Corrigendum: Dogs with Acute Myeloid Leukemia Have Clonal Re-arrangements in T and B Cell Receptors
- 1Department of Population Medicine and Diagnostic Sciences, College of Veterinary Medicine, Cornell University, Ithaca, NY, United States
- 2Animal Health Diagnostic Center, College of Veterinary Medicine, Cornell University, Ithaca, NY, United States
Clonality testing for rearrangements in the complementarity-determining region 3 of the immunoglobulin heavy chain of B lymphocytes (B cell receptor) and the T cell receptor of T lymphocytes helps distinguish between clonal and non-clonal expansions of lymphocytes. There are rare reports of clonally rearranged T and B cell receptors in dogs with acute myeloid leukemia (AML). Our objective was to determine the frequency of clonally rearranged T and B cell receptors in dogs with AML. Archived slides from historical cases of AML (from January 2010 to June 2013) and slides or liquid specimens [blood, bone marrow (BM), body cavity fluid, or tissue aspirates] from cases of AML diagnosed between June 2013 and February 2017 were used in the study. A diagnosis of AML was made on the basis of more than 20% immature neoplastic cells (“blasts”) in blood, BM, or extramedullary tissues, displaying features of myeloid differentiation. Myeloid differentiation was based on a combination of morphologic criteria, positive flow cytometric labeling for surface antigens typical of myeloid origin (e.g., CD11b, CD11c, CD14 with a general lack of expression of T or B cell markers), or positive cytochemical staining reactions for myeloid-associated enzymes (e.g., alkaline phosphatase, chloroacetate esterase). There were 63 cases of AML diagnosed during this period; however, slides or liquid specimens with sufficient DNA for testing were only obtained from 25 dogs. Affected dogs represented various breeds and were a median of 8 years old, with more male (64%) than female (36%) dogs. Common clinical signs were peripheral or internal lymphadenopathy (10/25 dogs, 40%) and hepatomegaly or splenomegaly (10/25 dogs combined, 40%). Typical hematologic findings were bi- or pancytopenia (23/25 dogs, 92%), with circulating blasts (21/25, 84%). Solitary clonal (4 B cell, 6 T cell) and biclonal (6 B and T cell) rearrangements in B or T cell receptors were found in 16 dogs (64%). Our results indicate that dogs with AML can have a high frequency of clonally rearranged T or B cell receptors, including biclonality, and clonality testing should not be used as a tool to distinguish between acute leukemia of myeloid or lymphoid origin.
Introduction
Testing for antigen rearrangements in T and B cell receptors on genomic DNA is a useful tool to help identify clonal expansions in lymphocytes in veterinary medicine. Testing is accomplished via polymerase chain reactions using primers designed to amplify the complementarity-determining region 3 (CDR3) of immunoglobulin chains (usually the heavy chain or IgH) of B cells (B cell receptor) and the T cell gamma receptor (1, 2). Thus, clonality testing is known colloquially as polymerase testing for antigen receptor rearrangements (2). Amplified products have been traditionally detected using ethidium bromide and agarose gel electrophoresis (3, 4); however, higher resolution techniques, such as capillary gel electrophoresis, are supplanting this older method (1, 2, 5–7). Clonality testing is primarily used to distinguish between neoplastic and reactive lymphocyte expansions (1, 2, 6–8); however, this testing is also used as a means to phenotype lymphoid neoplasms as B or T in origin, particularly with tumors showing expression of more than one lineage with flow cytometry or immunohistochemical (IHC) staining (2, 5, 6, 8–13). The use of clonality as a phenotyping tool is being extended to myeloid neoplasms, where clonality testing has been used as a means to distinguish between acute myeloid leukemia (AML) and lymphoid neoplasms (lymphoma or leukemia) (6, 14, 15). However, we have observed clonal rearrangements in both B and T cell receptors in cases of AML in dogs and a previous study documented a clonally rearranged B cell receptor in one of three dogs with AML (3). The goal of this study was to document the frequency of clonally arranged lymphoid receptors in a cohort of dogs with AML.
Materials and Methods
This study was conceived in June 2013 and included historical cases of AML, in which archived slides were available for clonality testing (January 2010 to June 2013), and new cases of AML diagnosed from samples submitted to the Clinical Pathology laboratory in the Animal Health Diagnostic Center at Cornell University for routine diagnostic testing or for leukemia classification with phenotyping techniques, including flow cytometry and cytochemical staining (June 2013 to February 2017). A diagnosis of AML was made on the basis of greater than 20% immature neoplastic cells (“blasts”) in blood, bone marrow (BM), body cavity fluids, or extramedullary tissues, in which neoplastic cells were displaying features of myeloid differentiation. Myeloid differentiation was based on a combination of morphologic features, expression of myeloid markers on flow cytometric analysis, or expression of enzymes characteristic of myeloid origin on cytochemical staining (16) (Table 1), with the help of an algorithm centered on the order in which the diagnostic tests were usually performed (Figure 1). Whenever possible, the leukemia was further classified into subtypes, using defined World Health Organization (WHO) criteria (17, 18) (Table 2). When the subtype was difficult to determine (e.g., lack of BM results), the most likely subtype was selected and defined as “suspect.” Clonality testing was performed on archived slides from historical and newly diagnosed cases of AML or on liquid samples (blood or BM, body cavity fluid, or tissue aspirates) on newly diagnosed cases of AML.
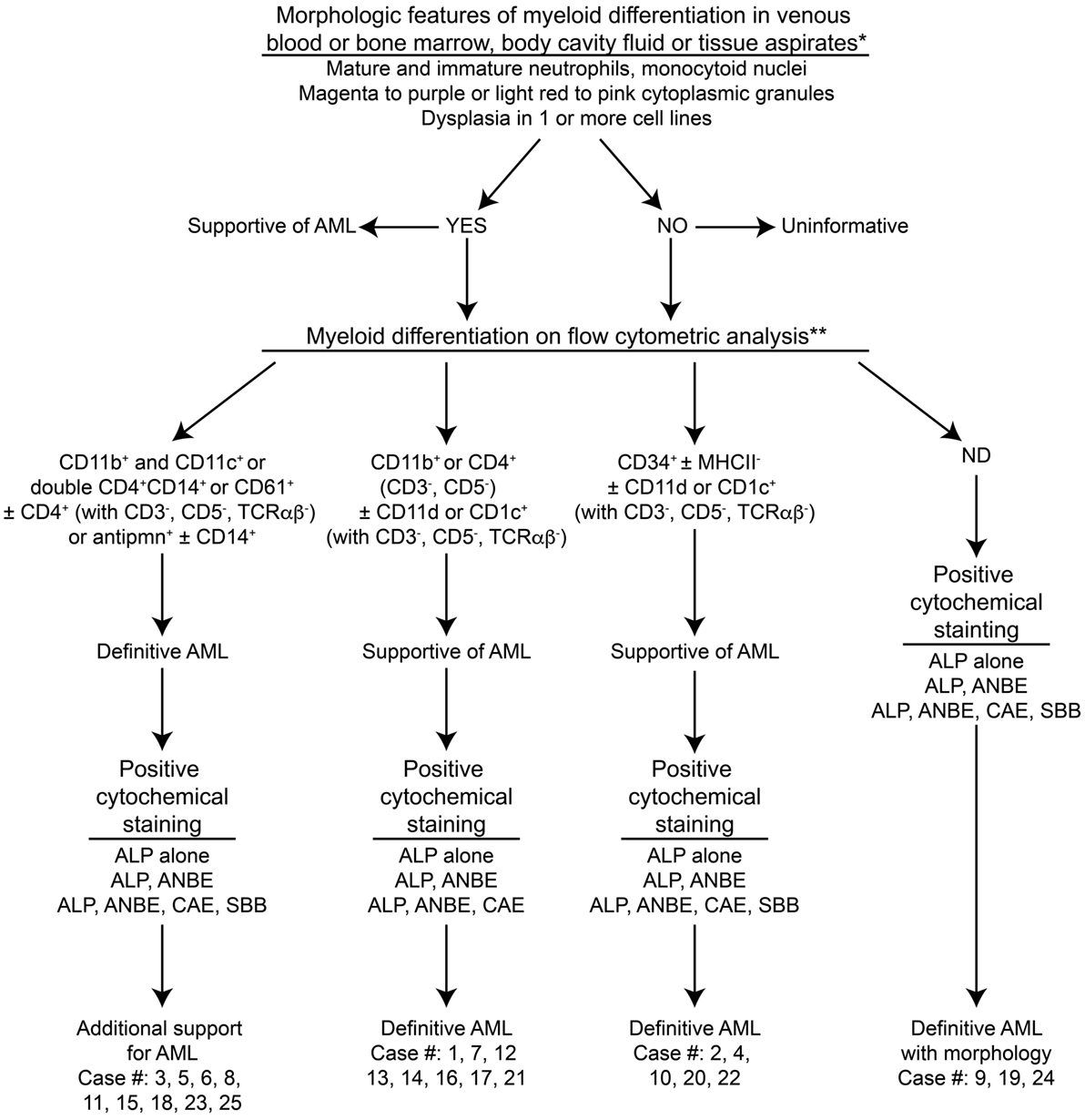
Figure 1. Algorithm used to diagnose acute myeloid leukemia (AML) in the 25 dogs of this study. This diagnostic algorithm was based on the order in which tests were generally performed in our laboratory, i.e., morphologic assessment of blood, bone marrow, or body cavity fluid or tissue aspirates, followed by flow cytometric analysis (performed routinely twice a week), followed by cytochemical staining (performed as needed). After completion of all the tests, the results were reevaluated, and a diagnosis of AML was based on the combined data. The path used to diagnose each case (#) is also shown. More details on the criteria are provided in Table 1.
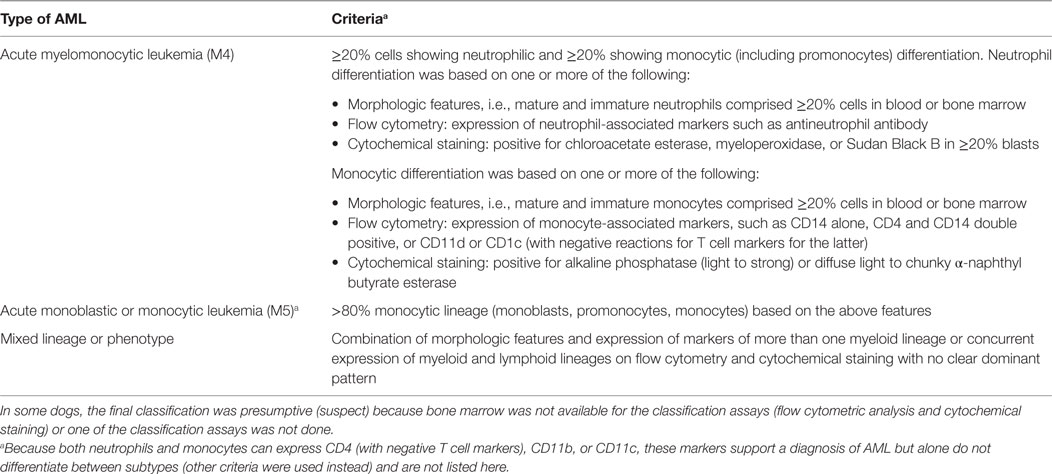
Table 2. Criteria used for classification of the subtype of acute myeloid leukemia (AML) based on the World Health Organization scheme of AML – not otherwise specified (17, 18).
Hematologic Analysis
Hemograms were performed on all EDTA-anticoagulated venous blood (VB) samples submitted to the Clinical Pathology Laboratory at Cornell University, with exceptions noted (blood results provided by the veterinarian submitting samples). Hemogram results were obtained from an automated hematology analyzer (ADVIA 2120, Siemens Healthcare Diagnostics Inc., Tarrytown, NJ, USA), and differential leukocyte counts and blood smear examination were performed by trained medical technologists on smears stained with modified Wright’s stain, using an automated stainer (Hematek 1000, Siemens Healthcare Diagnostic Inc.). The clinical pathologist on duty reviewed the blood smears.
Cytologic Evaluation of BM, Body Cavity Fluid, and Tissue Aspirates
Bone marrow, body cavity fluid (peritoneal or pleural), or tissue aspirates (lymph node, spleen, or liver) were performed on dogs admitted to the Cornell University Hospital for Animals and smears were prepared in the Clinical Pathology Laboratory or by the clinician obtaining the sample. For mailed-in samples, BM smears were prepared from submitted fluid samples, and these smears or submitted smears of BM, body cavity fluid, and tissue aspirates were stained with modified Wright’s stain (if not prestained) and examined by the clinical pathologist on duty. For testing done at Colorado State University (CSU), smears were submitted for review by one author (Stokol).
Flow Cytometric Analysis of Liquid Specimens
Flow cytometric analysis was performed on VB or BM or tissue aspirates at Cornell University in most dogs as previously described in detail (16), using conjugated and unconjugated antibodies, with the addition of antibodies against CD18 (a pan-leukocyte marker), CD61 (a platelet or megakaryocyte marker), and mature neutrophils (Table 3). In brief, cell populations were identified and gated on their forward and side scatter characteristics, with tumor cells (blasts) typically falling into the large lymphocyte or monocyte gate (Figure 2). In several cases, tumor cell events spanned the small lymphocyte and large lymphocyte/monocyte regions. Cells within the gated region of interest (tumor cell gate) were considered positive for a marker if greater than or equal to 20% of the neoplastic cells were labeled with the antibody (16), with the exception of CD34, in which normal leukocytes are negative for this marker and >5% of labeled cells was considered a positive reaction. Not all markers were applied to samples from each dog, and provided negative reactions in this study are mostly confined to the pan-leukocyte antigen CD18, MHCII [as a marker of AML (16)], and T or B cell markers (as a means to exclude lymphoid origin). Flow cytometric analysis was done on one case at CSU as part of their phenotyping diagnostic service. The service typically tests for CD45, CD18, CD3, CD5, CD4, CD8, CD21, CD14, MHCII, and CD34 using conjugated antibodies.
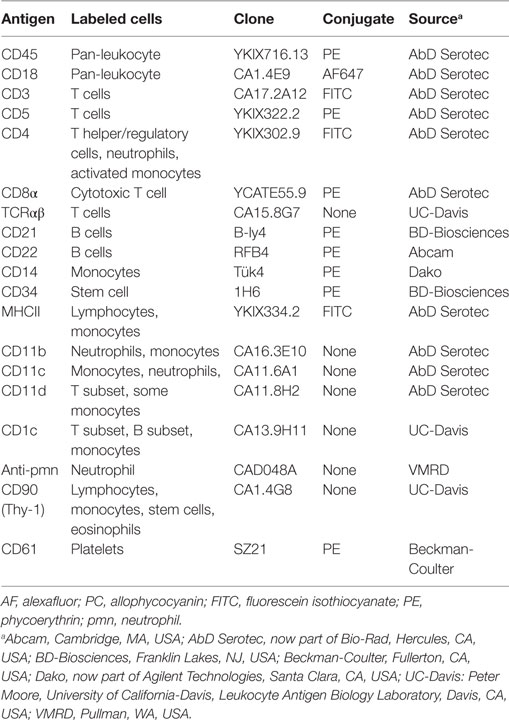
Table 3. Antibodies used in flow cytometry at Cornell University to label antigens on tumor cells in liquid samples (blood or bone marrow, body cavity fluid, or tissue aspirates) from dogs with acute myeloid leukemia.
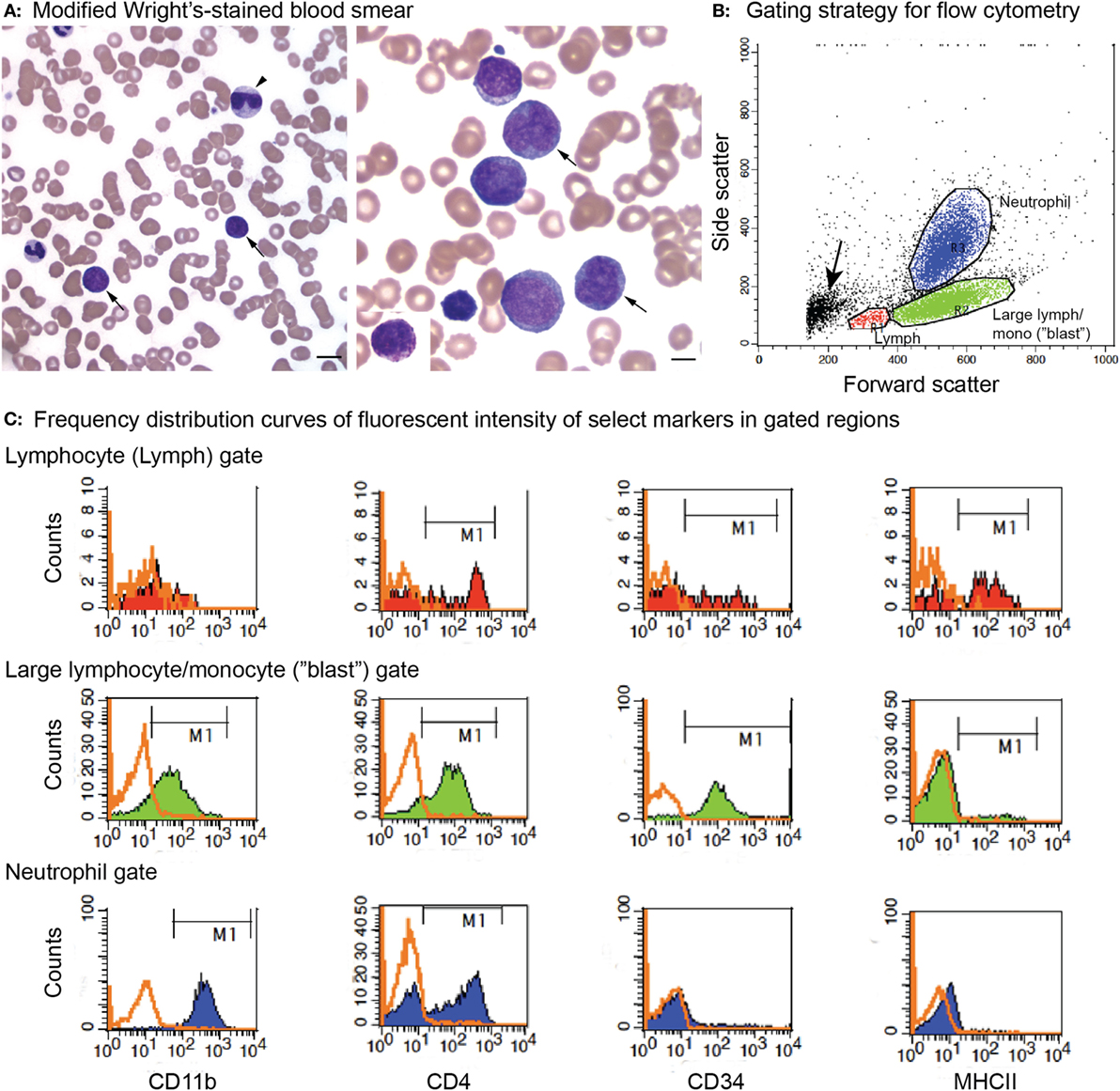
Figure 2. Peripheral blood morphologic features and select flow cytometric results from a dog with acute myeloid leukemia (AML) (case 12). The leukemia was classified as myelomonocytic (M4) based on a combination of ≥20% mature and immature neutrophils in blood, morphologic (monocytoid nuclei), and cytochemical evidence of monocytic differentiation (positive for alkaline phosphatase or α-naphthyl butyrate esterase) in tumor cells in blood and positive expression of differentiation antigens common to neutrophils and monocytes (CD11b, CD4) on flow cytometric analysis. (A) Representative images of modified Wright’s-stained smears of peripheral blood. Left panel: The dog had 37% blasts (arrows) with a normal neutrophil count but a moderate left shift (2.5 × 106/L immature neutrophils). Some of the immature neutrophils were dysplastic (giant band neutrophil with cytoplasmic basophilia, arrowhead). Bar = 10 µm. Right panel: Higher magnification of the blasts, with two cells displaying lobulated (monocytoid) nuclei (arrows), a morphologic feature supporting monocytic differentiation. Bar = 5 µm. Right panel inset: Rare blasts had moderate numbers of purple cytoplasmic granules. We have previously reported on the presence of these “granular blasts” in dogs with AML (16). (B) Flow cytometric gating strategy for analysis of marker expression of the tumor cells in dogs with AML. Cells were gated as small lymphocytes (red, lymph), large lymphocytes/monocytes (green, large lymph/mono), or neutrophils (blue) based on forward and side scatter characteristics. In this case, blasts fell mostly in the large lymphocyte/monocyte (“blast”) gate. With some leukemias, only one or two (“blast” with lymphocyte or neutrophil) gates could be generated. The small events (arrow) were likely dead cells or fragments of dead cells. (C) Events within each gate were displayed on a frequency distribution curve (histogram plot) of counts versus fluorescent intensity of the antibody against the marker of interest. Cells positive for the marker were identified by the M1 marker region, corresponding to the location of the solid curve relative to the isotype control (overlaid orange open curve). Cells within the large lymphocyte/monocyte or “blast” gate (middle row) were positive for the neutrophil or monocyte marker, CD11b (far left column), and the neutrophil or activated monocyte marker, CD4 (middle left column). They were also positive for the stem cell marker CD34 (middle right column) but negative for MHCII (far right column). A few small tumor cells were present in the small lymphocyte gate (top row, CD34-positive cells); however, most of the cells in this gate were positive for T (CD3 and CD5) or B (CD21 and CD22) cell markers (not shown) and MCHII, as expected for normal lymphocytes. The CD4 expression is likely mostly on T lymphocytes. Cells within the neutrophil gate (lower row) had staining characteristic of neutrophils, i.e., positive for CD11b and CD4 and negative for CD34 and MCHII, serving as an internal positive and negative control for these antigens. Note, the intensity of CD11b and CD4 (distance along the X-axis) was lower for the blasts than the neutrophils, which can be a helpful feature to identify subpopulations of blasts among residual normal cells.
Cytochemical Staining of Smears of Blood or BM, Body Cavity Fluid, or Tissue Aspirates
Cytochemical staining was performed at Cornell University, using various combinations of alkaline phosphatase (ALP), α-naphthyl butyrate esterase (ANBE), chloroacetate esterase (CAE), myeloperoxidase, and Sudan Black B, as previously described (16). Not all cytochemical stains were done on every case. As for our previous study on acute leukemia, 100 neoplastic cells were counted when possible, and tumor cells were considered positive if greater than or equal to 3% were positive for the cytochemical stain (16).
Clonality Testing
Clonality testing was done by the Molecular Diagnostics Laboratory in the Animal Health Diagnostic Center at Cornell University or the Clinical Immunology Laboratory at CSU (2). In the Molecular Diagnostics Laboratory, we used high-resolution melt curve analysis, followed by polyacrylamide gel electrophoresis for verification as needed. In brief, genomic DNA was retrieved from cytologic or blood smears by scraping material with a scalpel blade into a 1.5-mL polypropylene snap cap tube. DNA was extracted from the retrieved material or liquid samples using DNeasy DNA extraction columns (Qiagen, Germantown, MD, USA). The DNA was amplified using MeltDoctor™ HRM Master Mix, according to the manufacturer’s recommendations (Life Technologies, Carlsbad, CA, USA). The mixture includes a fluorescent dye that intercalates with double-stranded DNA. Four primer sets (final primer concentration of 0.52 µM) were used, including two sets to amplify the IgH CDR3 region, one set to amplify the T cell gamma receptor CDR3 region, and one set to amplify the constant region of the IgM heavy chain (Cμ) as an internal positive control. Primer sequences were based on previously published data (3). All reactions were run in duplicate. Cycling conditions were 95°C for 15 min, followed by 40 cycles at 94°C for 8 s, 60°C for 10 s, and 72°C for 15 s. As the temperature increases with each cycle, the DNA melts yielding single-stranded products, leading to the release of the intercalated fluorescent dye and a decrease in fluorescence. The melting temperature is predictable and based on the sequence of nucleotides in the starting DNA. The rate of decrease in fluorescence is monitored in real time, producing a melt curve, where normalized fluorescent intensity is plotted against temperature. This is converted to a negative derivative curve, which displays fluorescent intensity versus the temperature at which 50% of the DNA has melted (19). Clonal populations result in a single- or double-sharp peak on the derivative curve, whereas polyclonal populations produce multiple, broader, or wider derivative curves due to the different DNA sequences melting at different temperatures (Figure 3). Melt curves were generated using the default setting of the software (High Resolution Melt Software v3.0.1, Applied Biosystems, Foster City, CA, USA). When needed, reaction products were resolved with a 10% polyacrylamide gel in a Tris buffer (89 mM Tris, 89 mM boric acid, 2 mM EDTA, pH 8.4), and bands were visualized by immersion in a DNA dye (3× GelRed Nucleic Acid Gel Stain, Biotium, Fremont, CA, USA). Bands were visualized by UV transillumination and photographed (Figure 3).
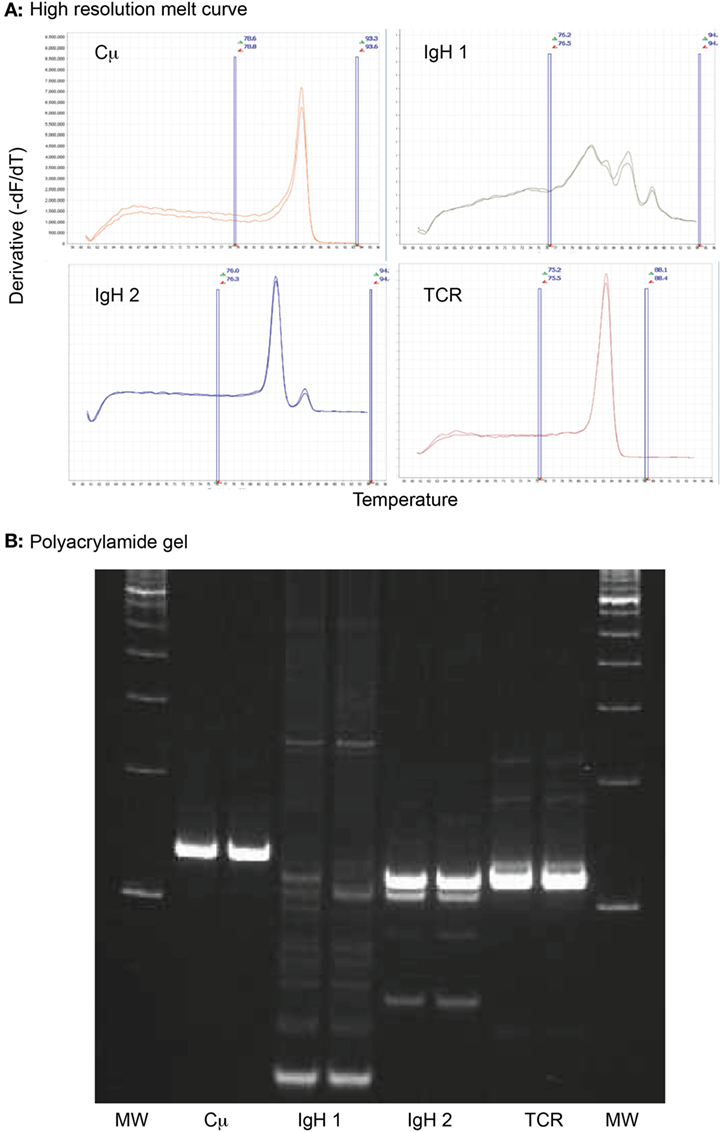
Figure 3. High-resolution melt curve analysis of amplified DNA products retrieved from an archived venous blood smear of a dog with acute myeloid leukemia (case 1). (A) Sharp peaks in the negative derivative curves yielded from the normalized DNA melt curve showed that the tumor cells had clonal rearrangements in complementarity-determining region 3 (CDR3) of the immunoglobulin heavy chain with primer set 2 (IgH 2) and the CDR3 of T cell gamma receptor (TCR), i.e., a biclonal rearrangement. The multiple broad peaks with the first primer set for the CDR3 of the immunoglobulin heavy chain (IgH 1) is compatible with a polyclonal product. The positive control (Cμ) yielded the expected single peak. All reactions were run in duplicate. Note that all curves have the same Y- and X-axis. The area between the vertical blue lines represent the active melt curve region, in which the calculations are performed. (B) A 10% polyacrylamide gel was performed on the sample to verify the results of the high-resolution melt curve analysis. This showed a broad smear (polyclonal) with primer set 1 for the heavy chain CDR3 (IgH 1), a strong clonal band with primer set 2 for the heavy chain CDR3 (IgH 2), and a strong single clonal band with the T cell gamma receptor CDR3 primer set (TCR). The positive control (Cμ) yielded a single strong product, as expected. All reactions were run in duplicate. MW represents the molecular weight marker (100 base pair ladder).
Internal validation of the procedure was performed on samples from 28 cases, including 18 cases of lymphoid neoplasia (12 B cell, 6 T cell) and 10 reactive conditions. Clonality was identified in 14 of 18 (10/12 B cell and 4/6 T cell) samples with lymphoid neoplasia, yielding a sensitivity of 78%. Non-clonal results were seen in 9 of 10 reactive conditions, yielding a specificity of 90%. The one reactive sample with B cell clonality was diagnosed with lymphoid hyperplasia on a splenic aspirate. The positive result was confirmed with repeat testing at CSU. The sensitivity of our assay falls within that reported for similar primer sets in other studies (5–7, 10, 20).
Ethics Statement
Residual samples were used (archived slides or that remaining after routine clinical pathologic testing); therefore, approval from the Institutional Biosafety and Animal Use Committee or client consent was not required.
Results
Over the time period of this study (June 2010 to February 2017), we diagnosed AML in 63 dogs; however, archived or fresh specimens were only available for 27 dogs. The yield of DNA from samples of 2 dogs was too low to provide results for clonality testing, and these dogs were excluded, leaving a total of 25 dogs from our institution (#1–25) for inclusion in the study. Dr. A. Avery (CSU, with consent from the referring veterinarian, Dr. C. Herrera) kindly provided historical information and flow cytometric and clonality results from case #25. Dogs were of various breeds, consisting mostly of Labrador Retrievers (n = 5) with fewer German Shepherd dogs (n = 3), Golden Retrievers (n = 2), Boxers (n = 2), and Rottweilers (n = 2). Single dogs represented other pure breeds and mixed breeds. Dogs were generally older, with a median age of 8 years (range, 1.9–12 years). There were 16 male dogs (64%), most of which were neutered, and 9 female dogs (36%), most of which were spayed. Presenting clinical signs were vague and not specific in many dogs, but some consistent findings were mild to moderate lymphadenopathy, affecting single or multiple peripheral or internal lymph nodes (n = 10), organomegaly (splenomegaly, hepatomegaly, or both, n = 10), suspect leukemia (e.g., leukocytosis, big buffy coat, n = 9), and previously documented single or multiple cytopenias (n = 5). Two dogs had a prior diagnosis of lymphoid neoplasia (lymphoma and lymphoblastic leukemia) (Table 4).
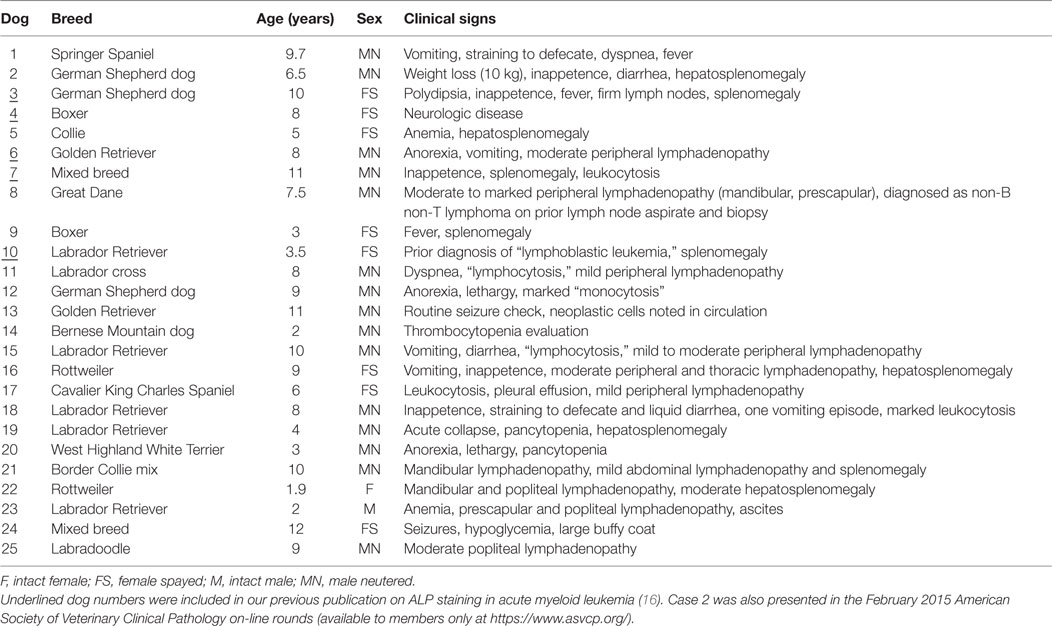
Table 4. Signalment and presenting clinical signs in 25 dogs with acute myeloid leukemia in which clonality testing was performed.
Hemogram results were available for all dogs, two of which were provided by the referring veterinarian (with no concurrent blood smear) (Table 5). Most of the dogs were anemic (21/25, 84%), which was normocytic and normochromic in 57% (12/21) and non-regenerative (absolute reticulocyte counts within reference intervals) in 79% of anemic dogs in which a reticulocyte count was performed (15/19, including one dog with a marginally increased reticulocyte count of 93 × 106/L). Four dogs had a mildly to moderately regenerative anemia. Red blood cells were macrocytic and normochromic or hypochromic in eight dogs. In two of these dogs, the blood samples were mailed to the laboratory, and the macrocytic hypochromic red blood cell indices could be an artifact of red blood cell swelling with storage. Two dogs had evidence of erythroid dysplasia, which could be contributing to the macrocytosis. A normoblastosis was detected in six dogs. Leukocytosis was more common (14/25, 56%) than leukopenia (8/25, 32%) and, in most dogs, the leukocytosis was due to high numbers of blasts (12/14, 86%). Blasts were seen in 22 dogs overall (88%), with rare circulating blasts identified on blood smear examination, but not included in the differential cell count, in 4 dogs. A neutrophilia was seen in three dogs (12%), all of which had an increase in immature neutrophils (left shift), with a monocytosis in seven dogs (28%) and lymphocytosis in three dogs (12%). Ten dogs were neutropenic (40%), only one of which had a left shift, and thrombocytopenia was seen in most dogs (22/25, 88%). The mean platelet volume was high in 14 of 18 dogs (78%) in which a result was obtained. Seven dogs were pancytopenic (28%), with pancytopenia defined as a non-regenerative anemia, neutropenia, and thrombocytopenia (Table 5). Of the 23 dogs in which blood smears were reviewed, 7 dogs (30%) had cytologic evidence of dysplasia in one or more cell lineages in blood (Table 6).
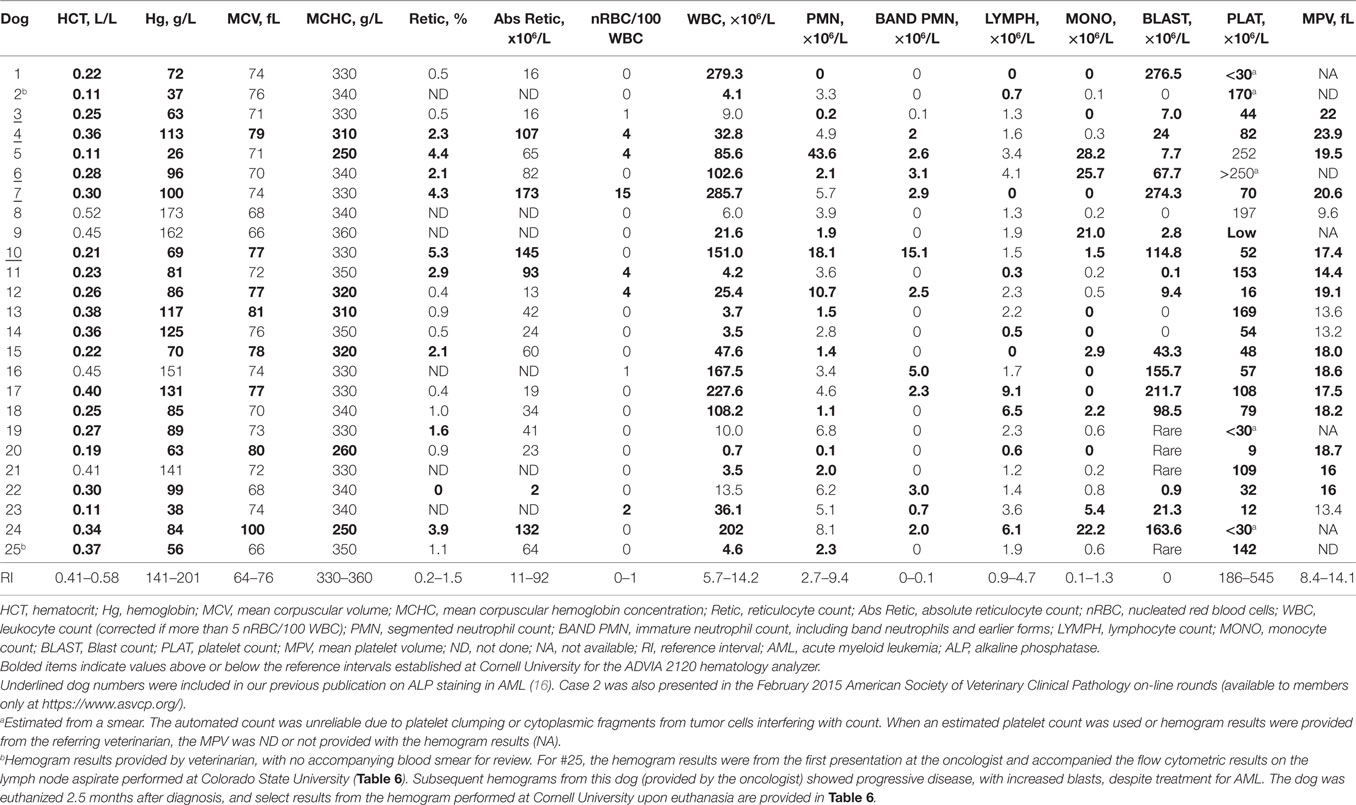
Table 5. Hematologic findings in 25 dogs with acute myeloid leukemia in which clonality testing was performed.
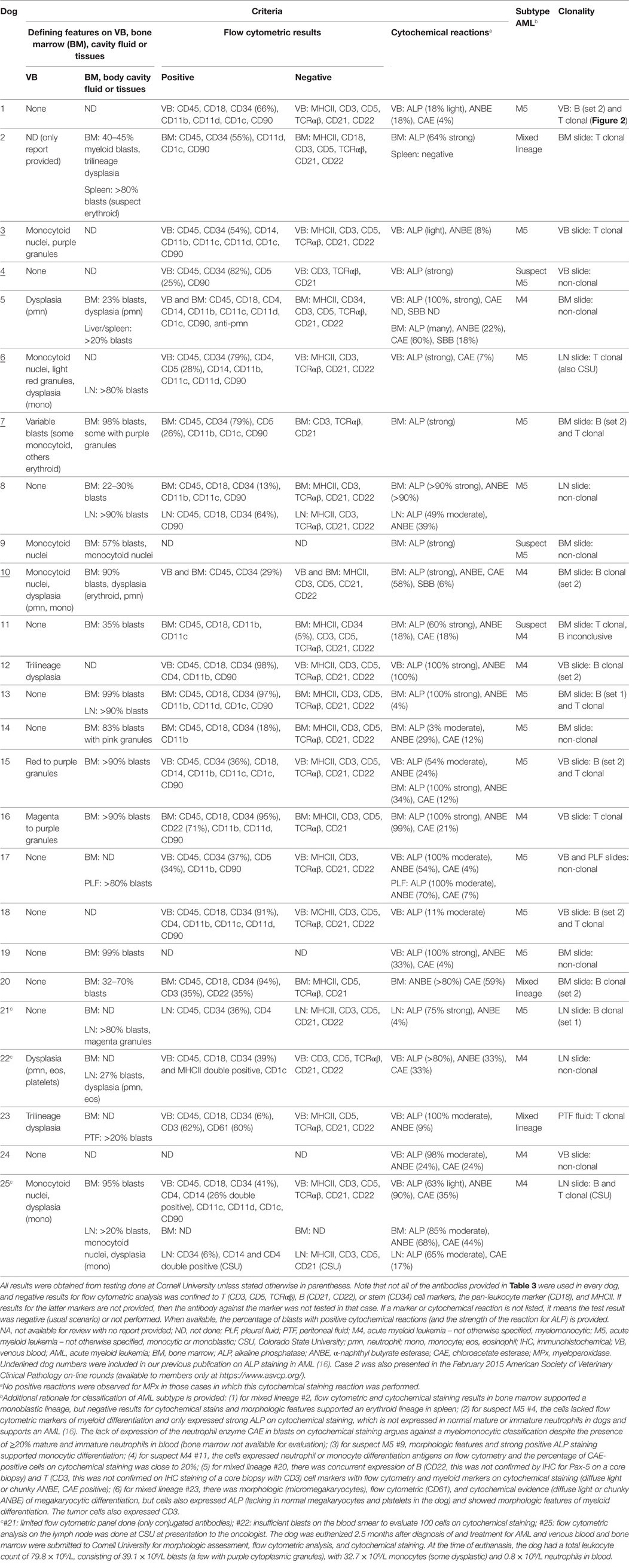
Table 6. Morphologic findings from blood or cytologic smears and results from flow cytometric labeling, cytochemical staining, and clonality testing in 25 dogs with AML.
Bone marrow aspirates were done in 14 dogs, all of whom had a blast count >20%, compatible with a diagnosis of acute leukemia (17). In one of these dogs, the BM aspirate was done after the dog had been treated with AML for 2.5 months (#25). The dog stopped responding to treatment and was euthanized, at which time blood and BM aspirates were sent to Cornell University for morphologic assessment, flow cytometric analysis, and cytochemical staining. These results supplemented the original results obtained from lymph node aspirates (flow cytometry done at CSU; Table 6). Tissue infiltrates (liver, spleen, lymph nodes) or involvement of body cavities (abdomen and thorax) were seen in 10 dogs on aspiration. Dysplasia was evident in cells in the BM, body cavity, or tissue aspirates of five dogs. On flow cytometric labeling, most of the tumor cells in the dogs with AML expressed CD45, CD18, and CD34 with aberrant negative expression of MHCII, as previously reported (16). CD34 expression was negative in two cases, both of which were subtyped as likely acute myelomonocytic leukemia. In 22 dogs in which flow cytometry was performed, tumor cells in 15 dogs lacked expression of T (CD3, CD5) or B (CD21, CD22) cell markers. Tumor cells from four dogs weakly expressed CD5, but the cells in three of these four dogs expressed other myeloid antigens, such as CD11b, and all had strong positive reactions for ALP or other myeloid enzymes (diffuse light or chunky ANBE, CAE) and were negative for CD3 and TCRαβ, supporting a diagnosis of AML over acute lymphoid leukemia (ALL). Similarly, two cases expressed CD3 but not CD5 or TCRαβ; the CD3 expression in one of these cases was not confirmed on IHC staining of a core biopsy of the BM (#20). The latter case of mixed lineage AML was also positive for CD22, but negative for Pax-5 on IHC staining of the marrow core sections. CD22, but not CD21, was expressed on a case of myelomonocytic AML (#16) (Table 6).
Clonal rearrangements in T or B cell receptors were seen in 16 dogs (64%). Of these, six dogs had clonal arrangements in both T and B (mostly with primer set 2) cell receptors (biclonal), six dogs had only a clonally rearranged T cell receptor (DNA quantity was insufficient to identify B cell clonality in one of these dogs) and four dogs had only a clonally rearranged B cell receptor (mostly with primer set 2). In one dog (#6), clonality testing was performed at both Cornell University and CSU as part of internal verification studies and yielded the same result of T cell clonality.
Discussion
Our results indicate that a high proportion of dogs with AML (64% in this cohort) can express clonally rearranged CDR3 regions in B or T cell receptors. This result indicates that clonality testing should not be relied upon for distinguishing between AML and lymphoid neoplasms, such as ALL or lymphoma with a secondary leukemia, as previously recommended (2). Recently, Keller and colleagues have also advocated against the use of clonality testing for distinguishing B from T cell neoplasms (1). However, in many cases of lymphoid neoplasia, the tumor shows fidelity between clonality and other phenotyping tests (IHC or flow cytometry) (2, 6, 20), and clonality assessment may still be useful, at the very least for confirming neoplasia, in sites where sufficient cells cannot be retrieved for other phenotyping techniques or invasive diagnostic procedures are contraindicated (12).
Our results of clonally rearranged lymphoid receptors are similar to that reported previously in one of three dogs (3) and people with AML. In 4 studies of patients with AML diagnosed with cytochemical staining using traditional French-American-British working group criteria (21), 7% of 14 patients had a clonal T cell rearrangement (22), 36% of 25 patients had B (n = 3), T (n = 4), or biclonal (n = 2) rearrangements (23), 13% of 24 patients had clonal T (n = 2) or biclonal (n = 1) rearrangements (24), and 40% of 35 patients had clonal B cell rearrangements (T cell clonality not tested) (25). Of note, in three of these studies of human patients with AML, tumor cells aberrantly expressed T or B cell antigens; however, expression of lineage-associated antigens was not associated with clonality of the same lineage in all cases (23–25). We found a similar result in this study, in which tumor cells in dogs with AML expressed B or T cell surface antigens, yet displayed non-clonal, biclonal, or B or T cell receptor clonal rearrangements. Although non-clonal or polyclonal results on clonality testing have been used to support a diagnosis of AML in previous reports (6, 10, 14, 15), false-negative reactions for clonality do occur in lymphoid neoplasms, with reported sensitivities ranging from 67 to 98% (5–7, 10, 20), using similar primer sets to that used herein.
Dogs with AML had a high frequency of biclonal rearrangements, i.e., 38% of those cases with clonal rearrangements (6/16) or 24% of this cohort of 25 dogs. Clonal rearrangements in both B and T cell receptors have been reported in dogs with lymphoid neoplasms; however, the proportion of biclonality in dogs with AML is higher than that reported for dogs with lymphoid neoplasia, which ranges from 1 to 10% (3, 5, 6, 10). These data indicate that if clonality testing is done in a dog with acute leukemia of unclear phenotype, a B and T cell biclonal result should raise the index of suspicion for an underlying AML and prompt further testing for AML, such as expanded testing for myeloid antigen expression on flow cytometric analysis or cytochemical staining. Similarly, a diagnosis of AML should be considered with a non-clonal test result, particularly if the leukemia lacks expression of B or T cell markers with flow cytometry and is positive for the stem cell marker CD34 and negative for MHCII. The latter results are characteristic findings in dogs with AML (16) but unusual with lymphoid neoplasms, which frequently express MHCII and often lack CD34 (4, 16). However, there are known variants of T and B cell lymphoma or leukemia that drop off MHCII expression (26, 27) or that express CD34 (13, 16). Furthermore, two dogs (both likely myelomonocytic leukemia, #5 and #11) lacked CD34 and MHCII expression, as we have previously reported for this subtype of AML (16), indicating that not all AML express CD34.
A limitation of this study is that we used less-sensitive techniques and “older” primer sets for performing clonality assessment at our institution. We unfortunately still do not have the equipment to perform more sensitive assays, and there is insufficient material remaining to submit non-clonal samples to other sites for testing. Thus, it is possible that some of the cases that were classified as non-clonal would have been reclassified as clonal if newer primer sets or more sensitive techniques were used (1, 2, 6, 7, 28). A lower sensitivity of clonality detection in this study could mean that an even higher proportion of dogs with AML had clonal rearrangements. In addition, the assessment of clonality with high-resolution melt curve analysis is subjective, and there are more quantitative techniques that can be done with this method to reduce subjectivity (10). However, we always performed confirmatory polyacrylamide gel electrophoresis with equivocal or inconclusive results for the high-resolution melt curve analysis and defaulted to the results of the gel if there were discrepancies in interpretation. Because a direct comparison between quantitative and subjective assessments was not made in the aforementioned study (10), it remains to be determined if quantitation is truly superior. It is intriguing that clonality in the CDR3 region of the B cell receptor was detected more frequently with primer set 2 than primer set 1. These primer sets differ with the primer that amplifies the junctional region of CDR3 (3), raising the possibility that this area of the B cell receptor shows more frequent clonal rearrangements than the junctional region amplified by primer set 1. Sequencing of relevant regions of the DNA in dogs with AML may prove informative and lead to the design of primers that are more specific for AML versus B cell neoplasms. However, this theory remains to be tested in future studies.
Dogs in this study were typically older, as reported for other studies of AML in dogs (29, 30), but AML can occur in dogs as young as 1–2 years (31). Similar to two other studies, German Shepherd dogs were one of the more common affected breeds (30, 31), which may represent a true breed disposition to AML or breed popularity. It is notable that several dogs presented with lymphadenopathy (peripheral or internal, solitary, or multiple nodes), organomegaly (spleen, liver, or both), or body cavity effusions, signs that are more typical for lymphoma than acute leukemia. Indeed, a presumptive diagnosis of lymphoid neoplasia was made before referral or after cytologic examination of body cavity fluid or tissue aspirates in six dogs (#8, 17, 20, 21, and 25). It was only after flow cytometric analysis or cytochemical staining was performed that the diagnosis was changed to AML. These results indicate that AML can mimic lymphoma in clinical presentation and, although less common than lymphoma, should still be considered as a differential diagnosis for animals presenting with lymphadenopathy or organomegaly, particularly if the organ enlargement is mild to moderate or if there are concurrent cytopenias or blasts identified in blood smears.
Most of the dogs in this study had bicytopenia or pancytopenia and an obvious leukemia with >20% blasts in peripheral blood. However, some dogs had a regenerative anemia, and one dog (#8) had a normal hemogram with no evidence of circulating tumor cells. The latter dog was diagnosed with a non-B non-T lymphoma before referral to our institution and had lower numbers of marrow blasts than is typical for dogs with AML, in which >70% blasts or marrow effacement is usually seen. Thus, a normal hemogram or regenerative anemia does not rule out a diagnosis of AML. In some cases, tumor cells in blood smears were presumptively identified as “lymphocytes” or as “lymphoblastic leukemia,” which may lead to an erroneous diagnosis of lymphoid neoplasia. It is difficult to impossible to determine if blasts are myeloid or lymphoid in origin on the basis of morphologic criteria alone, although features of myeloid differentiation can be apparent in some neoplastic cells and dysplasia in hematopoietic cell lineages can be additional support (but not confirmatory) for an underlying AML. Thus, at our institution, we identify immature neoplastic cells as generic “blasts” and rely on combined results from morphologic assessment, immunophenotyping, and cytochemical assays to distinguish between AML and lymphoproliferative disorders (16).
In contrast to human medicine (17, 18), there are currently no standardized criteria for diagnosis of AML in animals. In the past, the diagnosis of AML was based primarily on morphologic features and cytochemical staining (32). With the increasing availability of cross-reactive or canine-specific reagents for identification of lineage-associated antigens, immunophenotyping with flow cytometric analysis has largely supplanted cytochemical staining for diagnosis of AML (29, 30). A limitation of this approach is the lack of commercial reagents that can identify earlier myeloid-associated antigens, such as CD15, CD33, and CD123, in dogs, with most available antibodies being restricted to stem cell antigens and late stage differentiation antigens, such as CD14. For this reason, we used the presence of >20% blasts in blood or BM, body cavity fluid, or tissue aspirates and a combination of morphologic criteria, flow cytometric, and cytochemical results to confirm a diagnosis of AML in this study, as we have described previously (16). Dogs that did not have >20% blasts in blood had >20% blasts in marrow or aspirates of tissues or body cavity fluids. In this study, the combination of different tests was required for a diagnosis of AML, because the tumor cells in some dogs with AML displayed lineage infidelity or aberrant expression patterns and expressed B or T cell antigens, particularly CD5, on flow cytometric analysis, as we have previously reported (16). Aberrant expression of lymphoid antigens is also seen in human patients with AML (23–25, 33). In addition, positive cytochemical reactions for ALP or ANBE are not specific for AML, because these enzymes can be expressed in lymphoid tumors (16, 34, 35). Similarly, there have been rare reports of focal cytoplasmic reactivity for CAE in lymphocytes (35). For this reason, we used the preponderance of evidence, specifically the lack of or weak expression of lymphoid markers and MHCII with expression of myeloid-associated and stem cell antigens, and cytochemical staining more typical of myeloid cells (strong ALP, diffuse light or chunky ANBE, CAE) to make a diagnosis of AML over ALL or lymphoma. We have found that CD11b and strong ALP expression are particularly useful markers of AML, because lymphoid neoplasms are typically negative for CD11b and only weakly express or do not express ALP (16). However, neither of these markers are 100% specific for AML. Thus, it is still possible that some of cases in this study that lacked expression of myeloid-associated antigens but were diagnosed as AML on the basis of morphologic features and cytochemical staining patterns (#4 and #9) were in fact ALL. In five dogs, there were discrepant results from the flow cytometric analysis (#8) or cytochemical staining (#2, #5, #15, and #25) in samples from different sites. The converse was also true in four dogs, which had the same phenotype with flow cytometry (#5 and #10) or cytochemical staining (#8 and #17). Taken together, the results from the dogs with discrepant results between sites suggest that phenotyping, whether by flow cytometric analysis or cytochemical staining, may be more informative if done on all involved sites and not just one site. However, this is not always feasible and may be cost-prohibitive for some patients. It should be noted that there is a move toward standardization of flow cytometric testing for hematopoietic neoplasia in veterinary medicine (36). An ad hoc flow cytometric working group has also been established by clinical pathologists in the American Society of Veterinary Clinical Pathology. This group is working toward defining a minimum recommended flow cytometric panel of conjugated antibodies that can be used across institutions worldwide for diagnosis of hematopoietic neoplasia.
Due to the lack of genetic abnormalities that characterize newer AML classifications (17, 18), we used standard criteria to subtype the AML in this study, which included morphologic assessment and cytochemical staining reactions. These criteria were originally established by the French–American–British working group (21) and are now defined as AML – not otherwise specified by WHO (17, 18). Most of the dogs in this study (14/25, 56%) were subtyped as AML-M5 or monoblastic/monocytic leukemia, which is one of the more common subtypes in some reports of AML in dogs (31, 32). In contrast, another study of AML in dogs have found that myeloid leukemia with minimal differentiation (M0) is the most common subtype (30). However, the latter study used a limited set of markers to classify AML with flow cytometry and did not perform cytochemical staining. In some cases in this study, the classification of AML subtype was based primarily on strong positive cytochemical reactions for ALP (#2, #4, and #9), which we have found to be a useful marker of this subtype of AML. However, it is possible that ALP is a marker of less differentiated earlier myeloid precursors, and some of these cases could be AML with minimal maturation (M0), AML without maturation (M1), or AML with maturation (M2).
In conclusion, the results of our study indicate that clonal rearrangements in B and T cell receptors are a frequent finding in dogs diagnosed with AML. Thus, clonality testing should not be used a tool to discriminate between AML and lymphoid neoplasia (ALL or lymphoma). The current lack of a standardized scheme for diagnosis of AML in dogs creates substantial variability between published studies, making it difficult to compare across them. Establishing new consensus criteria for diagnosis of AML, by updating and incorporating new diagnostic testing modalities into the 1991 classification scheme (32), would be a worthwhile endeavor.
Author Contributions
TS conceived and designed the study, reviewed and interpreted the data, and wrote the manuscript. GN performed the clonality testing at Cornell University. MS performed and interpreted the cytochemical staining reactions. NB performed and interpreted the flow cytometric analysis. All authors critically reviewed and edited the manuscript.
Conflict of Interest Statement
The authors declare that the research was conducted in the absence of any commercial or financial relationships that could be construed as a potential conflict of interest.
Acknowledgments
The authors thank Drs. Avery and Herrara for provision of case #25 for this study. We also thank Drs. Dial and DeHeer for provision of cases of acute leukemia for consideration for inclusion (a confirmatory diagnosis of AML was not obtained for these cases and they were not included in the study). We thank Dr. Behling-Kelly for independent review and interpretation of some of the high resolution melt curves and polyacrylamide gels.
References
1. Keller SM, Vernau W, Moore PF. Clonality testing in veterinary medicine: a review with diagnostic guidelines. Vet Pathol (2016) 53(4):711–25. doi: 10.1177/0300985815626576
2. Avery AC. Molecular diagnostics of hematologic malignancies in small animals. Vet Clin North Am Small Anim Pract (2012) 42(1):97–110. doi:10.1016/j.cvsm.2011.11.001
3. Burnett RC, Vernau W, Modiano JF, Olver CS, Moore PF, Avery AC. Diagnosis of canine lymphoid neoplasia using clonal rearrangements of antigen receptor genes. Vet Pathol (2003) 40(1):32–41. doi:10.1354/vp.40-1-32
4. Vernau W, Moore PF. An immunophenotypic study of canine leukemias and preliminary assessment of clonality by polymerase chain reaction. Vet Immunol Immunopathol (1999) 69(2–4):145–64. doi:10.1016/S0165-2427(99)00051-3
5. Thalheim L, Williams LE, Borst LB, Fogle JE, Suter SE. Lymphoma immunophenotype of dogs determined by immunohistochemistry, flow cytometry, and polymerase chain reaction for antigen receptor rearrangements. J Vet Intern Med (2013) 27(6):1509–16. doi:10.1111/jvim.12185
6. Waugh EM, Gallagher A, Haining H, Johnston PE, Marchesi F, Jarrett RF, et al. Optimisation and validation of a PCR for antigen receptor rearrangement (PARR) assay to detect clonality in canine lymphoid malignancies. Vet Immunol Immunopathol (2016) 182:115–24. doi:10.1016/j.vetimm.2016.10.008
7. Gentilini F, Calzolari C, Turba ME, Bettini G, Famigli-Bergamini P. GeneScanning analysis of Ig/TCR gene rearrangements to detect clonality in canine lymphomas. Vet Immunol Immunopathol (2009) 127(1–2):47–56. doi:10.1016/j.vetimm.2008.09.014
8. Ohmura S, Leipig M, Schopper I, Hergt F, Weber K, Rutgen BC, et al. Detection of monoclonality in intestinal lymphoma with polymerase chain reaction for antigen receptor gene rearrangement analysis to differentiate from enteritis in dogs. Vet Comp Oncol (2017) 15(1):194–207. doi:10.1111/vco.12151
9. Fogle JE, Tarigo JL, Thalheim L, Williams LE, English LB, Suter SE. CD45+ and CD45− lymphocyte populations identified by flow cytometry from dogs with lymphoma exhibit similar morphology and the same clonal (B cell or T cell) lineage. Vet Immunol Immunopathol (2015) 168(3–4):242–8. doi:10.1016/j.vetimm.2015.10.004
10. Schopper I, Ohmura S, Rutgen B, Tsujimoto H, Weber K, Hirschberger J. Melting curve analysis in canine lymphoma by calculating maximum fluorescence decrease. Vet Comp Oncol (2016). doi:10.1111/vco.12200
11. Momoi Y, Nagase M, Okamoto Y, Okuda M, Sasaki N, Watari T, et al. Rearrangements of immunoglobulin and T-cell receptor genes in canine lymphoma/leukemia cells. J Vet Med Sci (1993) 55(5):775–80. doi:10.1292/jvms.55.775
12. Pate DO, Gilger BC, Suter SE, Clode AB. Diagnosis of intraocular lymphosarcoma in a dog by use of a polymerase chain reaction assay for antigen receptor rearrangement. J Am Vet Med Assoc (2011) 238(5):625–30. doi:10.2460/javma.238.5.625
13. Wilkerson MJ, Dolce K, Koopman T, Shuman W, Chun R, Garrett L, et al. Lineage differentiation of canine lymphoma/leukemias and aberrant expression of CD molecules. Vet Immunol Immunopathol (2005) 106(3–4):179–96. doi:10.1016/j.vetimm.2005.02.020
14. Tomiyasu H, Fujino Y, Takahashi M, Ohno K, Tsujimoto H. Spontaneous acute erythroblastic leukaemia (AML-M6Er) in a dog. J Small Anim Pract (2011) 52(8):445–7. doi:10.1111/j.1748-5827.2011.01096.x
15. Froment R, Bedard C. Marked hyperphosphatasemia associated with an acute leukemia in a Great Dane. Vet Clin Pathol (2016) 45(3):459–65. doi:10.1111/vcp.12389
16. Stokol T, Schaefer DM, Shuman M, Belcher N, Dong L. Alkaline phosphatase is a useful cytochemical marker for the diagnosis of acute myelomonocytic and monocytic leukemia in the dog. Vet Clin Pathol (2015) 44(1):79–93. doi:10.1111/vcp.12227
17. Swerdlow SH, Campo E, Harris NL, Jaffe ES, Stein H, Thiele J, et al. WHO Classification of Tumours of Haematopoietic and Lymphoid Tissues. 4th ed. Bosman FT, Jaffe ES, Lakhani SR, Ohgaki H, editors. Lyon, France: International Agency for Research on Cancer (2008).
18. Arber DA, Orazi A, Hasserjian R, Thiele J, Borowitz MJ, Le Beau MM, et al. The 2016 revision to the World Health Organization classification of myeloid neoplasms and acute leukemia. Blood (2016) 127(20):2391–405. doi:10.1182/blood-2016-03-643544
19. Erali M, Voelkerding KV, Wittwer CT. High resolution melting applications for clinical laboratory medicine. Exp Mol Pathol (2008) 85(1):50–8. doi:10.1016/j.yexmp.2008.03.012
20. Yagihara H, Uematsu Y, Koike A, Tamura K, Isotani M, Yamaguchi T, et al. Immunophenotyping and gene rearrangement analysis in dogs with lymphoproliferative disorders characterized by small-cell lymphocytosis. J Vet Diagn Invest (2009) 21(2):197–202. doi:10.1177/104063870902100203
21. Bennett JM, Catovsky D, Daniel MT, Flandrin G, Galton DA, Gralnick HR, et al. Proposed revised criteria for the classification of acute myeloid leukemia. A report of the French-American-British Cooperative Group. Ann Intern Med (1985) 103(4):620–5. doi:10.7326/0003-4819-103-3-460
22. Casares S, Rodriguez JM, Martin A, Parrado A. T-cell receptor gene rearrangements in lymphoid and non-lymphoid leukaemias. Eur J Clin Invest (1994) 24(2):119–25. doi:10.1111/j.1365-2362.1994.tb00976.x
23. Seremetis SV, Pelicci PG, Tabilio A, Ubriaco A, Grignani F, Cuttner J, et al. High frequency of clonal immunoglobulin or T cell receptor gene rearrangements in acute myelogenous leukemia expressing terminal deoxyribonucleotidyltransferase. J Exp Med (1987) 165(6):1703–12. doi:10.1084/jem.165.6.1703
24. Cheng GY, Minden MD, Toyonaga B, Mak TW, McCulloch EA. T cell receptor and immunoglobulin gene rearrangements in acute myeloblastic leukemia. J Exp Med (1986) 163(2):414–24. doi:10.1084/jem.163.2.414
25. Kyoda K, Nakamura S, Matano S, Ohtake S, Matsuda T. Prognostic significance of immunoglobulin heavy chain gene rearrangement in patients with acute myelogenous leukemia. Leukemia (1997) 11(6):803–6. doi:10.1038/sj.leu.2400662
26. Rao S, Lana S, Eickhoff J, Marcus E, Avery PR, Morley PS, et al. Class II major histocompatibility complex expression and cell size independently predict survival in canine B-cell lymphoma. J Vet Intern Med (2011) 25(5):1097–105. doi:10.1111/j.1939-1676.2011.0767.x
27. Avery PR, Burton J, Bromberek JL, Seelig DM, Elmslie R, Correa S, et al. Flow cytometric characterization and clinical outcome of CD4+ T-cell lymphoma in dogs: 67 cases. J Vet Intern Med (2014) 28(2):538–46. doi:10.1111/jvim.12304
28. Keller SM, Moore PF. A novel clonality assay for the assessment of canine T cell proliferations. Vet Immunol Immunopathol (2012) 145(1–2):410–9. doi:10.1016/j.vetimm.2011.12.019
29. Adam F, Villiers E, Watson S, Coyne K, Blackwood L. Clinical pathological and epidemiological assessment of morphologically and immunologically confirmed canine leukaemia. Vet Comp Oncol (2009) 7(3):181–95. doi:10.1111/j.1476-5829.2009.00189.x
30. Tasca S, Carli E, Caldin M, Menegazzo L, Furlanello T, Gallego LS. Hematologic abnormalities and flow cytometric immunophenotyping results in dogs with hematopoietic neoplasia: 210 cases (2002–2006). Vet Clin Pathol (2009) 38(1):2–12. doi:10.1111/j.1939-165X.2008.00099.x
31. Grindem CS, Stevens JB, Perman V. Morphologic classification and clinical and pathological characteristics of spontaneous leukemia in 17 dogs. J Am Anim Hosp Assoc (1985) 21:219–26.
32. Jain NC, Blue JT, Grindem CB, Harvey JW, Kociba GJ, Krehbiel JD, et al. Proposed criteria for classification of acute myeloid leukemia in dogs and cats. Vet Clin Pathol (1991) 20(3):63–82. doi:10.1111/j.1939-165X.1991.tb00571.x
33. Lewis RE, Cruse JM, Sanders CM, Webb RN, Suggs JL. Aberrant expression of T-cell markers in acute myeloid leukemia. Exp Mol Pathol (2007) 83(3):462–3. doi:10.1016/j.yexmp.2007.08.010
34. Raskin RE, Nipper MN. Cytochemical staining characteristics of lymph nodes from normal and lymphoma-affected dogs. Vet Clin Pathol (1992) 21(2):62–7. doi:10.1111/j.1939-165X.1992.tb00585.x
35. Raskin RE. Cytochemical staining. 6th ed. In: Weiss DJ, Wardrop KJ, editors. Schalm’s Veterinary Hematology. Ames, IA: Wiley-Blackwell (2010). p. 1141–56.
Keywords: acute myelogenous leukemia, canine, polymerase testing for antigen receptor rearrangements, clonality testing, phenotyping, leukemia, flow cytometry, cytochemistry
Citation: Stokol T, Nickerson GA, Shuman M and Belcher N (2017) Dogs with Acute Myeloid Leukemia Have Clonal Rearrangements in T and B Cell Receptors. Front. Vet. Sci. 4:76. doi: 10.3389/fvets.2017.00076
Received: 15 March 2017; Accepted: 03 May 2017;
Published: 31 May 2017
Edited by:
Stefano Comazzi, Università degli Studi di Milano, ItalyReviewed by:
Barbara C. Rütgen, VetmeduniVienna, AustriaMaria Elena Gelain, University of Padua, Italy
Copyright: © 2017 Stokol, Nickerson, Shuman and Belcher. This is an open-access article distributed under the terms of the Creative Commons Attribution License (CC BY). The use, distribution or reproduction in other forums is permitted, provided the original author(s) or licensor are credited and that the original publication in this journal is cited, in accordance with accepted academic practice. No use, distribution or reproduction is permitted which does not comply with these terms.
*Correspondence: Tracy Stokol, tracy.stokol@cornell.edu