- 1Tianjin Key Laboratory of Conservation and Utilization of Animal Diversity, College of Life Sciences, Tianjin Normal University, Tianjin, China
- 2Tianjin Key Laboratory of Agricultural Animal Breeding and Healthy Husbandry, College of Animal Science and Veterinary Medicine, Tianjin Agricultural University, Tianjin, China
- 3College of Life Sciences, Tianjin Institute of Animal Husbandry and Veterinary Medicine, Tianjin, China
Intestinal health is a critical issue for piglets during their weaning transition period. Previous reports have emphasized the promise of distinct probiotics in improving the enteric health. Here in this research, a newly isolated Lactobacillus salivarius strain was pretreated to Lipopolysaccharide (LPS)-challenged piglets and its association with integrity of the intestinal barrier coupled with effective dosage were expected to be signified. In the present study, 72 piglets (Landrace × Yorkshiere × Duroc) were randomly allotted to four groups, each group with six replicates. The subjects in the control group were provided with basal diet while those in other tested groups with extra 0.05, 0.1, and 0.2% L. salivarius, respectively. Fourteen days later, LPS was intraperitoneally injected and sodium pentobarbital was then delivered to euthanize those LPS-challenged piglets. An increase of average daily gain and body weight along with an apparent decline of diarrhea rate were observed in L. salivarius-treated groups. Both 0.1 and 0.2% L. salivarius supplement in total diet had the capability to markedly elevate levels of CAT, GSH-Px, SOD, anti-inflammatory cytokine from the serum as well as tight junction proteins (Claudin-1, Occludin, and ZO-1) extracted from intestine in LPS-challenged piglets. These changes were accompanied by the obvious downregulation of D-lactic acid, DAO, MDA and pro-inflammatory mediators in the serum, including IL-1β, IL-6, IFN-γ, and TNF-α. Meanwhile, the expression levels of TLR2 and TLR4 in spleen and mesenteric lymph nodes were significantly lower whereas the oxidation-related gene, ho-1 was up-regulated with L. salivarius administration. Our findings suggested that relatively high dose L. salivarius (0.1–0.2%) could regulate the progression of inflammatory response and oxidative stress when individuals were exposed to LPS, thus probably offering valuable assistance in restoring barrier function and improving overall performance.
Introduction
Weaning, coming after lactation, is a critical and stressful stage in which piglets have to undergo various challenges and makes those post-weaned piglets more susceptible to some intestinal diseases. For instance, bacterial diarrhea is quite frequent among piglets especially 2 weeks after weaning, which is commonly known as post-weaning diarrhea (PWD). Previous studies have stated that PWD is closely correlated with Escherichia coli (E. coli) infection from the environment (1) and those affected individuals tend to develop yellow watery diarrhea and suffer from dehydration (2). Meanwhile, an abrupt separation from lactating sows is another stress factor for those young piglets to handle and corticotropin-releasing factor (CRF) was previously suggested to stimulate stress-induced intestinal dysfunctions (3, 4). Since the resulting elevated rate of mortality, growth retardation and requirement of extra medication have adverse influence on the swine industries; broader attentions with respect to porcine intestinal health have been constantly paid.
An epithelial barrier is maintained between the luminal enclosure of the intestine and the internal environment of the body (5). The role of this barrier is not only restrained in preventing harmful entities, such as dietary antigens and pathogens, from the gut lumen into other tissue compartments or circulation (6), but also has the capability to permit the nutrient absorption and waste exclusion (7). The nutrient absorption is initiated either through transmembrane pathway, generally the solute transport, or paracellular one (8). Functional analysis indicates that the selective paracellular permeability could be regulated by tight junctions, the multi-protein complexes located in the lateral space between individual cells (9). Tight junctions consist of a branching network of proteins, among which Occludin (10) and Claudin (11) are trans-membrane proteins and scaffolding ZO-1 anchors the cytoskeleton to the trans-membrane domains of Occludin (12). All these integral proteins are suggested to be dynamic (13) and have pivotal roles in regulating barrier junction and even cell survival (10–12).
A bunch of physiological and pathological factors could contribute to the dysfunction of intestinal barrier. For example, the apoptosis of epithelial cells is associated with tight junction disruptions since both Occludin and ZO-1 might go through cleavage with distinctive manners (14, 15). Pro-inflammatory cytokines mediated by infections or traumas appear with the dissembling of tight junction organization and structure, and thus lead to an increased permeability (16, 17). This epithelial breach is responsible for the discontinuous intestinal barrier and makes functional substances in gut penetrate into the portal blood, such as diamine oxidase (DAO), a digestive enzyme, and D-lactic acid (18). Under this terrible circumstance, bacteria [mainly Firmicutes and Bacteroidetes (19)] and food antigens are more likely to be translocated from the lumen into the mesenteric lymph nodes (MLNs), blood stream and other organs like the spleen and liver. Obviously, this bacterial passage is a contributor to the immune response and inflammatory cytokines will be simultaneously released. In this case, perpetuation of chronic inflammation in intestinal site is likely and probably keeps giving rise to a vicious cycle.
Besides, some studies have reported that post weaning is a potent activator of oxidative stress, a condition where the imbalance between free radicals, or also called reactive oxygen species (ROS) and the antioxidants occurs. These free radicals, partly generated as the byproducts of mitochondrial respiration (20), are normally regarded as the main cause of oxidation because of their capability to easily react with other molecules, which is either beneficial or harmful for bodies. As a matter of fact, ROS with non-toxic quantity plays a crucial role in maintaining the cellular functions and supporting physiological metabolism, such as cell differentiation, proliferation and viability (21, 22). However, overproduction of ROS could put risks to an assortment of cellular abnormalities, including but not limited to biomacromolecule damage, cell apoptosis, and oxidative stress as mentioned before. Therefore, antioxidants provided by cells offer an electron to free radicals which could then be inactivated and eradicated; thus, they usually act as the oxidant defensive system to antagonize the disturbed homeostasis caused by oxidative burst. These include the use of Superoxide dismutase (SOD), catalase (CAT), and Glutathione peroxidase (GSH-Px) to counteract the excessive amount of ROS, all of which could be considered as trustworthy parameters for oxidative stress. The oxidant degradation of lipids results in a process called lipid peroxidation during which the generated lipid peroxides could be decomposed into Malondialdehyde (MDA). As a biochemical product of lipid peroxidation, it represents another reliable indicator for fat oxidant status in cells, and could indirectly reveal the damage of cells (23).
In this study, we took advantage of Lipopolysaccharide (LPS), a major component of cell wall from the Gram-negative bacteria, to primarily establish a bacteria-induced piglet model. Toll-like receptors (TLRs) are believed to participate in the innate immune system of the host by recognizing a diversified array of foreign antigens known as pathogen associated molecular patterns (PAMPs) (24). This identification has been designated as a potent inducer of activating inflammatory cytokines and chemokines (25). Many groups have manifested that TLR2 and TLR4 are able to sense and bind LPS, generally acting as crucial mediators of distinct cellular responses under this pathogenic condition (26).
Despite the fact that probiotics, a common type of living microbes, have been routinely involved in piglet diet in an attempt to boost pig health and performance, the administration of probiotics and its mechanisms have still been an uncharted territory in practice. The proper application of probiotics has been reported to have connection with gut improvement and superior nutrient utilization (27). Plentiful experiments in vivo or in vitro have pronounced the protective strategies of different Lactobacillus species against the intestinal dysfunction of piglets. However, L. salivarius is a newly separated strain and the efficacy of this particular strain as well as the appropriate dosage still remain ambiguous when aiming to combat digestive issues of post-weaned piglets. Our study was committed to discover the positive influence of L. salivarius with a tailored concentration that may bring to the gut health and try to explore its role in the modulation of inflammation as well as oxidative stress after bacterial infection.
Materials and Methods
Bacterial Strains
The L. salivarius strains used in this study were isolated from the feces of healthy piglets. After purifying, L. salivarius was cultured in De Man, Rogosa and Sharpe (MRS) agar medium at 37°C for 24 h with an anaerobic environment. The culture solution was later centrifuged at 3,000 rpm for 10 min at 4 °C. The vacuum freeze-drying machine (Tofflon, Shang Hai, China) was used to acquire the bacterial powder in which 1 × 1010 CFU/g L. salivarius could be detected. The bacterial concentration was finally measured by an ultraviolet spectrophotometer (Nano Drop, Thermo Fisher, America) with the optical density being set at 550 nm.
Animals, Diets, and Experimental Design
All pigs used in this experiment were born naturally at 114 days gestation which was considered as the full term pregnancy. A total of 72 crossbred healthy female piglets (Landrace × Yorkshiere × Duroc) were reared by sows and weaned at 21 ± 2 days of age. Piglets with initial body weight (BW) 6.44 ± 0.25 kg were randomly assigned to 1 of 4 treatments, each treatment with six replicates. All the subjects were housed in 6 pigpens with an ambient temperature of 20–30°C, and each pen was equipped with a feeder and a drinking nipple to allow piglets free access to food as well as drinking water. The corn-soybean meal-fish meal basal diet (Table 1) for the subjects was formulated to meet the estimating nutrient requirements released by the National Research Council (NRC 2012), and all the added ingredients stayed the same as those used in our previous study (28). Piglets were carefully weighed throughout the test by using the electronic weighing system. Through the entire growing period, the average daily gain (ADG) of each piglet was defined as the difference between the ending and beginning weight divided by feeding days. Meanwhile, the equation for the average daily feed intake (ADFI) was the total of feed used per pen day averaged by three piglets together with trial days.
Experimental Procedures
The selected piglets were randomly divided into four dietary groups: the basal diet (C), basal diet supplemented with 0.05% L. salivarius (T1), basal diet with 0.1% L. salivarius (T2), and basal diet with 0.2% L. salivarius. LPS from E. coli serotype O55:B5 (Sigma Chemical Co., St. Louis, MO) was intraperitoneally injected (200 μg/kg body weight) right after the 14-days trial. The applied dosage of LPS was referred to previous description in our lab (29) and the sterile 0.9% NaCl solution was used for diluting LPS to reach the confirmed concentration.
Collections of Blood and Tissue Samples
After LPS injection, the blood samples (5 ml/piglet) of piglets (one piglet per pen) were collected from precava 6 h after intraperitoneal administration, from which the serum was later separated by centrifugation at 3,000 rpm for 20 min with the temperature of 4°C. The collected serum was transferred into microtubes and then stored at −80°C for the detection of cytokines and antioxidant levels. Sodium pentobarbital (50 mg/kg body weight) was delivered by a rapid intracardial injection for euthanizing the piglets and the jugular exsanguination procedures were also initiated to ensure the death. After sacrifice, certain tissue samples were obtained for the performances of quantitative PCR (qPCR) and western blotting. An ~10 cm segment of intestine was incised from the mid-jejunum, flushed with ice-cold saline and opened longitudinally. MLNs, spleens and livers were dissected out. All the above harvested samples were immediately immersed in liquid nitrogen and stored at −80°C for RNA isolation.
Measurements of D-lactic acid, DAO, and CRF From the Serum
The enzyme activity of DAO and CRF levels were measured using enzyme-linked immunoassay kits (Jiancheng Bioengineering Institute, Nanjing, China). A chemical assay kit from the same company was purchased for D-lactic acid analysis. All the performances were based on the supplier's protocols.
Determination of Cytokines From Serum by ELISA
The porcine enzyme-linked immunosorbent assay (ELISA) was employed for detecting a variety of soluble cytokines produced in the serum of piglets from designed four groups, including IL-1β, IL-6, IL-10, TNF-α, and TNF-γ (R&D Systems, Minneapolis, MN). All the reagents from the kit were placed in the room temperature for 15 min prior to performances. 50 μL of Assay Diluent was firstly added to each well and 50 μL of both high and low standard, control and tested serum were separately added per well. After mixing, the plate was covered with adhesive sealer at shaker for 2 h at room temperature. Each well was then aspirated and washed with 400 μL wash buffer four times. Next, 100 μL conjugates was gently added into each well and incubated for 2 h at the shaker with room temperature. Substrate solution, protected from light, was finally used for 30 min incubation, after which the stop solution was added. The result of optical density was collected through the micro-plate reader set to 450 nm.
RNA Isolation
The frozen samples were replaced on ice to thoroughly thaw and the shredded parts were poured with 1,000 μL TRIzol for 5 min complete degradation of cell membrane and proteins. 200 μL chloroform was added to the cell lysate and the mixture was inverted several times. After inversion, the substances with three different layers were centrifuged at 12,000 g for 15 min at 4°C. The upper layer containing RNA was removed and mixed with 500 μL isopropanol and final pellet was obtained after centrifugation for 10 min with the previously mentioned rotational speed and temperature. 1 ml 75% ethanol served as the reagent for washing pellet which was finally air dried for 10–15 min and dissolved in RNase free water for the following analysis. Both the amount and the purity of RNA product were measured by Picodrop Microliter Spectrophotometer. In addition, the integrity of the total RNA was determined with 1% agarose gel electrophoresis.
cDNA Synthesis and Quantitative PCR
cDNA was synthesized from the tissue-derived RNA with an amount of 2 μg. This reverse transcription included the use of MMLV-reverse transcriptase, 5X buffer, dNTP mix and RNasin ribonuclease inhibitor to a total volume of 25 μL under the guidance of instruction's protocol (Promega, Madison, WI). The qPCR was then conducted in triplicate on ABI 7500 Real-Time PCR detector with a SYBR Premix Ex Taq (Tli RNaseH Plus) and qPCR kit (TaKaRa Biotechnology, Inc., Shiga, Japan). The primer pairs for detected genes were referred to previous reports (30, 31) and the sequences are listed in Supplementary Table 1. The relative gene expression data were analyzed by normalizing the threshold cycle (Ct) value of each sample to that of GAPDH, which was calculated with 2−ΔΔCt method.
Biochemical Assay of MDA
The reagent mixture provided by commercial MDA Assay Kit (Nanjing Jiancheng Bioengineering Institute, Nanjing, China) and 200 μL serum were homogenized according to the manufacture's guidelines. Briefly, the mixture was boiled for 40 min in a water bath. After incubation and cooling, the solution was centrifuged at 4,000 rpm for 10 min. The obtained supernatant was pipetted for ultra-violet spectrophotometry. The intensity of the formed color, corresponding to the level of MDA, was read at 532 nm and the final concentration was determined by plotting the obtained value against standard curve.
Estimation of Antioxidant Enzyme Activities From the Serum
The activity of SOD, CAT and GSH-Px were assessed by using SOD Assay Kit, CAT Assay Kit and GSH-Px Assay Kit (Nanjing Jiancheng Bioengineering Institute, Nanjing, China), respectively. All the procedures of each particular detection as well as the calculation were based on the manufacturer's instructions. Some main steps were described below. For determining SOD activity, 100 μL prepared-serum with kit provided reagent mixture were firstly vortexed and incubated in a 37°C water bath for 40 min. The presence of SOD could reduce the component of nitrite in the reaction and 2 ml color developing agent was later utilized to generate a particular reddish-purple compound whose absorbance was later determined spectrophotometrically at 550 nm. In order to evaluate CAT activity, 100 μL prepared-serum with mixed reagents were kept in a 37°C water bath for 60 s, after which the color developing reagent was added and a light-yellow product finally appeared after reaction. The maximum absorbance of this compound was colorimetrically measured at 405 nm. One unit of CAT activity was defined as the amount of enzyme required for destroying 1 μmole H2O2 per minute. GSH-Px activity was measured by orderly adding provided reagents into the prepared supernatant and 5 min was required for this reaction. The absorbance of the yellowish mixture was finally determined at 420 nm.
Protein Extraction and Western Blotting
For protein extraction, about 50–100 mg intestinal tissue was firstly immersed in 1 ml NP-40 lysis buffer together with a cocktail of protease and phosphatase inhibitors. Obtained homogenates were then vortexed, and after which the lysates were subjected to a 12,000 × g rotational speed produced by centrifugation for 15 min at 4°C. After spinning, the supernatants containing proteins were collected for electrophoresis. Protein concentration was estimated by the Bradford protein assay, a simple spectroscopic technique, to make sure the amount of protein sample loaded in each well was kept constant. After electrophoresis, separated proteins on the gel were transferred onto polyvinylidene difluoride (PVDF) Membrane (Millipore). This membrane was then coated with blocking buffer and followed by incubation of primary antibodies for 2 h at room temperature. After several rinsing steps, the horseradish peroxidase (HRP)-conjugated secondary antibodies were applied on the same membrane for 1 h at room temperature. The Western blot luminescence detection kit (Santa Cruz Biotechnology, Santa Cruz, CA) was utilized to present protein bands after appropriate exposure by AlphaImager 2200 and the band densities were finally quantified. Antibodies used in this study were purchased from Abcam, including anti-Zo-1, anti-Occludin, anti-Claudin-1, and anti-β-actin.
Statistical Analysis
All experiments were performed with six independent replicates. Statistical analysis was created by using SPSS v. 22.0, and differences in data were evaluated by one-way ANOVA with p < 0.05 being considered statistically significant.
Results
L. salivarius Exerted Growth-Promoting Effects in Piglets
Piglet fed diets containing 0.1% L. salivarius contributed to an improved growth 14 days after weaning as observed by the significant increase of ADG along with BW. Aside from the fairly consistent data of ADFI derived from four groups, the feed and gain radio (F/G) also showed no statistical significance but a tendency of reduction in all groups treated with L. salivarius. It's also worth noting that the diarrhea incidence was counted in each group and the acutely lower possibilities were attributed to 0.1 or 0.2% L. salivarius (Table 2).
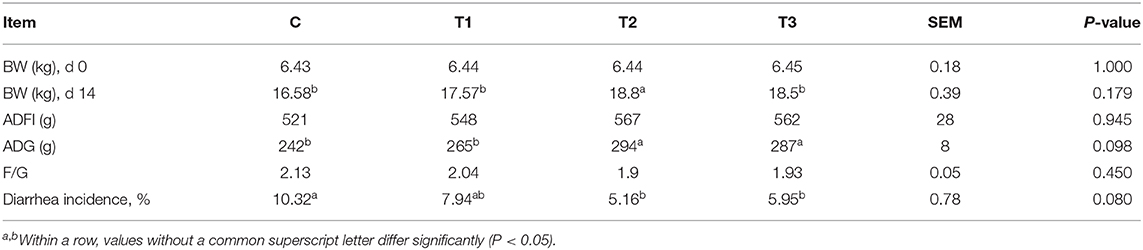
Table 2. Potential effect of L. salivarius on growth performance and diarrhea incidence of weaned-piglets after LPS stimulation.
The Serum Level of D-Lactic Acid, DAO, and CRF After L. salivarius Ingestion
Both the D-lactic and DAO levels were obviously attenuated in response to either 0.1% or 0.2% L. salivarius (P < 0.05) (Figures 1A,B). However, no difference of CRF was presented in L. salivarius-supplemented groups when compared to the control one (Figure 1C).
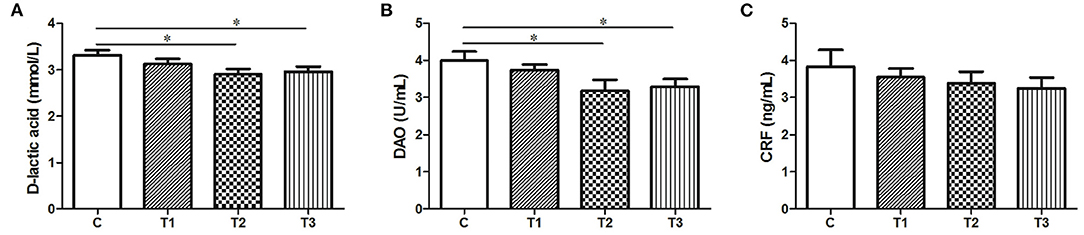
Figure 1. The effect of L. salivarius on intestinal permeability and CRF level in LPS-injected subjects during weaning transition. (A) D-lactic acid, (B) DAO, and (C) CRF were evaluated in the serum. *P < 0.05. Error bars plotted as mean ± SEM.
The Protective Effects of L. salivarius on Epithelial Integrity
Candidate proteins of tight junctions were analyzed by western blotting. Compared with the control group, an increased trend of ZO-1, Occludin and Claudin-1 were detected in L. salivarius-treated subjects regardless of the using amount (Figure 2). Specifically, ZO-1 and Claudin-1 were significantly increased by both 0.1 and 0.2% L. salivarius pretreatment (Figures 2A,C) while 0.1% L. salivarius exerted an obvious enhancement of Claudin-1 (p < 0.05) (Figure 2B).
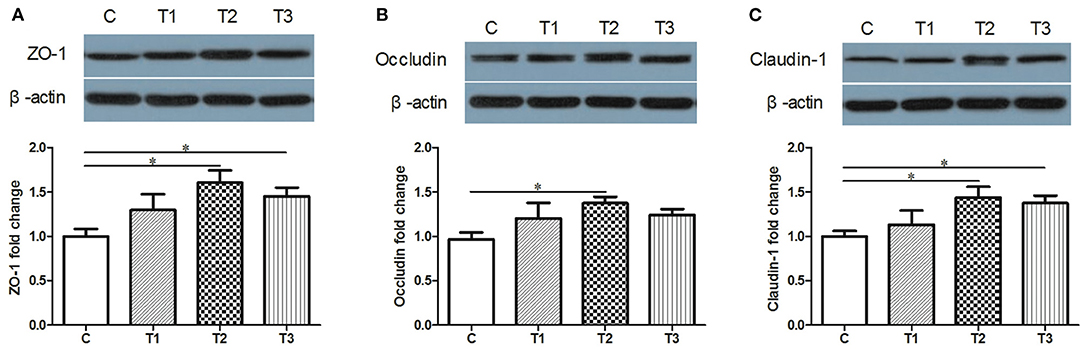
Figure 2. The positive influence of L. salivarius on the expression levels of tight junction proteins in post-weaned piglets after stimulated with LPS. (A) ZO-1, (B) Occludin, (C) Claudin-1 were detected in part of mid-jejunum by western blotting. β-actin, the housekeeping protein, was used as a loading control for proper interpretation. *P < 0.05. Error bars plotted as mean ± SEM.
The Influence of L. salivarius on the Alternation of Pro-Inflammatory and Anti-Inflammatory Cytokines in the Serum
In our effort to figure out the potential function of L. salivarius in inflammatory process, a group of cytokines secreted in the serum were determined by ELISA. After infection, the uptake of 0.1 or 0.2% L. salivarius enabled the levels of IL-1β, IL-6, IFN-γ, and TNF-α to be markedly down-regulated while the decreasing level of IFN-γ and TNF-α caused by 0.05% L. salivarius did not reach the statistical significance (Figures 3A,B,D,E). Meanwhile, a significant elevation of IL-10 was reported in almost all L. salivarius pretreated piglets. Still, we presented an exception that even though there was a marginally increasing trend of IL-10 triggered by 0.05% L. salivarius, the change was not obvious (Figure 3D). Generally speaking, the variation of each inflammation-related cytokine detected in our study was significantly altered by 0.1 or 0.2% L. salivarius instead of 0.05% dosage.
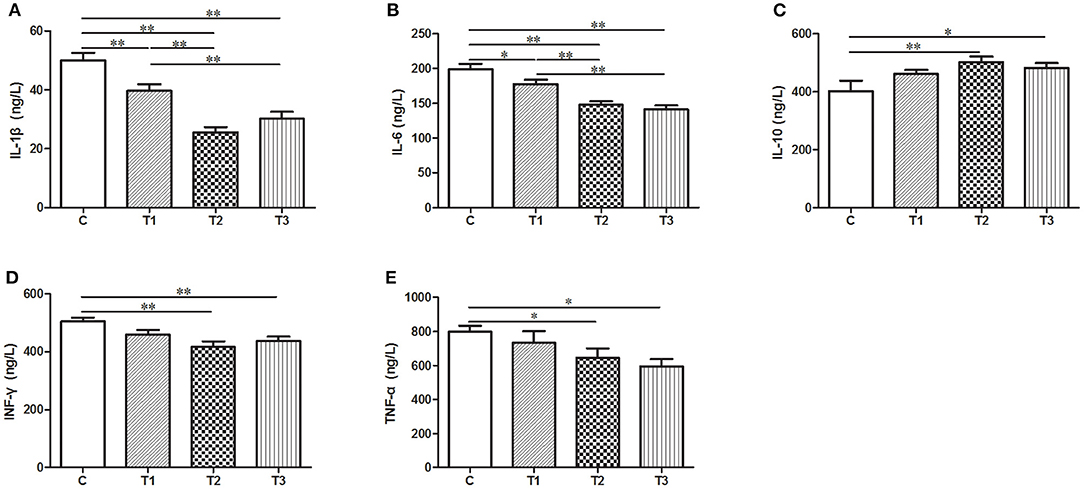
Figure 3. A wide range of pro- and anti-inflammatory cytokines were regulated following L. salivarius treatment on LPS-challenged individuals. The cytokine levels of (A) IL-1β, (B) IL-6, (C) IL-10, (D) IFN-γ, and (E) TNF were evaluated from the serum by ELISA. *P < 0.05; **P < 0.01. Error bars plotted as mean ± SEM.
The Abundance of TLR2 and TLR4 in Spleen and MLNs With L. salivarius Involvement
Expression of TLR2 and TLR 4 mRNA were examined in either spleen or MLNs isolated from the control and L. salivarius-treated piglets. In case of spleen, a decrease of TLR2 or TLR4 was found in groups treated with 0.05, 0.1, and 0.2% L. salivarius, respectively (Figures 4A,B). On the other hand, in MLNs, the level of TLR2 and TLR4 showed noticeable decrease only with the involvement of 0.1 or 0.2% L. salivarius. In fact, the alteration level of TLR2 and TLR4 in groups with 0.05% L. salivarius compared to that of the control was not quantitatively sufficient despite the tendency to decline (Figures 4C,D).
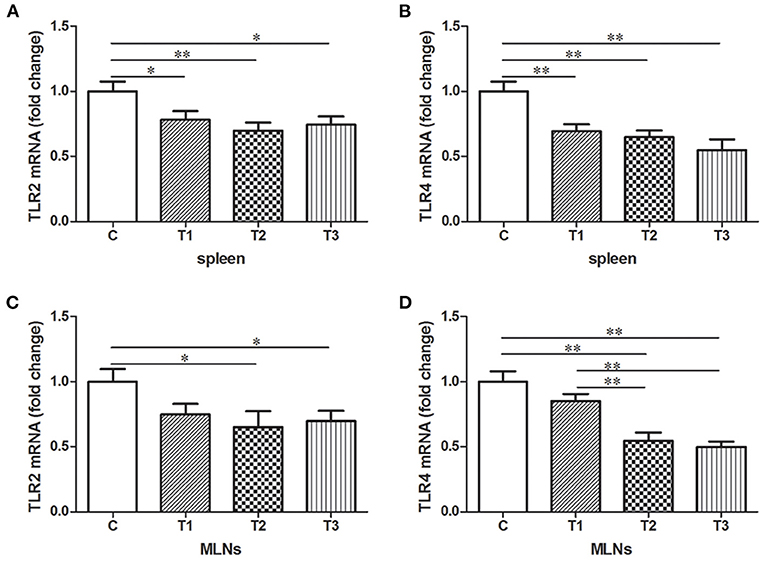
Figure 4. The down-regulated mRNA expression patterns of TLR2 and TLR4 induced by L. salivarius in spleen and MLNs of LPS-challenged piglets 14 days after weaning. The mRNA levels of (A) TLR2 and (B) TLR4 in spleen accompanied by (C) TLR2 and (D) TLR4 in MLNs were detected by qPCR. The relative gene expression data were analyzed by normalizing the threshold cycle value of each sample to that of GAPDH. *P < 0.05; **P < 0.01. Error bars plotted as mean ± SEM.
The Modulation of Parameters Reflecting Oxidative Stress With L. salivarius Administration
As biochemical markers for oxidative stress in the body, the level of SOD, CAT, GSH-Px and MDA in the serum were examined by ELISA. L. salivarius administration with gradient concentration could all induce an elevation of oxidative enzymes as well as a markedly reduction of MDA compared to the control group (Figure 5). It's also worth mentioning that 0.1% L. salivarius had the best performance not only in significantly increasing SOD, CAT, and GSH-Px expression levels (P < 0.05), but also scavenging MDA (P < 0.01) (Figure 5).
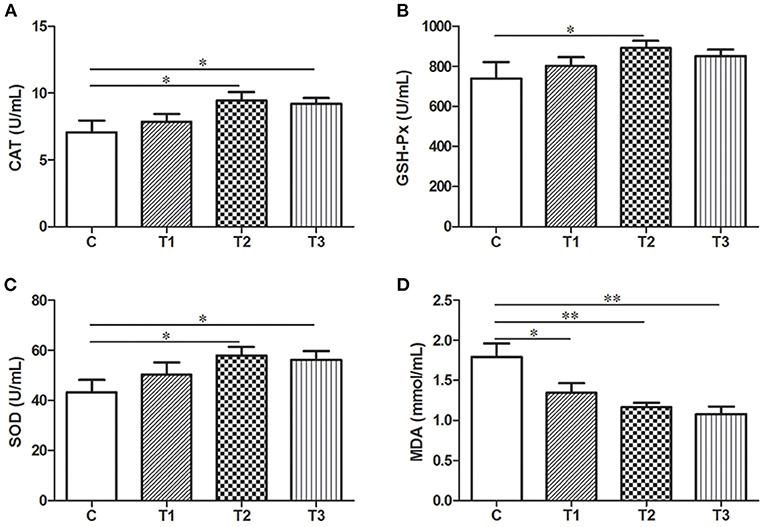
Figure 5. Biochemical alternations of indicators regarding oxidative stress in post-weaned piglets with LPS stimulation. (A) CAT, (B) GSH-Px, (C) SOD, and (D) MDA were detected in the serum. *P < 0.05; **P < 0.01. Error bars plotted as mean ± SEM.
Quantitative Assessment of Potential Genes Related to Oxidative Stress in the Liver With Probiotic Treatment
In order to present a quantitative assessment of these oxidative stress markers, qPCR was performed with samples from the liver since it is a vital organ for detoxification and metabolism. There was a huge improvement of CAT mRNA expression in three L. salivarius involvement groups, like at least one-fold better than the control group (Figure 6A). Similarly, L. salivarius could also give rise to an enriched transcription of sod1 and gsh-px4 and the increasing level was significant. However, the incorporation of L. salivarius did not result in any noticeable change of SOD2, SOD3, and GSH-Px1 mRNA (Figures 6B,E,F). The mRNA level of HO-1 was significantly down-regulated in groups with L. salivarius treatment while no difference was noticed regarding to the expression level of NQO1 (Figures 6G,H).
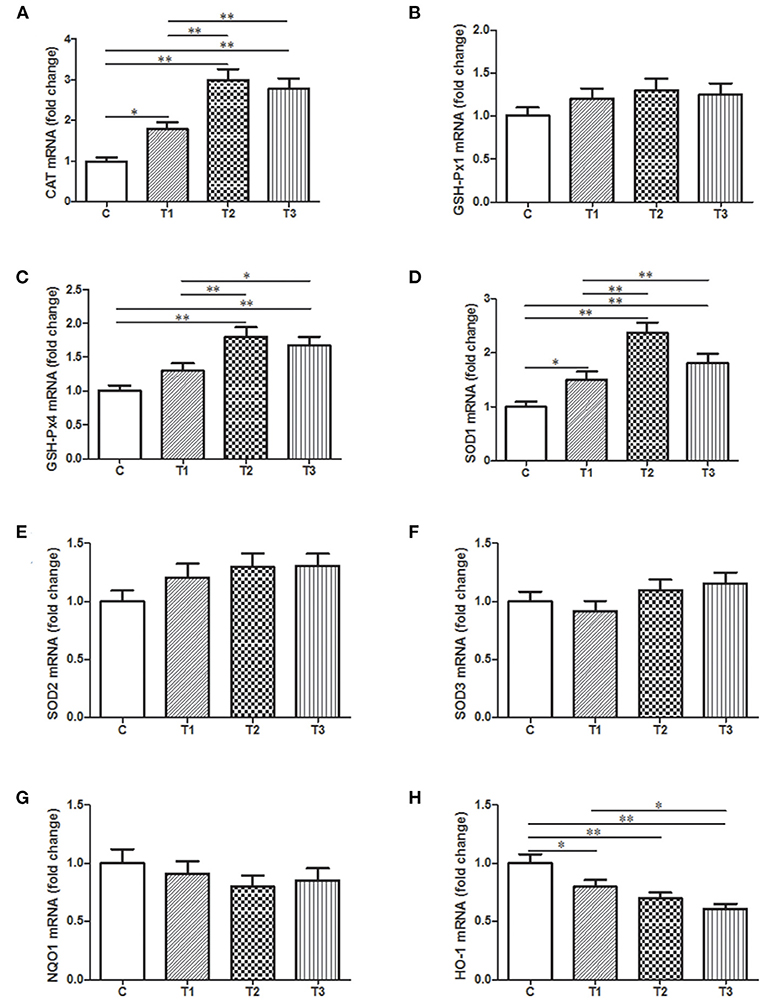
Figure 6. Relative mRNA expression levels of genes involved in the oxidation-related signaling pathways as well as the antioxidant system from the liver tissues of weaned-piglets. (A) CAT, (B) GSH-Px1, (C) GSH-Px4, (D) SOD1, (E) SOD2, (F) SOD3, (G) NQO1, and (H) HO-1 were measure by qPCR with GAPDH acting as reference gene for normalization. *P < 0.05; **P < 0.01. Error bars plotted as mean ± SEM.
Discussion
The growth of piglets could be compromised due to a variety of posted challenges during the weaning period, such as a changing diet, a different surrounding setup, social interaction with other littermates and a higher incidence of getting viral or bacterial infection from pens. Researches posits that PWD is tied to the above-mentioned environmental or psychological factors, among which the ingestion of E. coli is always considered as the causal agent for intestinal disturbances with diarrhea being a common and severe clinical sign. Aside from an improvement of hygienic rearing condition, the diarrhea developed in post-weaned piglets is practically prevented by food additives, antibiotics, and oral vaccines (32). However, there is a report stating that due to the gut microbiota dysbiosis during early life, the vaccine efficacy could not be guaranteed based on the reduction of antibody levels (33). Since the excessive use of antibiotics have kept ongoing concerns about the development of antibiotic-resistant bacteria strains as well as the detrimental impact on human beings, the usage of antibiotics has been gradually minimized and even eliminated in EU since 2006 (34). Previously published scientific consensus in relation to distinct probiotics demonstrated that this category of live microorganisms could contribute to the enhancement of piglet enteric health after weaning (35). As a major component of probiotics, certain Lactobacillus strains have been delineated to function in gut health promotion. For example, the treatment of Lactobacillus plantarum is proposed as a feasible approach for improving diarrhea resistance and protecting intestinal morphology when young piglets are challenged by E. coli (36). In a similar study, the dietary addition of Lactobacillus rhamnosus GG (LGG) is also proved to help intestinal recovery of E. coli-challenged piglets (37). However, there is a noteworthy research stating that some risks associated with high-dose L. rhamnosus in an E. coli model of piglet diarrhea should not be underestimated (38). Since the exact mechanisms by which Lactobacillus confer benefits to the gut health are still limited, we here used L. salivarius, a newly separated strain belonging to the family of Lactobacillus, to target the infected individuals for exploring its efficacy in maintaining intestinal physiology and it remains to be seen whether the predicted efficacy based on a dose-dependent manner or not.
An LPS model was selected in our study to mimic the E. coli infection to which newborn piglets may be exposed in their normal living environment. Earlier results have proved that Lactobacillus reuteri D8 and Lactobacillus delbrueckii (39, 40) are conducive to the increased BW as well as decreased diarrhea rate, two promising signs of healthy status of intestine in which nutrient absorption usually occurs. Here in our work, it was remarkable to mention that 0.1% L. salivarius brought the maximum BW and ADG as well as the minimum diarrhea incidence among three treated groups (Table 2), indicating an advantageous effect of this strain with suitable dosage on the growth performance of post-weaned piglets.
Tight junctions of intestinal mucosa serve as a multifunctional assemble residing between epithelial cells. In fact, this complicated structure contains a cluster of proteins and their quantity and distribution appear to have impacts on the integrity of intestinal barrier and functional permeability (41, 42). As noted previously, the increased permeability in colitis was preceded by Dextran sulfate sodium via the loss of ZO-1, facilitating inflammatory infiltrate (43). In this case, there is also higher possibility for certain substances in the lumen to leak into the surrounding blood due to the increased permeability, such as pathogens. To our knowledge, the intestinal wall of piglets tends to be incomplete during their early days (44), so investigators have been trying to figure out possible solutions to improve this discontinuity. For instance, the up-regulated levels of Occludin and ZO-1 induced by either Lactobacillus reuteri I5007 or L. plantarum are conducive to the improved function of the intestinal mucosal barrier (36, 45). Therefore, it appears justifiable that L. salivarius has a potential role in making compensation for the impaired intestine because in this study, the abundance of tight junction Occludin, claudin-1 and ZO-1 were significantly augmented with actions of this probiotic (Figure 2). Since D-lactic acid and DAO normally present in lumen and villous cells, respectively (46), these two substances were able to indirectly reflect intestinal status after damage. Therefore, the decreased levels of D-lactic acid and DAO in groups with L. salivarius involvement (Figure 1), as demonstrated in our study, could be seen as powerful evidence for showing the ameliorated barrier after damage. Another interesting finding of this part is that low additive of L. salivarius barely had any difference until 0.1% L. salivarius started to markedly help synthesize tight junction proteins as well as drop the level of D-lactic acid and DAO, which is in line with the fact that this particular dosage was also able to maximize the growth performance of piglets (Table 2). This specific probiotic strain seems to confer protection against intestinal damage caused by bacterial infection and a particular dosage, 0.1%, may display the most effective potency in optimizing the tight junctions when common infection occurs.
In fact, a para-cellular seal might not be amply acquired with the existence of Occludin and ZO-1 (47), which means other proteins, like junctional adhesion molecules (8), still await characterization. Our study also presented a negligibly decreasing trend of CRF from the serum (Figure 1C). One previous review has hypothesized the disturbance of the brain-gut axis could lead to dysfunction of intestine in both human and animal models via emitting CRF systemically or peripherally (48). Alongside the harmful pathogens, it has been acceptable that side effects on gut could be produced due to the psychological stress from which piglets are more likely to suffer after weaning. The relationship of stress and the regulation of gastrointestinal permeability has been thoroughly summarized in the same review as well (48), so it was no wonder that a slightly decline tendency of CRF was observed in probiotics-added groups.
The immune system is responsible for distinguishing invading microorganisms depending on a sophisticated recognizing system. In general, TLRs and NOD-like receptors (NLRs) feature in identifying particular components of pathogens and then activate a bunch of signaling pathway for inflammatory response. Once this activation initiates, a cascade of immunostimulatory cytokines, such as IL-1β, IL-6, IL-10, IFN-γ, and TNF, will be initiated to amplify the inflammatory reaction whereas the opposite consequence brought by immunosuppressive cytokines like IL-10 is to limit the inflammation. From this perspective, the protective effects of probiotics on the inflammatory process in piglets have been illustrated by some investigators. Lactobacillus acidophilus and LGG could counteract gut inflammatory response to ETEC by down-regulating pro-inflammatory cytokines while increasing anti-inflammatory regulators via MAPK/NF-κB signaling pathway (28, 49). Selected Lactobacilli is suggested to modulate TLR4 signaling in intestinal cell lines, resulting in the suppression of inflammation (50). L. plantarum N14 has the immunoregulatory capacity to change the pro-inflammatory cytokines in porcine intestinal epithelial cells in response to ETEC challenge via TLR2 (51). Here in our study, it turned out that IL-1β, IL-6, IFN-γ, and TNF-α were significantly potentiated by 0.1 and 0.2% L. salivarius. In contrast, the expression levels of IL-10 shown in piglets fed with 0.1 and 0.2% L. salivarius was significantly higher (Figure 3). In agreement with current knowledge, the improved permeability of intestinal barrier might be achieved when the adding concentration of L. salivarius reaches 0.1% and this strain is beneficial for partially ameliorating the LPS-induced inflammatory response.
Spleen and MLNs, defined as the major immunological sites, have crucial capability of maintaining homeostasis in the organism. An enormous number of macrophages placed in these two locations have prominent roles in scavenging blood-borne pathogens and activating adaptive immunity with the support of TLRs. One research stated that the absence of spleen in the body may lead to an impaired host defense response after pneumococci infection (52). In case of spleen or MLNs, both TLR2 and TLR4 were observed significantly higher in control groups compared to groups treated with 0.1–0.2% L. salivarius (Figure 4), which indirectly indicated the contribution made by L. salivarius to ease the inflammation. One previous research posits that the presence of L. acidophilus significantly reduces the level of TLR2 and TLR4 in both spleen and MLNs, the data of which is consistent with ours (28). Meanwhile, we also assume that the decreased level of TLR2 and TLR4 in both spleen and MLNs could be considered as a result from L. salivarius improving intestinal barrier after infection since the pathogens leaked from lumen might be reduced. Macrophages from MLNs and spleen might be recruited to the infectious sites and start to engulf foreigners via identifying the membrane TLRs. Our data provide us with another clue that thanks to the L. salivarius with applicable dosage, the dampened pro-inflammatory cytokines in the serum might make the attraction of these macrophages unnecessary. Nevertheless, the definite mechanism by which L. salivarius might be involved in inhibition of this movement remains vague and additional exploration is still required to obtain a complete understanding.
It's obvious that the dynamic balance between ROS and antioxidants is kept normal under regular physiological condition. When functioning improperly, free radicals will interact with available biological molecules in the body and thus act as an inducer of oxidative stress. In this case, antioxidants are generated to function in detoxifying the reactive ROS and thus conferring oxidative stress resistance. The initiation of this defense will activate a classic redox-sensitive signaling pathway, in which erythroid 2-related factor 2 (Nrf2) will detach from kelch-like ECH-associated protein-1 (Keap1) in the cytoplasm and then translocate into the nucleus as a transcription factor. The regulation of Nrf2 on heme oxygenase-1 (HO-1) and NADPH quinineoxidoreductase-1 (NQO1) is responsible for an assortment of enzymatic or non-enzymatic compounds as antioxidants (53). For instance, SOD, CAT and GSH-Px are defined as antioxidant enzymes and have been taken for granted in numerous researches as the biomarkers for oxidative defense. Four types of GSH-Px have been discovered to be responsible for the reduction of superoxide radicals and lipid hydroperoxides (54), among which GSH-Px1 and GSH-Px4 are most widely investigated. The unbalanced ROS in the body could be the target for these antioxidant enzymes which consequently render the oxidative stress suppressed. However, these native enzymatic substances sometimes are not enough to protect the living creatures from oxidative damage. New interests concerning antioxidant capacity of probiotics in protecting creatures against oxidative damage have been constantly aroused. Multispecies probiotic supplementation is able to relieve oxidative stress in individuals with typeII diabetes, presenting a potent pharmacotherapeutic action (55). Bacillus SC06 could relieve the oxidative stress-related intestinal disorders in rats by reversing diquat-induced MDA augmentation as well as increasing GSH-Px activity (56). Up-regulation of antioxidant enzymes (SOD, CAT, and glutathione S-transferase) resulted from L. plantarum AS1 in the colon of cancer-bearing subjects suggests an anticancer benefit of this probiotic through its antioxidant attributes (57). In case of piglets, since previous studies have shown that weaning or diet contamination could cause the disordered intestine of piglets where the occurrence and the development of oxidative stress are common (58, 59). Dietary supplement including some chemical complexes or microorganisms have been reported to have positive influence on the oxidative stress (59). For instance, Baicalin-zinc or -copper is capable of exerting antioxidant capability in deoxynivalenol-challenged piglets, which is reflected in the reduction of HO-1, NQO-1, NF-κB (60, 61). One week after weaning, there is a decrease of SOD and GSH-Px activities as well as an increase of MDA, reflecting an imbalanced redox status during this stage (58). Under this circumstance, an improved antioxidant capability of weaned piglets is observed after the addition of L. plantarum by promoting of SOD, CAT, and GSH-Px activities (62). From a practical perspective, we aim to explore the mode of action of L. salivarius in ameliorating oxidative issues occurring in weaned piglets. Here in our study, L. salivarius brought a significant increase in serum levels of SOD, CAT, and GSH-Px coupled with a marked decrease of MDA in post-weaned piglets (Figure 5), the final product of lipid peroxidation and a commonly used lipid marker of oxidative stress as well. To further validate, quantitative assessment was evaluated on genes encoding these cytoprotective proteins by qPCR (Figures 6A–F) and the analysis showed that the mRNA level of SOD1, GSH-Px4, CAT, and HO-1were highly augmented by the pretreatment of 0.1–0.2% L. salivarius (Figures 6A,C,D,H). Presumably, a protective effect of L. salivarius could be exerted against oxidative stress in LPS-treated piglets by the promoted expression of SOD1, GSH-Px4, and CAT via Nrf2/HO-1 pathway.
The reciprocal interaction between inflammation and oxidative stress is not something new, which has been witnessed for past decades by many investigators. There has been reached a consensus that oxidative damage is a major contributor to the decreased immune function, as thoroughly demonstrated in a review (63). Nevertheless, there has been a gap in our knowledge as to the mutual crosstalk between L. salivarius, inflammation and oxidative. We wonder there might be a possibility for L. salivarius to depress the inflammation firstly through assisting in the relief of oxidative stress, but this assumption required further theoretical and practical evidence.
Conclusion
As alternatives to antibiotics and other species of probiotics, the supply of relatively high dose L. salivarius (0.1–0.2%) could bring positive contribution to the intestinal permeability through increasing tight junction proteins as well as inhibiting the leak of D-lactic acid and DAO in piglets challenged with LPS. Meanwhile, it appears justifiable that the pretreatment of L. salivarius possesses a feasible role in depressing the immune response by balancing the expression level of pro- or anti-inflammatory cytokines. We also speculate this weaken immunity might partially result from the effective instrument of L. salivarius on oxidant defensive process, during which the expression levels of SOD1, GSH-Px4, and CAT might be positively regulated via Nrf2/HO-1 pathway. In summary, the integrity of intestinal barrier in post-weaned piglets was restored through the suppressed immune response and oxidative stress after infection. This research provides new novel sights into the mechanism of probiotics involved in the intestinal improvement and the thorough understanding is the goal for treating or preventing intestinal diseases developed in post-weaned piglets.
Data Availability Statement
The original contributions presented in the study are included in the article/Supplementary Materials, further inquiries can be directed to the corresponding author/s.
Ethics Statement
The animal study was reviewed and approved by Animal Care and Use Committee of College of Life Science in Tianjin Normal University.
Author Contributions
JQ and HL conceived and designed the study. ZS and YL performed the experiment. JQ analyzed the data and completed the manuscript. All the authors have read and approved the final manuscript.
Funding
This research was funded by National Natural Science Foundation of China (31702147), Tianjin Natural Science Foundation (18JCYBJC30000, 20JCZDJC00190), and Tianjin 131 Innovative Talents Team (20180338).
Conflict of Interest
The authors declare that the research was conducted in the absence of any commercial or financial relationships that could be construed as a potential conflict of interest.
Acknowledgments
The authors would like to thank all the lab members for technical assistance in generating data for this manuscript.
Supplementary Material
The Supplementary Material for this article can be found online at: https://www.frontiersin.org/articles/10.3389/fvets.2020.547425/full#supplementary-material
References
1. Fairbrother JM, Nadeau E, Gyles CL. Escherichia coli in postweaning diarrhea in pigs: an update on bacterial types, pathogenesis, prevention strategies. Anim Health Res Rev. (2005) 6:17–39. doi: 10.1079/AHR2005105
2. van Beers-Schreurs HM, Vellenga L, Wensing T, Breukink HJ. The pathogenesis of the post-weaning syndrome in weaned piglets: a review. Vet Q. (1992) 14:29–34. doi: 10.1080/01652176.1992.9694322
3. Taché Y, Mönnikes H, Bonaz B, Rivier J. Role of CRF in stress-related alterations of gastric and colonic motor function. Ann N Y Acad Sci. (1993) 697:233–43. doi: 10.1111/j.1749-6632.1993.tb49936.x
4. Ottaway CA. Role of the neuroendocrine system in cytokine pathways in inflammatory bowel disease. Aliment Pharmacol Ther. (1996) 10(Suppl.2):10–5. doi: 10.1046/j.1365-2036.1996.22164015.x
5. Camilleri M, Madsen K, Spiller R, Greenwood-Van Meerveld B, Verne GN. Intestinal barrier function in health and gastrointestinal disease. Neurogastroenterol Motil. (2012) 24:503–12. doi: 10.1111/j.1365-2982.2012.01921.x
6. Groschwitz KR, Hogan SP. Intestinal barrier function: molecular regulation and disease pathogenesis. J Allergy Clin Immunol. (2009) 124:3–20. doi: 10.1016/j.jaci.2009.05.038
7. Blikslager AT, Moeser AJ, Gookin JL, Jones SL, Odle J. Restoration of barrier function in injured intestinal mucosa. Physiol Rev. (2007) 87:545–64. doi: 10.1152/physrev.00012.2006
8. Tsukita S, Furuse M, Itoh M. Multifunctional strands in tight junctions. Nat Rev Mol Cell Biol. (2001) 2:285–93. doi: 10.1038/35067088
9. Buckley A, Turner JR. Cell biology of tight junction barrier regulation and mucosal disease. Cold Spring Harb Perspect Biol. (2018) 10:a029314. doi: 10.1101/cshperspect.a029314
10. McCarthy KM, Skare IB, Stankewich MC, Furuse M, Tsukita S, Rogers RA, et al. Occludin is a functional component of the tight junction. J Cell Sci. (1996) 109:2287–98. doi: 10.1016/j.jff.2019.103591
11. Agarwal R, D'Souza T, Morin PJ. Claudin-3 and claudin-4 expression in ovarian epithelial cells enhances invasion and is associated with increased matrix metalloproteinase-2 activity. Cancer Res. (2005) 65:7378–85. doi: 10.1158/0008-5472.CAN-05-1036
12. Suzuki T, Elias BC, Seth A, Shen L, Turner JR, Giorgianni F, et al. PKC eta regulates occludin phosphorylation and epithelial tight junction integrity. Proc Natl Acad Sci USA. (2009) 106:61–6. doi: 10.1073/pnas.0802741106
13. Shen L, Weber CR, Turner JR. The tight junction protein complex undergoes rapid and continuous molecular remodeling at steady state. J Cell Biol. (2008) 181:683–95. doi: 10.1083/jcb.200711165
14. Sun Z, Wang X, Wallen R, Deng X, Du X, Hallberg E, et al. The influence of apoptosis on intestinal barrier integrity in rats. Scand J Gastroenterol. (1998) 33:415–22. doi: 10.1080/00365529850171053
15. Bojarski C, Weiske J, Schoneberg T, Schroder W, Mankertz J, Schulzke JD, et al. The specific fates of tight junction proteins in apoptotic epithelial cells. J Cell Sci. (2004) 117:2097–107. doi: 10.1242/jcs.01071
16. Capaldo CT, Nusrat A. Cytokine regulation of tight junctions. Biochim Biophys Acta. (2009) 1788:864–71. doi: 10.1016/j.bbamem.2008.08.027
17. Barreau F, Hugot JP. Intestinal barrier dysfunction triggered by invasive bacteria. Curr Opin Microbiol. (2014) 17:91–8. doi: 10.1016/j.mib.2013.12.003
18. Feng S, Liu W, Zuo S, Xie T, Deng H, Zhang Q, et al. Impaired function of the intestinal barrier in a novel sub-health rat model. Mol Med Rep. (2016) 13:3459–65. doi: 10.3892/mmr.2016.4978
19. Isaacson R, Kim HB. The intestinal microbiome of the pig. Anim Health Res Rev. (2012) 13:100–9. doi: 10.1017/S1466252312000084
20. Poyton RO, Ball KA, Castello PR. Mitochondrial generation of free radicals and hypoxic signaling. Trends Endocrinol Metab. (2009) 20:332–40. doi: 10.1016/j.tem.2009.04.001
21. Zhang Y, Choksi S, Chen K, Pobezinskaya Y, Linnoila I, Liu ZG. ROS play a critical role in the differentiation of alternatively activated macrophages and the occurrence of tumor-associated macrophages. Cell Res. (2013) 23:898–914. doi: 10.1038/cr.2013.75
23. Chang D, Zhang X, Rong S, Sha Q, Liu P, Han T, et al. Serum antioxidative enzymes levels and oxidative stress products in age-related cataract patients. Oxid Med Cell Longev. (2013) 2013:587826. doi: 10.1155/2013/587826
24. Mukherjee S, Karmakar S, Babu SP. TLR2 and TLR4 mediated host immune responses in major infectious diseases: a review. Braz J Infect Dis. (2016) 20:193–204. doi: 10.1016/j.bjid.2015.10.011
25. Kawai T, Akira S. Toll-like receptors and their crosstalk with other innate receptors in infection and immunity. Immunity. (2011) 34:637–50. doi: 10.1016/j.immuni.2011.05.006
26. Park BS, Lee JO. Recognition of lipopolysaccharide pattern by TLR4 complexes. Exp Mol Med. (2013) 45:e66. doi: 10.1038/emm.2013.97
27. Liao SFF, Nyachoti M. Using probiotics to improve swine gut health and nutrient utilization. Anim Nutr. (2017) 3:331–43. doi: 10.1016/j.aninu.2017.06.007
28. Li H, Zhang L, Chen L, Zhu Q, Wang W, Qiao J. Lactobacillus acidophilus alleviates the inflammatory response to enterotoxigenic Escherichia coli K88 via inhibition of the NF-kappaB and p38 mitogen-activated protein kinase signaling pathways in piglets. BMC Microbiol. (2016) 16:273. doi: 10.1186/s12866-016-0862-9
29. Li HH, Li YP, Zhu Q, Qiao JY, Wang WJ. Dietary supplementation with Clostridium butyricum helps to improve the intestinal barrier function of weaned piglets challenged with enterotoxigenic Escherichia coli K88. J Appl Microbiol. (2018) 125:964–75. doi: 10.1111/jam.13936
30. Guo T, Huang J, Zhang H, Dong L, Guo D, Guo L, et al. Abcb1 in pigs: molecular cloning, tissues distribution, functional analysis, and its effect on pharmacokinetics of enrofloxacin. Sci Rep. (2016) 6:32244. doi: 10.1038/srep32244
31. Meng Q, Guo T, Li G, Sun S, He S, Cheng B, et al. Dietary resveratrol improves antioxidant status of sows and piglets and regulates antioxidant gene expression in placenta by Keap1-Nrf2 pathway and Sirt1. J Anim Sci Biotechnol. (2018) 9:34. doi: 10.1186/s40104-018-0248-y
32. Duan Q, Wu W, Pang S, Pan Z, Zhang W, Zhu G. Co-immunization with two enterotoxigenic Escherichia coli (ETEC) fimbriae MEFAs (multiepitope fusion antigens) induces neutralizing antibodies against five ETEC fimbriae: F4, F5, F6, F18, and F41. Appl Environ Microbiol. (2020) 86:e00217–20. doi: 10.1128/AEM.00217-20
33. Lynn MA, Tumes DJ, Choo JM, Sribnaia A, Blake SJ, Leong LEX, et al. Early-life antibiotic-driven dysbiosis leads to dysregulated vaccine immune responses in mice. Cell Host Microbe. (2018) 23:653–60.e5. doi: 10.1016/j.chom.2018.04.009
34. Heo JM, Opapeju FO, Pluske JR, Kim JC, Hampson DJ, Nyachoti CM. Gastrointestinal health and function in weaned pigs: a review of feeding strategies to control post-weaning diarrhoea without using in-feed antimicrobial compounds. J Anim Physiol Anim Nutr. (2013) 97:207–37. doi: 10.1111/j.1439-0396.2012.01284.x
35. Barba-Vidal E, Martin-Orue SM, Castillejos L. Review: are we using probiotics correctly in post-weaning piglets? Animal. (2018) 12:2489–98. doi: 10.1017/S1751731118000873
36. Yang KM, Jiang ZY, Zheng CT, Wang L, Yang XF. Effect of lactobacillus plantarum on diarrhea and intestinal barrier function of young piglets challenged with enterotoxigenic Escherichia coli K88. J Anim Sci. (2014) 92:1496–503. doi: 10.2527/jas.2013-6619
37. Zhang L, Xu YQ, Liu HY, Lai T, Ma JL, Wang JF, et al. Evaluation of lactobacillus rhamnosus GG using an Escherichia coli K88 model of piglet diarrhoea: effects on diarrhoea incidence, faecal microflora and immune responses. Vet Microbiol. (2010) 141:142–8. doi: 10.1016/j.vetmic.2009.09.003
38. Li XQ, Zhu YH, Zhang HF, Yue Y, Cai ZX, Lu QP, et al. Risks associated with high-dose lactobacillus rhamnosus in an Escherichia coli model of piglet diarrhoea: intestinal microbiota and immune imbalances. PLoS ONE. (2012) 7:e40666. doi: 10.1371/journal.pone.0040666
39. Wang M, Wu H, Lu L, Jiang L, Yu Q. Lactobacillus reuteri promotes intestinal development and regulates mucosal immune function in newborn piglets. Front Vet Sci. (2020) 7:42. doi: 10.3389/fvets.2020.00042
40. Li YH, Hou SL, Chen JS, Peng W, Wen W, Chen FM, et al. Oral administration of Lactobacillus delbrueckii during the suckling period improves intestinal integrity after weaning in piglets. J Func Foods. (2019) 63:103591. doi: 10.1155/2019/6919803
41. Turner JR, Buschmann MM, Romero-Calvo I, Sailer A, Shen L. The role of molecular remodeling in differential regulation of tight junction permeability. Semin Cell Dev Biol. (2014) 36:204–12. doi: 10.1016/j.semcdb.2014.09.022
42. Wang J, Ji H. Tight junction proteins in the weaned piglet intestine: roles and regulation. Curr Protein Pept Sci. (2019) 20:652–60. doi: 10.2174/1389203720666190125095122
43. Poritz LS, Garver KI, Green C, Fitzpatrick L, Ruggiero F, Koltun WA. Loss of the tight junction protein ZO-1 in dextran sulfate sodium induced colitis. J Surg Res. (2007) 140:12–9. doi: 10.1016/j.jss.2006.07.050
44. Hu CH, Xiao K, Luan ZS, Song J. Early weaning increases intestinal permeability, alters expression of cytokine and tight junction proteins, and activates mitogen-activated protein kinases in pigs. J Anim Sci. (2013) 91:1094–101. doi: 10.2527/jas.2012-5796
45. Yang F, Wang A, Zeng X, Hou C, Liu H, Qiao S. Lactobacillus reuteri I5007 modulates tight junction protein expression in IPEC-J2 cells with LPS stimulation and in newborn piglets under normal conditions. BMC Microbiol. (2015) 15:32. doi: 10.1186/s12866-015-0372-1
46. Smith SM, Eng RH, Campos JM, Chmel H. D-lactic acid measurements in the diagnosis of bacterial infections. J Clin Microbiol. (1989) 27:385–8. doi: 10.1128/JCM.27.3.385-388.1989
47. Pinton P, Nougayrede JP, Del Rio JC, Moreno C, Marin DE, Ferrier L, et al. The food contaminant deoxynivalenol, decreases intestinal barrier permeability and reduces claudin expression. Toxicol Appl Pharmacol. (2009) 237:41–8. doi: 10.1016/j.taap.2009.03.003
48. Rodino-Janeiro BK, Alonso-Cotoner C, Pigrau M, Lobo B, Vicario M, Santos J. Role of corticotropin-releasing factor in gastrointestinal permeability. J Neurogastroenterol Motil. (2015) 21:33–50. doi: 10.5056/jnm14084
49. Mao J, Qi S, Cui Y, Dou X, Luo XM, Liu J, et al. Lactobacillus rhamnosus GG attenuates lipopolysaccharide-induced inflammation and barrier dysfunction by regulating MAPK/NF-kappaB signaling and modulating metabolome in the piglet intestine. J Nutr. (2020) 150:1313–23. doi: 10.1093/jn/nxaa009
50. Finamore A, Roselli M, Imbinto A, Seeboth J, Oswald IP, Mengheri E. Lactobacillus amylovorus inhibits the TLR4 inflammatory signaling triggered by enterotoxigenic Escherichia coli via modulation of the negative regulators and involvement of TLR2 in intestinal Caco-2 cells and pig explants. PLoS ONE. (2014) 9:e94891. doi: 10.1371/journal.pone.0094891
51. Murofushi Y, Villena J, Morie K, Kanmani P, Tohno M, Shimazu T, et al. The toll-like receptor family protein RP105/MD1 complex is involved in the immunoregulatory effect of exopolysaccharides from Lactobacillus plantarum N14. Mol Immunol. (2015) 64:63–75. doi: 10.1016/j.molimm.2014.10.027
52. Brown EJ, Hosea SW, Frank MM. The role of the spleen in experimental pneumococcal bacteremia. J Clin Invest. (1981) 67:975–82. doi: 10.1172/JCI110148
53. Li D, Qi J, Wang J, Pan Y, Li J, Xia X, et al. Protective effect of dihydroartemisinin in inhibiting senescence of myeloid-derived suppressor cells from lupus mice via Nrf2/HO-1 pathway. Free Radic Biol Med. (2019) 143:260–74. doi: 10.1016/j.freeradbiomed.2019.08.013
54. Arthur JR. The glutathione peroxidases. Cell Mol Life Sci. (2000) 57:1825–35. doi: 10.1007/PL00000664
55. Asemi Z, Zare Z, Shakeri H, Sabihi SS, Esmaillzadeh A. Effect of multispecies probiotic supplements on metabolic profiles, hs-CRP, and oxidative stress in patients with type 2 diabetes. Ann Nutr Metab. (2013) 63:1–9. doi: 10.1159/000349922
56. Wu Y, Wang B, Xu H, Tang L, Li Y, Gong L, et al. Probiotic bacillus attenuates oxidative stress- induced intestinal injury via p38-mediated autophagy. Front Microbiol. (2019) 10:2185. doi: 10.3389/fmicb.2019.02185
57. Kumar RS, Kanmani P, Yuvaraj N, Paari KA, Pattukumar V, Thirunavukkarasu C, et al. Lactobacillus plantarum AS1 isolated from south Indian fermented food kallappam suppress 1,2-Dimethyl Hydrazine (DMH)-induced colorectal cancer in male wistar rats. Appl Biochem Biotechnol. (2012) 166:620–31. doi: 10.1007/s12010-011-9453-2
58. Cao ST, Wang CC, Wu H, Zhang QH, Jiao LF, Hu CH. Weaning disrupts intestinal antioxidant status, impairs intestinal barrier and mitochondrial function, and triggers mitophagy in piglets. J Anim Sci. (2018) 96:1073–83. doi: 10.1093/jas/skx062
59. Liao P, Li Y, Li M, Chen X, Yuan D, Tang M, et al. Baicalin alleviates deoxynivalenol-induced intestinal inflammation and oxidative stress damage by inhibiting NF-kappaB and increasing mTOR signaling pathways in piglets. Food Chem Toxicol. (2020) 140:111326. doi: 10.1016/j.fct.2020.111326
60. Zha A, Liao P, Tan B, Cui Z, Liao S, Chen L, et al. Dietary baicalin zinc supplementation alleviates oxidative stress and enhances nutrition absorption in deoxynivalenol challenged pigs. Curr Drug Metab. (2020) 21:614–25. doi: 10.2174/1389200221666200302124102
61. Zha A, Yuan D, Cui Z, Qi M, Liao S, Liao P, et al. The evaluation of the antioxidant and intestinal protective effects of baicalin-copper in deoxynivalenol-challenged piglets. Oxid Med Cell Longev. (2020) 2020:5363546. doi: 10.1155/2020/5363546
62. Cui K, Wang Q, Wang S, Diao Q, Zhang N. The Facilitating effect of tartary buckwheat flavonoids and lactobacillus plantarum on the growth performance, nutrient digestibility, antioxidant capacity, and fecal microbiota of weaned piglets. Animals. (2019) 9:986. doi: 10.3390/ani9110986
Keywords: L. salivarius, inflammatory response, oxidative stress, post-weaned piglets, LPS
Citation: Sun Z, Li H, Li Y and Qiao J (2020) Lactobacillus salivarius, a Potential Probiotic to Improve the Health of LPS-Challenged Piglet Intestine by Alleviating Inflammation as Well as Oxidative Stress in a Dose-Dependent Manner During Weaning Transition. Front. Vet. Sci. 7:547425. doi: 10.3389/fvets.2020.547425
Received: 04 April 2020; Accepted: 23 November 2020;
Published: 16 December 2020.
Edited by:
Andrea Serra, University of Pisa, ItalyReviewed by:
Camila Peres Rubio, Sao Paulõ State University, BrazilPeng Liao, Chinese Academy of Sciences, China
Copyright © 2020 Sun, Li, Li and Qiao. This is an open-access article distributed under the terms of the Creative Commons Attribution License (CC BY). The use, distribution or reproduction in other forums is permitted, provided the original author(s) and the copyright owner(s) are credited and that the original publication in this journal is cited, in accordance with accepted academic practice. No use, distribution or reproduction is permitted which does not comply with these terms.
*Correspondence: Jiayun Qiao, c2t5cWp5QHRqbnUuZWR1LmNu