedding, T. R., Saio, H., and White, T. R. (2022). Pulsating B stars in the scorpius-centaurus association with TESS. MNRAS 515, 828–840. doi:10.1093/mnras/stac1816
Struve, O., Sahade, J., Lynds, C. R., and Huang, S. S. (1957). On the spectrum and brightness of Maia (20 C tauri). ApJ 125, 115. doi:10.1086/146286
Keywords: stars: oscillations, stars: δ Scuti, stars: γ Doradus, stars: SPB, stars: β Cephei
Citation: Balona LA (2023) Maia variables and other anomalies among pulsating stars. Front. Astron. Space Sci. 10:1266750. doi: 10.3389/fspas.2023.1266750
Received: 25 July 2023; Accepted: 24 August 2023;
Published: 14 September 2023.
Edited by:
Yogesh Chandra Joshi, Aryabhatta Research Institute of Observational Sciences, IndiaReviewed by:
Mkrtichian Egishe David, National Astronomical Research Institute of Thailand, ThailandGiovanni Catanzaro, Osservatorio Astrofisico di Catania (INAF), Italy
Copyright © 2023 Balona. This is an open-access article distributed under the terms of the Creative Commons Attribution License (CC BY). The use, distribution or reproduction in other forums is permitted, provided the original author(s) and the copyright owner(s) are credited and that the original publication in this journal is cited, in accordance with accepted academic practice. No use, distribution or reproduction is permitted which does not comply with these terms.
*Correspondence: L. A. Balona, bGFiQHNhYW8uYWMuemE=