- 1Department of Paraclinical Sciences, Faculty of Veterinary Science, University of Pretoria, Pretoria, South Africa
- 2Department of Veterinary Pathobiology, Texas A&M University, College of Veterinary Medicine & Biomedical Sciences, College Station, TX, United States
- 3Department of Veterinary Tropical Diseases, Faculty of Veterinary Sciences, University of Pretoria, Pretoria, South Africa
Babesiosis is a worldwide, tick-borne disease of economic importance in livestock caused by Babesia spp., which are hemoparasitic piroplasms that target the host erythrocytes. Cattle, dogs, small ruminants, and wild ruminants are the species most commonly affected, while in cats, horses, and pigs, it is less frequently reported. Although babesiosis has been observed worldwide, porcine babesiosis remains an uncommon disease with a very limited number of cases reported. Here, we describe a case in a 12-year old pot-bellied pig from South Africa that died after a history of anorexia and reluctance to rise for 2 days. A complete necropsy, blood smear cytology, reverse line blot (RLB) hybridization and 18S rRNA sequencing were performed. Numerous Babesia spp. hemoparasites and a moderate regenerative anemia were identified on blood smear, and a urine dipstick test yielded 4+ heme. Diffuse icterus and splenomegaly were observed upon gross examination. Histopathology revealed hemoglobin casts within renal tubules and collecting ducts, pulmonary edema, splenic congestion, and intrahepatic cholestasis. BLASTN homology of the 18SrRNA sequence revealed a 100% identity to the published sequence of Babesia sp. Suis isolated from pigs in Italy. This case of babesiosis in a pig highlights the clinical manifestations and gross and pathological findings of porcine babesiosis.
Introduction
Babesiosis, caused by Babesia spp., is a tick-borne disease of economic importance in livestock. This disease is found worldwide but is most prevalent in tropical and subtropical regions, where climatic conditions are more favorable for ticks (1). Babesia spp. infect and multiply within red blood cells, and the continual replication and exit of the intraerythrocytic piroplasms results in erythrocyte lysis. A complex interaction of direct and indirect mechanisms of erythrocyte damage result in hemolytic anemia and clinical signs of icterus, hemoglobinuria, and nephrosis (2–5). Anorexia, anemia, lethargy, lameness, reluctance to stand, high fever, and abortion manifest secondary to reduced oxygen-carrying capacity and compromised tissue perfusion (3, 6). Case fatality rates can be significant in severe acute infection or chronic infection without treatment.
Babesiosis affects a wide range of domestic vertebrates including humans. Cattle (B. bigemina, B. bovis), dogs (B. canis, B. gibsoni, B. rossi, B. vogeli), and small ruminants (B. motasi, B. ovis) are the most commonly affected species. Disease in cats (B. felis), horses (B. caballi), and pigs is uncommon (1, 3, 7, 8). Swine babesiosis is primarily caused by B. trautmanni or less frequently by B. perroncitoi (9). Porcine babesiosis is rare in southern Africa, but two outbreaks were historically reported in domestic pigs in Limpopo Province, South Africa and Zimbabwe (9, 9–11). The causative species, B. trautmanni, was identified by the size and morphology of the parasites in blood or splenic smears (9–11).
This report describes gross and histopathological changes in a 12-year old, pot-bellied sow diagnosed with babesiosis following a brief course of anorexia and reluctance to rise for 2 days. The animal was treated by the owners for suspected constipation, but the pig subsequently died and was submitted to the Department of Paraclinical Sciences at the University of Pretoria for post-mortem examination. Babesiosis was diagnosed based on peripheral blood cytology, and the species was identified by reverse line blot (RLB) hybridization and sequence analysis of partial 18S rRNA isolated from the spleen.
The genetic diversity of Babesia species in pigs is largely unexplored, but recent molecular studies have identified a new subspecies, Babesia sp. Suis, in infected domestic pigs and ticks (6, 12) This report describes the first recognition of porcine babesiosis due to B. sp. Suis in a pet pot-bellied pig from Gauteng province, South Africa.
Materials and Methods
A full necropsy was performed, and samples of the lungs, liver, kidneys, spleen, and heart were collected in 10% buffered formalin for histological examination. Post-mortem blood smears were stained with Kyro-Quick [Kyron Laboratories (Pty) Ltd] Romanowsky stain to evaluate cytology. Formalin-fixed samples were routinely processed and stained with hematoxylin and eosin.
Fresh tissue from the spleen was analyzed for the presence of Theileria and Babesia spp. using the RLB hybridization assay. Genomic DNA extracted using the Purelink™ Genomic DNA Mini Kit (Invitrogen by Life Technologies™, United States) was subjected to PCR using the Theileria/Babesia genus–specific primers RLB F2 [5′-GAC ACA GGG AGG TAG TGA CAA G-3′] and biotin labeled RLB R2 [5′-Biotin-CTA AGA ATT TCA CCT CTA ACA GT-3′] (13–15). Reactions were performed in 25 μl volume containing 12.5 μl of 2X Phusion Flash High-Fidelity PCR Master Mix (Thermo Scientific, LTC Tech, South Africa), 0.2 μM of each primer (forward and reverse), 9.5 μl nuclease-free water, and 2.5 μl of DNA template (between 50 and 100 ng DNA). B. bigemina DNA (Onderstepoort Biological Products, South Africa) was included as a positive control; the negative control was PCR master mix without template DNA. PCR amplification was performed under the conditions described in Supplementary Table 1, and the products were hybridized on the RLB membrane, as described by Gubbels et al. (13), to the genus- and species-specific oligonucleotide probes summarized in Supplementary Table 2.
RLB results were confirmed by cloning and sequencing a partial sequence (~750 bp) of the 18S rRNA gene using BT2-F (5′-GGA GTA TGG TCG CAA GTC TG−3′) and BT2-R (5′-ctt ctg cag gtt cac cta cg-3′) (16) primers. PCR reactions were performed as described above with the following conditions: initial denaturation of 10 s at 98°C, followed by 35 cycles of 1 s at 98°C, 5 s at 61°C, and 15 s at 72°C, and a final extension of 1 min at 72°C.
PCR amplicons were purified using the Purelink™ Quick Gel Extraction and PCR Purification Combo Kit (Invitrogen by Life Technologies™, United States). Using the CloneJET PCR Cloning Kit (Thermo Scientific, LTC Tech, South Africa), the purified amplicons were ligated into the pJET1.2/blunt cloning vector and transformed into competent Escherichia coli JM109 High Efficiency Competent cells (Promega, Madison, USA), according to the manufacturer's instructions. Plasmid isolation was done using the PureLink™ HiPure Plasmid Miniprep Kit (Invitrogen by Life Technologies™, United States), and 3 recombinant plasmids were sequenced on an ABI 3500XL Genetic Analyzer, using the vector primers pJET1.2_F and pJET1.2_R, at Inqaba Biotechnical Industries (Pty) Ltd (Pretoria, South Africa).
Sequences were assembled and edited using the Staden software suite (17). The sequences were compared to the GenBank database for homologous sequences using nucleotide blast BLASTN (18). Phylogenetic analyses were performed by aligning the partial 18S rRNA sequences obtained from this case with a set of 20 sequences (Supplementary Table 2), mainly representative of the Babesidae clade VI, as previously described (6, 12, 19). The Ungulibabesids, as previously defined by Criado-Fornelio et al. (16) fall within this clade of Babesiadae and is where Babesia sp. Suis is currently grouped. The tree was rooted using the 18S rRNA sequence of Toxoplasma gondii (X68523.1).
A multiple sequence alignment was performed with the above-mentioned sequences using the Multiple Alignment Fast Fourier Transform (MAFFT) (version 7) (20, 21). The alignments were manually truncated, using BioEdit (version 7. 2) (22), to the length of the shortest sequence. The evolutionary history was inferred by using the Maximum Likelihood method and General Time Reversible model (23). Phylogenetic trees were constructed by the neighbor-joining, maximum-likelihood and maximum-parsimony methods using MEGA X (version 10.1.8) (24). Initial tree(s) for the heuristic search were obtained automatically by applying Neighbor-Joining (NJ) and BioNJ algorithms to a matrix of pairwise distances estimated using the Maximum Composite Likelihood (MCL) approach, and then selecting the topology with the highest log likelihood value. A discrete Gamma distribution was used to model evolutionary rate differences among sites [five categories (+G, parameter = 0.1986)]. The rate variation model allowed for some sites to be evolutionarily invariable [(+ I), 37.13% sites]. The tree was drawn to scale, with branch lengths measured in the number of substitutions per site. The analysis involved 22 nucleotide sequences. Bootstrap analysis was carried out for each method using a 1000 iterations per tree (25). Evolutionary analysis was conducted in MEGA X (24).
Results
At the postmortem examination, the blood was thin and watery. Blood smear cytology revealed 1 to 4, round- to piriform, protozoan piroplasms (2–3 μm) with round to oval eccentric basophilic to amphophilic nuclei and abundant pale basophilic cytoplasm, consistent with Babesia spp. in numerous erythrocytes (Figure 1). Erythrocytes had mild anisocytosis and polychromasia due to reticulocytosis demonstrating mild to moderate regenerative anemia.
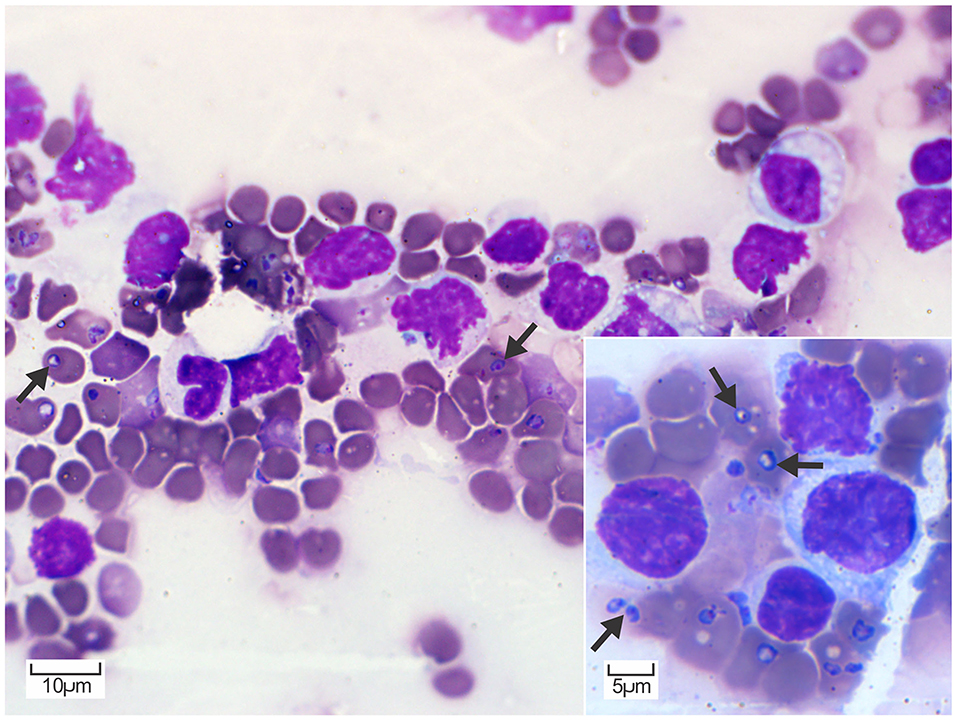
Figure 1. Peripheral blood smear cytology. Erythrocytes contain multiple, round to piriform, 2–3 μm, pale basophilic piroplasms with a small amphophilic nucleus (arrows). Diff-Quik. Inset: Higher magnification of erythrocytes containing piroplasms consistent with Babesia spp. occurring as individual or occasionally paired structures (arrows). Diff-Quik.
The mucous membranes, fat, and the intimal surface of the aorta were yellow. Approximately 100 ml and 20 ml of yellow-tinged fluid were present within the thoracic cavity and pericardial sac, respectively. The lungs were congested and edematous with rib impressions on the pleural surface. Petechial hemorrhages were scattered on the pleural surface of the lungs and in the subepicardial myocardium of the right atrium. The spleen was moderately enlarged and congested. The kidneys were mildly enlarged and soft. The urine was opaque, olive green, and contained dark red- to black particles. Urine analysis noted 4+ heme, 2+ protein, and 1+ glucose.
Histopathology of the kidneys revealed eosinophilic, finely granular hemoglobin casts in numerous renal tubular lumens and collecting ducts accompanied by scattered foci of tubular degeneration and necrosis. Tubular epithelial cell regeneration was characterized by increased numbers of low cuboidal epithelial cells with large round to oval nuclei and pale basophilic cytoplasm (Figure 2A). In the lungs, the alveolar wall interstitium was expanded by lymphocytes and macrophages, and numerous alveoli contained proteinaceous edema fluid (Figure 2B). The spleen was congested and expanded by proliferation of macrophages (Figure 2C) and extramedullary hematopoiesis (EMH). Other areas of EMH included the renal cortical interstitium mainly at the corticomedullary junction and multifocally throughout the hepatic parenchyma and portal tracts. The liver displayed widespread intrahepatic canicular cholestasis (Figure 2D).
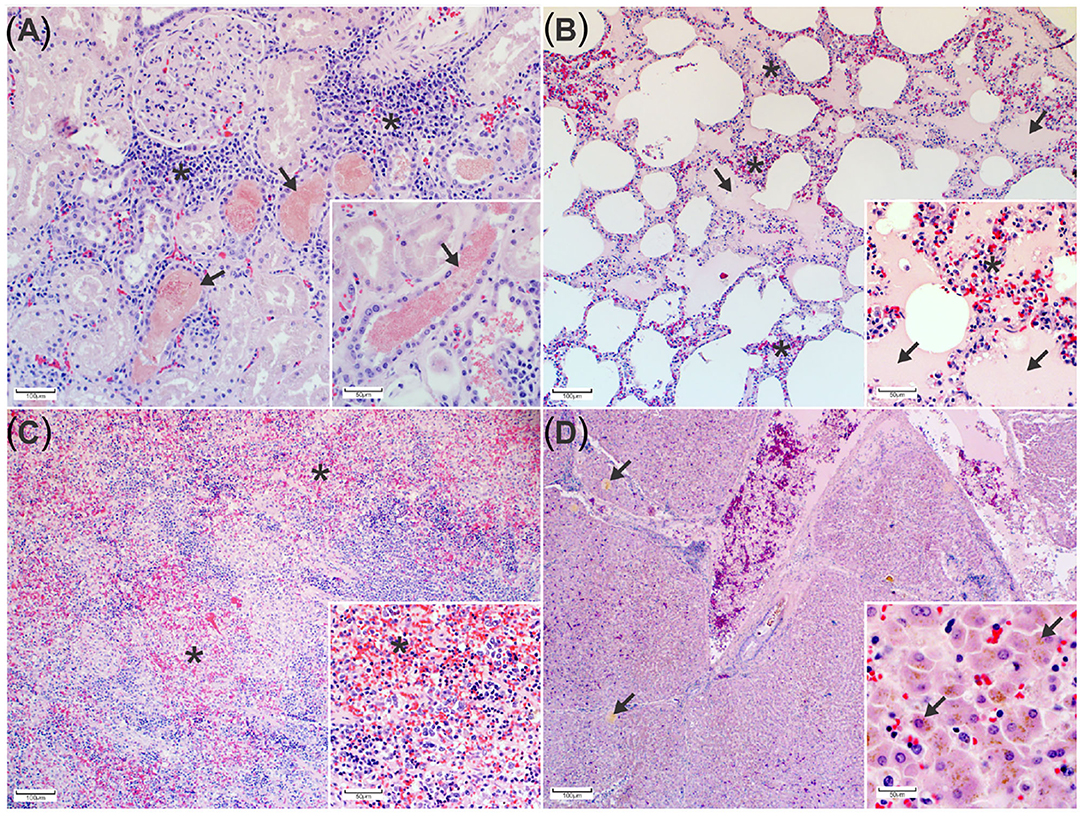
Figure 2. Histopathological changes associated with porcine babesiosis. (A) Kidney: Tubules and collecting ducts contain eosinophilic, finely granular hemoglobin casts. The interstitial space is expanded by extramedullary hematopoiesis (*). (H&E). Inset: Higher magnification of hemoglobin casts in tubules indicating hemoglobinuria (arrows) (H&E). (B) Lung: Alveolar spaces contain proteinaceous edema (arrows). The alveolar walls are expanded by mononuclear inflammatory cells (*) (H&E). Inset: alveolar edema and alveolar macrophage proliferation (arrows) (H&E). (C) Spleen with multiple areas of congestion (*) (H&E). Insert: splenic macrophage proliferation and extramedullary hematopoiesis (H&E). (D) Liver The hepatic canaliculi are expanded by bile (arrows) (H&E) Insert: bile pigment in hepatocytes (H&E).
Babesia 18S rRNA in the spleen sample hybridized with the Theileria/Babesia genus-specific probe, Babesia catch-all 1 and 2 genus-specific probes, and B. bigemina species-specific probe (Supplementary Figure 1). The 18S rRNA sequence was then used for BLAST analysis and demonstrated a 100% sequence identity to B. sp. Anglona/AA-2011 (HQ437690.1). This species, referred to as B. sp. Suis, was originally isolated from a pig sample in Italy (6). Phylogenetically, the Babesia sequence obtained from the pig spleen is closely related to the Ungulibabesids clade along with B. sp. Suis, B. sp. Kashi 1 and 2, and B. orientalis (Figure 3).
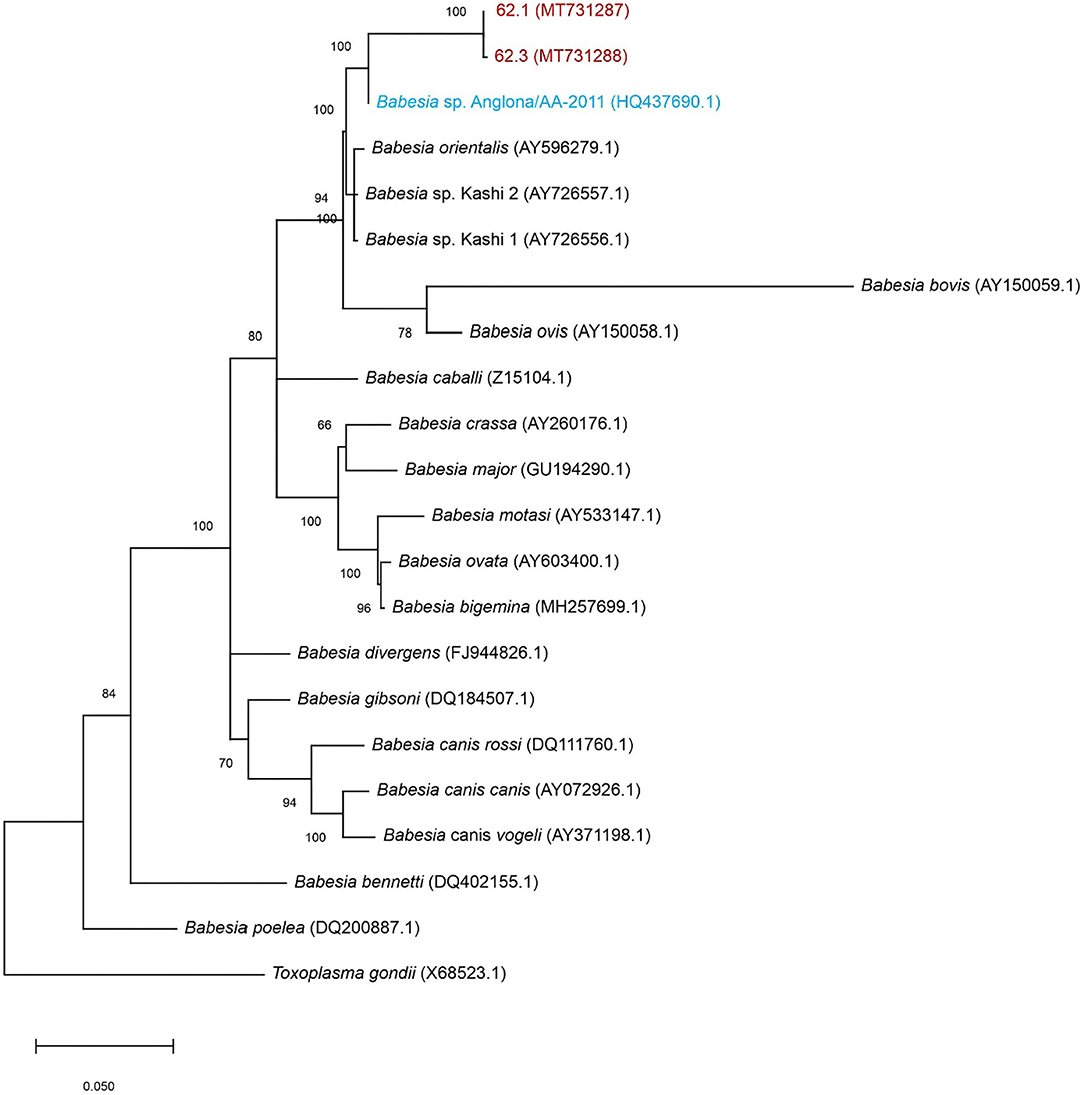
Figure 3. Maximum-likelihood tree based on 18S rRNA nucleotide sequences. The tree shows the phylogenetic relationship between the porcine isolates (MT731287 and MT731288-red), B. sp. Suis (HQ437690.1-blue) compared to other Babesia spp. Toxoplasma gondii was included as outgroup. The numbers at the internal nodes represent the percentage of 1,000 replicates (bootstrap) for which the same branching patterns were obtained. The percentage of trees in which the associated taxa clustered together is indicated next to the branches. The tree with the highest log likelihood (−3,933.11) is shown.
Discussion
Porcine babesiosis may be missed if peripheral blood smears are not performed or if babesiosis is not considered as a differential diagnosis in areas with historically low incidence of tick-borne disease. Gross findings such as icterus, anemia, and hemoglobinuria are non-specific, and differential diagnoses to consider in pigs included babesiosis, Eperythrozoon suis, and acute leptospirosis (9).
The pathogenesis of Babesia spp. starts with a tick taking a blood meal from a mammalian host (1, 7). The parasite attaches to and enters erythrocytes via endocytosis. At this stage, cytology can be used to identify parasites within erythrocytes on peripheral blood smears in live animals or tissues at necropsy. Blood smear cytology was a critical tool needed to diagnose Babesia spp. as the causative agent in this case, but sequencing was necessary to identify the species responsible (9). Sequencing of the partial 18S ribosomal RNA in this case was 100% similar to the sequence for B. sp. Suis (6).
The rapid and continual intraerythrocytic multiplication of Babesia spp. parasites results in intravascular and extravascular hemolytic anemia from erythrocyte lysis by emerging parasites (5, 26, 27). Intravascular hemolysis causes hemoglobinuria and acute tubular necrosis (28–31). Extravascular hemolysis also occurs leading to bilirubinemia and cholestasis of the liver, which manifests as icterus. Clinical symptoms of lethargy, lameness, and fever are secondary to reduced oxygen-carrying capacity from red blood cell loss (3, 6). EMH and regenerative anemia, characterized by reticulocytosis and polychromasia, are compensatory responses to erythrocyte destruction. Thrombocytopenia is a feature of babesiosis that results in petechial hemorrhage, but the mechanism of platelet damage is unknown (32, 33). Acute lung injury and pneumonitis is less common, but has been reported in dogs infected with B. rossi and in human cases (33–35).
The life cycle of Babesia spp. begins when a tick ingests Babesia gametes while feeding on infected animals. Ixodes spp. ticks are common vectors, but Rhipicephalus spp., Haemaphysalis spp., or Dermacentor spp. ticks may also vector Babesia spp. (7, 27). Once inside the tick, Babesia spp. undergo schizogony to form gametes within the tick's gut and then mature into infectious sporozoites within the salivary glands (7). Ticks pass sporozoites to new vertebrate hosts upon feeding, and the sporozoites differentiate into merozoites within host erythrocytes (3, 7). Transovarial transmission between ticks is also possible when Babesia spp. vermicules migrate to the female tick's ovaries (36).
The most common Babesia species identified in pigs is B. trautmanni, which is vectored by Rhipicephalus spp. ticks (6, 9, 36). The previous reports of babesiosis in southern Africa all occurred in pig production units in the 1940s−1950s, and the causative species reported based on morphologic features of the parasite in blood was B. trautmanni (10, 11). The natural hosts for B. trautmanni are thought to be the warthog (Phacochoerus africanus) and bushpig (Potamochoerus larvatus) (6, 36, 37). Intriguingly, based on piroplasm size and genetic homology, B. sp. Suis is a relative of B. trautmanni. Interestingly, icterus was not reported in any of the previous cases where B. trautmanni was identified as the causative species (9–11). Variation in disease severity and clinical manifestations may be due to pathogen factors or the response of the host to infection. Zobba et al. speculated that different strains identified in clinically ill and in asymptomatic pigs may be responsible for different degrees of pathogenicity (12).
The ability to successfully transmit babesiosis from one host to another depends on the specific invertebrate host and the vertebrate host's competency to maintain the agent in its infectious state (3). Climate change may exacerbate disease transmission events by redistributing the tick vectors' spatial and temporal ranges (38). External pressures may also change tick behavior and generate evolutionary pressure on the pathogen, which may affect host range or pathogenicity (39, 40). Climate variables such as temperature, humidity, and rainfall are known to influence adult tick activity (1, 38). Increasing environmental temperatures may expand tick geographical ranges and introduce the pathogen to naïve animal populations (40–42). As human populations grow and wildlife habitats are fragmented and reduced, increased contact between reservoir hosts (e.g., bushpigs and warthogs for B. trautmanni) and domestic pigs may increase transmission. Close proximity exposes animals to carriers, and the length of time of tick attachment is directly related to efficacy of transmitting Babesia spp. sporozoites (3).
Conclusion
The gross findings, histological changes, and identification of Babesia sp. on blood smear were consistent with a case of babesiosis caused by a recently recognized species, Babesia sp. Suis. This case represents an unusual pathogen identified in an unusual host and emphasizes the increasing importance of public awareness in regions where porcine babesiosis has been historically rare, such as in southern Africa. Multiple environmental and evolutionary pressures on tick vectors and related causative agents are anticipated to cause widened spread of tickborne diseases. As a result, babesiosis should be considered a possible differential diagnosis for pigs that present with lethargy, anemia, icterus, and hemoglobinuria. Blood smears and molecular techniques will complement routine necropsy techniques in the diagnosis of sporadic and emerging protozoal diseases including babesiosis.
Data Availability Statement
The datasets presented in this study can be found in online repositories. The names of the repository/repositories and accession number(s) can be found at: https://www.ncbi.nlm.nih.gov/genbank/, SUB7720577 62.1 MT731287, https://www.ncbi.nlm.nih.gov/genbank/, SUB7720577 62.3 MT731288.
Author Contributions
AA: pathological examination, writing—original draft, and writing—review and editing. JP: writing—original draft and writing—review and editing. IV: molecular analysis, phylogenetic analysis, writing—original draft, and writing—review and editing. EM and AA-G: writing—original draft (supporting), writing—review and editing, and supervision. All authors contributed to the article and approved the submitted version.
Funding
The National Institutes of Health–NIH 5T35OD010991-15 (JP), the Faculty of Veterinary Science, University of Pretoria and the College of Veterinary Medicine & Biomedical Sciences, Texas A&M University, supported this work.
Conflict of Interest
The authors declare that the research was conducted in the absence of any commercial or financial relationships that could be construed as a potential conflict of interest.
Acknowledgments
The authors would like to thank Dr. Charles Byaruhanga (Department of Veterinary Tropical Diseases, Faculty of Veterinary Sciences, University of Pretoria, South Africa) for his assistance in the phylogenetic analysis, the staff of the histopathology laboratory (Pathology section, Department of Paraclinical Sciences, University of Pretoria, South Africa) for preparing the histological slides, Ms. Estelle Mayhew (Graphics Unit, Faculty of Veterinary Science, University of Pretoria, South Africa) for her excellent graphical expertise, Dr. Mary B. Nabity (Texas A&M University, USA) for interpretation of blood smear cytology, and Dr. Martha Hensel (Texas A&M University, USA) for her editing and pathology expertise support.
Supplementary Material
The Supplementary Material for this article can be found online at: https://www.frontiersin.org/articles/10.3389/fvets.2020.620462/full#supplementary-material
References
1. Laha R, Das M, Sen A. Morphology, epidemiology, and phylogeny of Babesia: an overview. Trop Parasitol. (2015) 5:94–100. doi: 10.4103/2229-5070.162490
2. Morita T, Saeki H, Imai S, Ishii T. Erythrocyte oxidation in artificial Babesia gibsoni infection. Vet Parasitol. (1996) 63:1–7. doi: 10.1016/0304-4017(95)00892-6
3. Homer MJ, Aguilar-Delfin I, Telford SR, Krause PJ, Persing DH. Babesiosis. Clin Microbiol Rev. (2000) 13:451–69. doi: 10.1128/CMR.13.3.451
4. Otsuka Y, Yamasaki M, Yamato O, Maede Y. The effect of macrophages on the erythrocyte oxidative damage and the pathogenesis of anemia in Babesia gibsoni-infected dogs with low parasitemia. J Vet Med Sci. (2002) 64:221–6. doi: 10.1292/jvms.64.221
5. Bilić P, Kuleš J, Barić Rafaj R, Mrljak V. Canine babesiosis: where do we stand? Acta Vet. (2018) 68:127–60. doi: 10.2478/acve-2018-0011
6. Zobba R, Parpaglia MLP, Spezzigu A, Pittau M, Alberti A. First molecular identification and phylogeny of a Babesia sp. from a symptomatic sow (Sus scrofa Linnaeus 1758). J Clin Microbiol. (2011) 49:2321–4. doi: 10.1128/JCM.00312-11
7. Uilenberg G. Babesia-a historical overview. Vet Parasitol. (2006) 138:3–10. doi: 10.1016/j.vetpar.2006.01.035
8. Dantas-Torres F, Alves LC, Uilenberg G. Babesiosis. In: Marcondes CB, editor. Arthropod Borne Diseases. 1st Edn. Cham: Springer (2017). p. 347–354.
9. de Waal DT. Porcine babesiosis. In: Coetzer JAW, Thomson GE, Maclachlan NJ, Penrith ML, editors. Infectious Diseases of Livestock. Anipedia (2019). Available online at: https://www.anipedia.org/resources/infectious-diseases-of-livestock/196
10. Lawrence DA, Shone DK. Porcine piroplasmosis. Babesia trautmanni infection in Southern Rhodesia. J S Afr Vet Assoc. (1955) 26:89–94.
11. Naude TW. An outbreak of porcine babesiosis in the Northern Transvaal. J S Afr Vet Assoc. (1962) 33:209–11.
12. Zobba R, Nuvoli AM, Sotgiu F, Lecis R, Spezzigu A, Dore GM, et al. Molecular epizootiology and diagnosis of porcine Babesiosis in sardinia, Italy. Vector Borne Zoonotic Dis. (2014) 14:716–23. doi: 10.1089/vbz.2014.1648
13. Gubbels JM, De Vos AP, Van Der Weide M, Viseras J, Schouls LM, de Vries E, et al. Simultaneous detection of bovine Theileria and Babesia species by reverse line blot hybridization. J Clin Microbiol. (1999) 37:1782–9. doi: 10.1128/JCM.37.6.1782-1789.1999
14. Nijhof AM, Penzhorn BL, Lynen G, Mollel JO, 1Morkel P, Bekker et al. Babesia bicornis sp. nov. and Theileria bicornis sp. nov tick-borne parasites associated with mortality in the black rhinoceros (Diceros bicornis). J Clin Microbiol. (2003) 41:2249–54. doi: 10.1128/JCM.41.5.2249-2254.2003
15. Nijhof AM, Pillay V, Steyl J, Prozesky L, Stoltsz WH, Lawrence JA, et al. Molecular characterization of Theileria species associated with mortality in four species of African antelopes. J Clin Microbiol. (2005) 43:5907–11. doi: 10.1128/JCM.43.12.5907-5911.2005
16. Criado-Fornelio A, Martinez-Marcos A, Buling-Saraña A, Barba-Carretero JC. Molecular studies on Babesia, Theileria and hepatozoon in southern Europe: part II. phylogenetic analysis and evolutionary history. Vet Parasitol. (2003) 114:173–94. doi: 10.1016/S0304-4017(03)00141-9
17. Staden R. The Staden sequence analysis package. Mol Biotechnol. (1996) 5:233–41. doi: 10.1007/BF02900361
18. Altschul SF, Gish W, Miller W, Myers EW, Lipman DJ. Basic local alignment search tool. J Mol Biol. (1990) 215:403–10. doi: 10.1016/S0022-2836(05)80360-2
19. Schnittger L, Rodriguez AE, Florin Christensen M, Morrison DA. Babesia: a world emerging. Infect Genet Evol. (2012) 12:1788–809. doi: 10.1016/j.meegid.2012.07.004
20. Katoh K, Misawa K, Kuma KI, Miyata T. MAFFT: a novel method for rapid multiple sequence alignment based on fast Fourier transform. Mol Biol Evol. (2002) 30:3059–66. doi: 10.1093/nar/gkf436
21. Kuraku S, Zmasek CM, Nishimura O, Katoh K. aLeaves facilitates on-demand exploration of metazoan gene family trees on MAFFT sequence alignment server with enhanced interactivity. Nucleic Acids Res. (2013) 41:W22–8. doi: 10.1093/nar/gkt389
22. Hall TA. BioEdit: a user-friendly biological sequence alignment editor and analysis program for Windows 95/98/NT. Nucleic Acids Symp Ser. (1999) 95–98.
23. Nei M, Kumar S. Molecular Evolution and Phylogenetics. Oxford University Press. (2000). Available online at: https://global.oup.com/academic/product/molecular-evolution-and-phylogenetics-9780195135855?cc=fr&lang=en& (accessed July 06, 2020).
24. Kumar S, Stecher G, Li M, Knyaz C, Tamura K. MEGA X: molecular evolutionary genetics analysis across computing platforms. Mol Biol Evol. (2018) 35:1547–9. doi: 10.1093/molbev/msy096
25. Felsenstein J. Confidence limits on phylogenies – an approach using the bootstrap. Evolution. (1985) 39:783–91. doi: 10.1111/j.1558-5646.1985.tb00420.x
26. McCullough J. RBCs as targets of infection. Hematology. (2014) 2014:404–9. doi: 10.1182/asheducation-2014.1.404
27. Krause PJ, Edoard G, Vannier P. Babesiosis: Microbiology, Epidemiology and Pathogenesis (2019). Available online at: https://www.uptodate.com/contents/search?search=microbiology-pathogenesis-and-epidemiology-of-&sp=0&searchType=PLAIN_TEXT&source=USER_INPUT&searchControl=TOP_PULLDOWN&searchOffset=1&autoComplete=false&language=&max=0&index=&autoCompleteTerm=
28. Johns JL, Christopher MM. Extramedullary hematopoiesis: a new look at the underlying stem cell niche, theories of development, and occurrence in animals. Vet Pathol. (2012) 49:508–23. doi: 10.1177/0300985811432344
29. Boes KM, Durham AC. Bone marrow, blood cells, and the lymphoid/lymphatic system. In: Zachary F, editor. Pathologic Basis of Veterinary Disease Expert Consult. 6th Edn. St. Louis, Missouri: Elsevier Inc (2017). p. 733.
30. Breshears MA, Confer AW. The urinary system. In: Zachary JF, editor. Pathologic Basis of Veterinary Disease Expert Consult. 6th Edn. St. Louis, Missouri: Elsevier Inc (2017). p. 655.
31. Miller MA, Zachary JF. Mechanisms and morphology of cellular injury, adaptation, and death. In: Zachary JF, editors. Pathologic Basis of Veterinary Disease Expert Consult. 6th Edn. St Louis, Missouri: Elsevier Inc (2017). p. 38–9.
32. Jacobson LS, Clark IA. The pathophysiology of canine babesiosis: new approaches to an old puzzle. J S Afr Vet Assoc. (1994) 65:134–45.
33. Leisewitz AL, Goddard A, Clift S, Thompson PN, de Gier J, van Engelshoven JMAJAJ, et al. A clinical and pathological description of 320 cases of naturally acquired Babesia rossi infection in dogs. Vet Parasitol. (2019) 271:22–30. doi: 10.1016/j.vetpar.2019.06.005
34. Horowitz ML, Coletta F, Fein AM. Delayed onset adult respiratory distress syndrome in babesiosis. Chest. (1994) 106:1299–301. doi: 10.1378/chest.106.4.1299
35. Ubah AS, Abalaka SE, Idoko IS, Obeta SS, Ejiofor CE, Mshelbwala PP. Canine babesiosis in a male Boerboel: Hematobiochemical and anatomic pathological changes in the cardiorespiratory and reproductive organs. Vet Anim Sci. (2019) 7:100049. doi: 10.1016/j.vas.2019.100049
36. de Waal DT, Lopez Rebollar LM, Potgieter FT. The transovarial transmission of Babesia trautmanni by Rhipicephalus simus to domestic pigs. Onderstepoort J Vet Res. (1992) 59:219–21.
37. Penzhorn BL. Babesiosis of wild carnivores and ungulates. Vet Parasitol. (2006) 138:11–21. doi: 10.1016/j.vetpar.2006.01.036
38. Leschnik MW, Kirtz G, Tichy AG, Leidinger E. Seasonal occurrence of canine babesiosis is influenced by local climate conditions. Int J Med Microbiol. (2008) 298:243–8. doi: 10.1016/j.ijmm.2008.03.008
39. Koelle K, Pascual M, Yunus M. Pathogen adaptation to seasonal forcing and climate change. Proc R Soc B Biol Sci. (2005) 272:971–7. doi: 10.1098/rspb.2004.3043
40. Dantas-Torres F. Climate change, biodiversity, ticks and tick-borne diseases: the butterfly effect. Int J Parasitol Parasites and Wildl. (2015) 4:452–61. doi: 10.1016/j.ijppaw.2015.07.001
41. Olwoch JM, Van Jaarsveld AS, Scholtz CH, Horak IG. Climate change and the genus rhipicephalus (Acari: Ixodidae) in Africa. Onderstepoort J Vet Res. (2007) 74:45–72. doi: 10.4102/ojvr.v74i1.139
42. Bett B, Lindahl J, Delia G. Climate change and infectious livestock diseases: the case of rift valley fever and tick-borne diseases. In: Rosenstock TS, Nowak A, Girvets E, editors. The Climate-Smart Agriculture Papers - Investigating the Business of a Productive, Resilient and Low Emission Future. Cham: Springer (2019). p. 36–44.
Keywords: anemia, babesiosis, Babesia sp. Suis, hemoglobinuria, icterus, pig, porcine
Citation: Avenant A, Park JY, Vorster I, Mitchell EP and Arenas-Gamboa AM (2021) Porcine Babesiosis Caused by Babesia sp. Suis in a Pot-Bellied Pig in South Africa. Front. Vet. Sci. 7:620462. doi: 10.3389/fvets.2020.620462
Received: 22 October 2020; Accepted: 02 December 2020;
Published: 06 January 2021.
Edited by:
Jennifer Johns, Oregon State University, United StatesReviewed by:
Alberto Alberti, University of Sassari, ItalyGabriella Gaglio, University of Messina, Italy
Copyright © 2021 Avenant, Park, Vorster, Mitchell and Arenas-Gamboa. This is an open-access article distributed under the terms of the Creative Commons Attribution License (CC BY). The use, distribution or reproduction in other forums is permitted, provided the original author(s) and the copyright owner(s) are credited and that the original publication in this journal is cited, in accordance with accepted academic practice. No use, distribution or reproduction is permitted which does not comply with these terms.
*Correspondence: Angela M. Arenas-Gamboa, YWFyZW5hc0Bjdm0udGFtdS5lZHU=
†These authors have contributed equally to this work and share first authorship