- 1GenPhySE, Université de Toulouse, INRAE, ENVT, Castanet-Tolosan, France
- 2CCPA, Janzé, France
- 3EVIALIS, Lieu dit Talhouët, Saint-Nolff, France
- 4MiXscience, Bruz, France
- 5Wisium, Chierry, France
- 6TECHNA, Couëron, France
- 7GeT-PlaGe, Genotoul, INRAE, Castanet-Tolosan, France
Early introduction of a nutritional substrate is a promising biomimetic strategy for controlling the implantation of the microbiota and preserving the health of young animals. In this study, we provided experimental solid substrate in a gel form to stimulate suckling rabbits' intake and to investigate its effects on microbiota implantation and colonization. All the rabbits had access to solid feed outside the nest as of 15 days of age. Except for the control group, rabbits were offered starter feed gels inside the nests from 3 to 18 days of age. These gels were either free of additives (AF_GEL) or contained 4% of fructo-oligosaccharides (FOS_GEL) or 4% of mannan-oligosaccharides and β-glucans mixtures (MOS_GEL). The cecal content of 160 rabbits was sampled at 18, 29, 38, and 57 days of age and analyzed using 16S rRNA gene sequencing. Pups consumed an average of 3.95 ± 1.07 g of starter feed gel with a higher intake when it was supplemented with fructo-oligosaccharides (+1.2 g; P < 0.05). Starter feed gel consumption increased the ensuing intake of pellets (+17 g from 15 to 21 days; P < 0.05). Alpha-diversity indexes were similar between groups and prebiotic supplementation did not induce a clear shift in microbiota pattern. Conversely, when considering rabbits that consumed more starter feed, the highest proportions of bacteria with plant-degrading abilities, such as species from the Lachnospiraceae and Ruminococcaceae families, were observed at 18 days of age. However, fermentative activities were not affected by starter feed intake at 29, 38, and 57 days of age. By providing comprehensive results on the regulation of microbial community structure at the onset of solid feed intake, this research paves the way for further studies on digestive ecosystem maturation.
Introduction
Young mammals are prone to enteritis around weaning (e.g., pigs, ruminants, rabbits, etc.), which represents a stressful period due to the end of milk consumption and the separation from the mother. Although the etiology of these digestive disorders is not yet clear, gut microbiota may play a pivotal role. Indeed, disruption of the gut microbiota homeostasis, characterized by the greater abundance of opportunistic and pathogenic species and the decreased abundance of fibrolytic and butyrate-producing bacteria, appears to predispose the young animals to enteric infections (1–3). On the contrary, when an equilibrium between mammalian host and gut microbiota is established, the microbiota will contribute to host defense in different ways (4). In particular, the gut microbiota prevents the establishment of detrimental bacteria through competition for shared nutrients and niches (5). In addition to this “barrier” function, numerous studies have underlined the action of the gastrointestinal microbiota on the immune system (6). Early-life exposure to the gut microbiota is determinant, in particular, for the establishment of a normal immune function (7, 8).
Longitudinal studies in young mammals underlined highly variable gut taxonomies between neonatal animals, followed by the establishment of “climax” communities with greater uniformity in late post-weaning states [humans: (9); rabbits: (10); pigs: (11); calves: (12)]. As a consequence of this immaturity, the early post-natal stage represents a period of permissiveness that has been described as a “window of opportunity” for microbiota and immune system engineering (13). Gut microbiota manipulation in early life can be performed with different tools, including dietary interventions. Indeed, numerous studies have shown that the introduction of solid feed strongly affects the gut microbiota (14–16) since dietary nutrients provide substrates for microbial growth.
The rabbit pup is a good model to study early dietary intervention incidence on gut microbiota installation. Unlike other altricial species, wild rabbit pups are able to ingest solid substrates shortly after birth in the burrow (17). Under controlled breeding conditions, it was demonstrated that pups exhibit a coprophagous behavior within the first week of life (18, 19) and consume the feed provided in the nest as of 8 days of age (20, 21). The ability of the suckling rabbit to consume feed at an early stage can therefore be used as a biomimetic strategy to study the intestinal colonization of commensal bacterial communities.
Little is known about the effects of the quality of solid feed ingested in early life on gut microbiota, but the use of prebiotics to target bacterial groups of interest appears promising. Prebiotics contribute to digestive health preservation by functioning as anti-adhesives, preventing pathogen implantation, stimulating immune maturation and gut barrier function, and serving as fermentable substrates for gut bacteria. The use of mannan-oligosaccharides (MOS) in pre- and post-weaning rabbit diets effectively promotes cecal fermentation and gut barriers (22–24). Moreover, prebiotics are present in mammal's milk (25–27) and are essential to support the development of the commensal microbiota in infants. In humans, fructo-oligosaccharides (FOS) are routinely added to infant formulas to mimic the beneficial effects of milk oligosaccharides on the commensal microbiota (28). Consequently, maintaining prebiotic supplementation at the milk/solid diet transition by providing non-digestible carbohydrates in the starter diet might ensure the installation of a proper ecological succession of bacterial species.
We hypothesized in this study that an early solid dietary intervention would drive microbiota establishment toward the installation of beneficial microorganisms and that prebiotic supplementations would potentiate those effects. In agreement with our previous findings (29), a hydrated starter diet was given to suckling rabbits to stimulate early solid ingestion. The effects of solid feed supplemented or not with prebiotics (FOS or MOS) from 3 to 18 days of age were assessed in terms of animal development, microbiota colonization, and fermentative activity up to 57 days of age. To our knowledge, this study is the first to investigate the effects of post-natal solid feed supplementation on rabbit microbiota structure.
Materials and Methods
Ethics Statement
This study was carried out at the PECTOUL Experimental Unit (INRAE, Castanet-Tolosan, France). Animals were raised and handled according to the European Union's recommendations concerning the protection of animals used for scientific purposes (2010/63/EU) and in agreement with French legislation (NOR: AGRG1238753A 2013). The experiment received the approval of the local ethics committee (SSA_2019_001).
Animal Management
Animal Handling and Housing
Commercial rabbits raised for meat production were used in the experiment (crossbreed of the maternal line Hyplus PS19 and the paternal line Hyplus PS59; Hypharm, France). Before weaning, the does were housed individually with their pups in wire cages (width: 62 cm; length: 69 cm; height: 62 cm) equipped with nests for the pups (width: 25 cm; length: 38 cm; height: 20 cm), as previously reported (29). Two days after parturition (d2), nest quality was assessed on a 3-point scale, i.e., a nest of good quality corresponded to a nest that was fully covered by doe fur. After nest quality assessment, the litter size was standardized to ten pups per doe by cross-fostering or culling. From 3 to 21 days, nursing was controlled and pups had access to milk once a day. All the rabbits received commercial feed pellets ad libitum from 15 to 35 days in a feeder designed for young rabbits (30) that the doe could not reach. At weaning (d35), pups were assigned to collective cages of 5 rabbits and mixing rabbits from different litters was prevented. Until d64, they were fed the same commercial post-weaning diet restricted at 79% of the ad libitum intake (31). No antibiotics were provided to pups and their mothers throughout the experiment. Chemical composition analysis was performed on the commercial diets using ISO methods (DM and ash for dry feed: ISO 6496:1999; nitrogen content: ISO 16634-1:2008; crude fat content: ISO 6492:1999; gross energy: ISO 9831:1998) and the procedures described by the European Group on Rabbit Nutrition [(32); Table 1]. The litter weight after suckling was recorded at 3, 14, 21, and 28 days of age. Rabbits were individually weighed at weaning (d35), 50, 64, and 71 days of age.
Experimental Groups
A total of 44 litters were equally distributed between four experimental groups at 3 days of age by stratified randomization based on the does' parity (multiparous does, an average of five parities), litter weight at standardization (72 ± 7 g) and the allocation in the farm (n = 3 rooms). In the CONTROL group, rabbits had access to solid feed as of day 15 with commercial pellets. An additional starter feed was offered to the three other groups in a hydrated gel form from 3 to 18 days in two plastic cups (volume: 30 mL; Ø = 40 mm; height: 32 mm; GOSSELIN®, Le Mans, France) that were vertically clipped to each side of the nest (Figure 1). The gels were removed before suckling to prevent the doe from eating them. The starter feed gels were renewed every day and their consumption was measured as of 7 days of age. To process the gels, the commercial pellets provided during the pre-weaning period were first mashed (particle sizes smaller than 2 mm). An attractive flavoring additive was added to the mash (vanilla flavor at 0.06%, supplied by Phodé, Terssac, France). For diets including prebiotics, the corresponding additive (powder form) was also mixed with the mash at this stage. Dry products were then thoroughly mixed with hot water (80–90°C) and agar to shape the gels (mash-to-water ratio of 1:4 with 0.6% of agar). Litters that received starter feed gels without an additive belonged to the AF_GEL (Additive-Free Gel) group. The prebiotics used were either fructo-oligosaccharides (FOS_GEL group) or a mixture of mannan-oligosaccharides and β-glucans (MOS_GEL group). Short chain fructo-oligosaccharides were provided as Profeed® (Tereos, Lille, France). This product is obtained from beet sugar through a bio-enzymatic process. It is characterized by a degree of polymerization between 3 and 5 and is composed of three glucose-fructose chains, resulting in a final concentration of 95% of FOS. Mannan-oligosaccharides and β-glucans combinations such as AGRIMOS® were provided by Lallemand Animal Nutrition (Blagnac, France). This additive is obtained by the autolysis of yeast cell walls of Saccharomyces cerevisiae, leading to a final concentration of 45% of MOS. Manufacturers' recommendations for inclusion levels for post-weaned rabbits are typically close to 2 kg per ton of feed (0.2%). Considering the specificity of our experimental design, the additives constituted 0.9% of the gels, the equivalent of 4% of dry matter (DM). The DM content of starter feed gels obtained by freeze-drying for 48 h was similar between gel types (25.6–26.3%). Given the high moisture content of starter feed gels, water losses due to evaporation were evaluated daily for each type of gel. Those autogenic changes in gel mass were taken into account with a correction factor based on the quantity of gel supplied. Feed intake before weaning was measured at the litter level and expressed per rabbit. For feed consumption after 18 days of age, the number of pups was adjusted assuming that dead animals did not consume feed 2 days before their death.
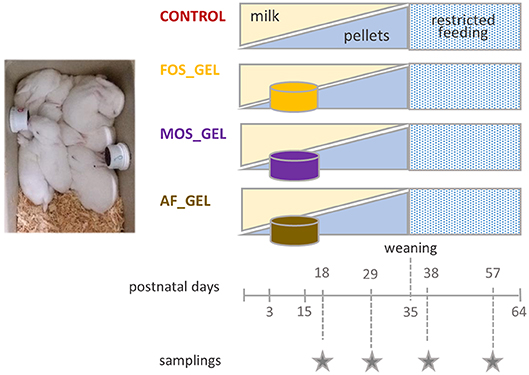
Figure 1. Experimental design: three groups of 11 rabbit litters were provided with a starter feed in a gel form from 3 to 18 days of age in addition to doe milk. The additive composition of the gels varied according to treatments (CONTROL, no starter feed gel provided; FOS, fructo-oligosaccharides; MOS, a mixture of mannan-oligosaccharides and β-glucans; AF, no additive in the starter feed gel).
Measurement of Digestive Tract Development and Cecal Content Sampling
At days 18, 29, 38, and 57, 10 pups per group (one pup per litter) were randomly selected, weighed and killed by electronarcosis and exsanguination (n = 160 pups in total). Blood samples were collected at exsanguination in EDTA tubes immediately stored on ice. After centrifugation (800 g for 10 min at 4°C), the plasmas were stored at −20°C until further analysis. The cecum was isolated and weighed before collection of the digesta in sterile tubes (storage at −80°C). At days 29, 38, and 57, cecal pH was measured by introducing a glass electrode at the ileocecal junction (VWR Collection SP225; Radnor, PA, USA) and fresh cecal contents were in sufficient quantities to be sampled for the following analyses: DM (2 g), volatile fatty acids (VFA) (1 g diluted in 2 mL of H2SO4 at 2% w/v), and ammonia (1 g diluted in 3 mL of H2SO4 at 2% w/v). The cecum was then emptied and weighed with an OHAUS scale (Parsippany, NJ, USA). Finally, the stomach, small intestine and colon were isolated and weighed.
Evaluation of the Fermentative Activity of the Cecal Microbiota: Determination of the Concentration of Ammonia and Volatile Fatty Acids
Ammonia (NH3) and VFA were analyzed after centrifugation and dilution at 1:10. Ammonia concentrations were quantified using a colorimetric method with a continuous flow analyzer (SAN++; Skalar, Norcross, GA, USA), as previously described (33). Volatile fatty acids were determined by gas chromatography (CPG HP 7890A; Agilent, Santa Clara, CA, USA) following a method previously reported (34). Results were expressed according to the liquid phase of cecal content after determination of cecal sample DM by drying at 103°C for 24 h.
ELISA Measurements of IgG and IgA
Total plasma IgG or cecal content IgA levels were determined in duplicates by sandwich ELISA in 96-well plates coated with specific polyclonal goat anti-rabbit IgG or IgA antibody (Bethyl Laboratories, Montgomery, Texas, USA) with further plate reading at 450 nm as previously described (35). IgG were quantified by using a reference IgG serum (Bethyl Laboratories). Regarding IgA, 10 samples were pooled to build a reference sample for the standard curve construction.
DNA Extraction and PCR Amplification of Bacterial 16S Ribosomal Genes for Illumina Sequencing
Total genomic DNA was extracted from 50 mg of cecal digesta using the Quick-DNA Fecal/Soil Microbe 96 Kit (ZymoResearch, Irvine, CA, USA) according to the manufacturer's instructions after mechanical lyses at 30 Hz for 15 min (TisueLyzer II, Qiagen, Hilden, Germany). The 16S rRNA V3-V4 region was amplified by PCR and paired reads were sequenced by MiSeq Illumina Sequencing at the Genomic and Transcriptomic Platform (INRAE, Toulouse, France), as previously described (36).
Sequence Analysis
The Galaxy-supported FROGS pipeline (37) was used to process the 7,503,813 16S ribosomal DNA amplicon sequences obtained. Amplicons without any ambiguous base, with a length between 350 and 500 nucleotides and matching V3 and V4 proximal PCR primer sequences, were kept for clustering. Reads were clustered into OTUs (operational taxonomic units) using the iterative growth process SWARM (aggregation distance = 3) (38). Chimera were detected using VSEARCH (39) and then discarded (15.3% of the total sequences corresponded to 41.8% of the total OTUs determined). Remaining OTUs were filtered to keep OTUs present in at least three samples, representing more than 0.005% of all of the sequences.
The mean number of reads per sample was 30,025 (min: 14,110; max: 61,030). OTU taxonomic affiliation was performed using the BLAST algorithm against the SILVA SSU Ref NR 132 database with a pintail quality of 80 (40). Within-community diversity metrics (α-diversity), including Shannon and Inverse Simpson, were calculated after rarefaction of the OTU table at 14,110 sequences (41). In order to evaluate the repeatability of the relative abundances of the OTUs, 16S rDNA sequences from 15 cecal samples extracted, sequenced and processed twice according to the same procedure were compared. As previously observed (42), the quantification of OTUs with low abundances is poorly repeatable (Figure 2A). We chose the threshold of 0.5% of relative abundances for quantitative repeatability, according to Figure 2B. With such a filter, OTU relative abundance differed by an average of 1.4-fold between two DNA extractions and 16S sequencing analyses of the same sample. As a consequence, the OTUs with average relative abundances below 0.5% within each age*treatment group were removed before further statistical analysis.
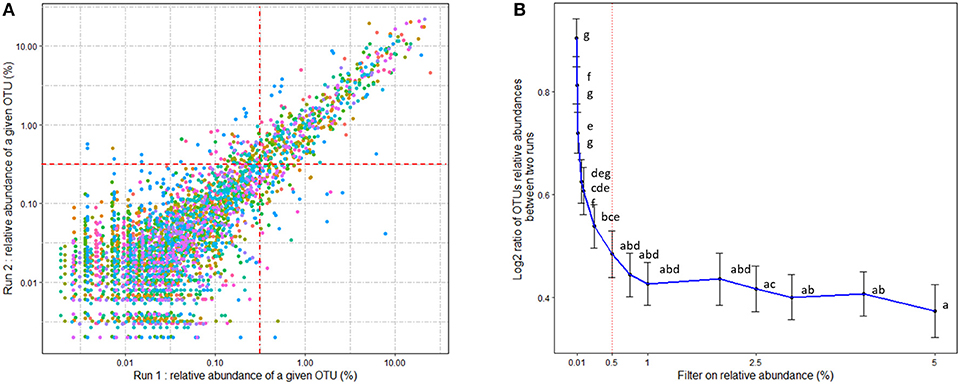
Figure 2. (A) Comparison of OTU relative abundances obtained from 15 rabbit cecal samples extracted and sequenced two times. Technical procedures and bioinformatic treatments were similar for replications. Colors stand for the different rabbit samples. Axes are represented on a log10 scale. (B) Evaluation of a threshold on OTU relative abundances for quantitative repeatability. Binary log ratio of the relative abundances of each OTU on the two extractions and sequencing analyses for a given sample were calculated as a function of a selected filter on the relative abundance. Means followed by a common letter are not significantly different according to the Tukey HSD test at the 0.05 significance level. The minimum threshold filter of 0.5% was selected.
Statistical Analysis
All statistical analyses were performed using R software (version 3.5.1). Differences were considered to be significant when P ≤ 0.05. The Partial Least Squares Discriminant Analysis (PLS-DA) multivariate regression model was used to identify the OTUs that allow discrimination of the response variable using mixOmics package (43). This model uses OTU counts after Total Sum Scaling normalization and Centered Log Ratio Transformation as input to predict the response variable (either experimental group or early feed intake level). The effects of the groups on the discriminant OTUs identified with PLS-DA were analyzed with multiple regression models by controlling the false discovery rate after fourth root transformation applied on relative abundances. Taxonomic assignments to family and genus level obtained by BLAST were considered with a cutoff of 80% for coverage and 80 and 97% for identity, respectively. Taxonomic relative abundances at phylum, family and genus levels, diversity indexes, cecal and blood parameters, longitudinal intakes, and performance data were analyzed using the linear mixed procedure of the nlme package. The mixed model included age, group and their interaction as fixed effects, litter as a random effect, and a correction for age heteroscedasticity was applied when necessary (Supplementary Table S1). Post-hoc comparisons were made with the emmeans R package. The total intake in the nest (from 7 to 17 days) was analyzed using nest quality and experimental group as categorical factors, and litter weight at equalization as the quantitative covariable. Mortality data were analyzed using adjusted chi-squares according to the Donner and Banting procedure (44) so that litter cluster was accounted for Reed (45) and Princée (46).
Results
Effects of Starter Feed Gels and Prebiotic Supplementation on Solid Feed Ingestion by Suckling Rabbits
The first step of our study was to determine if starter feed gels provided from 3 to 18 days of age promoted early-life solid ingestion in suckling rabbits and if these effects were modulated by prebiotic supplementation (FOS or MOS). Rabbit pups began to ingest the starter feed gels provided in the nest as early as 7 days of age, with high variability between litters (Figure 3A). Daily ingestion was significantly affected by the type of gels provided (P = 0.014). Total gel intake in the nest amounted to 3.95 ± 1.07 g of fresh matter and 1.03 ± 0.27 g of DM per pup on average. Total starter feed gel intake in the nest was significantly higher for the FOS_GEL group compared to the MOS_GEL group (+1.3 g of fresh matter; P = 0.011) and tended to be higher for the FOS_GEL group than for the AF_GEL group (+1.0 g of fresh matter; P = 0.057; Figure 3B). Considering the initial prebiotic concentration of the additives used and their rate of incorporation into the gel, pups from the FOS_GEL group ingested a total of 47 ± 11 mg of FOS/rabbit, whereas those in the MOS_GEL group ingested 16 ± 4 mg of MOS/rabbit. The weight of the litter after standardization (d3, average rabbit weight of 98 ± 10 g) positively affected the total starter feed gel intake (P = 0.028; Pearson correlation = 0.41), while the nest quality score did not affect early feed ingestion. All the rabbits had access to similar pellets as of 15 days of age but their intake levels varied according to dietary intervention in early life (Supplementary Table S2). Pre-weaning pellet intake was significantly higher in the FOS_GEL group than in the others, with 17 additional grams of dry matter consumed from 15 to 21 days (P < 0.05; Figure 3C). Altogether, our results show that starter feed gels promoted pre-weaning solid feed ingestion in suckling rabbits and that these effects were amplified when the gels were supplemented with the FOS prebiotic.
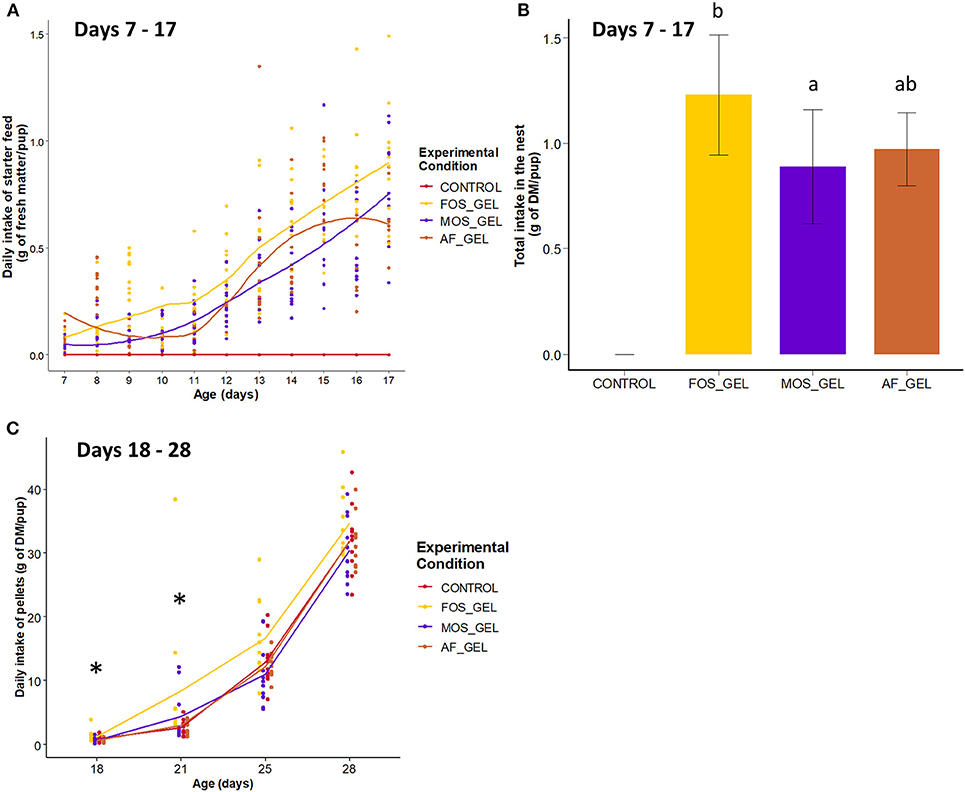
Figure 3. Ingestion of starter feed gels during early life according to treatment and long-term effects of early dietary interventions on subsequent pellet intake before weaning in rabbits. (A) Kinetics of starter feed gel ingestion from 7 to 17 days of age in rabbit pups in the nest, where each point stands for one litter's intake. (B) Total starter feed gel intake in the nest from 7 to 17 days of age, means with different letters differ at P < 0.05. (C) Daily Intake of pellets from 18 to 28 days of age in young rabbits. Significant differences between experimental groups found within ages are represented with an asterisk. Error bars represent standard deviation data. CONTROL, no starter feed gel provided; FOS_GEL, fructo-oligosaccharides; MOS_GEL, a mixture of mannan-oligosaccharides and β-glucans; AF_GEL, no additive in the starter feed gel.
Effects of Starter Feed Gels and Prebiotic Supplementation on the Growth and Health of Young Rabbits
In the next step, we analyzed the consequences of the stimulation of solids' ingestion induced by starter feed gels and prebiotics on the growth and health of young rabbits. Thirteen rabbits were found dead shortly after equalization and before rabbit pups began to eat starter gel feed (3–6 day). After this period, mortality was 2.8% from 7 to 35 days and 0.6% after weaning. Survival between 7 and 35 days was numerically lower in the litters from the MOS_gel group (Figure 4A) but this was not significant (χ2 = 2.9, df = 3, P = 0.403). Relative to the CONTROL group, the odds ratios calculated in the FOS_GEL, MOS_GEL, and AF_GEL groups were 0.5 (), 2.7 (), and 1 respectively. Animal weight before and after weaning and average daily weight gain were not affected by experimental treatments (Figure 4B and Supplementary Table S3). We analyzed immunoglobulins in plasma and cecal content as markers of the young rabbits' immune status. IgA relative concentration in cecal content was the highest at 18 days (P < 0.05), probably due to passive immunity (Figure 4C). Indeed, relative IgA concentration in cecal content dropped as milk ingestion decreased and reached the lowest level at day 38 (when rabbits eat only solid feed). Cecal IgA concentration then increased at day 57 (P < 0.05), probably in association with the development of the young rabbits' adaptive immune system. IgA levels were affected by the interaction age*experimental group (P = 0.009), with effects visible as of 29 days of age (Figure 4D). At 29 and 57 days, cecal IgA levels were significantly higher for rabbits from the FOS_GEL group compared to the AF_GEL group. Similarly, higher cecal IgA concentrations were found for the FOS_GEL group compared to the MOS_GEL group at 57 days. Plasma levels of IgG varied with age (P < 0.001) but were similar between groups (Figure 4E). Similarly to relative cecal IgA concentrations, IgG plasma levels were the highest at 18 days of age and reached the lowest level at days 29–38 before an increase at day 57. Overall, our results show that the stimulation of early-life solid feed ingestion by the supply of starter feed gels without an additive had no effect on the growth and health status of young rabbits. In contrast, supplementation of the starter feed gel with the FOS prebiotic increased IgA concentration in the cecum.
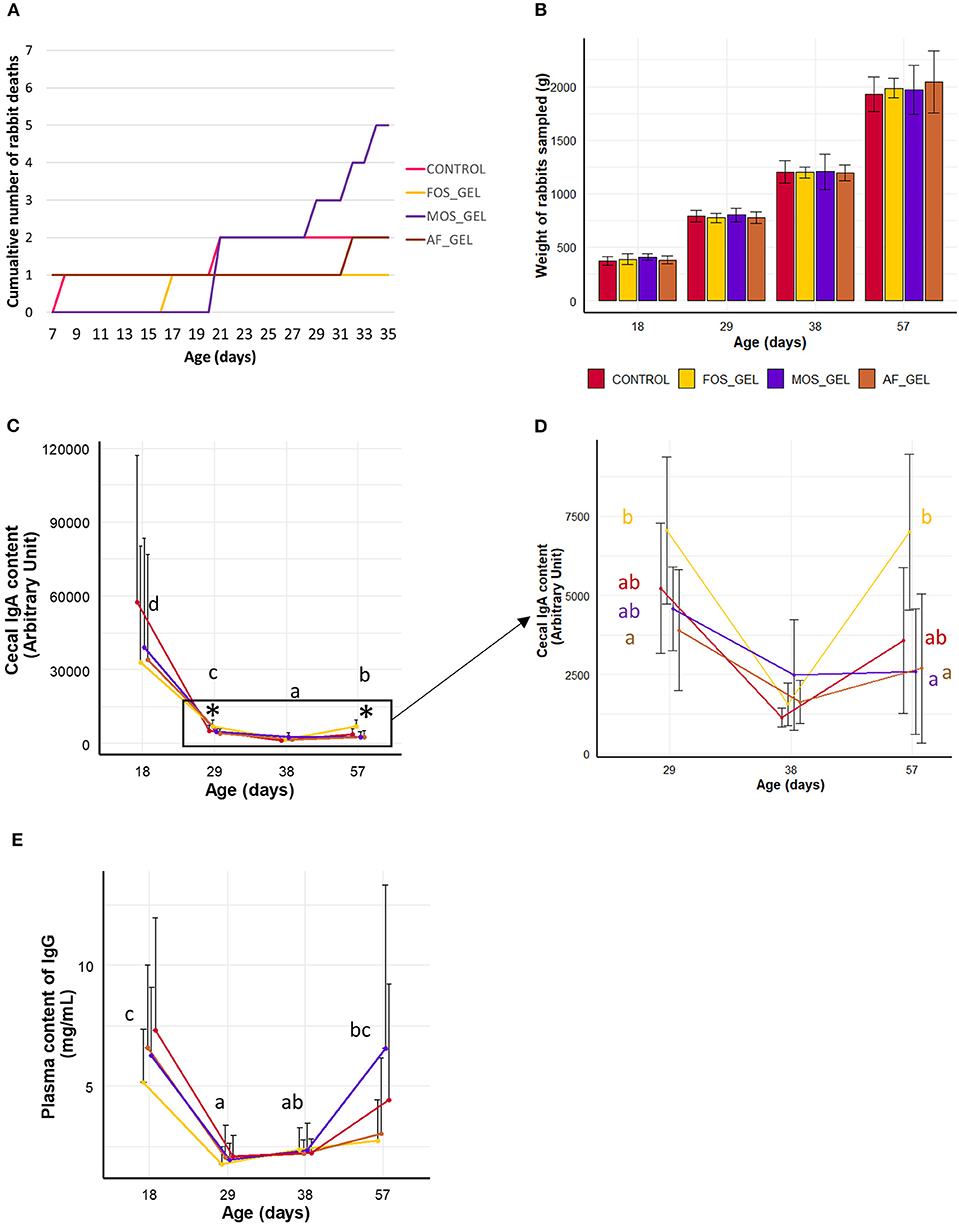
Figure 4. Health parameters and growth of the rabbits according to early dietary interventions in the nest in the form of a starter gel feed. (A) Mortality from 7 to 35 days of age. (B) Rabbit weight from 18 to 57 days. (C,D) Cecal IgA and (E) plasma IgG levels in rabbits. The animals sampled for immunoglobulin analyses were healthy and had comparable live weights between groups (B). Significant differences between experimental groups found within ages are represented with an asterisk. Black letters are meant to indicate age effects. Error bars represent standard deviation data. The additive composition of the gels varied according to treatments (CONTROL, no starter feed gel provided; FOS_GEL, fructo-oligosaccharides; MOS_GEL, a mixture of mannan-oligosaccharides and β-glucans; AF_GEL, no additive in the starter feed gel).
Effects of Starter Feed Gels and Prebiotic Supplementation on the Microbiota of Young Rabbits
We evaluated the effects of starter feed gel intake and prebiotics on gut microbiota diversity and composition in young rabbits. In addition to the expected increase of observed OTUs and diversity indexes (Shannon and InvSimpson) with age, no significant difference could be seen regarding early nutritional intervention at the different time points of the analysis (Table 2). For analyses at the OTU level, we focused on the earliest time point (i.e., day 18) corresponding to the end of the gel supply. A Venn diagram was built to represent the shared OTUs in each experimental group of 18-day-old rabbits based on the OTUs present in at least 75% of individuals of each group, e.g., representative of the core bacterial community at this age (Figure 5A). Among the 354 OTUs studied, 31% were shared among all the rabbit cecal ecosystems, which accounted for 82% of total relative abundances. Only five OTUs were specific to the CONTROL group, while the other groups exhibited more specific taxa patterns. Animals from FOS_GEL and MOS_GEL groups had 12 and 18% of specific OTUs, respectively, but the latter only represented 0.4 and 0.9% of total relative abundances, respectively. The 35 OTUs that were shared between groups with early nutritional interventions (FOS_GEL, MOS_GEL, and AF_GEL) accounted for 6% of relative abundances. As for the OTUs not present in the CONTROL group (219 OTUs), they accounted for 12% of total relative abundances. A PLS-DA procedure was performed to determine if experimental groups had their own microbial composition signature based on OTUs with relative abundances >0.5% (Figure 5B). The PLS-DA individual plot at 18 days suggested two clusters: CONTROL/MOS_GEL and FOS_GEL/AF_GEL (Figure 5B). Among the discriminant OTUs, OTU_61, affiliated to the Ruminococcus genus, was significantly higher in the FOS_GEL group than in the MOS_GEL group (P < 0.05), while OTU_48, affiliated to the Lachnospiraceae NK4A136 group, had a higher level in the AF_GEL than in the CONTROL and MOS_GEL groups (Figure 5C and Supplementary Figure S1). As expected, OTU taxonomic assignation revealed that the shift in gut microbiota composition was coincident with aging and increased levels of solid feed in rabbit diets, with a progressive decrease in Bacteroidetes and Proteobacteria phyla (resp. from 46 and 9% at 18 days to 9 and 1% at 57 days) and a sharp increase in the Firmicutes phylum (from 44% at 18 days to 89% at 57 days) (Figure 5D). The Tenericutes phylum tended to be affected by the experimental group (P = 0.08). A total of 99.5 and 68.1% of the sequences could be assigned at the family and genus levels, respectively. The proportions of families with relative abundances >0.5% were similar between the four experimental groups (Figure 5E). Overall, our results show that the stimulation of early life solid feed ingestion by starter feed gels had no effect on alpha diversity but seemed to allow the implantation of specific OTUs, some of them related to the prebiotic supplementation. However, their abundances in the ecosystems remained moderate.
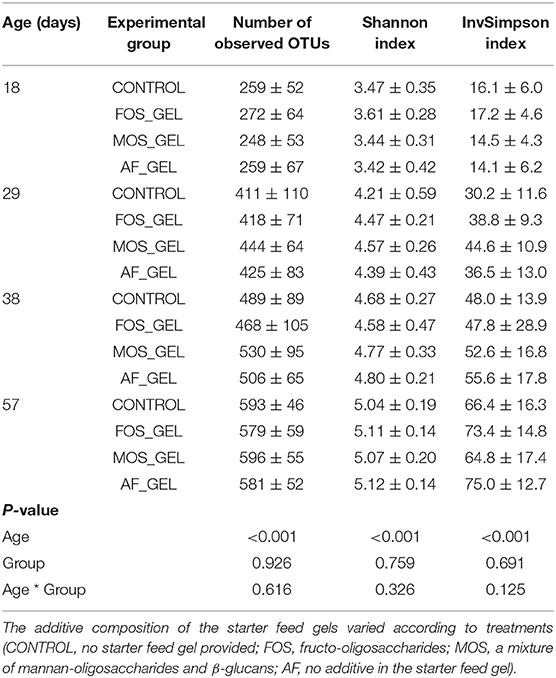
Table 2. Effects of early nutritional interventions on the alpha-diversity metrics of rabbit microbial cecum ecosystems (mean ± standard deviation).
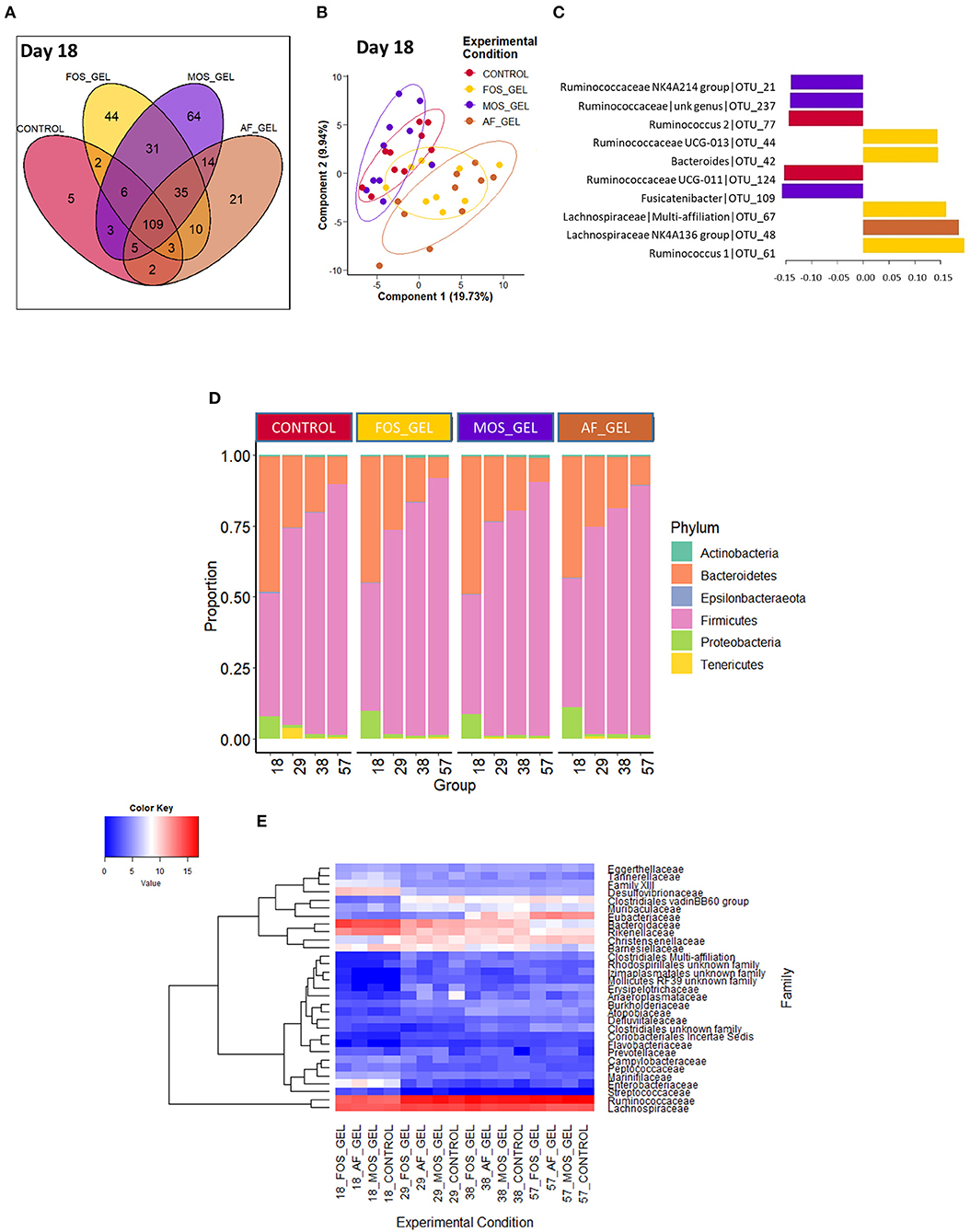
Figure 5. Venn diagram (A) occurrence of the OTUs across the cecal microbiota of 18-day-old rabbits according to early nutritional intervention in the form of a starter gel feed. The OTUs present in at least 75% of the samples within each group were kept to build the diagram. Partial least square discriminant analysis (PLS-DA, B,C) at the onset of early solid feed ingestion (d18) to classify samples according to the experimental group; 80% confidence ellipses are represented (B). Loadings of the 10 most discriminant OTUs according to component 1 (C). Phylum (D) and family (E) distributions according to early feed intake level. The heatmap (E) was built with rarefied count data after fourth root transformation. The additive composition of the gels varied according to treatments (FOS_GEL, fructo-oligosaccharides; MOS_GEL, a mixture of mannan-oligosaccharides and β-glucans; AF_GEL, no additive in the starter feed gel; CONTROL, no starter feed gel provided).
Effects of the Level of Early-Life Solid Feed Ingestion on the Microbiota of Young Rabbits
Based on our observation that the MOS_GEL group had the lowest solid feed intake compared to the FOS_GEL and AF_GEL groups and that its microbiota composition clustered with that of the CONTROL group with no gel supplementation, we hypothesized that ingestion levels in the nest were a structural factor of bacterial community implantation rather than our prebiotic supplementation. Consequently, we added a new categorial variable in our analysis based on the total starter feed intake in the nest of the litters sampled: it was either null (Null group, n = 10 litters), below the median (<3.8 g of fresh gel consumed/rabbit; n = 15), or above the median (>3.8 g; n = 15). One litter from the MOS_GEL group, five from the AF_GEL group and nine from the FOS_GEL group were classified with high early feed intake (“above the median”) (Supplementary Figure S2). Pellet intake in feeders was also the highest in rabbits from the “above the median” group at 18 and 21 days (+15 g of dry matter from 15 to 21 days) (Supplementary Figure S3).
A new Venn diagram was built based on the OTUs present in at least 75% of individuals in the three intake level groups (Figure 6A). This diagram clearly emphasized the presence of specific OTUs in relation to early solid feed ingestion. They represented 219 OTUs out of 354, with a higher number of OTUs belonging to the Ruminococcaceae family and a lower number belonging to the Bacteroidaceae family compared to the shared OTUs (χ2 = 4.95, df = 1, P = 0.026, and χ2 = 7.26, df = 1, P = 0.007, respectively; Figure 6B). Cumulated specific OTUs of the “above median” and “below median” groups represented 4.2 and 0.5%, respectively, of total relative abundances (Supplementary Figure S4). Subsequent PLS-DA two-component projections at 18 days (Figure 6C) suggested that individuals that consumed the highest quantities of gel in the nest are clustered in a separate group. Among the 22 discriminant OTUs (Figure 6D), OTU_67 and OTU_14, affiliated to the Lachnospiraceae family and the Ruminoclostridium 6 genus, respectively, exhibited the highest relative abundances in rabbits with the highest starter feed gel ingestion level in the nest (“above median” group) (Supplementary Figure S5). This clustering pattern was also observable at 29 days of age (Supplemental Figure S6). Interestingly, several days after weaning, the levels of early feed ingestion still affected microbiota composition. Indeed, supervised multivariate analysis at 38 and 57 days (Supplementary Figure S6) revealed that the three intake level groups still exhibited differences in their microbial communities. However, as of 29 days of age, PLS-DA total explained variance was low (≃10% on the two first components).
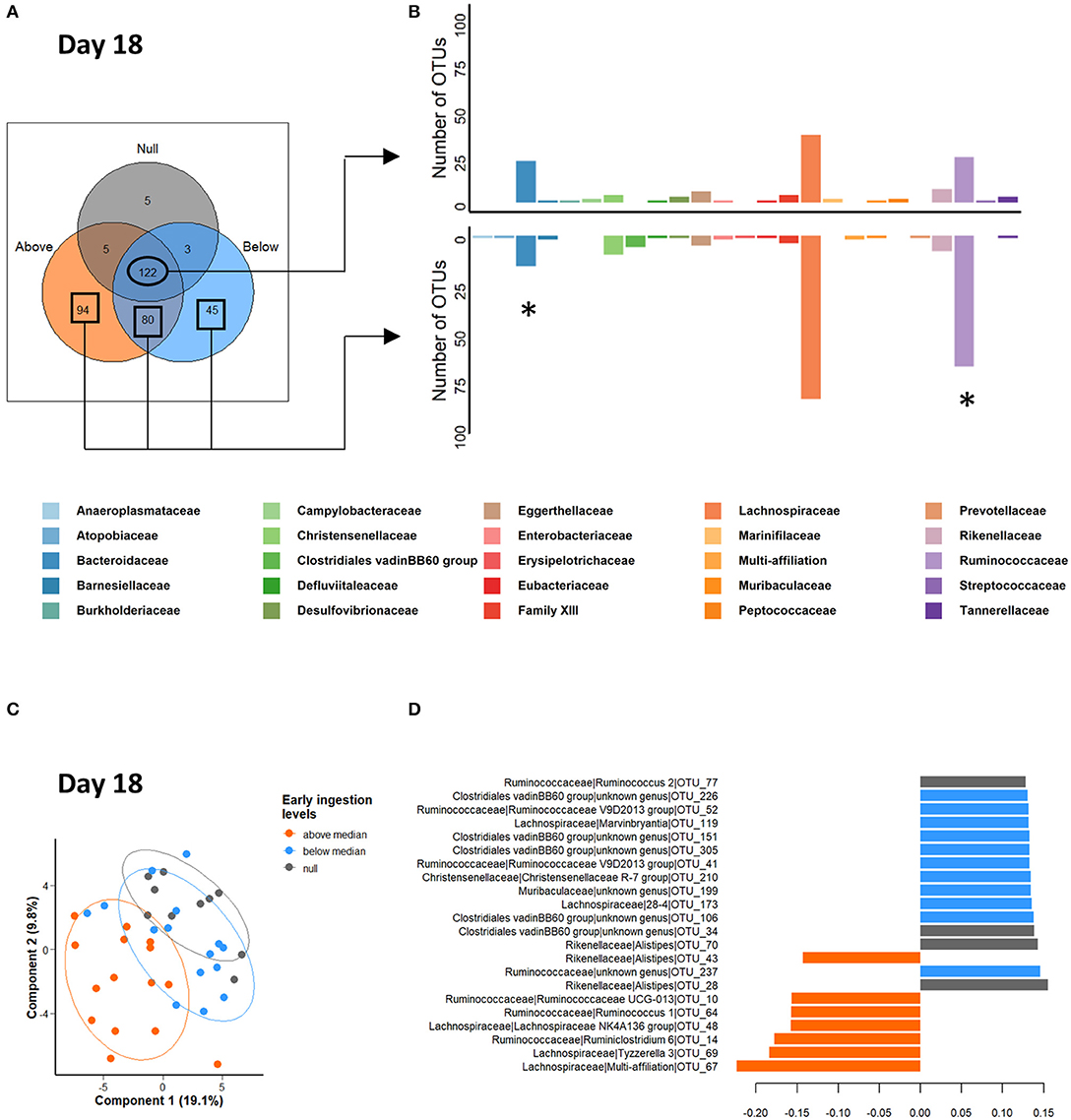
Figure 6. Venn diagram (A) occurrence of the OTUs across the cecal microbiota of 18-day-old rabbits according to starter feed gel ingestion level. The OTUs present in at least 75% of the samples within each group were kept to build the diagram. (B) Distribution among bacterial family affiliations of the OTUs shared by all the rabbits (upper barplot) and by the rabbits with a starter gel feed ingestion in the nest (lower barplot). The stars represent significant differences of Bacteroidaceae and Ruminococcaceae distributions. (C,D) Partial least square discriminant analysis at the onset of early solid feed ingestion (d18) to classify samples according to starter gel feed ingestion level; 80% confidence ellipses are represented (C). Loadings of the 22 discriminant OTUs according to component 1 (D). Null group: no feed intake corresponding to the 10 CONTROL group litters; “Below median group”: with an intake under 3.8 g of fresh gel consumed/rabbit (n = 15 litters); and “Above median” group: an intake over 3.8 g of fresh gel consumed/rabbit (n = 15 litters).
Analysis of the taxonomic assignation of OTUs revealed that the abundances of the Tenericutes phylum in the cecum of 29-day-old rabbits with no previous access to starter feed gels was 36 and 6 times greater than for rabbits with high (P < 0.001) and intermediate early feed intake (P = 0.02), respectively (Figure 7A). The distribution of the 32 taxonomic families in the rabbit cecum was similar regardless of early feed ingestion (Figure 7B) except for Prevotellaceae, which was more abundant at 29 days (P = 0.009) when suckling rabbits consumed large quantities of gels. At the genus level, only the Paraprevotella genus was affected by early feed intake levels, with a greater abundance in rabbits from the “above median” intake group (P = 0.02). Altogether, our results show that starter gel feed intake level was a better explanatory factor of differential microbial compositions up to 57 days than the original experimental groups. When rabbits consumed more starter feed, the highest proportions of bacteria with plant-degrading abilities, such as species from the Lachnospiraceae and Ruminococcaceae families, were observed.
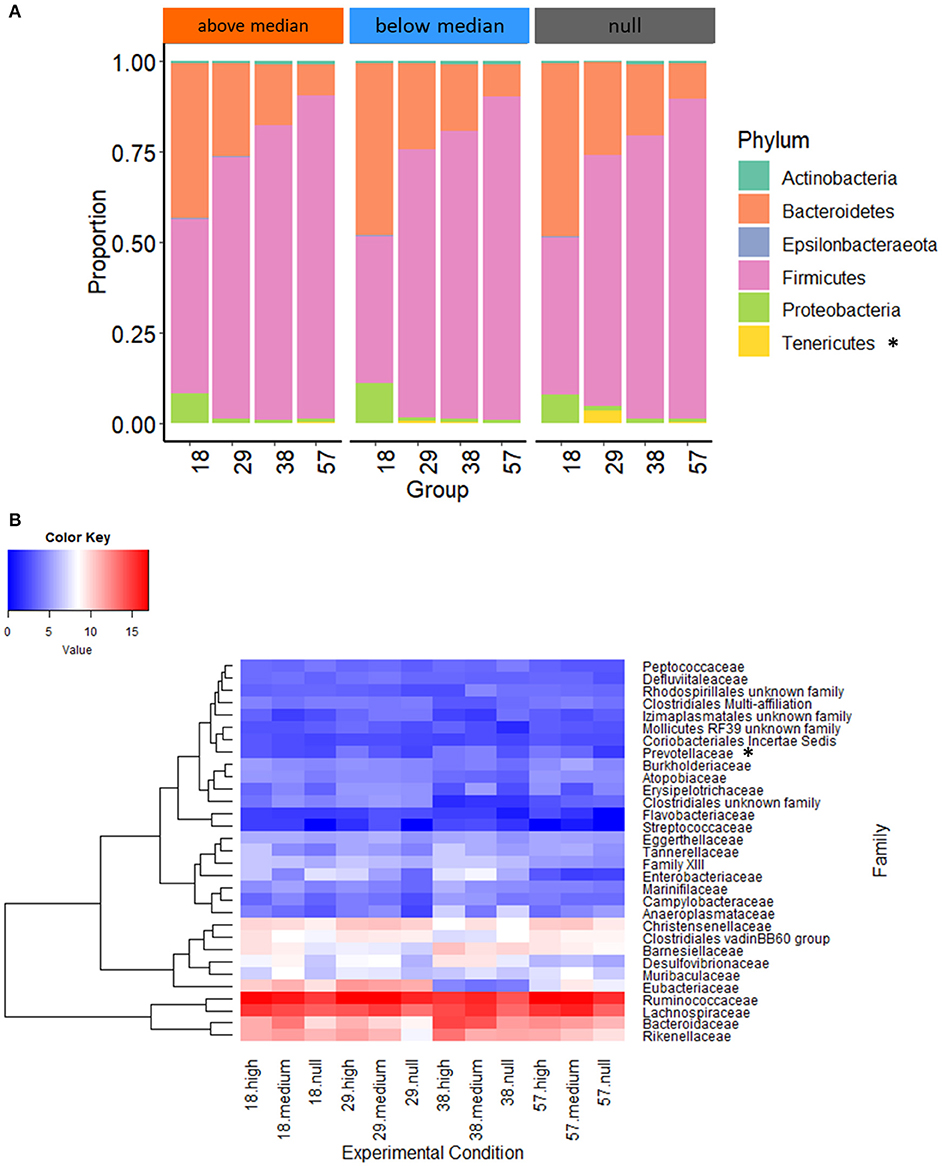
Figure 7. Phylum (A) and family (B) distributions according to early feed intake level. The heatmap (B) was built with rarefied count data after fourth root transformation. Null group: no feed intake corresponding to the 10 CONTROL group litters: “Below median” group: with an intake under 3.8 g of fresh gel consumed/rabbit (n = 15 litters); and “Above median” group: an intake over 3.8 g of fresh gel consumed/rabbit (n = 15 litters). Phyla or family with significant differences of distribution between experimental groups at one sampling date are followed by an asterisk.
Cecal Activity and Gut Morphology
No differences were observed between prebiotic supplementation groups or early feed intake level groups on cecal fermentative activity at 29, 38, and 57 days (Table 3). Gut characteristics, determined with cecum, cecal digesta, stomach, and intestine weights, did not vary according to prebiotic supplementation groups or early feed intake level groups (Supplementary Table S4).
Discussion
In young mammals, progressive implantation of the digestive microbiota initiates the microbiota-host dialogue that conditions the development and stimulation of the immune system (8, 47). This process is largely impacted by the substrate that arrives in the gut. The weaning transition, i.e., the dietary transition from a milk-based diet to a solid diet, shifts the microbial balance and contributes to digestive maturation (15, 16, 36). Managing this feed transition is therefore crucial for the construction of the animal's health and its future preservation. The purpose of our study was, on the one hand, to assess the effects of stimulation of early solid feed intake on rabbit microbiota establishment and, on the other, to determine if two selected prebiotics can be relevant tools to manipulate the developing microbiota. We demonstrated that the quantity of feed consumed in early life was a stronger driver of microbiota implantation than the nature of the prebiotic supplementation.
Stimulation of Early Feed Intake: Consequences on Rabbit Growth and Health
In most mammal organisms, the onset of a solid diet intake depends on their ability to exhibit feed prehension motor, mastication, and swallowing patterns (48). The beginning of solid feed intake is variable between species: for example, guinea pigs are able to process hard feed as of 1 day of age, whereas feeding activities begin at 18 days in rat pups (49). In commercial rabbit farms, rabbits start to consume feed at around 17–20 days, once they are mobile enough to leave the nest (50). However, rabbits are able to ingest solid substrates at an earlier age: wild rabbits are known to consume nest material 8 days after birth (17), and in a situation of choice between pellets differing in size or hardness, pups consumed them as of 8 days of age and expressed dietary preferences (21). Considering previous results on young rabbit feeding behavior (29), we designed a starter feed in a gel form that was rich in moisture and adapted to the suckling rabbit's physiological and physical constraints. It was supplemented with attractive vanilla flavor to take advantage of the fully-developed olfactory system of rabbit neonates (51, 52). This starter feed gel was provided in the nest with easy access in order to mimic the feed nibbling behavior observed in wild conditions. We opted to include prebiotics in the experimental starter feed based on their relevance to modify rabbit microbiota (23, 53) and phenotypic traits (54, 55), and based on the presence of oligosaccharides in milk as an essential driver of gastrointestinal microbiota development (56). Consequently, in one of the experimental groups, we provided FOS, which are known to stimulate bifidobacteria and lactobacilli growth but can also target other species from the Actinobacteria phylum or Olsenella genus, for example (57). In another group, we distributed MOS, which were found to block enteric pathogen colonization in numerous studies (58), in addition to the immunomodulating β-glucans (59).
In good agreement with the early feed intake kinetics previously described (21), feed intake started as early as 7 days of age and exponentially increased over time. This study confirmed that birth weight was a determining factor of early solid feed intake (29). Interestingly, the nature of the gels provided affected their intake in the nest, with higher ingestion of gels containing FOS compared to MOS. Prebiotics are known to modify sensory feed characteristics, and FOS additives contribute to a sweet taste similar to that of sucrose (60). Knowing that rabbits are attracted by bitter and sweet aromas (61), this taste difference probably explains the greater attractiveness of FOS gels. The taste of MOS highly depends on the type of product (distillery, brewery by-products or primary grown yeast). The MOS product used in this study was a yeast cell wall with a low protein content processed from primary fermentation, and with no specific flavor-contributing properties. Another hypothesis to explain ingestion level differences due to prebiotic additives may be linked to their effects on hormone production (62). These authors demonstrated that prebiotic supplementation in healthy humans was associated with an increase in plasmatic gut peptide concentrations that reduced appetite sensations.
To our knowledge, this study is the first one to highlight variations in cecal IgA content as a function of prebiotic supplementation in rabbits, although it has been well-described in infants who were fed formula containing prebiotics (63). IgA are essential for gut homeostasis since they represent first-line mucosal defenses by providing non-inflammatory immune protection (64). Microbiota modulation induced by starter feed intake could explain the effects on IgA content observed after weaning since some bacteria can induce the development of isolated lymphoid follicles (65). Further experiments are now necessary to determine if the higher levels of IgA after early FOS intake are due to the prebiotics themselves or the higher level of solids ingested in this group.
Attempts to Modulate Cecal Microbiota With Early Nutritional Interventions
When we tried to discriminate rabbit microbiota composition according to the prebiotic supplementation before weaning, we obtained unsuccessful partitioning. Nevertheless, when the analysis was performed by considering the variations in starter feed intake between litters rather than the nature of the prebiotics provided, better patterns were evidenced. Limited effects of the prebiotics used can be explained by the specificity of the suckling rabbit microbiota. Indeed, in the first 2 weeks of life, bacterial density and diversity in the cecum are low compared to older rabbits (10). Lactobacilli, a potential target of FOS, are rare inhabitants of the digestive tract in rabbits and, even if their growth is stimulated, they poorly adhere to rabbit intestinal epithelial cells (18, 66). Moreover, we did not infect the animals with enteric pathogens during this study whose sanitary conditions were good, which could explain why MOS effects on the adhesion of enteropathogenic bacteria were not evidenced. Thus, the young rabbit gut may not be a relevant bacterial reservoir for the prebiotics used, which need to target specific indigenous microorganisms to be effective. Moreover, it should be pointed out that rabbit diets are naturally rich in fibrous ingredients, some of them having significant amounts of oligosaccharides (67), which could have hidden effects of prebiotic treatments.
Early-life solid feed consumption was associated with 219 specific OTUs at 18 days of age, which mainly belonged to the Lachnospiraceae and Ruminococcaceae families. Members of those families are efficient fermenters of complex plant materials such as cellulose and have been associated with gut health maintenance (68). The role of some Lachnospiraceae strains in butyrate production was demonstrated in vivo, a function that can have subsequent protective health effects, notably through colonic Treg induction (69). We observed that high proportions of the Paraprevotella genus were also associated with high consumption of starter feeds. One of the two species described from this genus can break down the xylans in plant cell walls to produce succinate and acetate (70). Taken together, those findings may indicate that the small amounts of feed ingested at the onset of a solid diet are sufficient to prepare the weaning transition by selecting bacteria able to ferment plant-based diets with end products such as butyrate, a well-known promoter of the epithelial barrier (71).
It was observed that an early nutritional intervention had long-term consequences with differential microbiota structures up to 39 days afterwards. We can hypothesize that those subsequent modifications are related to the increase of pellet intake in the feeders during the middle nursing period, induced by greater starter feed consumption. This phenomenon has been well-described in pig farming systems (72) where suckling pigs offered starter feed in a liquid or gruel form in addition to milk increased pellet intake later during the nursing period. Since a similar phenomenon was observed in suckling rabbits (29) and given the impact of solid feed on bacterial community structures (15, 73), this posterior effect of early feed intake stimulation on feeding behavior appears interesting and should be further explored.
Despite some changes in cecal ecosystems induced by the amount of starter feed that reached the gut, it is worthwhile noting that no changes in cecal fermentation activity were observed. The reason for this lack of functional modifications could be the functional redundancy within the microbiome since different bacterial populations within a community can perform the same functions (74). The fact that alpha and beta diversity were not modified by early feed intake stimulation and that limited effects on taxonomic profiles were revealed could also be possible explanations for the lack of functional changes in the cecum. It is also likely that few effects were observed due to predominant milk intake in the young rabbit's diet up to 25–30 days (50). In a previous experiment, we estimated that a total pellet intake of 1.8 g of fresh matter in the nest from 8 to 17 days only amounted to 1.3% of the total milk intake over this period (21). Thus, large amounts of milk may have “diluted” the effects of solid supplementation since rabbit milk constrains bacterial community structures (36). Indeed, mammals' milk contains its own microbiota, prebiotics (oligosaccharides), immunoglobulins, and other microbiota-shaping compounds such as antimicrobial casein-derived peptides or lipids (75, 76) that probably induce a selective pressure. This dominant impact of milk consumption was shown in human cohort studies where the duration of exclusive breastfeeding was a stronger driver for microbial diversity at 9 months of age than the time when solid food was introduced into the infant's diet (77). In accordance, previous attempts to modulate rabbit microbiota before weaning with diet change (36, 78); had moderate effects, possibly due to milk constraint. Another possible explanation for early dietary intervention limitations is the heterogeneity of the solid intake in the nest within litters. Competition for feed might have occurred between rabbits, as observed during nursing (79), and similarly with pigs whose variability in individual starter feed intake was demonstrated with a stool marker (80). In our trial, rabbits were randomly sampled due to the absence of a methodology to determine “good eater” rabbit up until now.
Conclusion
Starter feed provided in a gel form was accepted by suckling rabbit pups with intake differences depending on the type of gels offered. Gels preferably consumed in the nest were associated with increased solid feed intake later in life. Supplementation with prebiotics in early life did not have a notable effect on the gut microbiota of suckling rabbits before and after weaning. When considering the amounts of starter feed ingested instead of the nature of the prebiotics consumed, more pronounced effects on bacterial composition could be observed. Increased quantities of feed consumed at an early age seemed to promote the development of microorganisms adapted to plant degradation, which could efficiently prepare the rabbits for weaning transition. Further studies are now necessary to identify optimal gut-shaping starter feeds and to confirm subsequent effects on ecosystem functionalities and gut health.
Data Availability Statement
All raw sequences were deposited in the NCBI Sequence Read Archive (SRA) under accession number PRJNA589727.
Ethics Statement
This animal study was reviewed and approved by Science et Sante Animales No. 115, Ecole Nationale Vétérinaire, France.
Author Contributions
CP, TG, KB, JD, CG, EG-G, GR, and SC conceived and designed the experiments. CP, OB, CB, and PA carried out and planned the experiments. CP and SC analyzed the data and wrote the manuscript.
Funding
This work was funded by the Institut Carnot Sciences Animales (ICSA, France, project eFeedIT-ICSA-2015).
Conflict of Interest
CP was employed by the company CCPA for the Groupe d'Experimentation Cunicole network (French network of companies that includes CCPA, EVIALIS, MiXscience, TECHNA, and Wisium). KB was employed by the company CCPA. JD was employed by the company EVIALIS. CG was employed by the company MiXscience. GR was employed by the company TECHNA. EG-G was employed by the company Wisium.
The remaining authors declare that the research was conducted in the absence of any commercial or financial relationships that could be construed as a potential conflict of interest.
Acknowledgments
The authors gratefully acknowledge A. M. Debrusse, M. Moulis, and J. M. Bonnemere for their assistance at the rabbit experimental unit, as well as M. Segura and I. Ahn who contributed to sample preparation, C. Bannelier who performed the feed analysis, and S. Levade who contributed to ELISA dosages. They also would like to thank C. Achard, M. Beaumont, C. Knudsen, M. Castex, and E. Apper for their valuable remarks regarding data analysis and their careful proofreading. The authors are grateful to the Genotoul bioinformatics platform Toulouse Midi-Pyrenees and the Sigenae group for providing computing and storage resources thanks to Galaxy instance http://sigenae-workbench.toulouse.inra.fr. The authors are grateful to G. Wagman for her correction of the English spelling.
Supplementary Material
The Supplementary Material for this article can be found online at: https://www.frontiersin.org/articles/10.3389/fvets.2020.00261/full#supplementary-material
Abbreviations
AF, additive-free; DM, dry matter; FOS, fructo-oligosaccharides; MOS, mannan-oligosaccharides; OUT, operational taxonomic units; PLS-DA, partial least squares discriminant analysis; VFA, volatile fatty acids.
References
1. Bäuerl C, Collado MC, Zúñiga M, Blas E, Martínez GP. Changes in cecal microbiota and mucosal gene expression revealed new aspects of epizootic rabbit enteropathy. PLoS ONE. (2014) 9:e105707. doi: 10.1371/journal.pone.0105707
2. Argüello H, Estellé J, Zaldívar-López S, Jiménez-Marín Á, Carvajal A, López-Bascón MA, et al. Early Salmonella Typhimurium infection in pigs disrupts microbiome composition and functionality principally at the ileum mucosa. Sci Rep. (2018) 8:7788. doi: 10.1038/s41598-018-26083-3
3. Jin DX, Zou HW, Liu SQ, Wang LZ, Xue B, Wu D, et al. The underlying microbial mechanism of epizootic rabbit enteropathy triggered by a low fiber diet. Sci Rep. (2018) 8:12489. doi: 10.1038/s41598-018-30178-2
4. Sekirov I, Finlay BB. The role of the intestinal microbiota in enteric infection. J Physiol. (2009) 587:4159–67. doi: 10.1113/jphysiol.2009.172742
5. Kamada N, Chen GY, Inohara N, Núñez G. Control of pathogens and pathobionts by the gut microbiota. Nat Immunol. (2013) 14:685–90. doi: 10.1038/ni.2608
6. Hooper LV, Littman DR, Macpherson AJ. Interactions between the microbiota and the immune system. Science. (2012) 336:1268–73. doi: 10.1126/science.1223490
7. Olszak T, An D, Zeissig S, Vera MP, Richter J, Franke A, et al. Microbial exposure during early life has persistent effects on natural killer T cell function. Science. (2012) 336:489–93. doi: 10.1126/science.1219328
8. Al Nabhani Z, Dulauroy S, Marques R, Cousu C, Al Bounny S, Déjardin F, et al. A weaning reaction to microbiota is required for resistance to immunopathologies in the adult. Immunity. (2019) 50:1276–88.e5. doi: 10.1016/j.immuni.2019.02.014
9. Yatsunenko T, Rey FE, Manary MJ, Trehan I, Dominguez-Bello MG, Contreras M, et al. Human gut microbiome viewed across age and geography. Nature. (2012) 486:222–7. doi: 10.1038/nature11053
10. Combes S, Michelland RJ, Monteils V, Cauquil L, Soulie V, Tran NU, et al. Postnatal development of the rabbit caecal microbiota composition and activity. FEMS Microbiol Ecol. (2011) 77:680–9. doi: 10.1111/j.1574-6941.2011.01148.x
11. Motta V, Luise D, Bosi P, Trevisi P. Faecal microbiota shift during weaning transition in piglets and evaluation of AO blood types as shaping factor for the bacterial community profile. PLoS ONE. (2019) 14:e0217001. doi: 10.1371/journal.pone.0217001
12. Rey M, Enjalbert F, Combes S, Cauquil L, Bouchez O, Monteils V. Establishment of ruminal bacterial community in dairy calves from birth to weaning is sequential. J Appl Microbiol. (2014) 116:245–57. doi: 10.1111/jam.12405
13. Torow N, Hornef MW. The neonatal window of opportunity: setting the stage for life-long host-microbial interaction and immune homeostasis. J Immunol. (2017) 198:557–63. doi: 10.4049/jimmunol.1601253
14. Padilha MTS, Licois D, Gidenne T, Carré B. Caecal microflora and fermentation pattern in exclusively milk-fed young rabbits. Reprod Nutr Dev. (1999) 39:223–30. doi: 10.1051/rnd:19990207
15. Koenig JE, Spor A, Scalfone N, Fricker AD, Stombaugh J, Knight R, et al. Succession of microbial consortia in the developing infant gut microbiome. Proc Natl Acad Sci USA. (2011) 108(Suppl. 1):4578–85. doi: 10.1073/pnas.1000081107
16. Bian G, Ma S, Zhu Z, Su Y, Zoetendal EG, Mackie R, et al. Age, introduction of solid feed and weaning are more important determinants of gut bacterial succession in piglets than breed and nursing mother as revealed by a reciprocal cross-fostering model. Environ Microbiol. (2016) 18:1566–77. doi: 10.1111/1462-2920.13272
17. Hudson R, Distel H. The pattern of behaviour of rabbit pups in the nest. Behaviour. (1982) 79:255–71. doi: 10.1163/156853982X00292
18. Kovács M, Szendrõ Zs, Milisits G, Biro-Nemeth E, Radnai I, Posa R, et al. Effect of nursing method and faeces consumption on the development of bacetroides, lactobacillus and coliform flora in the caecum of the newborn rabbits. Reprod Nutr Dev. (2006) 46:205–10. doi: 10.1051/rnd:2006010
19. Combes S, Gidenne T, Cauquil L, Bouchez O, Fortun-Lamothe L. Coprophagous behavior of rabbit pups affects implantation of cecal microbiota and health status. J Anim Sci. (2014) 92:652–65. doi: 10.2527/jas.2013-6394
20. Kacsala L, Szendro Z, Gerencsér Z, Radnai I, Kovács M, Kasza R, et al. Early solid additional feeding of suckling rabbits from 3 to 15 days of age. Animal. (2018) 12:28–33. doi: 10.1017/S1751731117001367
21. Paës C, Fortun-Lamothe L, Bébin K, Duperray J, Gohier C, Guené-Grand E, et al. Onset of feed intake of the suckling rabbit and evidence of dietary preferences according to pellet physical properties. Anim Feed Sci Technol. (2019) 255:114223. doi: 10.1016/j.anifeedsci.2019.114223
22. Bovera F, Lestingi A, Marono S, Iannaccone F, Nizza S, Mallardo K, et al. Effect of dietary mannan-oligosaccharides on in vivo performance, nutrient digestibility and caecal content characteristics of growing rabbits. J Anim Physiol Anim Nutr. (2012) 96:130–6. doi: 10.1111/j.1439-0396.2011.01134.x
23. Oso AO, Idowu OMO, Haastrup AS, Ajibade AJ, Olowonefa KO, Aluko AO, et al. Growth performance, apparent nutrient digestibility, caecal fermentation, ileal morphology and caecal microflora of growing rabbits fed diets containing probiotics and prebiotics. Livest Sci. (2013) 157:184–90. doi: 10.1016/j.livsci.2013.06.017
24. Volek Z, Marounek M, Skrivanova V. Effect of a starter diet supplementation with mannan-oligosaccharide or inulin on health status, caecal metabolism, digestibility of nutrients and growth of early weaned rabbits. Animal. (2007) 1:523–30. doi: 10.1017/S1751731107685012
25. Coppa GV, Bruni S, Morelli L, Soldi S, Gabrielli O. The first prebiotics in humans: human milk oligosaccharides. J Clin Gastroenterol. (2004) 38(Suppl. 6):S80–3. doi: 10.1097/01.mcg.0000128926.14285.25
26. Maertens L, Lebas F, Szendro ZS. Rabbit milk: a review of quantity, quality and non-dietary affecting factors. World Rabbit Sci. (2010) 14:205–30. doi: 10.4995/wrs.2006.565
27. Bode L. Human milk oligosaccharides: every baby needs a sugar mama. Glycobiology. (2012) 22:1147–62. doi: 10.1093/glycob/cws074
28. Verkhnyatskaya S, Ferrari M, de Vos P, Walvoort MTC. Shaping the infant microbiome with non-digestible carbohydrates. Front Microbiol. (2019) 10:343. doi: 10.3389/fmicb.2019.00343
29. Paës C, Fortun-Lamothe L, Coureaud G, Bébin K, Duperray J, Gohier C, et al. Insights into suckling rabbit feeding behaviour: acceptability of different creep feed presentations and attractiveness for sensory feed additives. Animal. (2020) 1–9. doi: 10.1017/S1751731120000038. [Epub ahead of print].
30. Fortun-Lamothe L, Gidenne T, Lapanouse A, De Dapper J. Technical note : an original system to separately control litter and female feed intake without modification of the mother - young relations. World Rabbit Sci. (2000) 8:177–180. doi: 10.4995/wrs.2000.708
31. Gidenne T, Combes S, Fortun-Lamothe L. Feed intake limitation strategies for the growing rabbit: effect on feeding behaviour, welfare, performance, digestive physiology and health: a review. Animal. (2012) 6:1407–19. doi: 10.1017/S1751731112000389
32. EGRAN. Technical note: attempts to harmonise chemical analyses of feeds and faeces, for rabbit feed evaluation. World Rabbit Sci. (2001) 9:57–64. doi: 10.4995/wrs.2001.446
33. Verdouw H, van Echteld CJA, Dekkers EMJ. Ammonia determination based on indophenol formation with sodium salicylate. Water Res. (1977) 12:399–402. doi: 10.1016/0043-1354(78)90107-0
34. Filípek J, Dvorák R. Determination of the volatile fatty acid content in the rumen liquid: comparison of gas chromatography and capillary isotachophoresis. Acta Vet Brno. (2009) 78:627–33. doi: 10.2754/avb200978040627
35. Beaumont M, Paës C, Mussard E, Knudsen C, Cauquil L, Aymard P, et al. Gut microbiota derived metabolites contribute to intestinal barrier maturation at the suckling-to-weaning transition. Gut Microbes. (2020) 1–19. doi: 10.1080/19490976.2020.1747335. [Epub ahead of print].
36. Read T, Fortun-Lamothe L, Pascal G, Le Boulch M, Cauquil L, Gabinaud B, et al. Diversity and co-occurrence pattern analysis of cecal microbiota establishment at the onset of solid feeding in young rabbits. Front Microbiol. (2019) 10:973. doi: 10.3389/fmicb.2019.00973
37. Escudié F, Auer L, Bernard M, Mariadassou M, Cauquil L, Vidal K, et al. FROGS: find, rapidly, OTUs with galaxy solution. Bioinformatics. (2018) 34:1287–94. doi: 10.1093/bioinformatics/btx791
38. Mahé F, Rognes T, Quince C, Vargas C, Dunthorn M. Swarm: robust and fast clustering method for amplicon-based studies. PeerJ. (2014) 2:e593. doi: 10.7717/peerj.593
39. Rognes T, Flouri T, Nichols B, Quince C, Mahé F. VSEARCH: a versatile open source tool for metagenomics. PeerJ. (2016) 4:e2584. doi: 10.7717/peerj.2584
40. Quast C, Pruesse E, Yilmaz P, Gerken J, Schweer T, Yarza P, et al. The SILVA ribosomal RNA gene database project: improved data processing and web-based tools. Nucleic Acids Res. (2013) 41:D590–6. doi: 10.1093/nar/gks1219
41. McMurdie PJ, Holmes S. phyloseq: an R package for reproducible interactive analysis and graphics of microbiome census data. PLoS ONE. (2013) 8:e61217. doi: 10.1371/journal.pone.0061217
42. Benson AK, Kelly SA, Legge R, Ma F, Low SJ, Kim J, et al. Individuality in gut microbiota composition is a complex polygenic trait shaped by multiple environmental and host genetic factors. Proc Natl Acad Sci USA. (2010) 107:18933–8. doi: 10.1073/pnas.1007028107
43. Rohart F, Gautier B, Singh A, Cao K-AL. mixOmics: an R package for ‘omics feature selection and multiple data integration. PLoS Comput Biol. (2017) 13:e1005752. doi: 10.1371/journal.pcbi.1005752
44. Donner A, Banting D. Adjustment of frequently used chi-square procedures for the effect of site-to-site dependencies in the analysis of dental data. J Dent Res. (1989) 68:1350–4. doi: 10.1177/00220345890680091201
45. Reed JF. Adjusted chi-square statistics: application to clustered binary data in primary care. Ann Fam Med. (2004) 2:201–3. doi: 10.1370/afm.41
46. Princée FPG. Exploring Studbooks for Wildlife Management and Conservation. London Springer International Publishing (2016). p. 291. doi: 10.1007/978-3-319-50032-4
47. Mage RG, Lanning D, Knight KL. B cell and antibody repertoire development in rabbits: the requirement of gut-associated lymphoid tissues. Dev Comp Immunol. (2006) 30:137–53. doi: 10.1016/j.dci.2005.06.017
48. Bels VL. Feeding in Domestic Vertebrates: From Structure to Behaviour. Wallingford: CABI Publishing (2006). p. 384. doi: 10.1079/9781845930639.0000
49. German RZ, Crompton AW, Thexton AJ. Ontogeny of feeding in mammals. In Bels V, editor. Feeding in Domestic Vertebrates: from Structure to Behavior. Wallingford: CABI Publishing (2006). p. 50–60. doi: 10.1079/9781845930639.0050
50. Gidenne T, Lebas F, Fortun-Lamothe L. Feeding behaviour of rabbits. In: De Blas C, Wiseman J, editors. Nutrition of the Rabbit. CABI Publishing. Wallingford: CABI Publishing (2010). p. 233–252. doi: 10.1079/9781845936693.0233
51. Coureaud G, Schaal B, Langlois D, Perrier G. Orientation response of newborn rabbits to odours of lactating females: relative effectiveness of surface and milk cues. Anim Behav. (2001) 61:153–62. doi: 10.1006/anbe.2000.1563
52. Schaal B, Coureaud G, Langlois D, Giniès C, Sémon E, Perrier G. Chemical and behavioural characterization of the rabbit mammary pheromone. Nature. (2003) 424:68–72. doi: 10.1038/nature01739
53. Bovera F, Nizza S, Marono S, Mallardo K, Piccolo G, Tudisco R, et al. Effect of mannan oligosaccharides on rabbit performance, digestibility and rectal bacterial anaerobic populations during an episode of epizootic rabbit enteropathy. World Rabbit Sci. (2010) 18:9–16. doi: 10.4995/WRS.2010.18.02
54. Attia YA, Hamed RS, Abd El-Hamid AE, Al-Harthi MA, Shahba HA, Bovera F. Performance, blood profile, carcass and meat traits and tissue morphology in growing rabbits fed mannanoligosaccharides and zinc-bacitracin continuously or intermittently. Anim Sci Pap Rep. (2015) 33:85–101.
55. Attia YA, Hamed RS, El-Hamid AEA, Shahba HA, Bovera F. Effect of inulin and mannan-oligosaccharides compared with zinc-bacitracin on growing performance, nutrient digestibility and hematological profiles of growing rabbits. Anim Prod Sci. (2016) 55:80–6. doi: 10.1071/AN13286
56. Borewicz K, Suarez-Diez M, Hechler C, Beijers R, Weerth C, Arts I, et al. The effect of prebiotic fortified infant formulas on microbiota composition and dynamics in early life. Sci. Rep. (2019) 9:2434. doi: 10.1038/s41598-018-38268-x
57. Mao B, Li D, Zhao J, Liu X, Gu Z, Chen YQ, et al. Metagenomic insights into the effects of fructo-oligosaccharides (FOS) on the composition of fecal microbiota in mice. J Agric Food Chem. (2015) 63:856–63. doi: 10.1021/jf505156h
58. Halas V, Nochta I. Mannan oligosaccharides in nursery pig nutrition and their potential mode of action. Animals. (2012) 2:261–74. doi: 10.3390/ani2020261
59. Kogan G, Kocher A. Role of yeast cell wall polysaccharides in pig nutrition and health protection. Livest Sci. (2007) 109:161–5. doi: 10.1016/j.livsci.2007.01.134
60. Yun JW. Fructooligosaccharides—Occurrence, preparation, and application. Enzyme Microb Tech. (1996) 19:107–17. doi: 10.1016/0141-0229(95)00188-3
61. Gidenne T. Le Lapin. De la biologie à l'élevage. Versailles: Quae éditions. (2015). p. 288. doi: 10.35690/978-2-7592-2418-0
62. Cani PD, Lecourt E, Dewulf EM, Sohet FM, Pachikian BD, Naslain D, et al. Gut microbiota fermentation of prebiotics increases satietogenic and incretin gut peptide production with consequences for appetite sensation and glucose response after a meal. Am J Clin Nutr. (2009) 90:1236–43. doi: 10.3945/ajcn.2009.28095
63. Scholtens PAMJ, Alliet P, Raes M, Alles MS, Kroes H, Boehm G, et al. Fecal secretory immunoglobulin A is increased in healthy infants who receive a formula with short-chain galacto-oligosaccharides and long-chain fructo-oligosaccharides. J Nutr. (2008) 138:1141–7. doi: 10.1093/jn/138.6.1141
64. Pérez-Berezo T, Franch A, Ramos-Romero S, Castellote C, Pérez-Cano FJ, Castell M. Cocoa-enriched diets modulate intestinal and systemic humoral immune response in young adult rats. Mol Nutr Food Res. (2011) 55:S56–66. doi: 10.1002/mnfr.201000588
65. Bouskra D, Brézillon C, Bérard M, Werts C, Varona R, Boneca IG, et al. Lymphoid tissue genesis induced by commensals through NOD1 regulates intestinal homeostasis. Nature. (2008) 456:507–10. doi: 10.1038/nature07450
66. Yu B, Tsen HY. Lactobacillus cells in the rabbit digestive tract and factors affecting their distribution. J Appl Bact. (1993) 75:269–75. doi: 10.1111/j.1365-2672.1993.tb02776.x
67. Falcão E, Cunha L, Castro-Solla L, Maertens L, Marounek M, Pinheiro V, Freire J, et al. Alternatives to antibiotic growth promoters in rabbit feeding: a review. World Rabbit Sci. (2007) 15:127–40. doi: 10.4995/wrs.2007.597
68. Biddle A, Stewart L, Blanchard J, Leschine S. Untangling the genetic basis of fibrolytic specialization by Lachnospiraceae and Ruminococcaceae in diverse gut communities. Diversity. (2013) 5:627–40. doi: 10.3390/d5030627
69. Narushima S, Sugiura Y, Oshima K, Atarashi K, Hattori M, Suematsu M, et al. Characterization of the 17 strains of regulatory T cell-inducing human-derived clostridia. Gut Microbes. (2014) 5:333–9. doi: 10.4161/gmic.28572
70. Morotomi M, Nagai F, Sakon H, Tanaka R. Paraprevotella clara gen. nov., sp. nov. and Paraprevotella xylaniphila sp. nov., members of the family “Prevotellaceae” isolated from human faeces. Int J Syst Evol Micrbiol. (2009) 59:1895–900. doi: 10.1099/ijs.0.008169-0
71. Zheng L, Kelly CJ, Battista KD, Schaefer R, Lanis JM, Alexeev EE, et al. Microbial-derived butyrate promotes epithelial barrier function through IL-10 receptor-dependent repression of Claudin-2. J Immunol. (2017) 199:2976–84. doi: 10.4049/jimmunol.1700105
72. Pluske JR, Turpin DL, Kim JC. Gastrointestinal tract (gut) health in the young pig. Anim Nutr. (2018) 4:187–96. doi: 10.1016/j.aninu.2017.12.004
73. de Rodas B, Youmans BP, Danzeisen JL, Tran H, Johnson TJ. Microbiome profiling of commercial pigs from farrow to finish. J Anim Sci. (2018) 96:1778–94. doi: 10.1093/jas/sky109
74. Heintz-Buschart A, Wilmes P. Human gut microbiome: function matters. Trends Microbiol. (2018) 26:563–74. doi: 10.1016/j.tim.2017.11.002
75. Baranyi M, Thomas U, Pellegrini A. Antibacterial activity of casein-derived peptides isolated from rabbit (Oryctolagus cuniculus) milk. J Dairy Res. (2003) 70:189–97. doi: 10.1017/S0022029903006150
76. van den Elsen LWJ, Garssen J, Burcelin R, Verhasselt V. Shaping the gut microbiota by breastfeeding: the gateway to allergy prevention? Front Pediatr. (2019) 7:47. doi: 10.3389/fped.2019.00047
77. Laursen MF, Andersen LBB, Michaelsen KF, Mølgaard C, Trolle E, Bahl MI, et al. Infant gut microbiota development is driven by transition to family foods independent of maternal obesity. mSphere. (2016) 1:e00069-15. doi: 10.1128/mSphere.00069-15
78. Xiccato G, Trocino A, Sartori A, Queaque PI. Effect of weaning diet and weaning age on growth, body composition and caecal fermentation of young rabbits. Anim Sci. (2003) 77:101–11. doi: 10.1017/S1357729800053704
79. Coureaud G, Schaal B, Coudert P, Rideaud P, Fortun-Lamothe L, Hudson R, et al. Immediate postnatal suckling in the rabbit: its influence on pup survival and growth. Reprod Nutr Dev. (2000) 40:19–32. doi: 10.1051/rnd:2000117
80. Bruininx EMAM, Schellingerhout AB, Binnendijk GP, Peet-Schwering CMC, Schrama JW, Hartog LA, et al. Individually assessed creep food consumption by suckled piglets: influence on post-weaning food intake characteristics and indicators of gut structure and hind-gut fermentation. Anim Sci. (2004) 78:67–75. doi: 10.1017/S1357729800053856
Keywords: early life, rabbit, microbiome, weaning, gel starter diet, oligosaccharide, FOS, MOS
Citation: Paës C, Gidenne T, Bébin K, Duperray J, Gohier C, Guené-Grand E, Rebours G, Bouchez O, Barilly C, Aymard P and Combes S (2020) Early Introduction of Solid Feeds: Ingestion Level Matters More Than Prebiotic Supplementation for Shaping Gut Microbiota. Front. Vet. Sci. 7:261. doi: 10.3389/fvets.2020.00261
Received: 09 March 2020; Accepted: 20 April 2020;
Published: 15 May 2020.
Edited by:
Vincenzo Tufarelli, University of Bari Aldo Moro, ItalyReviewed by:
Youssef A. Attia, King Abdulaziz University, Saudi ArabiaMohamed E. Abd El-Hack, Zagazig University, Egypt
Copyright © 2020 Paës, Gidenne, Bébin, Duperray, Gohier, Guené-Grand, Rebours, Bouchez, Barilly, Aymard and Combes. This is an open-access article distributed under the terms of the Creative Commons Attribution License (CC BY). The use, distribution or reproduction in other forums is permitted, provided the original author(s) and the copyright owner(s) are credited and that the original publication in this journal is cited, in accordance with accepted academic practice. No use, distribution or reproduction is permitted which does not comply with these terms.
*Correspondence: Sylvie Combes, c3lsdmllLmNvbWJlc0BpbnJhZS5mcg==