- 1Jiangsu Key Laboratory of Zoonosis, Yangzhou University, Yangzhou, China
- 2Key Laboratory of Prevention and Control of Biological Hazard Factors (Animal Origin) for Agrifood Safety and Quality, Ministry of Agriculture of China, Yangzhou University, Yangzhou, China
- 3Jiangsu Co-innovation Center for Prevention and Control of Important Animal Infectious Diseases and Zoonoses, Yangzhou University, Jiangsu, China
- 4Joint International Research Laboratory of Agriculture and Agri-Product Safety of the Ministry of Education, Yangzhou University, Jiangsu, China
- 5Lianshui Animal Husbandry and Veterinary Station, Lianyungang, China
- 6Department of Quality and Safety Control, Heyi Food Co. Ltd., Zaozhuang, China
Campylobacter is one of the most important foodborne pathogens worldwide, and poultry is regarded as the main reservoir of Campylobacter. The contamination of Campylobacter in broiler chickens at the farm level is closely related to the transmission of Campylobacter in the poultry production chain. This study identified 464 Campylobacter isolates from 1,534 samples from broiler rearing period and slaughtering process including 233 Campylobacter jejuni isolates and 231 Campylobacter coli isolates. We have observed a dynamic distribution of Campylobacter during broiler chicken production, that 66.3% of Campylobacter isolates were C. jejuni during broiler rearing period, while C. coli occupied 60.4% of Campylobacter isolates during the broiler slaughtering process. A tag-label method allowed us to track the dynamic of Campylobacter in each broiler chicken from 31-day age at rearing to the partition step in the slaughterhouse. At the 31-day during rearing, 150 broiler chicken were labeled, and was tracked for Campylobacter positive from rearing period to slaughtering process. Among the labeled broiler, 11 of the tracking broiler samples were able to detect Campylobacter from rearing period to slaughtering. All Campylobacter isolates from the 11 tracking samples were sequenced and analyzed. C. jejuni isolates were divided into four STs and C. coli isolates were divided into six STs. Isolates with identical core genome were observed from the same tag-labeled samples at different stages indicating a vertical transmission of Campylobacter in the early broiler meat production. Meanwhile, the core genome analysis elucidated the cross-contamination of Campylobacter during the rearing period and the slaughtering process. The virulotyping analysis revealed that all C. jejuni isolates shared the same virulotypes, while C. coli isolates were divided into three different virulotypes. The antimicrobial resistance gene analysis demonstrated that all Campylobacter isolates contained at least two antibiotic resistance genes (ARGs), and the ARG profiles were well-corresponding to each ST type. Our study observed a high prevalence of Campylobacter during the early chicken meat production, and further studies will be needed to investigate the diversity and transmission of Campylobacter in the poultry production chain.
Introduction
Campylobacter is a leading cause of foodborne gastroenteritis in humans with an infection dose causing Campylobacteriosis as low as 500–800 CFU (1, 2). Poultry is a major reservoir of Campylobacter, which takes the main response to transmit this pathogen to humans (3). In Europe, chicken meat consumption was estimated to account for 20–30% of Campylobacteriosis cases, whereas the chicken reservoir might attribute to 50–80% of these cases (3, 4). The contamination of Campylobacter at early chicken meat production stages plays an important role in transmitting Campylobacter from farm to fork. The prevalence of Campylobacter on the farm and during process in the slaughterhouse can also reflect the Campylobacter contamination in meat products (3, 5).
Campylobacter can appear in broiler chicken as early as 14-day age at rearing with a low percentage and increase to a high contamination level at the end of grow-out period (6). During the broiler chicken commercial production, flocks usually consist of 10,000–30,000 chickens per house (3). Campylobacter rarely transmits vertically from parents to chicks, whereas flocks at commercial production have a high risk of Campylobacter amplification and rapid spread due to the intensive production model (6). The slaughter process is one of the most important factors causing Campylobacter cross-contamination in chicken. During the slaughter process, the intestinal content will inevitably contaminate the broiler carcass and the slaughtering environment, which will further introduce Campylobacter to chicken meat (7).
In China, random sampling is the most common method to evaluate the prevalence of Campylobacter in the poultry processing line (5). However, the information provided by these prevalence studies was limited, that the potential transmission routine during broiler chicken production could only be primarily investigated. Our previous study with a “label-tracking method” in slaughterhouse showed the evisceration had the highest Campylobacter positive rate of 97.5% and a contamination load of 2.80 ± 2.52 LogCFU/100 cm2 (5). By now, limited numbers of studies have conducted the investigation on the trackable contamination of Campylobacter from rearing to slaughtering, which can provide more information for the risk assessment decisions.
Virulence factors related to Campylobacter pathogenesis and stress response including the adhesion, invasion, chemotaxis, motility, toxin-activity, immune-evasion, iron-uptake, and secretion system (7). Genes associated to virulence have already been detected by several studies to evaluate the potential risk of Campylobacter isolates to the food safety and public health including flaA for flagellin protein FlaA, outer membrane protein cadF for CadF for adhesion, cdtA, cdtB, and cdtC for cytolethal distending toxin (CDT) subunits, cheY for chemotaxis-related response regulator CheY, iamA for invasion-associated protein iamA and virB11 for Campylobacter invasion located on plasmid (8–10). Recently, the Type VI secretion system (T6SS) have been known to be important for Campylobacter stress survival and pathogenesis, which the hcp gene encoding hemolysin coregulated protein was regarded as a key component for evaluating the pathogenesis and stress resistance of Campylobacter isolates from different sources (8, 11, 12).
The multidrug-resistant (MDR) Campylobacter have been frequently reported from clinic and poultry meat production. Campylobacter has been reported to resistant to several antibiotics including fluoroquinolones, tetracyclines, macrolides, aminoglycosides and β-lactams (13). The persistence of MDR Campylobacter during the early poultry production stage was corresponding to the uncontrolled antibiotic use during the production, which provides a selection pressure for MDR Campylobacter spreading in the production chain (14).
This study aimed to investigate the transmission of Campylobacter from broiler rearing period to slaughtering stages by applying a tracking method. The isolates from tracking samples were selected for whole genome sequence analysis and characterized by MLST and the presence of antibiotic resistance genes and virulence factors.
Materials and Methods
Experimental Design and Sample Collection
To investigate the transmission of Campylobacter from the broiler rearing to slaughter production, this study conducted the sampling from the same selected broiler chickens from rearing to slaughtering by a tracking method. The sampling were conducted according to the advice from a chicken slaughterhouse in Eastern China. The broiler farm was selected as the broiler supplier for the slaughterhouse. The slaughterhouse normally slaughtered 10,000–15,000 broilers per day. In total, three broiler flocks were chosen with relatively small scales of 5,000–10,000 boilers per flock. At each flock, 50 broiler chickens were randomly selected and labeled by plastic vervel tag with numbers at 31-day age. Cloacal samples of each labeled broiler chicken were taken at 31-day age, 37-day age, and at the age when chicken entering slaughterhouse (41–44-day age). At the slaughterhouse, chicken carcass were sampled at four slaughtering stages according to the Hazard Analysis and Critical Control Point including dehairing, evisceration, cooling, and partition (5). During slaughtering, chicken carcasses were hanged for dehairing and evisceration. At the cooling stage, chickens carcasses went through a water pre-cooling tank containing 50 mg/kg sodium hypochlorite with a temperature of 10°C before hanging for partition. Labeled chicken carcasses were tracked as much as possible at four sampling steps during the slaughter process. In addition, random sampling was also conducted at each slaughter sampling stage to overcome the limited sampling size of tracking samples, and investigate the prevalence of Campylobacter during the broiler chicken slaughter process.
In total, 1,534 samples were taken from chicken rearing stage, slaughtering stages, and their relative environments. The detail sampling size for each stage was listed in Table 1. Samples were obtained from the slaughterhouse at a medium-scale slaughterhouse in Eastern China during August to October 2018. Samples were collected as previously described (5). In brief, each cloacal sample was collected by a sterilized cotton stick and stored in Cary-Blair transport medium. At each slaughtering step, wiping samples were collected by phosphate buffer (PBS, pH 7.2) immersed sterilized cotton balls. The whole chicken carcasses surface were swabbed after dehairing, while half exterior surface and half interior surface of the chicken carcasses were swabbed after evisceration, cooling and partition, which the sampling area were ~250 cm2 for each sample (5). Environmental samples at the rearing stage included feed, water, floor, sole, net, bedding, and stool. Feed, water, bedding and stool samples were directly picked up from chicken flocks, and floor, sole and net samples were collected by the surface wiping method. Environmental samples at the slaughtering stages were all collected by wiping the process related machine surface of 250 cm2. Each collected sample was sealed in a sterile homogeneous bag and directly transported to the laboratory for further treatment.
Identification of Campylobacter spp.
For cloacal samples, each cotton stick was immersed in 1 ml PBS for 20 min. For chicken carcass wiping samples, PBS in cotton balls of each sample was squeezed to an Eppendorf tube. Campylobacter from both cloacal samples and carcass wiping samples was identified as previously described Huang et al. (15). Briefly, PBS rinsing solution was serially diluted in saline, and 100 μl of each dilution serial was spread on Campylobacter selective blood free agar mCCDA (modified CCDA, Preston, Oxoid, UK) plate with antibiotics (16). All plates were incubated at 42°C for 48 h under microaerobic condition with 10% CO2, 5% O2, 85% N2. Four or five of presumptive Campylobacter colonies from each mCCDA plate were further identified as Campylobacter jejuni or Campylobacter coli by multiplex PCR. MALDI-TOF analysis was conducted to isolates with identical Campylobacter colony morphology, but could not be identified by multiplex PCR. The multiplex PCR targeted to genes including 16S rRNA for Campylobacter genus, mapA for C. jejuni and ceuE for C. coli. Primers for this multiplex PCR were listed in Table S1. The program of the multiplex PCR was performed with an initial denaturation at 95°C for 10 min, followed by 30 cycles of denaturation at 95°C for 30 s, annealing at 59°C for 90 s, and elongation at 72°C for 60 s, and a final extension at 72°C for 10 min.
Genomic Characterization of Campylobacter From Tracking Samples
From the tracking broiler samples, 11 samples were able to isolate Campylobacter from rearing period to slaughtering operation. A total of 40 Campylobacter isolates were identified from 11 tracking broiler samples. All isolates were sequenced to investigate the contamination route of Campylobacter from broiler chicken rearing to slaughtering process. Genomic DNA of all chosen Campylobacter isolates was extracted by TIANamp Bacteria DNA Kit (Tiangen, Beijing, China) and sequenced by Illumina Hiseq 2500. Reads were assembled to contigs by SPAdes version 3.10.0 (17). Multilocus sequence typing was analyzed by PubMLST database for Campylobacter (https://pubmlst.org/campylobacter/). All sequenced Campylobacter isolates was analyzed for the core genome regions by Parsnp software, and the whole genome sequencing (WGS) data submitted to European Nucleotide Archive database with the accession number PRJEB36059 for C. jejuni isolates and the PRJEB36073 for C. coli isolates. Virulence factors were detected by blastn including flaA, cadF, virB11, cdtA, cdtB, cdtC, iamA, ciaB, cheY, and hcp. Antimicrobial resistance genes of all sequenced isolates were detected by ReFinder 3.3 database (https://cge.cbs.dtu.dk/services/ResFinder/) (18).
Statistical Analysis
The proportions of Campylobacter in different sampling steps were based on Chi-square analysis with SPSS statistical package (SPSS Inc., Chicago, USA). Statistical significance was set at p ≤ 0.05.
Results
Prevalence of Campylobacter From Broiler Chicken Rearing to Slaughtering Production
A total of 1,534 samples were collected from broiler chicken farm to the slaughterhouse, which includes 1,196 chicken cloacal samples, and 338 environment samples (Tables 1, 2). In total, 27.2% of the samples were Campylobacter positive. During the rearing period, a total of 300 cloacal samples from broiler chicken were collected, including 150 samples at both 31-day and 37-day age. 39.3% of chicken cloacal samples were Campylobacter positive at 31-day age, while the Campylobacter positive rate was increased to 60% at 37-day age (p = 0.001**). A total of 150 environmental samples at the rearing period, which was 1.6% of the rearing environmental samples at 31-day age and 3.2% at 37-day age, respectively (Table 2).
At the slaughtering stage, 145 cloacal samples were collected before broiler entering the slaughter operation (41–44- day age broiler), which was 41.4% of Campylobacter positive. Compared to the cloacal samples before slaughter operation, the Campylobacter positive rate decreased to 12.9% at the dehairing stage (p = 0.000**), while, the percentage of Campylobacter positive samples had a steep rise to 53.4% during evisceration (p = 0.000**). At the cooling step, the prevalence of Campylobacter were decreased to 14.8% (p = 0.000**) and continuously kept at a low level (13.6%) during partition (p = 0.879). In slaughtering environment, the Campylobacter contamination from the machine surface were similar at dehairing (31.8%), evisceration (31.8%), and cooling (36.3%), and dramatically decreased at partition (9.1%) (Table 2).
A total of 233 C. jejuni isolates and 231 C. coli isolates were identified from the collected samples from the rearing period to the slaughtering process. At the rearing stage, a total of 120 C. jejuni isolates and 64 C. coli isolates were identified from broiler chicken cloacal samples. Before entering the slaughterhouse (41–44 day age), 30 C. jejuni isolates and 22 C. coli isolates were identified from the cloacal samples (Table 1). One hundred twenty-six C. coli isolates and 60 C. jejuni were identified from swabbing samples collected during the slaughtering process (Table 1).
Distribution of Campylobacter spp. From the Rearing Period to the Slaughtering Process
In total, 59 tagged broiler chickens with tag numbers were able to identify Campylobacter during rearing and/or slaughtering, which provide a primary distribution data of Campylobacter spp. for early chicken meat production (Table S2). Campylobacter jejuni were predominant during the rearing period, while C. coli were more frequently detected during the slaughter process. During the rearing period, no C. coli were detected at 31-day age and only four samples were identified with C. coli at 37-day age. Before entering the slaughter process, the distribution of C. jejuni and C. coli in labeled broiler chicken cloacal samples were at the same level, which were 25 and 22 positive samples, respectively. During slaughtering, C. jejuni was only detected in two samples from the dehairing step, and 11 samples from the evisceration step. On the other hand, C. coli were more prevalently observed from 11 of dehairing samples and 32 of evisceration samples. Both cooling and partition stage showed a sharp decrease of Campylobacter prevalence, which only one C. jejuni and one C. coli were identified from the cooling samples and one C. coli from the partition samples. In addition, one sample from 37-day age rearing stage and six samples from the evisceration steps during slaughter were contaminated by both C. jejuni and C. coli.
Genomic Characterization of Campylobacter From Tracking Broiler Samples
During the sampling process, 11 labeled samples were able to identify Campylobacter from both rearing period (31-day and/or 37-day age chicken) and slaughtering stages. A total of 40 Campylobacter isolates were identified including 20 C. jejuni isolates and 20 C. coli isolates (Table S3). All 40 Campylobacterisolates were whole genomic sequenced and conducted MLST and core genomic analysis to evaluate the similarity of Campylobacter isolates during the early broiler chicken production. In total, four ST types were observed in sequenced C. jejuni isolates from labeled samples including ST8089, ST10242, ST10244, and ST10243. The core genomic analysis divided C. jejuni isolates into four clusters with a predominant cluster of isolates with ST10242 mainly from cloacal samples at both rearing stages and before entering the slaughter process (Figure 1). Two labeled samples (No. 87 and 171) were able to identify C. jejuni from the same STs during rearing and slaughtering. For C. coli isolates, six different ST types were observed including ST1121, ST830, ST1568, ST1625, ST872, and ST829 (Figure 2). Fourteen C. coli isolates were grouped to ST1568, which were from nine labeled broiler chicken samples at slaughtering stages including cloacal samples before entering the slaughtering process (41–44-day age) and swabbing samples at dehairing, partition, and evisceration steps during slaughter (Figure 2). In addition, ST1568 isolates were detected at different slaughter steps in four labeled samples including sample No. 62, 64, 68, and 86. Meanwhile, ST830 were observed form labeled chicken No. 171 from cloacal sample before entering the slaughter process line and dehairing step (Figure 2). The detection of the same ST types of C. jejuni and C. coli indicated the transmission of the same ST types during the slaughtering process.
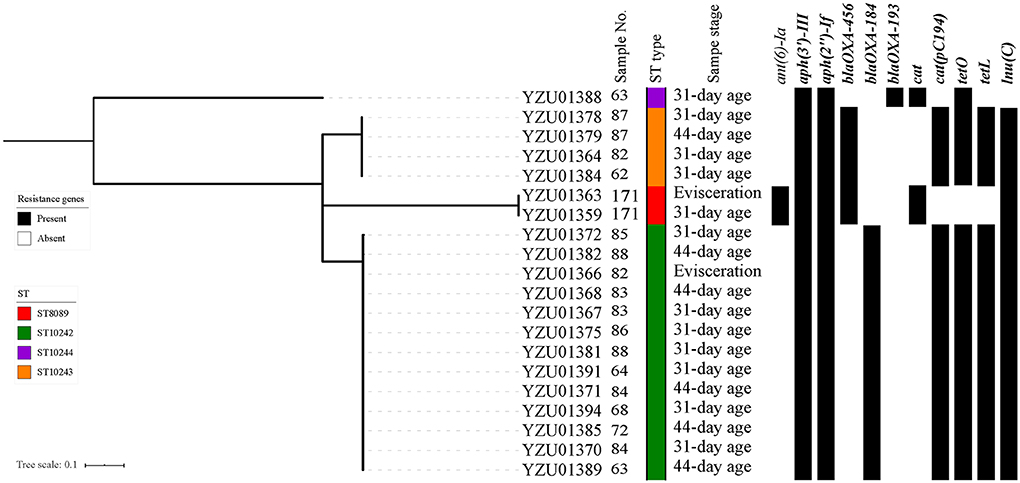
Figure 1. Phylogenetic tree based on core genome and drug resistance genes of Campylobacter jejuni. The analysis included 20 C. jejuni isolates from the tagged broiler sample from rearing period to the slaughter operation. The antimicrobial resistant genes were listed according to the WGS data.
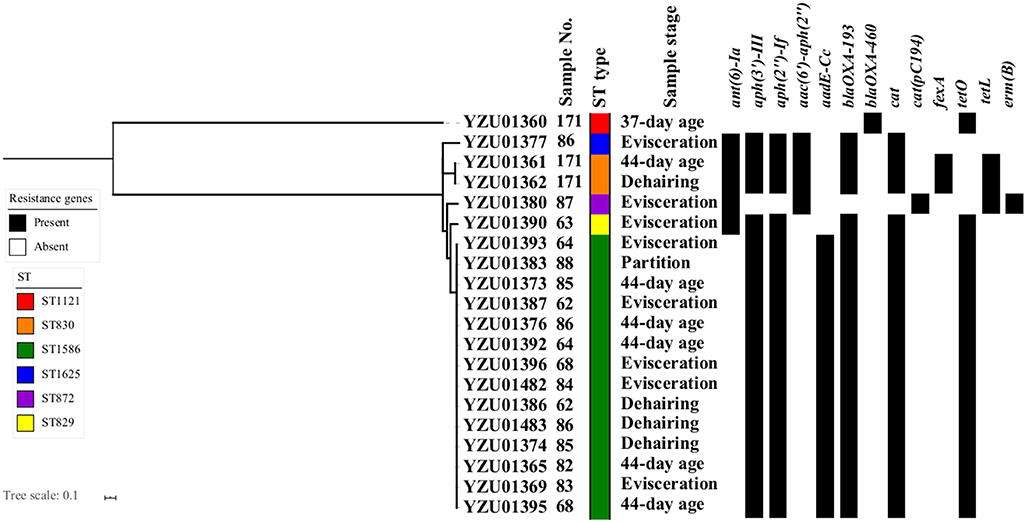
Figure 2. Phylogenetic tree based on core genome and drug resistance genes of Campylobacter coli. The analysis included 20 C. coli isolates from the tagged broiler sample from rearing period to the slaughter operation. The antimicrobial resistant genes were listed according to the WGS data.
Virulence Factors and Antibiotic Resistance Genes in Campylobacter spp.
Virulence factors were detected in all sequenced C. jejuni and C. coli isolates from tracking broiler chicken samples. All C. jejuni isolates contained eight virulence factors including flaA, cadF, cdtA, cdtB, cdtC, iamA, ciaB, cheY (Table 3). Three different virulent haplotypes were observed in the C. coli isolates, which one isolate belonged to ST1625 only containing cheY, two isolates belonged to ST830 containing flaA, cheY and virB11, and the rest of sequenced C. coli isolates (n = 17) containing flaA and cheY (Table 3).
In total, eleven antibiotic resistance genes (ARGs) were detected from 20 sequenced C. jejuni isolates (Figure 1) and thirteen ARGs were detected from 20 sequenced C. coli isolates (Figure 2). All C. jejuni isolates displayed ARGs resistant to four different antibiotic groups, and all C. coli isolates contained ARGs resistant to at least two different antibiotics. The presence of ARGs in both C. jejuni and C. coli were correlated to the ST types, which each ST type contained a distinct antibiotic resistance gene type (Figures 1, 2). All C. jejuni isolates and 18 out of 20 C. coli isolates contained aminoglycoside-resistant genes aph(3')-III, aph(2”)-If, and ant(6)-Ia was presented in two C. jejuni isolates and five C. coli isolates, and aac(6')-aph(2”) and aadE-Cc were only present in C. coli isolates. β-lactam resistant gene in C. jejuni and C. coli were diverse, which blaOXA−456 and blaOXA−184 were only present in C. jejuni, and blaOXA−460 were only present in one C. coli isolates. blaOXA−193 were observed in one C. jejuni isolate and 17 out of 19 C. coli isolates. The distribution of chlorophenol resistant genes were also distinct, which the cat gene was observed in three C. jejuni isolates and 17 out of 20 C. coli isolates, while cat(pC194) was observed in 17 C. jejuni isolates and one C. coli isolate, and fexA genes were presented in two C. coli isolates. Tetracycline resistant genes tetO were detected in 18 C. jejuni isolates and 16 C. coli isolates, while tetL were detected in 17 of C. jejuni isolates and three C. coli isolates. Macrolide resistant gene lnu(C) was observed in 19 C. jejuni isolates, while emr(B) were observed in one C. coli isolates.
Discussion
This study applied a tracking method to detect the prevalence of Campylobacter from the broiler chicken rearing period and the slaughter process. At the broiler rearing stage, 150 broilers were tagged by a specific number and tracked for Campylobacter positive by the cloacal sample at 31-day age and 37-day age and before slaughtering. During the rearing period, the Campylobacter positive rate was increased from 39.3 to 60.0%, while was decreased to 41.4% at 41–44- day age before entering the slaughter operation (Table 1). Before slaughtering, feed withdrawal is an important process to ensure the broiler chicken gastrointestinal system as clean as possible, which could increase the difficulty of cloacal samples due to the lack of intestinal contents (19). In Europe, the prevalence of Campylobacter was diverse among different countries, with the high prevalence observed in UK (87.5%) and the Netherland (80%), while the prevalence in Denmark, Germany and France was similar to this study with 40–50% (20, 21). The low prevalence of Campylobacter was reported in Norway (18%) and Italy (17.38%) (20, 22). In Asia, the prevalence of Campylobacter was 26.3% in Thailand and 45% in Japan (23, 24). The environment sample during slaughtering showed a very low Campylobacter contamination.
At the slaughter process, the positive percentage of Campylobacter changed dramatically during different processing steps. The highest prevalence of Campylobacter contamination was observed at the evisceration step due to the expose of intestinal contents. The cooling step is crucial for eliminating Campylobacter on chicken carcasses, that the Campylobacter positive rate decreased from 53.4% during evisceration to 14.75% after cooling. During cooling, the slaughterhouse applied sodium hypochlorite combined with low temperature, which contributed to the reduction of Campylobacter. Chlorine has been known to have bactericidal effect, and has been applied on microbial contamination during chicken processing (25). A previous study from South California also showed a significant decrease of the Campylobacter contamination load for 0.97 logCFU/g after the water cooling process (7, 26). In this study, the Campylobacter positive rate continuously kept at a low level (13.64%) during partition Campylobacter contamination in the environment during the slaughter process was relatively high indicating the possibility of cross contamination during slaughter.
The distribution of Campylobacter was different between broiler rearing period and slaughtering. The distribution of Campylobacter was diverse between rearing period and slaughter operation, which C. jejuni predominant during rearing while C. coli were more frequently observed during slaughtering. A previous prevalence study from China also showed that 208 C. jejuni isolates and 53 C, coli isolates were detected from 767 broiler rearing samples (27). At the slaughter operation, C. coli were more frequently observed compared to C. jejuni, which was also agreed with a previous prevalence study of Campylobacter in the slaughterhouse from Jiangsu province in China (13). This study applied a tracking method to track the Campylobacter from precise samples during the production with an initial sample size of 150 tagged broiler samples. Fifty-nine samples were able to identify Campylobacter at least two sampling steps during the rearing period and the slaughtering process. However, this method also had a drawback of the loss of sample tag during the slaughter process, which only influenced the sample sizes for the further analysis. The drawback were remedied by an additional random sampling during the process in our study.
The whole genomic analysis was performed on 40 Campylobacter isolates from 11 labeled samples to track the variation of Campylobacter at both the rearing stage and during the slaughter operation. The MLST and core genome analysis showed the cross-contamination of Campylobacter from the rearing period to the slaughter operation. At the rearing stage, C. jejuni ST10244 and ST10243 and C. coli ST830 and ST1586 were isolated from the same tagged broiler chicken samples at different production stages indicating that the rearing stage contamination of Campylobacter could influence the Campylobacter contamination in the downstream of food production chain. C. jejuni ST10242 was predominant at the broiler rearing period. The prevalence of C. coli ST1586 at the slaughter operation instead of C. jejuni ST10242 from rearing period indicating that cross-contamination during slaughter operation could be a main contribution of Campylobacter contamination in chicken meat products. C. jejuni ST 8089 was reported to be predominant during chicken slaughtering process from a previous Chinese study (13). Campylobacter coli ST1121 and ST1625 were only reported from animals (16, 28, 29), while C. coli ST830, ST1586, and ST829 was shared between poultry and humans indicating a potential threat to the public health by transmitting to humans through the production chain (24, 30).
We further analyzed the presence of virulence factors in both C. jejuni and C. coli, and observed a distinct different between the two species. All sequenced C. jejuni contained only one virulence gene pattern with the presence of eight out of nine detected virulent factor genes including flaA, cadF, cdtA, cdtB, cdtC, iamA, ciaB, cheY, which involved pathogenesis of motility, adhesion, invasion, toxin production, and chemotaxis. On the other hand, C. coli contained three different virulence gene patterns with the presence of only three virulent determinant genes including flaA, cheY, and virB11. A previous Canadian study demonstrated virulence factors in C. jejuni isolates from poultry meat relating to toxin production (cdtA, cdtB, cdtC), cell adhension (cadF) and invasion (ciaB, iam) (31). Meanwhile, A recent study of C. coli from duck sources in Korea reported the predominant of flaA, cadF, ceuE, and cdtA, while iamA, virB11 and hcp were also sporadically observed (30). Previous studies also demonstrated that C. jejuni carried more virulent-related genes compared to C. coli, which might contribute to the survival and colonization of C. jejuni in the poultry intestines (16, 32).
In this study, all sequenced Campylobacter isolates were analyzed for the presence of ARGs. In addition, the antibiotic resistant genotypes were well-corresponding to the ST types (Figures 1, 2). The aminoglycoside-resistant genes ant(6)-Ia, aph(3')-III, aph(2”)-If in C. jejuni and C. coli were frequently reported from other studies, which were related to the resistance to aminoglycoside (33, 34). All isolates contained the catA gene were associated with the resistance to chloramphenicol, which is relatively high compared to other studies. The cat gene had a prevalent of 14.3% in C. jejuni from broiler in Shanghai, China in 2016 (35). The WGS study of C. jejuni from the US revealed that two of 114 isolates carrying the catA gene (33). The fexA gene were related to phenicol exporter was presented in two C. coli isolates ST830 (33, 36). The tetracycline resistance gene tetO was reported from the previous study which were located on plasmid causing the spread of tetO in C. jejuni (33, 37). In addition, tetO was also observed in C. jejuni isolates from wild bird and humans (22). On the other hand, the tetL gene were only reported in Campylobacter spp. from patient in Taiwan (38). Nineteen out of twenty C. jejuni isolates contained the lnuC gene, which was associated with the resistance to lincomycin but susceptible to clindamycin and erythromycin. The lnuC gene in Campylobacter were firstly reported in the US, which two C. coli isolates from human were lnuC positive, and was rarely reported in livestock and meat (14, 33). The lnuC gene has not reported in Campyloabcter spp. in China yet. One of C. coli isolate belonging to ST872 contained the emr(B) gene associating with macrolide resistance in China. The emr(B) gene was located on the multidrug resistance genomic islands, which were most frequently observed in C. coli in China from both clinic and poultry isolates (39, 40).
Conclusion
A previous study by the Swedish Campylobacter program showed that Campylobacter contamination at the farm level could increase Campylobacter contamination at the slaughter level (41, 42). This study investigated the overall prevalence of Campylobacter from the broiler chicken rearing period to the slaughter process and characterized Campylobacter isolates by WGS analysis. The tracking sampling method provided evidence of Campylobacter species diversity from the broiler rearing period to the slaughtering process. We observed the predominant of C. jejuni during broiler rearing period and the predominant of C. coli during slaughtering operation, and provided evidence of Campylobacter transmission from the same label-tagged broiler samples during rearing period to the slaughter operation. In addition, the virulent factor in C. jejuni isolates and C. coli isolates showed a distinct difference. Meanwhile, multidrug-resistant patterns were observed in all sequenced isolates indicating a potential risk of transmission in the broiler meat production chain. Further studies would be required to analyze risk factors for Campylobacter contamination during the early stage broiler production, and the potential risk of the transmission of Campylobacter contamination to the final meat products.
Data Availability Statement
The WGS data has been published in ENA database. Requests to access the datasets should be directed to Yuanyue Tang, dGFuZ3l5QHl6dS5lZHUuY24=.
Ethics Statement
This study was carried out in accordance with the principles of the Basel Declaration and recommendations of the institutional administrative committee and ethics committee of laboratory animals, Animal Welfare and Ethics Committees of Yangzhou University. The protocol was approved by the Animal Welfare and Ethics Committees of Yangzhou University.
Author Contributions
YT, QJ, LK, and JH contributed to the conception and design of this study. YT, QJ, ZW, YY, and HT were responsible for the acquisition of the data analyzed in this study. YT, QJ, FR, JH, and XJ were involved in the analysis and interpretation associated with this work. All the authors were involved in manuscript revisions and final approval of the version to be published.
Conflict of Interest
LK was employed by the company Heyi Food Co. Ltd.
The remaining authors declare that the research was conducted in the absence of any commercial or financial relationships that could be construed as a potential conflict of interest.
Acknowledgments
We thank for the staff from food quality and safety department at the slaughterhouse in Eastern China for helping us process the sampling activities. We are grateful to all members of the Jiangsu Key Laboratory of Zoonosis for kind comments and suggestions. This work was supported by the National Key Research and Development Program of China (2018YFD0500500) and the National Natural Science Foundation of China (31872493).
Supplementary Material
The Supplementary Material for this article can be found online at: https://www.frontiersin.org/articles/10.3389/fvets.2020.00227/full#supplementary-material
References
1. Robinson DA. Infective dose of Campylobacter jejuni in milk. Br Med J. (1981) 282:1584. doi: 10.1136/bmj.282.6276.1584
2. Kaakoush NO, Castano-Rodriguez N, Mitchell HM, Man SM. Global epidemiology of Campylobacter infection. Clin Microbiol Rev. (2015) 28:687–720. doi: 10.1128/CMR.00006-15
3. Skarp CPA, Hanninen ML, Rautelin HIK. Campylobacteriosis: the role of poultry meat. Clin Microbiol Infect. (2016) 22:103–9. doi: 10.1016/j.cmi.2015.11.019
4. EFSA. EFSA panel on biological hazards (BIOHAZ); scientific opinion on campylobacter in broiler meat production: control options and performance objectives and/or targets at different stages of the food chain. EFSA J. (2011) 9:2105. doi: 10.2903/j.efsa.2011.2105
5. Huang JL, Zang XQ, Zhai WH, Guan CA, Lei TY, Jiao XN. Quantitative analysis of Campylobacter spp. contamination in chicken slaughtering lines by “label tracking method” in Eastern China. Food Control. (2017) 80:67–73. doi: 10.1016/j.foodcont.2017.03.052
6. Gregory E, Barnhart H, Dreesen DW, Stern NJ, Corn JL. Epidemiological study of Campylobacter spp. in broilers: source, time of colonization, and prevalence. Avian Dis. (1997) 41:890–8. doi: 10.2307/1592343
7. Rosenquist H, Sommer HM, Nielsen NL, Christensen BB. The effect of slaughter operations on the contamination of chicken carcasses with thermotolerant Campylobacter. Int J Food Microbiol. (2006) 108:226–32. doi: 10.1016/j.ijfoodmicro.2005.12.007
8. Biswas D, Hannon SJ, Townsend HG, Potter A, Allan BJ. Genes coding for virulence determinants of Campylobacter jejuni in human clinical and cattle isolates from Alberta, Canada, and their potential role in colonization of poultry. Int Microbiol. (2011) 14:25–32. doi: 10.2436/20.1501.01.132
9. Bolton DJ. Campylobacter virulence and survival factors. Food Microbiol. (2015) 48:99–108. doi: 10.1016/j.fm.2014.11.017
10. Han XF, Guan XA, Zeng H, Li JK, Huang XL, Wen YP, et al. Prevalence, antimicrobial resistance profiles and virulence-associated genes of thermophilic Campylobacter spp. Isolated from ducks in a chinese slaughterhouse. Food Control. (2019) 104:157–66. doi: 10.1016/j.foodcont.2019.04.038
11. Corcionivoschi N, Gundogdu O, Moran L, Kelly C, Scates P, Stef L, et al. Virulence characteristics of hcp (+) Campylobacter jejuni and Campylobacter coli isolates from retail chicken. Gut Pathog. (2015) 7:20. doi: 10.1186/s13099-015-0067-z
12. Siddiqui F, Champion O, Akram M, Studholme D, Eqani SA, Wren BW, et al. Molecular detection identified a type six secretion system in Campylobacterjejuni from various sources but not from human cases. J Appl Microbiol. (2015) 118:1191–8. doi: 10.1111/jam.12748
13. Zhang X, Tang M, Zhou Q, Zhang J, Yang X, Gao Y. Prevalence and characteristics of Campylobacter throughout the slaughter process of different broiler batches. Front Microbiol. (2018) 9:2092. doi: 10.3389/fmicb.2018.02092
14. Mourkas E, Florez-Cuadrado D, Pascoe B, Calland JK, Bayliss SC, Mageiros L, et al. Gene pool transmission of multidrug resistance among Campylobacter from livestock, sewage and human disease. Environ Microbiol. (2019) 21:4597–613. doi: 10.1111/1462-2920.14760
15. Huang JL, Xu HY, Bao GY, Zhou XH, Ji DJ, Zhang G, et al. Epidemiological surveillance of Campylobacter jejuni in chicken, dairy cattle and diarrhoea patients. Epidemiol Infect. (2009) 137:1111–20. doi: 10.1017/S0950268809002039
16. Zhang X, Yin T, Du X, Yang W, Huang J, Jiao X. Occurrence and genotypes of Campylobacter species in broilers during the rearing period. Avian Pathol. (2017) 46:215–23. doi: 10.1080/03079457.2016.1248374
17. Bankevich A, Nurk S, Antipov D, Gurevich AA, Dvorkin M, Kulikov AS, et al. SPAdes: a new genome assembly algorithm and its applications to single-cell sequencing. J Comput Biol. (2012) 19:455–77. doi: 10.1089/cmb.2012.0021
18. Zankari E, Hasman H, Cosentino S, Vestergaard M, Rasmussen S, Lund O, et al. Identification of acquired antimicrobial resistance genes. J Antimicrob Chemother. (2012) 67:2640–4. doi: 10.1093/jac/dks261
19. Van Dam L, Kuipers EJ, Van Leerdam ME. Performance improvements of stool-based screening tests. Best Pract Res Clin Gastroenterol. (2010) 24:479–92. doi: 10.1016/j.bpg.2010.03.009
20. Shane SM. Campylobacter infection of commercial poultry. Rev Sci Tech. (2000) 19:376–95. doi: 10.20506/rst.19.2.1224
21. Newell DG, Fearnley C. Sources of Campylobacter colonization in broiler chickens. Appl Environ Microbiol. (2003) 69:4343–51. doi: 10.1128/AEM.69.8.4343-4351.2003
22. Marotta F, Garofolo G, Di Marcantonio L, Di Serafino G, Neri D, Romantini R, et al. Antimicrobial resistance genotypes and phenotypes of Campylobacter jejuni isolated in Italy from humans, birds from wild and urban habitats, and poultry. PLoS ONE. (2019) 14:e0223804. doi: 10.1371/journal.pone.0223804
23. Prachantasena S, Charununtakorn P, Muangnoicharoen S, Hankla L, Techawal N, Chaveerach P, et al. Distribution and genetic profiles of Campylobacter in commercial broiler production from breeder to slaughter in Thailand. PLoS ONE. (2016) 11:e0149585. doi: 10.1371/journal.pone.0149585
24. Asakura H, Sakata J, Nakamura H, Yamamoto S, Murakami S. Phylogenetic diversity and antimicrobial resistance of Campylobacter coli from humans and animals in Japan. Microbes Environ. (2019) 34:146–54. doi: 10.1264/jsme2.ME18115
25. Trindade MA, Kushida MM, Montes Villanueva ND, Dos Santos Pereira DU, De Oliveira AE. Comparison of ozone and chlorine in low concentrations as sanitizing agents of chicken carcasses in the water immersion chiller. J Food Prot. (2012) 75:1139–43. doi: 10.4315/0362-028X.JFP-11-288
26. Ivanova M, Singh R, Dharmasena M, Gong C, Krastanov A, Jiang X. Rapid Identification of Campylobacter jejuni from poultry carcasses and slaughtering environment samples by real-time PCR. Poult Sci. (2014) 93:1587–97. doi: 10.3382/ps.2013-03736
27. Chen X, Naren GW, Wu CM, Wang Y, Dai L, Xia LN, et al. Prevalence and antimicrobial resistance of Campylobacter isolates in broilers from China. Vet Microbiol. (2010) 144:133–9. doi: 10.1016/j.vetmic.2009.12.035
28. Miller WG, Englen MD, Kathariou S, Wesley IV, Wang G, Pittenger-Alley L, et al. Identification of host-associated alleles by multilocus sequence typing of Campylobacter coli strains from food animals. Microbiology. (2006) 152:245–55. doi: 10.1099/mic.0.28348-0
29. Wang Y, Zhang M, Deng F, Shen Z, Wu C, Zhang J, et al. Emergence of multidrug-resistant Campylobacter species isolates with a horizontally acquired rRNA methylase. Antimicrob Agents Chemother. (2014) 58:5405–12. doi: 10.1128/AAC.03039-14
30. Guk JH, Kim J, Song H, Kim J, An JU, Kim J, et al. Hyper-aerotolerant Campylobacter coli from duck sources and its potential threat to public health: virulence, antimicrobial resistance, and genetic relatedness. Microorganisms. (2019) 7:579. doi: 10.3390/microorganisms7110579
31. Dasti JI, Tareen AM, Lugert R, Zautner AE, Gross U. Campylobacter jejuni: a brief overview on pathogenicity-associated factors and disease-mediating mechanisms. Int J Med Microbiol. (2010) 300:205–11. doi: 10.1016/j.ijmm.2009.07.002
32. Lapierre L, Gatica MA, Riquelme V, Vergara C, Yanez JM, San Martin B, et al. Characterization of antimicrobial susceptibility and its association with virulence genes related to adherence, invasion, and cytotoxicity in Campylobacter jejuni and Campylobacter coli isolates from animals, meat, and humans. Microb Drug Resist. (2016) 22:432–44. doi: 10.1089/mdr.2015.0055
33. Zhao S, Tyson GH, Chen Y, Li C, Mukherjee S, Young S, et al. Whole-genome sequencing analysis accurately predicts antimicrobial resistance phenotypes in Campylobacter spp. Appl Environ Microbiol. (2016) 82:459–66. doi: 10.1128/AEM.02873-15
34. Zhao S, Mukherjee S, Li C, Jones SB, Young S, Mcdermott PF. Cloning and expression of novel aminoglycoside phosphotransferase genes from Campylobacter and their role in the resistance to six aminoglycosides. Antimicrob Agents Chemother. (2018) 62:e01682–e01617. doi: 10.1128/AAC.01682-17
35. Li B, Ma L, Li Y, Jia H, Wei J, Shao D, et al. Antimicrobial resistance of Campylobacter species isolated from broilers in live bird markets in Shanghai, China. Foodborne Pathog Dis. (2017) 14:96–102. doi: 10.1089/fpd.2016.2186
36. Liu D, Li X, Liu W, Yao H, Liu Z, Fessler AT, et al. Characterization of multiresistance Gene cfr(C) variants in Campylobacter from China. J Antimicrob Chemother. (2019) 74:2166–70. doi: 10.1093/jac/dkz197
37. French NP, Zhang J, Carter GP, Midwinter AC, Biggs PJ, Dyet K, et al. Genomic analysis of fluoroquinolone- and tetracycline-resistant Campylobacter jejuni sequence type 6964 in humans and poultry, New Zealand, 2014-2016. Emerg Infect Dis. (2019) 25:2226–34. doi: 10.3201/eid2512.190267
38. Chang YC, Tien N, Yang JS, Lu CC, Tsai FJ, Huang TJ, et al. Class 1 integrons and plasmid-mediated multiple resistance genes of the Campylobacter species from pediatric patient of a university hospital in Taiwan. Gut Pathog. (2017) 9:50. doi: 10.1186/s13099-017-0199-4
39. Bolinger H, Kathariou S. The current state of macrolide resistance in Campylobacter spp.: trends and impacts of resistance mechanisms. Appl Environ Microbiol. (2017) 83:e00416–7. doi: 10.1128/AEM.00416-17
40. Liu D, Liu W, Lv Z, Xia J, Li X, Hao Y, et al. Emerging erm(B)-mediated macrolide resistance associated with novel multidrug resistance genomic islands in campylobacter. Antimicrob Agents Chemother. (2019) 63:e00153–119. doi: 10.1128/AAC.00153-19
41. Bouwknegt M, Van De Giessen AW, Dam-Deisz WD, Havelaar AH, Nagelkerke NJ, Henken AM. Risk factors for the presence of Campylobacter spp. in dutch broiler flocks. Prev Vet Med. (2004) 62:35–49. doi: 10.1016/j.prevetmed.2003.09.003
Keywords: Campylobacter, broiler chicken production, Campylobacter jejuni, Campylobacter coli, whole genome sequencing
Citation: Tang Y, Jiang Q, Tang H, Wang Z, Yin Y, Ren F, Kong L, Jiao X and Huang J (2020) Characterization and Prevalence of Campylobacter spp. From Broiler Chicken Rearing Period to the Slaughtering Process in Eastern China. Front. Vet. Sci. 7:227. doi: 10.3389/fvets.2020.00227
Received: 10 January 2020; Accepted: 03 April 2020;
Published: 30 April 2020.
Edited by:
Subhash Verma, Chaudhary Sarwan Kumar Himachal Pradesh Krishi Vishvavidyalaya, IndiaReviewed by:
Marja-Liisa Hänninen, University of Helsinki, FinlandElisabetta Di Giannatale, Experimental Zooprophylactic Institute of Abruzzo and Molise G. Caporale, Italy
Copyright © 2020 Tang, Jiang, Tang, Wang, Yin, Ren, Kong, Jiao and Huang. This is an open-access article distributed under the terms of the Creative Commons Attribution License (CC BY). The use, distribution or reproduction in other forums is permitted, provided the original author(s) and the copyright owner(s) are credited and that the original publication in this journal is cited, in accordance with accepted academic practice. No use, distribution or reproduction is permitted which does not comply with these terms.
*Correspondence: Jinlin Huang, amlubGluQHl6dS5lZHUuY24=