- 1Department of Poultry Science, University of Georgia, Athens, GA, United States
- 2St. Boniface Hospital Research Centre, Winnipeg, MB, Canada
We investigated how the microbiota in the ileum and cecum of broiler chickens fed a diet of low calcium (Ca) and available phosphorus (aP) and prebiotic fructooligosaccharides (FOS) supplements changed over a 3 weeks period. Three dietary treatments were randomly assigned to four replicate cages of five birds each, including: positive control (PC), a wheat-corn-soybean meal-based diet; negative control (NC), as PC with 0.2% reduced Ca and aP; and NC + FOS, as NC supplemented with 0.5% of FOS. Ileal and cecal digesta were sampled from each replicate (n = 4) on d21 and processed for 16S rRNA gene amplicon (V4 region) sequencing using Illumina platform. Statistical differences were observed in the microbiome by GI location as determined by 2-way ANOVA and Permutational MANOVA. On average, 24,216 sequence reads per sample were generated resulting in 800 and 1,280 operational taxonomic units in the ileal and cecal digesta, respectively. Difference (P < 0.0001) on alpha diversity and abundances of several phyla was observed between ileal and cecal digesta. ß-diversity was different (P < 0.05) between each treatment groups in the ileum but not in the cecum. In the cecum, species richness, phylogenetic diversity, and the number of observed species were higher in PC compared to NC + FOS (P < 0.05). Several phyla, including Cyanobacteria, Firmicutes, and Proteobacteria, had significantly different abundance in the ileal and cecal digesta (P < 0.05). In the ileal digesta, positive correlation were observed between Salinibacterium and Lysobacter and PC diet. Blautia, Faecalibacterium and Pseudomonas and the NC diet and Lactobacillus and Escherichia and the NC + FOS diet. In the cecal digesta, Butyrivibrio, and Allobaculum were positively correlated to PC. Although, Clostridium and Anaerotruncus were positively correlated to NC + FOS, they showed negative correlation to PC and NC. The study concludes that dietary Ca and aP level and FOS supplementation alters ileal microbiota of the broiler chickens.
Introduction
Phosphorus (P) is an essential macro-element in broiler diets and is responsible for multitude of physiological processes including skeletal system development, growth, and productivity of birds. Plant-based diets contain large amounts of unavailable P, which is partially hydrolyzed by the monogastric animals including broiler chickens (1). Therefore, in a standard poultry diet formulation inorganic P supplementation is required to ensure that the available P (aP) meets the requirements for poultry. Changes in the Ca:aP ratio of the broilers diet can affect physio-chemical properties of the digesta in the gastro-intestinal tract of the broilers (2). Increase of the crop (4.89–5.32) and ileum (6.62–7.39) pH by increasing dietary Ca from 10.7 to 25.3 g/Kg was reported by Shafey et al. (3). A negative correlation between broiler performance and the increased pH of the small intestine, by increasing of Ca level in the diet was reported by McDonald and Solvyns (4).
Fructooligosaccharides (FOS), obtained from plants, are non-digestible carbohydrates known to have prebiotic properties (5, 6). Several studies reported that supplementation of FOS has resulted in increased body weight and decreased feed conversion ratio of the birds (7–9). However, performance can be affected by variations in the levels of FOS supplementation. To date, no well-defined recommendation of dietary FOS supplementation is available for poultry. Apart from enhancing the bacterial fermentation in the intestine (10–12), there are also increasing evidence on the potential ability of FOS to increase the bioavailability of minerals. According to Xu et al. (7), 0.4% of FOS in a diet had substantial positive effects on intestinal morphology which might resulted in improved mineral absorption in broilers. Several studies have also proved that supplementation of FOS enhanced the growth of bacterial with probiotic properties (resistance to gastric pH and bile, adherence to mucus/epithelial cells) such as Bifidobacteria and Lactobacilli. These probiotic bacteria produce short-chain fatty acids which result in a lower pH of the gastrointestinal tract [GIT; (13, 14)]. A lower pH has been reported to be favorable for mineral solubility (15, 16). Furthermore, changes in the pH of GIT may result in shifts of the microbiota and their functional attributes. A lower pH in the GIT, due to its bacteriostatic effect, should have negative effect on the population of foodborne pathogens like Salmonella, Campylobacter, and Escherichia coli, while maintaining the integrity of the intestinal membrane (17). Hence, we were intrigued about microbial community dynamics in gastro intestinal tract of broilers in response to FOS supplemented diet containing reduced Ca and P levels. As very little data are available on the effect of low calcium, phosphorus and FOS supplementation on the microbiome of the host. Therefore, the aim of the present study was to investigate effects of dietary Ca and aP level and FOS supplementation on microbiota in the ileum and cecum segments of the broilers.
Materials and Methods
Birds and Housing
A total of 60, 1-day old, male Ross × Ross 308 chicks were obtained from a commercial hatchery (Carltons Hatchery, Grunthal, Manitoba, Canada). The chicks were housed in electrically heated Jamesway battery brooders (James Mfg. Co., Mount Joy, PA) for the first 4 days of pre-experimental period. The temperature during pre-experimental period was 32°C. On d 5, chicks were individually weighed and sorted into five weight classes. Groups of five birds, one from each weight class, were then randomly assigned to 12 battery pens such that the average initial BW was similar across pens. The chickens were raised for 21 d. During the experimental period, birds were housed in three electrically-heated Alternative Design Super Brooders (Alternative Design Manufacturing and Supply, Inc., Siloam Springs, AR) under a controlled environment. The temperature was monitored daily and was gradually reduced until a temperature of 24°C was reached on d 21. Light was provided for 24 h throughout the experimental period.
Dietary Treatments
Three dietary treatments were randomly assigned to four replicate cages of five birds each. Composition and analyzed nutrient values of the experiment diets are shown in Supplementary Table 1. The dietary treatments include: a positive control (PC), a wheat-corn-soybean meal-based diet contained adequate levels of Ca and available P (aP); a negative control (NC), as PC with 0.2% reduced Ca and aP; and a NC + FOS, as NC supplemented with 0.5% of FOS. The PC diet was fed to all the chickens for the first 4 d adaption period, and the experimental diets were provided from d 5–21. Water and feed were offered ad libitum. The basal diet was formulated to meet or exceed the National Research Council nutrient requirements for broiler chickens (18).
Sample Collection and DNA Extraction
On d 21, a total of 12 birds (one bird from each pen; four birds per treatment) were randomly selected and euthanized by cervical dislocation. Ileal and cecal samples were collected in sterile bags and snap frozen in liquid nitrogen and later stored at −80°C till further processing. The archived ileal and cecal samples were thawed and subjected to DNA extraction using ZR fecal DNA MiniPrep Kit (ZYMO Research, Irvine, CA). Before following the kit protocol, microbial cells were subjected to bead beating using Mini-BeadBeater-16 (Bio Spec Products, Bartlesville, OK) for 2 min. Extracted DNA was the quantified using a NanoDrop 2000 spectrophotometer (NanoDrop, Wilmington, DE, USA). DNA samples were normalized to 20 ng/μL, and quality was checked by PCR amplification of the 16S rRNA gene using universal primers 27F (5′ -GAAGAGTTTGATCATGGCTCAG-3′) and 342R (5′ -CTGCTGCCTCCCGTAG-3′) as described by Khafipour et al. (19). Amplicons were verified by running on 1.5% agarose gel.
Bioinformatics Analyses
Bioinformatic analyses were performed as described by Derakhshani et al. (20). In brief, the PANDAseq assembler (21) was used to merge overlapping paired-end Illumina fastq files. All the sequences with mismatches or ambiguous calls in the overlapping region were discarded. The output fastq file was then analyzed by downstream computational pipelines of the open source software package Quantitative Insights into Microbial Ecology [QIIME, (22)]. Assembled reads were demultiplexed according to the barcode sequences and exposed to additional quality-filters so that reads with more than 3 consecutive bases with quality scores below 1e−5 were truncated, and those with a read length shorter than 75 bases were removed from the downstream analysis. Chimeric reads were filtered using UCHIME (23) and sequences were assigned to Operational Taxonomic Units (OTU) using the QIIME implementation of UCLUST (24) at 97% pairwise identity threshold. Taxonomies were assigned to the representative sequence of each OTU using RDP classifier (25) and aligned with the Greengenes Core reference database (26) using PyNAST algorithms (27). The OTUs that classified to kingdom Archaea were removed from downstream analysis. Venn diagrams [VENNY; (28)] were produced based on classified and unclassified genera obtained from the Greengenes Core reference database, demonstrating the number of shared and unique genera across the PC, NC, and NC + FOS dietary treatments.
Within community diversity (α-diversity) was calculated based on OTU counts using QIIME to evaluate the biodiversity of the bacterial population at the genus level. Alpha rarefaction curve was generated using Chao 1 estimator of species richness (29).
Statistical Analysis
The α-diversity, major phylum, and genus percentage data from the microbiome sequencing were also analyzed using the two-way ANOVA of GLM procedure of SAS 9.2 (30), based on the dietary treatments and the two GIT samples (ileum and cecum). To compare microbial composition between samples and among different dietary treatments, β-diversity was measured by calculating the weighted and unweighted Unifrac distances (31) using QIIME default scripts. Principal coordinate analysis (PCoA) was applied on resulting distance matrices to generate two-dimensional plots using PRIMER v6 software [(32), PRIMER-E Ltd, Plymouth]. Permutational multivariate analysis of variance [PERMANOVA, (33)] was used to calculate P-values and test for significant differences of β-diversity among treatment groups.
All phyla were divided into two groups of abundance, high-abundance (≥1.0 %), and low-abundance (<1.0 %) of the population. Differences between groups were considered significant at P < 0.05, and trends were considered at P < 0.10. Outliers were examined and removed using Grubbs' test at α < 0.05 (34). Statistical analyses for microbial sequences were performed as described by Li et al. (33) and Derakhshani et al. (20). In brief, partial least square discriminant analysis (PLS-DA; SIMCA P+ 13.0, Umetrics, Umea, Sweden) was performed on bacterial genera to identify the effects of dietary treatments. The PLS-DA is a case of partial least square regression analysis in which Y is a set of variables describing the categories of a predictor variable on X (35). In this study, X variables were bacterial taxa and Y variables were observations that belong to different dietary groups (PC, NC, or NC + FOS). For this analysis, genera which have a population lower than 0.002% were trimmed, and data were scaled using Unit Variance in SIMCA. Cross-validation was performed to determine the number of significant PLS components, and a permutation testing was conducted to validate the model. To avoid over parameterization of the model, variable influence on projection value (VIP) was estimated for each genus and genera with VIP < 0.50 were removed from the final model (35, 36).
Results
Sequencing Information
A total of 5,81,187 Sequences were obtained after quality filtering and chimeras checking. The minimum sequence reads were 17,411 and 14,872 for cecum and ileum samples, respectively. One ileal digesta sample from each NC and NC + FOS was identified as outlier and omitted for downstream microbial diversity and composition analyses. On average, 24,216 sequence reads per sample were generated resulting in 800 and 1,280 operational taxonomic units in the ileum and cecum digesta, respectively.
Species Richness and Diversity
Alpha diversity indices showed difference (P < 0.0001) between ileal and cecal microbiota, however, no change in α-diversity was observed in response to diet or site × diet interaction (Table 1) in both ileum and cecum digesta samples.
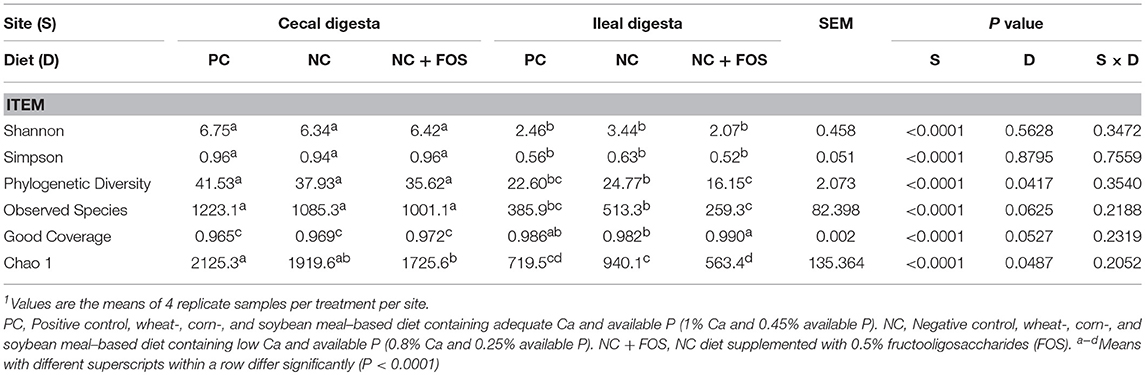
Table 1. Bacterial alpha diversity based on the main effects of diet and GIT sections (ileum and cecum) of broiler chickens at 21 days of age1.
The ileal digesta of NC group showed numerically higher species richness and observed species compared to PC and NC + FOS groups (Table 1). The Principal coordinate analysis (PCoA) plot (Figure 1) analyzed using Permanova for Unifrac weighted β-diversity showed no difference (P > 0.05) in the ileal digesta of each treatment group. However, unweighted analysis showed difference (P < 0.05) between each treatment group. In the cecal digesta, the observed species and species richness (not shown) were numerically higher in PC group compared to NC and NC + FOS groups. The PCoA plot (Figure 2) analyzed using Permanova for both Unifrac weighted and unweighted β-diversity demonstrated no difference (P > 0.05) in cecal digesta samples in each treatment group.
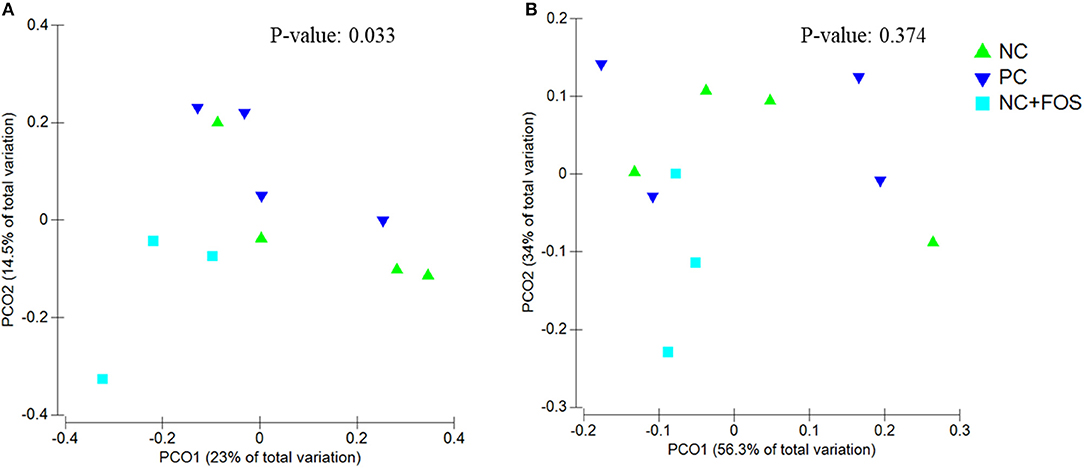
Figure 1. Principal coordinates analysis (PCoA) of (A) unweighted Unifrac (P = 0.033) and (B) weighted Unifrac (P = 0.374) distance of ileal digesta bacterial community between the chickens that fed PC, NC, and NC + FOS diets1 (n = 4/treatment). PC: Positive control, wheat-, corn-, and soybean meal–based diet containing adequate Ca and available P (1% Ca and 0.45% available P). NC: Negative control, wheat-, corn-, and soybean meal–based diet containing low Ca and available P (0.8% Ca and 0.25% available P). NC + FOS, NC diet supplemented with 0.5% fructooligosaccharides (FOS).
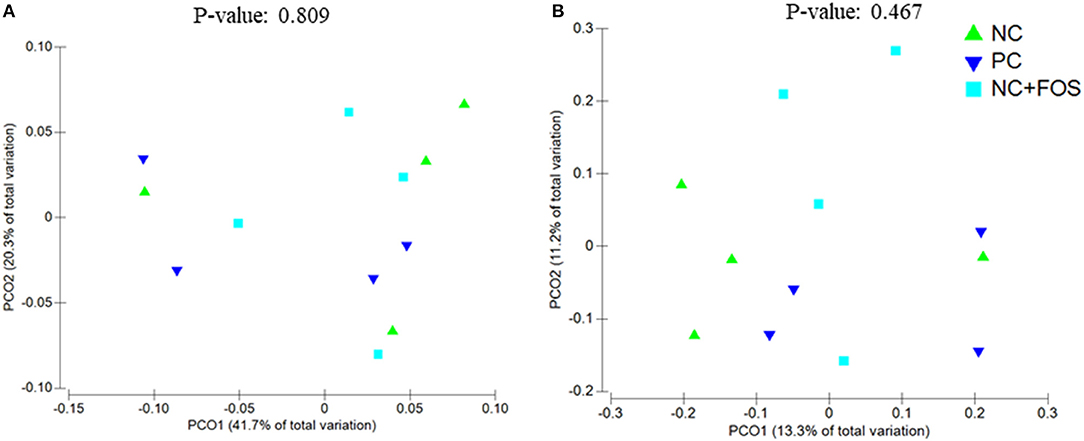
Figure 2. Principal coordinates analysis (PCoA) of (A) unweighted Unifrac (P = 0.467) and (B) weighted Unifrac (P = 0.809) distance of cecal digesta bacterial community between the chickens that fed PC, NC, and NC + FOS diets1 (n = 4/treatment). Refer to Figure 1 legends.
Phylogenetic Diversity
Taxonomic assignment of OTUs identified 13 phyla in the ileal digesta and 9 in the cecal digesta of the broiler chickens. Six phyla with relative abundance >0.1% are presented in Table 2. The relative abundance of most of the phyla did not differ (P > 0.05) in the ileum and cecum in response to diet and interaction between site and diet. However, relative abundance of phyla (Cyanobacteria, Firmicutes, and Proteobacteria) did change (P < 0.05) in the ileum and cecum digesta. Firmicutes had higher abundance in the cecum digesta (97%), whereas Proteobacteria (11%) and Cyanobacteria (9%) showed higher abundance in the ileum digesta. Although no difference (P > 0.05) in relative Firmicutes abundance was observed in the ileal digesta in different treatment groups, Firmicutes were numerically higher in abundance in the NC + FOS group followed by the NC and PC groups, whereas Proteobacteria was lower (P < 0.05) in the NC + FOS group.
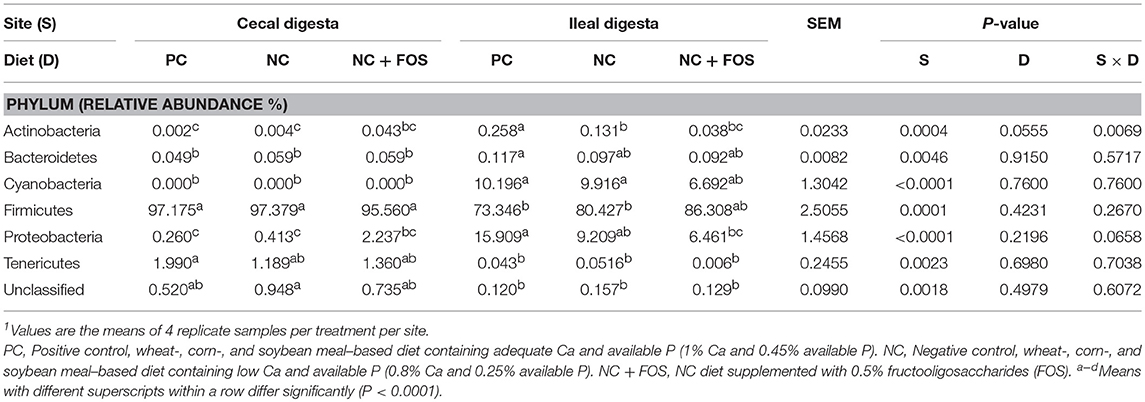
Table 2. Relative abundance of bacterial phyla based on the main effects of diet and GIT sections (ileum and cecum) of broiler chickens at 21 days of age1.
Dietary Effect on Ileal Microbiota
In the ileal digesta of broiler chickens, there were 75 bacterial taxa shared across the three dietary treatments (PC, NC, and NC + FOS). Eight genera were unique to NC + FOS group, whereas 28 and 18 genera were unique to PC and NC groups, respectively (Supplementary Figure 1). The taxa shared between all the three groups include Salinibacterium and Aurantimonadaceae (f). Clostridia were found in the PC and NC groups but not observed in the NC +FOS group. As shown on the PLS-DA loading plot (Figure 3), the genus Salinibacterium and Lysobacter were positively correlated to the ileal digesta of broiler chickens fed PC diet. Comparison between the NC and NC + FOS groups in the ileal digesta by PLS-DA (Figure 3) indicated that Clostridia, Blautia, Faecalibacterium, and Pseudomonas were associated with the NC group, whereas Escherichia, Lactobacillus, Prevotella were positively correlated to the NC + FOS group.
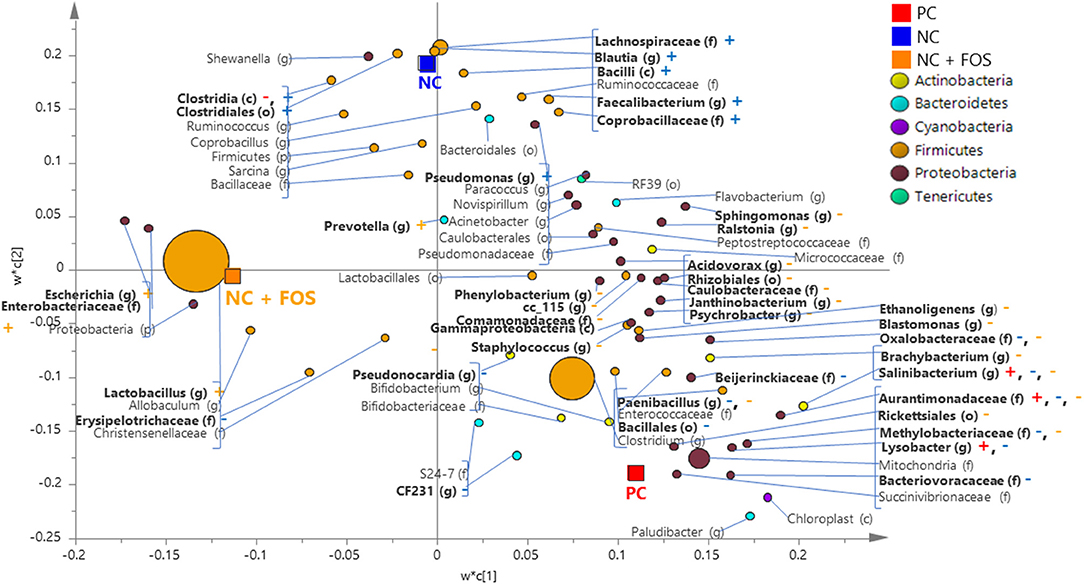
Figure 3. Partial least square discriminant analysis (PLS-DA) loading plot based on the relative abundance of bacterial taxa in the ileal digesta of broiler chickens that fed PC, NC, and NC + FOS diets1 (n = 4/treatment). The presenting taxa are chosen at variable influence on projection (VIP) value of above 0.6. The size of each circle indicates the abundance of taxa and is colored according to their corresponding phyla. The taxa are presented on phylum (p), class (c), order (o), family (f), or genus (g) levels after comparison of sequences to the Greengenes Core reference database. The colored “+” and “–” indicates positive or negative correlation of taxa to the same colored PC, NC, or NC + FOS dietary group. Refer to Figure 1 legends.
Dietary Effect on Cecal Digesta Microbiota
In the cecal digesta of broiler chickens, 65 bacterial taxa were shared among the three dietary treatments as shown in Supplementary Figure 2. Seven genera, such as Paludibacter, Clostridium, Blautia, Coprococcus, Coprobacillus, Ethanoligenes, and Oscillospira, were unique to the NC + FOS group. The PLS-DA comparison (at cut-off VIP value of 0.5) between PC and NC + FOS groups in the cecal digesta of broiler chickens (Figure 4) showed that members of Peptostreptococcaceae (f), Clostridiales (o) and genus Butyrivibrio and Allobaculum were positively associated with PC, whereas Blautia and Ruminococcus (g) exhibited positive association with NC + FOS.
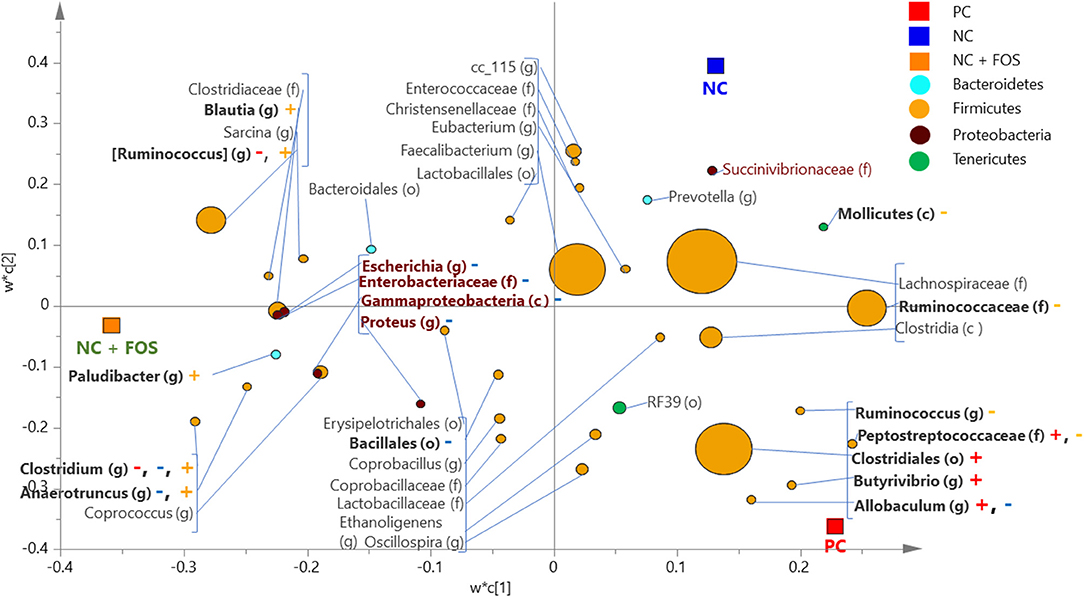
Figure 4. Partial least square discriminant analysis (PLS-DA) loading plot based on the relative abundance of bacterial taxa in the cecal digesta of broiler chickens that fed PC and NC + FOS diets1 (n = 4/treatment). The presenting taxa are chosen at variable influence on projection (VIP) value of above 0.5. The size of each circle indicates the abundance of taxa and is colored according to their corresponding phyla. The taxa are presented on phylum (p), class (c), order (o), family (f), or genus (g) levels after comparison of sequences to the Greengenes Core reference database. The colored “+” and “–” indicates positive or negative correlation of taxa to the same colored PC or NC + FOS dietary group. Refer to Figure 1 legends.
Discussion
Low Ca and aP level with and without FOS supplementation could potentially affect the structure of the microbial community in the digestive tract. In this study, cecal and ileal digesta were analyzed to evaluate the difference of microbial community in response to low Ca, aP, and FOS supplemented diets. The ileum and cecum sections contain diverse microbial populations that are responsible for degrading complex organic molecules to short chain fatty acids (SCFAs), such as the feed-based phytate degrading activities (37–40). On the other hand, the cecum has greater species richness and diversity compared to the ileum, and this complexity increases as the chicks advances in age (41–46).
Our findings were in accordance with previous reports that the cecum had greater species richness compared to the ileum (47, 48). However, no difference in diversity was observed with a low Ca and aP and/ or FOS dietary supplementation. Any dietary changes remarkably affect the richness of microbial community in the ceca compared to ilea (with shorter transit time for digesta, 3.5 h). Increased abundance of Clostridia in chicken ileal (PC and NC diets) and cecal digesta indicates a healthy chicken gut as members of Clostridial group plays an important role in SCFA metabolism (40). In addition to immunomodulatory and nutritional functions, SCFA are known for mineral absorption and inhibition of pathogenic microbes by reducing pH (49). Furthermore, genus Clostridium is associated with the production of cysteine phytase, one of the four distinct classes of phytate-degrading phytases. Although phytases are structurally different but they all have similar functions (50). Abundance of Clostridium in the ileum and cecum may increase the endogenous bacterial phytase production and thus potentially help improving the P availability, mineral absorption, ileal amino-acid digestibility, and thus can improve bird performance. However, their role depends on the levels of P and Ca in the diet.
Difference in α-diversity indices and phylogenetic community between the ileal and cecal digesta confirmed the results of Borda-Molina and coworkers (51) who reported greater distinction (p = 0.001) in bacterial community between the ileum and cecum samples, regardless of the diet. Similar results were also obtained by Stanley et al. (52) and Witzig et al. (53). The difference in microbial diversity of the cecal and ileal digesta can be attributed to many factors such as physiochemical conditions, passage rate of the digesta, pH, presence and absence of small and soluble particles. These conditions help in the establishment of complex microbiota and enhance their role in nutrient assimilation, vitamins, and amino acids production (54, 55), and pathogen elimination (52). Most of the colonized microorganisms in the two GIT sections belonged to phylum Firmicutes, which have been commonly described in earlier reports of the chicken GIT (38, 41, 51, 56, 57). The microbial community differences observed in the ileum of all three dietary groups (PC, NC, and NC + FOS) were in agreement with earlier study (51). It has been reported that low doses of Ca in the diets (NC and NC + FOS) can lead to a decrease in pH (2) and enhance the pre-cecal digestibility (58, 59) which lead to positive association of lactobacilli in the ileum (2, 51). Moreover, increased availability of P could increase the abundance of beneficial microbes such as Blautia and Faecalibacterium, which agrees with the results of Borda-Molina et al. (51). Genus Faecalibacterium, is known for its function of epithelial health enhancement, by increasing duodenal villus/crypt ratio (60), and for butyrate production (61). In addition, Faecalibacterium regulates inflammatory gene expressions and apoptosis in host cells (62). Blautia, a member of family Lachnospiraceae (63) and it constitutes one of the major taxonomic groups of the human/rumen/chicken gut microbiota where they are associated with corn-based diet and can degrade complex polysaccharides for energy utilization by the host (64, 65).
Supplementation of FOS along with the NC diet exhibited positive association with the genus Lactobacillus and Prevotella in the ileum. Earlier studies in chickens, mice and pigs have also shown that diets supplemented with P increased the Lactobacillus abundance (51, 57, 66–68). Further supplementation of dietary FOS has been known to selectively supporting the growth of Lactobacillus. Lactobacilli produces extracellular enzymes to degrade FOS (69) which results in an increase in SCFAs and lactate production, which could further enhance the immune system (70–72). Additionally, it has also been reported that a high availability of FOS could also be associated with the oligosaccharide transport system of the Lactobacillus species (69, 73, 74). Some gut microbes such as Prevotella also have prebiotic degrading capability, as shown in our study (69). Genus Prevotella are known for their oligosaccharides degrading property. Additionally, several species of Prevotella possess dipeptidyl peptidase type IV which is important for initial dietary protein catabolic activity (75, 76). These mechanisms positively correlate the abundance of Prevotella in wheat-corn based diets, as evident in this study. In a recent study, Poeker et al. (77) also demonstrated that fermentable dietary fibers can induce growth and/ or activity of specific beneficial populations. The abundance of Prevotella and Lactobacillus in NC + FOS fed broilers again indicates healthy gut with higher SCFA production and improved mineral absorption. Although PC fed groups showed presence of healthy bacteria, but to understand the abundance of Lysobacter and Salinibacterium and their attributed function in PC fed group. These identified functional attributes are descriptive in nature, therefore it is unclear if the modulated bacteria confer benefits to the host, if they are artifacts or if they are markers of a modulated gut bacteria that helps in well-being of the host. This study was limited to chicken cecum and ileum, future work should also include locations in the upper GI tract to determine the microbial changes that occur there, since they are also likely important in the overall health and performance of the poultry. Nevertheless, this study provides a more comprehensive glimpse at the gut microbial modulations in response to low Ca and P in broilers diet and provides future targets and markers while using alternative approaches with FOS and Ca and P levels in diets.
In conclusion, a low dietary Ca and aP level with or without FOS supplementation could influence the microbial community of the chicken gut. Diets supplemented with a low Ca and aP, affected the ileum microbiota, whereas the FOS supplementation led to an increased abundance of beneficial microbes such as Lactobacillus, Faecalibacterium, Blautia, Prevotella etc. Increased abundance of Clostridium in the cecum may be an evidence that common core members of the gut microbiota also have the ability to produce phytase which can further improve the availability of P in the chicken diet. However, to better understand the effect of low Ca, aP diet and FOS supplementation on the growth performance and gut health of broiler chickens, further mucosal microbial community studies are warranted. Moreover, in order to overcome the restrictions of 16S rRNA based microbial community analysis, metaproteomics, and transcriptomics are needed to determine the metabolic and functional properties of the chicken gut microbiota.
Ethics Statement
The study was conducted out in strict accordance with the guidelines established by the Canadian Council on Animal Care (78). All the experimental procedures were approved by the University of Manitoba Animal Care Protocol Management and Review Committee.
Author Contributions
WK brought research idea, designed the study, managed the project, and contributed to data interpretation and manuscript writing. SK analyzed the data, interpreted the data, and wrote the manuscript. YS conducted the study, collected the data, analyzed the data, and contributed to data interpretation and manuscript writing.
Conflict of Interest Statement
The authors declare that the research was conducted in the absence of any commercial or financial relationships that could be construed as a potential conflict of interest.
Supplementary Material
The Supplementary Material for this article can be found online at: https://www.frontiersin.org/articles/10.3389/fvets.2019.00095/full#supplementary-material
Supplementary Figure 1. Venn diagram of shared and unique bacterial taxa in ileal digesta of broiler chickens fed PC, NC, and NC + FOS diet1 (n = 4/treatment). See Figure 1 legends.
Supplementary Figure 2. Venn diagram of shared and unique bacterial taxa in cecal digesta of broiler chickens fed PC, NC, and NC + FOS diet1 (n = 4/treatment). See Figure 1 legends.
Supplementary Table 1. Composition of experimental diets (as-fed basis).
References
1. Ravindran V, Bryden WL, Kornegay ET. Phytates: occurrence, and implications in poultry nutrition. Poult Avian Biol Rev. (1995) 6:125–143.
2. Ptak A, Bedford MR, Swiatkiewicz S, Zyła K, Józefiak D. Phytase modulates ileal microbiota and enhances growth performance of the broiler chickens. PLoS ONE. (2015) 10:e0119770. doi: 10.1371/journal.pone.0119770
3. Shafey TM, McDonald MW, Dingle JG. Effect of dietary Ca and available phosphorus on digesta pH and on the availability of Ca, iron, magnesium and zinc content from the intestinal of meat chicken. Br J Poult Sci. (1991) 32:185–94.
4. McDonald MW, Solvyns A. Dietary calcium levels and chicken growth. In: Australasian Poultry Science Convention. Australian Branch, WPSA. Proceedings of the Australasian Poultry Science Convention. Surfers Paradise, QLd: Merch Sharp and Dhome (1964) p. 112–6.
5. Williams J, Mallet S, Leconte M, Lessire M, Gabriel I. The effects of fructo-oligosaccharides or whole wheat on the performance and digestive tract of broiler chickens. Br Poult Sci. (2008) 49:329–39. doi: 10.1080/00071660802123351
6. Kim GB, Seo YM, Kim CH, Paik IK. Effect of dietary prebiotic supplementation on the performance, intestinal microflora, and immune response of broilers. Poult Sci. (2011) 90:75–82. doi: 10.3382/ps.2010-00732
7. Xu ZR, Hu CH, Xia MS, Zhan XA, Wang MQ. Effects of dietary fructooligosaccharide on digestive enzyme activities, intestinal microflora and morphology of male broilers. Poult Sci. (2003) 82:1030–6. doi: 10.1093/ps/82.6.1030
8. Bailey JS, Blankenship LC, Cox NA. Effect of fructooligosaccharide on Salmonella colonization of the chicken intestine. Poult Sci. (1991) 70:2433–8. doi: 10.3382/ps.0702433
9. Ammerman E, Quarles C, Twining PV. Broiler response to the addition of dietary fructooligosaccharides. Poult Sci. (1988) 67:46.
10. Gudiel-Urabano M, Goni I. Effect of fructooligosaccharides on nutritional parameters and mineral bioavailability in rats. J Sci Food Agri. (2002) 82:913–7. doi: 10.1002/jsfa.1133
11. Zafar TA, Weaver CM, Zhao Y, Martin BR, Wastney ME. Nondigestible oligosaccharides increase calcium absorption and suppress bone resorption in ovariectomized rats. J Nutr. (2004) 134:399–402. doi: 10.1093/jn/134.2.399
12. Ohta A. Prevention of osteoporosis by foods and dietary supplements: the effect of fructooligosaccharides (FOS) on the calcium absorption and bone. Clin Calcium. (2006) 16:1639–45.
13. Wang Y, Zeng T, Wang SE, Wang W, Wang Q, Yu HX. Fructo-oligosaccharides enhance the mineral absorption and counteract the adverse effects of phytic acid in mice. Nutrition. (2010) 26:305–11. doi: 10.1016/j.nut.2009.04.014
14. Bogusławska-Tryk M, Piotrowska A, Burlikowska K. Dietary fructans and their potential beneficial influence on health and performance parameters in broiler chickens. J Cent Euro Agri. (2012) 13:272–91. doi: 10.5513/JCEA01/13.2.1045
15. Selle PH, Cowieson AJ, Ravindran V. Consequences of calcium interactions with phytate and phytase for poultry and pigs. Livest Sci. (2009) 124:126–41. doi: 10.1016/j.livsci.2009.01.006
16. Walk CL, Bedford MR, McElroy AP. Influence of limestone and phytase on broiler performance, gastrointestinal pH, and apparent ileal nutrient digestibility. Poult Sci. (2012) 91:1371–8. doi: 10.3382/ps.2011-01928
17. Perry GC. Avian Gut Function in Health and Disease—Poultry Science Symposium Series. Wallingford: CABI (2006) p. 124–55.
18. NRC. Nutrient Requirements of Poultry. 9th Edn. Washington, DC: National Academies Press (1994).
19. Khafipour E, Li S, Plaizier JC, Krause DO. Rumen microbiome composition determined using two nutritional models of subacute ruminal acidosis. Appl Environ Microbiol. (2009) 75:7115–24. doi: 10.1128/AEM.00739-09
20. Derakhshani H, Tun HM, Cardoso FC, Plaizer JC, Khafipour E, Loor JJ. Linking peripartal dynamics of ruminal microbiota to dietary changes and production parameters. Front Microbiol. (2016) 7:2143. doi: 10.3389/fmicb.2016.02143
21. Masella A, Bartram A, Truszkowski J, Brown D, Neufeld J. PANDAseq: paired-end assembler for illumina sequences. BMC Bioinformatics. (2012) 13:31. doi: 10.1186/1471-2105-13-31
22. Caporaso JG, Kuczynski J, Stombaugh J, Bittinger K, Bushman FD, Costello EK, et al. QIIME allows analysis of high-throughput community sequencing data. Nat Methods. (2010) 7:335–6. doi: 10.1038/nmeth.f.303
23. Edgar RC, Haas BJ, Clemente JC, Quince C, Knight R. UCHIME improves sensitivity and speed of chimera detection. Bioinformatics. (2011) 27:2194–200. doi: 10.1093/bioinformatics/btr381
24. Edgar RC. Search and clustering orders of magnitude faster than Blast. Bioinformatics. (2010) 26:2460–1. doi: 10.1093/bioinformatics/btq461
25. Wang Q, Garrity GM, Tiedje JM, Cole JR. Naive bayesian classifier for rapid assignment of rRNA sequences into the new bacterial taxonomy. Appl Environ Microbiol. (2007) 73:5261–7. doi: 10.1128/AEM.00062-07
26. DeSantis TZ, Hugenholtz P, Larsen N, Rojas M, Brodie EL, Keller K, et al. Green genes, a chimera-checked 16S rRNA gene database and workbench compatible with ARB. Appl Environ Microbiol. (2006) 72:5069–72. doi: 10.1128/AEM.03006-05
27. Caporaso JG, Bittinger K, Bushman FD, DeSantis TZ, Andersen GL, Knight R. PyNAST: a flexible tool for aligning sequences to a template alignment. Bioinformatics. (2010) 26:266–7. doi: 10.1093/bioinformatics/btp636
28. Oliveros JC. VENNY: An Interactive Tool for Comparing Lists With Venn Diagrams. (2007) Available online at: http://bioinfogp.cnb.csic.es/tools/venny/index.html
29. Chao A. Nonparametric estimation of the number of classes in a population. Scand J Stat. (1984) 11:265–70.
31. Lozupone C, Knight R. UniFrac: a new phylogenetic method for comparing microbial communities. Appl Environ Microbiol. (2005) 71:8228–35. doi: 10.1128/AEM.71.12.8228-8235.2005
33. Li R, Khafipour E, Krause DO, Entz MH, de Kievit TR, Fernando WD. Pyrosequencing reveals the influence of organic and conventional farming systems on bacterial communities. PLoS ONE. (2012) 7:e51897. doi: 10.1371/journal.pone.0051897
34. Grubbs FE. Procedures for detecting outlying observations in samples. Technometrics. (1969) 11:1–21. doi: 10.1080/00401706.1969.10490657
35. P'erez-Enciso M, Tenenhaus M. Prediction of clinical outcome with microarray data: a partial least squares discriminate analysis (PLS-DA) approach. Hum Genet. (2003) 112:581–92. doi: 10.1007/s00439-003-0921-9
36. Verhulst NO, Qiu YT, Beijleveld H, Maliepaard C, Knights D, Schulz S, et al. Composition of human skin microbiota affects attractiveness to malaria mosquitoes. PLoS ONE. (2011) 6:e28991. doi: 10.1371/journal.pone.0028991
37. Palacios MC, Haros M, Sanz Y, Rosel CM. Selection of lactic acid bacteria with high phytate degrading activity for application in whole wheat breadmaking. LWT. (2008) 41:82–92. doi: 10.1016/j.lwt.2007.02.005
38. Stanley D, Geier MS, Hughes RJ, Denman SE, Moore RJ. Highly variable microbiota development in the chicken gastrointestinal tract. PLoS ONE. (2013) 8: e84290. doi: 10.1371/journal.pone.0084290
39. Mann E, Schmitz-Esser S, Zebeli Q, Wagner M, Ritzmann M, Metzler-Zebeli BU. Mucosa-associated bacterial microbiome of the gastrointestinal tract of weaned pigs and dynamics linked to dietary calcium-phosphorus. PLoS ONE. (2014) 9:e86950. doi: 10.1371/journal.pone.0086950
40. Choi KY, Lee TK, Sul WJ. Metagenomic analysis of chicken gut microbiota for improving metabolism and health of chickens - a review. Asian Austr J Anim Sci. (2015) 28:1217–25. doi: 10.5713/ajas.15.0026
41. Kumar S, Chen C, Indugu N, Werlang GO, Singh M, Kim WK, et al. Effect of antibiotic withdrawal in feed on chicken gut microbial dynamics, immunity, growth performance and prevalence of foodborne pathogens. PLoS ONE. (2018) 13:e0192450. doi: 10.1371/journal.pone.0192450
42. Shaufi MAM, Sieo CC, Chong CW, Gan HM, Ho YW. Deciphering chicken gut microbial dynamics based on high-throughput 16S rRNA metagenomics analyses. Gut Pathog. (2015) 7:4. doi: 10.1186/s13099-015-0051-7
43. Danzeisen JL, Kim HB, Isaacson RE, Tu ZJ, Johnson TJ. Modulations of the chicken cecal microbiome and metagenome in response to anticoccidial and growth promoter treatment. PLoS ONE. (2011) 6:e27949. doi: 10.1371/journal.pone.0027949
44. Ballou AL, Ali RA, Mendoza MA, Ellis JC, Hassan HM, Croom WJ, et al. Development of the chick microbiome: how early exposure influences future microbial diversity. Front Vet Sci. (2016) 3:2. doi: 10.3389/fvets.2016.00002
45. Danzeisen JL, Calvert AJ, Noll SL, McComb B, Sherwood JS, Logue CM, et al. Succession of the turkey gastrointestinal bacterial microbiome related to weight gain. Peer J. (2013) 1:e237. doi: 10.7717/peerj.237
46. Hammons S, Oh PL, Martinez I, Clark K, Schlegel VL, Sitorius E, et al. A small variation in diet influences the Lactobacillus strain composition in the crop of broiler chickens. Syst Appl Microbiol. (2010) 33:275–81. doi: 10.1016/j.syapm.2010.04.003
47. Rehman H, Hellweg P, Taras D, Zentek J. Effects of dietary inulin on the intestinal short chain fatty acids and microbial ecology in broiler chickens as revealed by denaturing gradient gel electrophoresis. Poult Sci. (2008) 87:783–9. doi: 10.3382/ps.2007-00271
48. Pourabedin M, Zhao X. Prebiotics and gut microbiota in chickens. FEMS Microbiol Lett. (2015) 362:fnv122. doi: 10.1093/femsle/fnv122
49. Walugembe M, Hsieh JC, Koszewski NJ, Lamont SJ, Persia ME, Rothschild MF. Effects of dietary fiber on cecal short-chain fatty acid and cecal microbiota of broiler and laying-hen chicks. Poult Sci. (2015) 94:2351–9. doi: 10.3382/ps/pev242
50. Gruninger RJ, Selinger LB, Mosimann SC. Structural analysis of a multifunctional, tandemly repeated inositol polyphosphatase. J Mol Biol. (2009) 392:75–86. doi: 10.1016/j.jmb.2009.05.079
51. Borda-Molina D, Vital M, Sommerfeld V, Rodehutscord M, Camarinha-Silva A. Insights into broilers' gut microbiota fed with phosphorus, calcium, and phytase supplemented diets. Front Microbiol. (2016) 7:2033. doi: 10.3389/fmicb.2016.02033
52. Stanley D, Hughes RJ, Moore RJ. Microbiota of the chicken gastrointestinal tract: influence on health, productivity and disease. Appl Microbiol Biotechnol. (2014) 98:4301–10. doi: 10.1007/s00253-014-5646-2
53. Witzig M, Camarinha Da Silva A, Green-Engert R, Hoelzle K, Zeller E, Seifert J, et al. Spatial variation of the gut microbiota in broiler chickens as affected by dietary available phosphorus and assessed by T-RFLP analysis and 454 pyrosequencing. PLoS ONE. (2015) 10:e0143442. doi: 10.1371/journal.pone.0143442
54. Zhu XY, Zhong T, Pandya Y, Joerger RD. 16S rRNA-based analysis of microbiota from the cecum of broiler chickens. Appl Environ Microbiol. (2002) 68:124–37. doi: 10.1128/AEM.68.1.124-137.2002
55. Sergeant MJ, Constantinidou C, Cogan TA, Bedford MR, Penn CW, Pallen MJ. Extensive microbial and functional diversity within the chicken cecal microbiome. PLoS ONE. (2014) 9:e91941. doi: 10.1371/journal.pone.0091941
56. Deusch S, Tilocca B, Camarinha-Silva A, Seifert J. News in livestock research - use of Omics-technologies to study the microbiota in the gastrointestinal tract of farm animals. Comput Struct Biotechnol J. (2015) 13:55–63. doi: 10.1016/j.csbj.2014.12.005
57. Borda-Molina D, Seifert J, Camarinha-Silva A. Current perspective of the chicken gastrointestinal tract and its microbiome. Comput Struct Biotechnol J. (2018) 16:131–9. doi: 10.1016/j.csbj.2018.03.002
58. Adeola O, Walk CL. Linking ileal digestible phosphorus and bone mineralization in broiler chickens fed diets supplemented with phytase and highly soluble calcium. Poult Sci. (2013) 92:2109–17. doi: 10.3382/ps.2013-03068
59. Hamdi M, López-Vergé S, Manzanilla EG, Barroeta AC, Pérez JF. Effect of different levels of calcium and phosphorus and their interaction on the performance of young broilers. Poult Sci. (2015) 94:2144–51. doi: 10.3382/ps/pev177
60. Gangadoo S, Dinev I, Chapman J, Hughes RJ, Van TTH, Moore RJ, Stanley D. Selenium nanoparticles in poultry feed modify gut microbiota and increase abundance of Faecalibacterium prausnitzii. Appl Microbiol Biotechnol. (2018) 102:1455–66. doi: 10.1007/s00253-017-8688-4
61. Miquel S, Martin R, Rossi O, Bermudez-Humaran LG, Chatel JM, Sokol H, et al. Faecalibacterium prausnitzii and human intestinal health. Curr Opin Microbiol. (2013) 16:255–61. doi: 10.1016/j.mib.2013.06.003
62. Luo YH, Peng HW, Wright AD, Bai SP, Ding XM, Zeng QF, et al. Broilers fed dietary vitamins harbor higher diversity of cecal bacteria and higher ratio of Clostridium, Faecalibacterium, and Lactobacillus than broilers with no dietary vitamins revealed by 16S rRNA gene clone libraries. Poult Sci. (2013) 92:2358–66. doi: 10.3382/ps.2012-02935
63. Hayashi H, Sakamoto M, Kitahara M, Benno Y. Diversity of the Clostridium coccoides group in human fecal microbiota as determined by 16S rRNA gene library. FEMS Microbiol Lett. (2006) 257:202–7. doi: 10.1111/j.1574-6968.2006.00171.x
64. Biddle A, Stewart L, Blanchard J, Leschine S. Untangling the genetic basis of fibrolytic specialization by Lachnospiraceae and Ruminococcaceae in diverse gut communities. Diversity (Basel). (2013) 5:627–40. doi: 10.3390/d5030627
65. Eren AM, Sogin ML, Morrison HG, Vineis JH, Fisher JC, Newton RJ, et al. A single genus in the gut microbiome reflect host preference and specificity. ISME J. (2015) 9:90–100. doi: 10.1038/ismej.2014.97
66. Ten Bruggencate SJM, Bovee-Oudenhoven IMJ, Lettink-Wissink MLG, Katan MB, van der Meer R. Dietary fructooligosaccharides and inulin decrease resistance of rats to salmonella: protective role of calcium. Gut. (2004) 53:530–5. doi: 10.1136/gut.2003.023499
67. Metzler-Zebeli BU, Vahjen W, Baumgärtel T, Rodehutscord M, Mosenthin R. Ileal microbiota of growing pigs fed different dietary calcium phosphate levels and phytase content and subjected to ileal pectin infusion. J Anim Sci. (2010) 88:147–58. doi: 10.2527/jas.2008-1560
68. Metzler BU, Mosenthin R, Baumgärtel T, Rodehutscord M. Effects of P level, phytase and pectin on the chemical composition and carbohydrase activity of fecal bacteria and microbial metabolites in the gastrointestinal tract of pigs. J Anim Sci. (2008) 86:1544–55. doi: 10.2527/jas.2007-0267
69. Teng PY, Kim WK. Review: roles of prebiotics in intestinal ecosystem of broilers. Front Vet Sci. (2018) 5:245. doi: 10.3389/fvets.2018.00245
70. Micciche AC, Foley SL, Pavlidis HO, McIntyre DR, Ricke SC. A review of prebiotics: against salmonella in poultry: current and future for microbiome research applications. Front Vet Sci. (2018) 5:191. doi: 10.3389/fvets.2018.00191
71. Janardhana V, Broadway MM, Bruce MP, Lowenthal JW, Geier MS, Hughes RJ, et al. Prebiotics modulate immune responses in the gut associated lymphoid tissue of chickens. J Nutr. (2009)139:1404–9. doi: 10.3945/jn.109.105007
72. Arsi K, Donoghue AM, Woo-Ming A, Blore PJ, Donogue DJ. The efficacy of selected probiotic and prebiotic combinations in reducing Campylobacter colonization in broiler chickens. J Appl Poult Res. (2015) 24:327–34. doi: 10.3382/japr/pfv032
73. Kaplan H, Hutkins RW. Metabolism of fructooligosaccharides by Lactobacillus paracasei 1195. Appl Environ Microbiol. (2003) 69:2217–22. doi: 10.1128/AEM.69.4.2217-2222.2003
74. Saulnier DM, Molenaar D, de Vos WM, Gibson GR, Kolida S. Identification of prebiotic fructooligosaccharide metabolism in Lactobacillus plantarum WCFS1 through microarrays. Appl Environ Microbiol. (2007) 73:1753–65. doi: 10.1128/AEM.01151-06
75. Wallace RJ. Ruminal microbial metabolism of peptides and amino acids. J Nutr. (1996) 126:1326S−34S. doi: 10.1093/jn/126.suppl_4.1326S
76. Wu S, Baldwin-VI RL, Li W, Li C, Connor EE, Li RW. The bacterial community composition of the bovine rumen detected using pyrosequencing of 16S rRNA genes. Metagenomics. (2012) 1:235571. doi: 10.4303/mg/235571
Keywords: calcium, phosphorus, fructooligosaccharide, gut microbiome, chicken
Citation: Kumar S, Shang Y and Kim WK (2019) Insight Into Dynamics of Gut Microbial Community of Broilers Fed With Fructooligosaccharides Supplemented Low Calcium and Phosphorus Diets. Front. Vet. Sci. 6:95. doi: 10.3389/fvets.2019.00095
Received: 14 January 2019; Accepted: 08 March 2019;
Published: 29 March 2019.
Edited by:
Rajesh Jha, University of Hawaii at Manoa, United StatesReviewed by:
Elijah G. Kiarie, University of Guelph, CanadaKyung-Woo Lee, Konkuk University, South Korea
Copyright © 2019 Kumar, Shang and Kim. This is an open-access article distributed under the terms of the Creative Commons Attribution License (CC BY). The use, distribution or reproduction in other forums is permitted, provided the original author(s) and the copyright owner(s) are credited and that the original publication in this journal is cited, in accordance with accepted academic practice. No use, distribution or reproduction is permitted which does not comply with these terms.
*Correspondence: Woo Kyun Kim, d2traW1AdWdhLmVkdQ==