- 1Laboratory of Poultry Production, College of Animal Science, Shanxi Agricultural University, Jinzhong, China
- 2Department of Life Sciences, Luliang University, Luliang, China
This experiment was conducted to investigate the characteristics of folic acid (FA) absorption in laying hens and the effect of FA supplementation on cecal microbiota. A total of 432 healthy hens (30-week-old) were randomly assigned to four diets supplemented with FA: 0, 1, 6, and 24 mg/kg of feed for 8 w. Blood, duodenum, jejunum, ileum, cecum, and cecal chyme samples (six samples per treatment) were collected from the hens at the end of the feeding trial. Expression profiles of folate transport and transformation genes in intestine and cecal microbiota were detected. Results showed that serum folate level significantly increased (P < 0.01) with an increase in dietary FA supplementation, reaching a plateau at 6 mg/kg FA supplementation. The expression of FA transport and transformation genes was not affected in the cecum (P > 0.05) by dietary FA supplementation; however, it was affected in the duodenum, jejunum, and ileum and mostly showed a downward trend in treatment groups (P < 0.05). The genes affected include duodenal folate receptor (Folr) and dihydrofolate reductase (Dhfr), jejunal proton-coupled folate transporter (Pcft) and reduced folate carrier (Rfc), and ileal ATP binding cassette subfamily C member (Abcc2), Abcc3, Rfc, Folr, and Dhfr. Furthermore, according to the operational taxonomic unit classification and taxonomic position identification, the cecal microbiota population of the hens was not affected by dietary FA supplementation at the phylum, class, order, family, genus, and species levels (P > 0.05). However, the relative abundance of some microbiota was affected by dietary FA supplementation (P < 0.05). In conclusion, FA transport from the intestinal lumen into enterocytes, and then into the bloodstream, is strictly regulated, which may be associated with the regulation of the expression profiles of genes involved in FA absorption. Pathogenic bacteria decreased in the cecum, especially at 24 mg/kg supplementation, but the beneficial bacteria (Bifidobacteriaceae) decreased at this level, too. Overall, FA supplementation at 6 mg/kg, which was selected for folate-enriched egg production, did not affect the health and metabolism of laying hens negatively.
Introduction
The term folate (vitamin B9) refers to a group of compounds belonging to the water-soluble vitamins, playing an important role in one-carbon metabolism (1). Deficiency in folate has been linked to a variety of disorders, including anemia (2), neural tube defects during fetal development (3), mental illness (4), cancers (5), eye diseases (6). People have gradually realized folate's importance and have started looking for ways to supplement folate. Humans and animals lack the enzymes to synthesize folate de novo, so they must obtain it from their food. Green vegetables, yeast, animal livers, egg yolk, folic acid (FA)-fortified foods, or additives are all good folate sources for humans (7, 8). Because of folate's instability in vegetable sources (9, 10), the potential negative effects of synthetic FA (11–13), egg yolk folate draws considerable attention thanks to its stability, natural occurrence, and high bioavailability (14, 15). Studies have shown that folate content in egg yolk can be increased by supplementing laying hen feed with FA. However, attempts to increase folate concentration in eggs beyond the achieved enrichment level were unsuccessful. Egg folate has reached a maximum plateau and does not increase further with increased dietary FA (16–19). Intestinal absorption of FA is believed to be one of the main factors affecting the transfer of dietary FA into the egg.
The folate sources for laying hens are the feedstuff and synthetic crystalline FA. Plant-derived folate is mostly in the form of polyglutamate with 5–9 glutamate tails (20), and it is absorbed in the intestinal tract. While FA can be absorbed directly, the polyglutamated forms must be first converted to their monoglutamate form by folate hydrolase (1). Within the chicken intestinal tract, the monoglutamate forms are transported across the enterocytes through the action of specific transporters: folate receptor (FOLR), reduced folate carrier (RFC) (21), and proton-coupled folate transporter (PCFT) (22). Once inside the enterocytes, synthetic FA is reduced to tetrahydrofolate (THF) by dihydrofolate reductase (DHFR) to gain a metabolic activity similar to other folate species. Multidrug-resistance-associated protein 2 (MRP2), coded by ATP binding cassette subfamily C member 2 (ABCC2), is expressed at the small intestine apical brush-border membrane and can pump folates back into the intestinal lumen, thereby countering the inward transport mediated by PCFT. MRP3 (coded by ABCC3) and MRP5 (coded by ABCC5) are expressed in the basolateral membrane where they export folate for transit into the vascular system (23). FA absorption rate in the everted intestinal sac model of laying hens reaches a plateau after a certain FA content (24). The regulation of FA intestinal intake is crucial for folate transport to the egg, but the specific mechanism is unclear. The absorption transporter might be regulated at the gene expression, protein and translation levels. Alternatively, the process of enterocyte FA excretion is regulated, thus saturating FA absorption in the intestinal epithelial cells. In this study, the mRNA expression levels of Pcft, Rfc, Abcc2, Abcc3, Abcc5, Folr, and Dhfr in the duodenum, jejunum, ileum, and cecum were detected to understand folic acid absorption characteristics in the intestinal tract of laying hens.
Owing to the specific and saturable process of folate transport into the enterocytes (25), a large amount of FA or its degradants may be excreted through feces. When a large amount of FA is fed to hens for the production of folate-enriched eggs, excess FA is transferred into the cecum. The cecum contains several microbes, which play an important role in maintaining the nutritional status of birds (26, 27). Some of the microbes present in the cecum are capable of synthesizing folate, whereas others depend on dietary folate for growth. Gut microbe-synthesized folate is an important source for the host animal (28). The gut microbiota is an important factor regulating gastrointestinal tract homeostasis and energy metabolism, as well as the immune and central nervous systems of laying hens. Chicken performance and gut health dependent on the complex gut microbial community, which plays a role in nutrient assimilation, biosynthesis, and prevention of pathogen colonization.
Several studies have examined the gut microbiota of chickens under various experimental conditions; however, studies on the effect of FA on the intestinal microbiota of poultry are limited. Therefore, the aim of this study was to assess the effects of FA on the gut microbiota of laying hens and to determine whether the ecological balance of the cecal microorganisms is affected by FA supplementation. Additionally, we investigated the effect of FA supplementation on the cecal microbiota structure of laying hens using 16S rRNA high-throughput sequencing.
Methods and Materials
The study was carried out in accordance with the Chinese guidelines for animal welfare and experimental protocol, and was approved by Shanxi Agricultural University Animal Experiment Ethics Committee (SXAU-EAW-2018-002Chi.001).
Animals, Diets, and Experimental Design
The 8-week experiment (2-week pre-experiment and 6-week formal experiment) was performed in Xingmin Animal Husbandry Industry Cooperative (Taigu, Jinzhong, Shanxi, China) and a single factor test design was adopted. Four hundred and thirty-two 30-week-old healthy Jinghong laying hens were randomly allotted into four groups, each with six replicates of 18 birds. The replicates were divided into six cages with three birds per cage [50 × 50 × 45/38 cm (long × wide × front high/back high)]. The management of the indoor light, temperature followed the chickens' breeding manual (16 h:8 h light: dark cycles, 20 ± 3°C). The chickens had free access to water and food. The dietary treatments consisted of a corn-soybean meal-based diet (Table 1) supplemented with folic acid at 0, 1, 6, and 24 mg/kg (marked as FA0, FA1, FA6, and FA24, respectively), which was purchased from Ketai Biological Co. Ltd. (Shijiazhuang, Hebei, China) with purity of 99.8%. FA0 acted as the control group, 1 mg/kg was the supplemental amount for laying hens according the breeding manual, 6 mg/kg was the most appropriate dose for folate-enriched eggs production based on our previous results, and 24 mg/kg for excess added treatment. The production performance and egg quality measurements were performed weekly and detailed in Appendix Table 1.
Sample Collection
At the end of the experiment, one hen with a final body weight (BW) close to mean BW of each replication (a total of six samples per treatment) was euthanized and subjected to a full post-mortem examination following an overnight fast. Immediately before euthanasia, blood was collected from the wing vein into 5-mL vacuum tubes without anticoagulant to obtain a serum sample. The duodenum, jejunum, ileum, cecum, and the cecal chyme were sampled aseptically.
Blood samples were incubated in a water bath at 37°C for 1 h, followed by centrifugation at 3000 rpm for 10 min (TG16-WS, Xiangli Scientific Instruments Co. Ltd. Changsha, Hunan, China). The upper serum layer was aspirated with a pipette into a 0.5-mL Eppendorf tubes and immediately preserved at −20°C, pending further analysis. About 2 cm of the anterior duodenum, the middle jejunum, and ileum, and the right cecum were rinsed in precooled saline, packed into separate cryogenic tubes, plunged into liquid nitrogen, and then maintained at −80°C, pending detection by quantitative real-time polymerase chain reaction (qRT-PCR). The contents of the left cecum were collected for 16S rRNA sequencing to identify the gut microbiota.
Folate and Homocysteine Content in the Serum
The folate and homocysteine serum levels were assayed using a chicken enzyme linked immunosorbent assay kit (Solarbio Science and Technology Ltd., Beijing, China) following the manufacturer's instructions.
Quantitative Real-Time PCR Analysis of Gene Expression
The intestinal samples were ground with liquid nitrogen, and their total RNA was extracted using the RNAiso Plus reagent (Takara Biotechnology Co., Ltd, Dalian, Liaoning, China) following the manufacturer's instructions. The quantity and quality of the extracted total RNA were determined with a spectrophotometer (NanoDrop 2000, Thermo Fisher Scientific, Waltham, MA, USA) at 260 and 280 nm. First-strand cDNA was synthesized from 1,000 ng of total RNA using a PrimeScriptTM RT reagent Kit with gDNA Eraser (Perfect Real Time; Takara Biotechnology Co., Ltd.) following the manufacturer's instructions. The genes' mRNA level (gene names and the primer sequences were listed in Table 2) was analyzed by quantitative real-time PCR. The genes expression was quantified in triplicate with a StepOnePlus Real-Time PCR System (Applied Biosystems, Foster City, CA, USA) and TB Green Premix Ex Taq II (Tli RNase H Plus; Takara Biotechnology Co., Ltd.) following the manufacturer's instructions, at a reaction volume of 20 μl. The relative expression of the target genes was calculated using the 2−ΔΔCt method, with β-actin as the housekeeping gene.
Microbial DNA Extraction, 16S rRNA Gene Amplification of the V3-V4 Region, Sequencing, and Bioinformatic Analysis
Total genomic DNA of cecal chyme microbiota were extracted using the Fast DNA SPIN extraction kits (MP 122 Biomedicals, Santa Ana, CA, USA), following the manufacturer's instructions, and stored at −20°C, pending further analysis. The quantity and quality of the extracted DNAs were assessed using NanoDrop ND-1000 spectrophotometer (Thermo Fisher Scientific, Waltham, MA, USA) and agarose gel electrophoresis, respectively.
PCR amplification of the bacterial 16S rRNA genes in the V3-V4 region was performed using the 338F forward primer (5′-ACTCCTACGGGAGGCAGCA-3′) and the 806R reverse primer (5′-GGACTACHVGGGTWTCTAAT-3′). Sequencing was performed using the Illumina MiSeq platform with MiSeq Reagent Kit v3 at the Shanghai Personal Biotechnology Co., Ltd (Shanghai, China).
The Quantitative Insights into Microbial Ecology (QIIME, v1.8.0) pipeline was employed to process the sequencing data, as previously described (29). Briefly, raw sequencing reads with exact matches to the barcodes were assigned to respective samples and identified as valid sequences. The low-quality sequences were filtered based on the following criteria (30, 31): sequences that had a length of < 150 bp or an average Phred score of < 20, contained ambiguous bases or mononucleotide repeats of > 8 bp. Paired-end reads were assembled using Fast Length Adjustment of Short reads (FLASH) (32). After chimera detection, the remaining high-quality sequences were clustered into operational taxonomic units (OTUs) at 97% sequence identity by UCLUST (33). A representative sequence was selected from each OTU using default parameters. OTU taxonomic classification was done by Basic Local Alignment Search Tool (BLAST), searching the Greengenes Database (34) against sequence sets, using the best hit (35). An OTU table was generated to record the abundance of each OTU in each sample and the taxonomy of these OTUs. OTUs containing <0.001% of total sequences across all samples were discarded. To minimize differences in sequencing depth across samples, an averaged, rounded rarefied OTU table was generated by averaging 100 evenly resampled OTU subsets under 90% of the minimum sequencing depth for further analysis.
Sequence data analyses were mainly performed using QIIME and packages in R (Version 3.2.0). OTU-level α diversity indices, such as Chao1 richness estimator, Abundance-based Coverage Estimator (ACE) metric, Shannon diversity index, and Simpson index, were calculated using the OTU table in QIIME. Kruskal–Wallis test by ranks was applied to compare the relative abundance of bacteria at the phylum, family, and genus levels. Spearman correlation coefficient was used to analyze the correlation between FA supplementation and bacterial abundance.
Microbial functions were predicted by Phylogenetic Investigation of Communities by Reconstruction of Unobserved States (PICRUSt) based on high-quality sequences, and mapped the data to Kyoto Encyclopedia of Genes and Genomes (KEGG) pathway database. The correlation values between the relative abundance of KEGG pathways and the bacteria were shown in a heatmap based on spearman correlation analysis.
Statistical Analysis
The data of serum parameters, and expression level of genes were analyzed by one-way analysis of variance (ANOVA) with the Duncan post hoc test for multiple comparisons, using IBM SPSS statistical software for Windows (Version 26.0, IBM Corp., Armonk, NT, USA). Results are expressed as treatment means with their pooled standard error of the mean. A probability value of P < 0.05 was considered statistically significant.
Results
Serum Folate and Homocysteine Concentration
Serum folate and homocysteine levels were measured to determine the effect of dietary FA supplementation on folate metabolism in hens. There was an increase (P < 0.001) in serum folate level and a decrease (P = 0.001) in serum homocysteine level with increase in dietary FA supplementation (Table 3). Moreover, serum folate level was negatively correlated (R = −0.794; P = 0.002) with serum homocysteine level.
Relative mRNA Expression of Folic Acid Transfer Genes in the Duodenum, Jejunum, Ileum, and Cecum
The cecal expression characteristics of FA transport and transfer genes was distinct (Table 4). Cecal expression of Abcc2, Dhfr, Folr, Pcft, and Rfc was lower than that in the other parts of the intestines, whereas that of Abcc3 was higher, and they were not different among groups (P > 0.05), indicating differences in FA absorption efficiency between the intestinal segments, and that the contribution of folate absorption in cecum may be relatively small.
Pcft, Rfc, and Folr are relevant in FA absorption from the intestinal lumen into enterocytes. Duodenal Pcft expression showed a decreasing tendency (P = 0.067), whereas jejunal Pcft expression decreased (P = 0.049) in treatment groups. Duodenal Rfc expression was higher (P = 0.015) in birds in the FA1 group compared to birds in the other treatment groups, while jejunal Rfc expression was lower in birds in the FA1 and FA6 group (P = 0.026) and ileal Rfc expression was significantly lower in birds in the FA6 group (P = 0.035) compared to birds in control group. Duodenal and ileal Folr expression significantly decreased (P < 0.001, and P = 0.025, respectively) in birds in the treatment groups compared with that of birds in the control groups. The down regulation of these genes may suggest a decrease in FA transport from the intestinal lumen into the enterocytes.
When FA is absorbed into enterocytes, it must be converted into its active biological form, tetrahydrofolate, by DHFR. Duodenal Dhfr expression decreased in birds in the FA6 and FA24 treatment groups compared to birds in the control and FA1 groups (P = 0.002); jejunal Dhfr expression in the laying hens decreased in the treatment groups (P < 0.001). The absorption of FA may be limited by the down-regulation of Dhfr due to that FA cannot absorption into blood directly and must be reduced into THF by DHFR.
Abcc3 and Abcc5 are involved in the process of expelling folate from enterocytes into the vascular system, and Abcc2 is involved in the process of pumping folates back into the intestinal lumen. The Abcc5 expression in the different intestinal segments was not affected by dietary FA supplementation. Jejunal and ileal Abcc3 expression first decreased in FA1 group and then rose in FA6 group (P = 0.009, and P = 0.015, respectively). Ileal Abcc2 expression of the laying hens decreased in treatment groups (P = 0.003). That Abcc2 and Abcc3 expression changes with the concentration of FA in diet implies their involvement in the maintenance of folate homeostasis in the intestinal epithelium.
Effects of Folic Acid Supplementation on Cecal Microbiota of Laying Hens
A total of 1,243,900 sequence tags with a median length of 425 bp (V3 + V4, ~402 to 453 bp) were obtained from the samples after removing redundant sequences. Each sample from the experimental laying hens contained 52,794 ± 5,832 sequence tags. The sequences were further clustered into 35,392 OTUs using a 97% similarity cut-off value. Rarefaction curves generated from the OTUs suggested that a high sampling coverage (~99%) was achieved for all samples. Bacterial community richness index values (Chao1 and ACE) and diversity index values (Shannon and Simpson) were not affected (P > 0.05) by FA supplementation (Table 5). Based on unweighted UniFrac distance analysis, the β-diversity of cecal microbiota was significantly affected by the treatments (R = 0.2312; P = 0.003); however, analysis based on weighted UniFrac distance showed that the β-diversity of the cecal microbiota was not affected (R = 0.0076; P = 0.375) by dietary FA supplementation. Furthermore, according to the OTU classification and taxonomic position identification, the cecal microbial composition of the hens was not affected by dietary FA supplementation at the phylum, class, order, family, genus, and species levels (Figure 1).
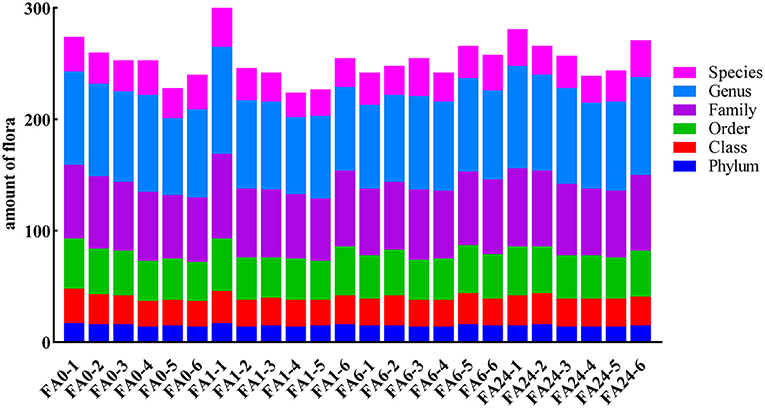
Figure 1. The composition of the cecal microbiota at different classification levels. The taxonomic-composition distribution histograms of each sample are shown at the phylum, class, order, family, genus, and species levels separately. FA, folic acid supplement, the numbers after FA refer to the amounts added in mg/kg feed, the numbers after (-) indicate samples in each group.
A total of 17 phyla were identified, with Firmicutes, Bacteroidetes, and Proteobacteria accounting for almost 95% of the sequences. Firmicutes was the most abundant phylum (45 to 68%) in all four groups, followed by Bacteroidetes (21 to 38%) and Proteobacteria (4 to 26%). The remaining 14 phyla accounted for only 5% of the total. The relative abundance of Verrucomicrobia, TM7, and Fusobacteria were affected (P < 0.05) by dietary FA supplementation. Higher FA consumption seemed to be associated with lower relative abundance of Fusobacteria, TM7, and WPS-2, and a higher relative abundance of Verrucomicrobia (Figure 2).
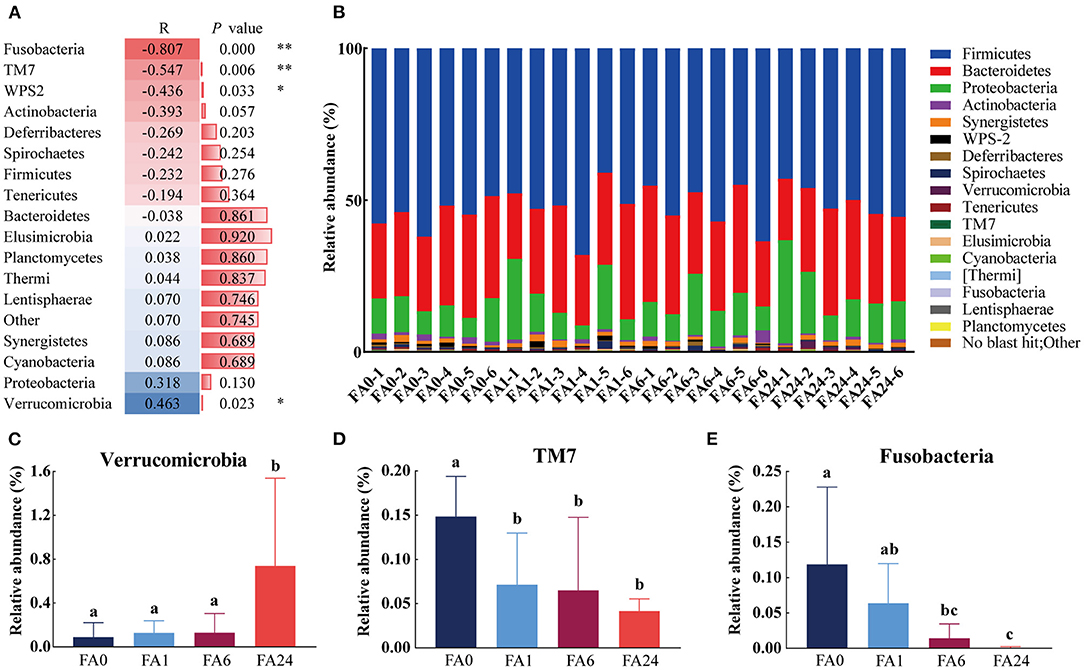
Figure 2. Distribution of the cecal microbiota at the phylum level. (A) Spearman's correlation coefficients of dietary folic acid supplementation and the relative abundance of bacteria at the phylum level, ** indicated highly significant correlation (P < 0.01), * indicated significant correlation (P < 0.05). (B) Taxonomic composition at the phylum level across different samples. (C)–(E) Comparison of the relative abundance of Verrucomicrobia,TM7, and Fusobacteria in different groups, respectively. Different letters in the column represent significant differences (P < 0.05). FA, folic acid supplement, the numbers after FA refer to the amounts added in mg/kg feed, the numbers after (-) indicate samples in each group.
At the family level, 107 families were identified in the cecal contents, of which, 71 families each accounted for more than 0.1% of the total microbial population in at least one sample and were selected for further analysis. After elimination of unclassified families, there were significant differences in the relative abundance of 10 families in the cecum of the laying hens. Spearman correlation coefficient analysis showed that the relative abundance of seven families was negatively correlated with dietary FA supplementation, whereas the relative abundance of five families was positively correlated with dietary FA supplementation (Figure 3).
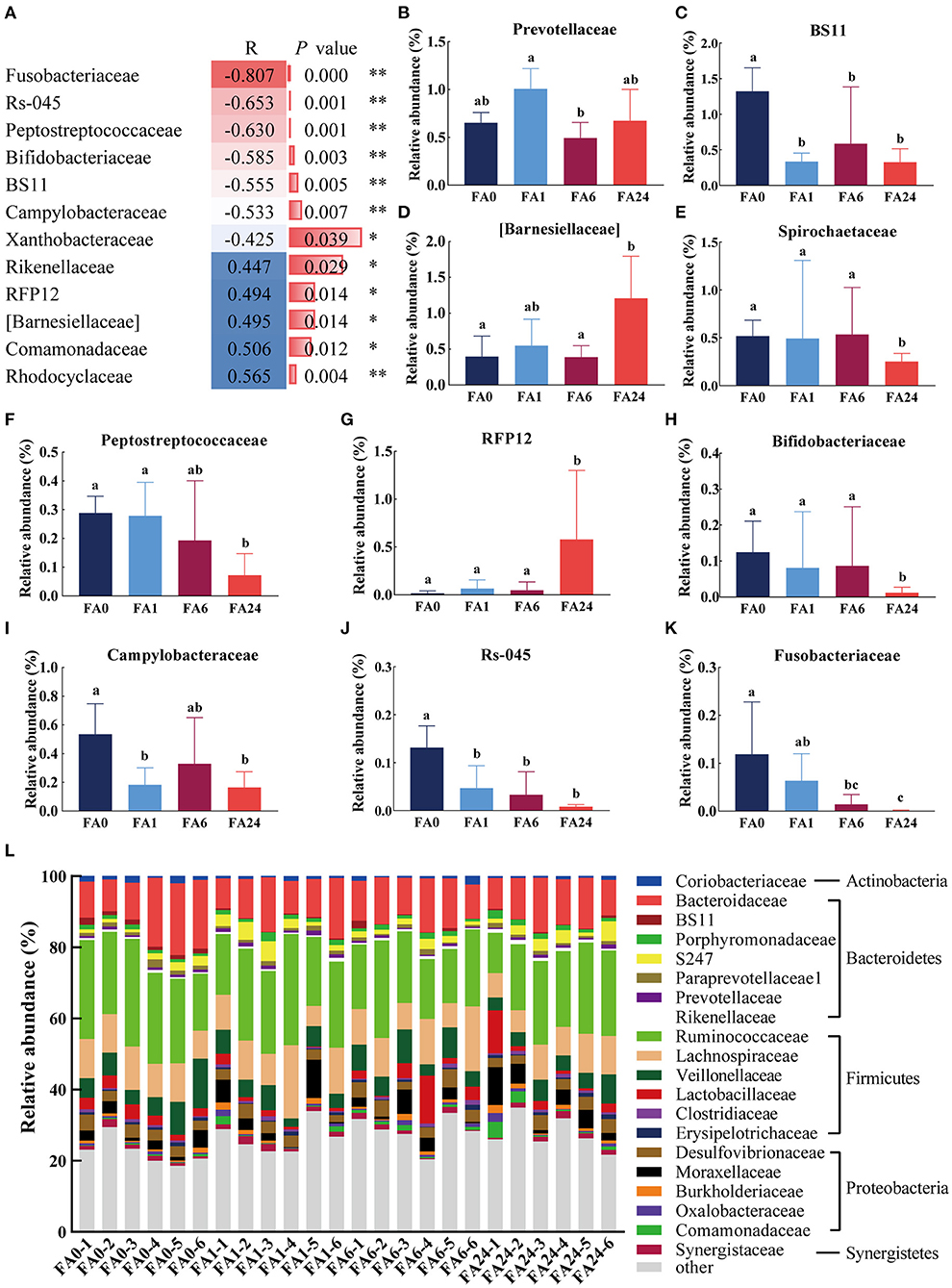
Figure 3. Distribution of the cecal microbiota at the family level. (A) Spearman's correlation coefficients of dietary folic acid supplementation and the relative abundance of bacteria at the family level [only statistically significant differences (P < 0.05) are shown], ** indicated highly significant correlation (P < 0.01), * indicated significant correlation (P < 0.05). (B–K) Comparison of the relative abundance of Prevotellaceae, BS11, [Barnesiellaceae], Spirochaetaceae, Peptostreptococcaceae, RFP12, Bifidobacteriaceae, Campylobacteraceae, Rs-045, Fusobacteriaceae, respectively. Different letters in the column represent significant differences (P < 0.05). (L) Taxonomic composition at the family level across different samples (top 20 classified bacteria are shown). FA, folic acid supplement, the numbers after FA refer to the added in mg/kg feed, the numbers after (-) indicate samples in each group.
At the genus level, 193 genera were identified in the cecal contents, of which, 105 genera each accounted for more than 0.1% of the population in at least one sample and were selected for further analysis. After eliminating unclassified genera, we observed that there were significant differences in the relative abundances of five genera in the cecum of the laying hens (P < 0.05). Spearman correlation coefficient analysis showed that the relative abundance of six genera was negatively correlated with dietary FA supplementation, whereas the relative abundance of five genera was positively correlated with dietary FA supplementation (Figure 4). Fusobacteriaceae and Fusobacterium were the only family and genus classified in Fusobacteria, and Campylobacter was the only genus classified in Campylobacteraceae. Therefore, only Fusobacterium and Campylobacter are discussed in the following sections.
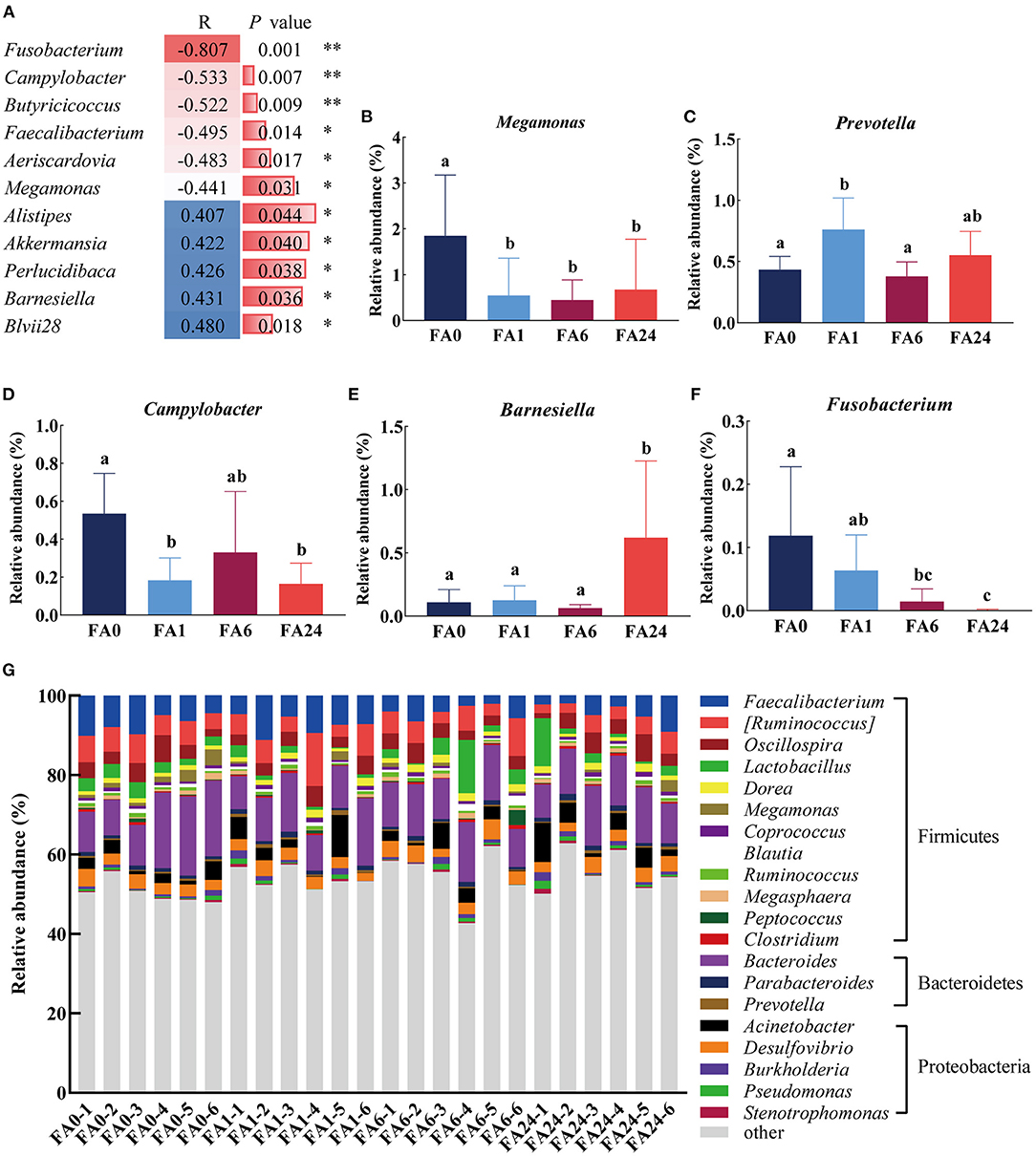
Figure 4. Distribution of the cecal microbiota at the genus level. (A) Spearman's correlation coefficients of dietary folic acid supplementation and the relative abundance of bavteria at the genus level [only statistically significant differences (P < 0.05) are shown], ** indicated highly significant correlation (P < 0.01), * indicated significant correlation (P < 0.05). (B–F) Comparison of the relative abundance of Megamonas, Prevotella, Campylobacter, Barnesiella, Fusobacterium, respectively. Different letters in the column represent significant differences (P < 0.05). (G) Taxonomic composition at the genus level across different samples. (top 20 classified bacteria are shown). FA, folic acid supplement, the numbers after FA refer to the amounts added in mg/kg feed, the numbers after (-) indicate samples in each group.
In summary, compared with the FA0 group, the number of different bacteria in the FA1, FA6, and FA24 groups were 7, 7, and 16 at the three levels, respectively, and 4, 8, and 6 bacteria differed between FA1 and FA6, FA1 and FA24, FA6 and FA24, respectively (Appendix Table 2).
The functional profiles of the microbiota based on the analysis of KEGG level 2 categories showed six pathways were different among the relative abundance of the groups (P < 0.05). Four pathways were positively correlated with the dietary FA supplementation, whereas seven pathways were negatively correlated with the dietary FA supplementation. Of the pathways, four (membrane transport, carbohydrate metabolism, metabolism of cofactors and vitamins, and nucleotide metabolism) were among the top 10 according to relative abundance. The number of different predominant gene functions of the three pair-wise comparisons of FA0, FA1, and FA6 was one. Compared with FA24, the numbers of different predominant gene functions of FA0, FA1, and FA6 groups were 10, 6, and 5, respectively. The microbial gene functions related to metabolic pathways (carbohydrate metabolism, metabolism of cofactors and vitamins, nucleotide metabolism, and metabolic diseases) were negatively correlated with dietary FA supplementation (Table 6).
The correlation between the bacteria of different relative abundance and the top 10 microbial gene functions was analyzed (Figure 5). At the phylum level, Actinobacteria and TM7 were positively correlated with carbohydrate metabolism, and TM7 was positively correlated with nucleotide metabolism. At the family level, the Rs-045 group in TM7 and Bifidobacteriaceae were positively correlated with carbohydrate metabolism and nucleotide metabolism; the BS11 group in Bacteroidetes was positively correlated with the metabolism of cofactors and vitamins, while Comamonadaceae and Rhodocyclaceae were negatively correlated with functions involved in the metabolism of cofactors, and vitamins and nucleotide metabolism; Rikenellaceae was negatively correlated with carbohydrate metabolism, and the metabolism of cofactors and vitamins. The RFP12 group in Verrucomicrobia and Rhodocyclaceae were positively correlated with membrane transport, while BS11 and Peptostreptococcaceae were negatively correlated with membrane transport. At the genus level, Fusobacteria was positively correlated with the metabolism of cofactors and vitamins, and negatively correlated with membrane transport. Faecalibacterium and Aeriscardovia were positively correlated with carbohydrate metabolism; additionally, Faecalibacterium was positively correlated with the metabolism of cofactors and vitamins. Perlucidibaca was negatively correlated with the metabolism of cofactors and vitamins, and nucleotide metabolism.
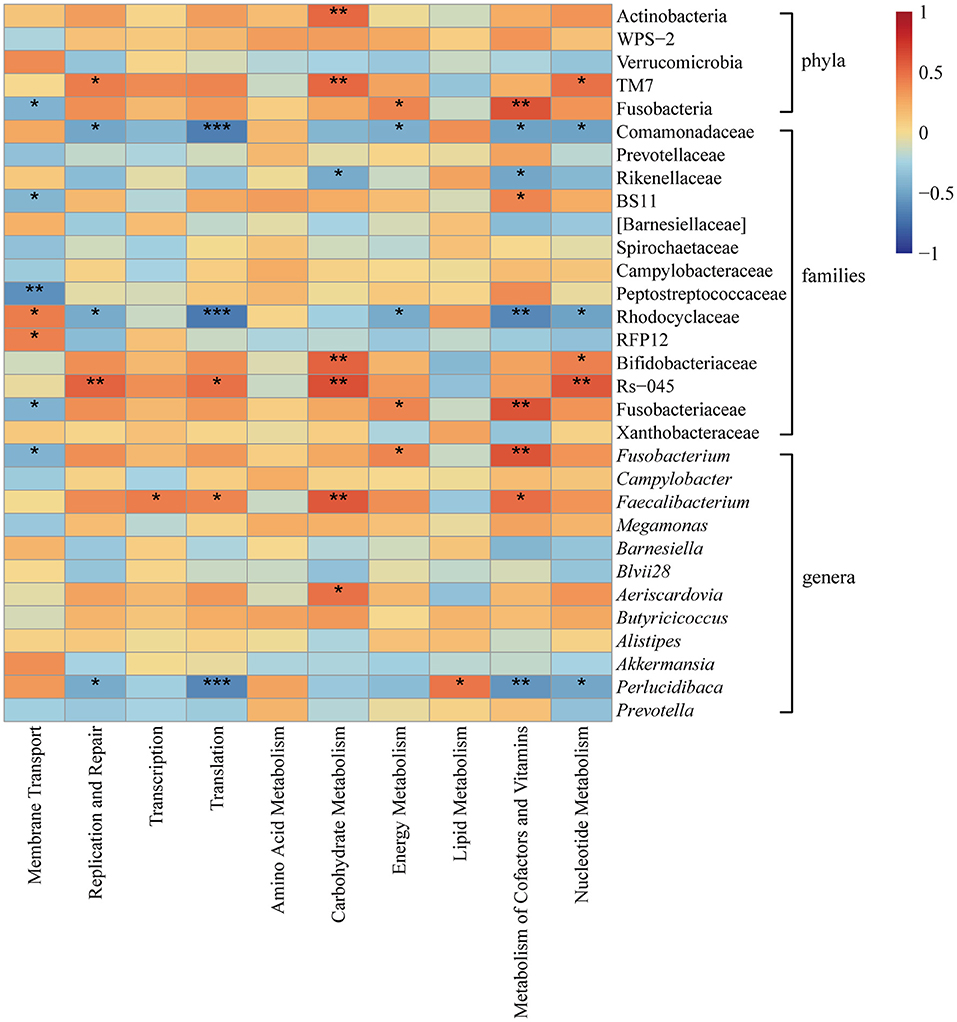
Figure 5. Heatmap illustrating correlation between the predicted gene functions (the KEGG level 2 categories in the top 10) and bacteria with different relative abundances among groups at the phylum, family and genus levels. The color scale indicates the degree of correlation (red: strong positive correlation, blue: strong negative correlation). *P < 0.05, **P < 0.01, ***P < 0.001.
Discussion
Folic Acid Absorption Characteristic in the Intestines of Laying Hens
Plasma folate concentration appeared to reach saturation at a FA concentration between 2 and 4 mg/kg of the hen diet (16). In the present study, the serum folate level reached a plateau at a dietary FA concentration of 6 mg/kg. Perhaps, the strain used in this study had a different sensitivity to FA. The sensitivity of plasma homocysteine to folate status is well-documented the literature, which can be attributed to the role of folate in homocysteine re-methylation to methionine (18). Tactacan et al. (36) reported that the inclusion of crystalline FA or 5-methyltetrahydrofolic acid (5-MTHF) in the hens' diet increased serum and egg folate levels and decreased plasma homocysteine level. Although the effect of FA supplementation on plasma homocysteine level may differ between hen strains, the decrease in homocysteine level is not indefinite and it reaches a plateau, similar to that of serum folate (16). These findings indicate that FA absorption in the intestines, metabolism in the liver, and excretion into the blood is well-regulated. The intestines, liver, and kidneys play essential roles in regulating folate homeostasis in the body.
The mucosal-to-serosal FA uptake rate in the jejunum of laying hens reaches a plateau at FA concentrations higher than 0.1 μM; this pattern is reminiscent of the response demonstrated by laying hens in egg folate concentration when their dietary FA supplementation is increased (24). We assume that a folate homeostasis regulation mechanism exists in intestinal epithelial cells. In the present study, we focused on intestinal factors that might play a role in folate homeostasis. The relative mRNA expression levels of transporters and enzymes involved in folate homeostasis in the duodenum, jejunum, ileum, and cecum of laying hens were compared.
The mRNA expression of enzymes and transporters involved in FA absorption was detected in the four segments of the intestinal tract, indicating the presence of an FA transport system throughout the intestinal tract of laying hens. However, the uptake rate was higher in the duodenum and jejunum than in the ileum and cecum (24). Rfc and Pcft were extensively expressed in most tissues, suggesting that they play important roles in chickens. FA supplementation (10 mg/kg) did not affect the expression of these genes, but jejunal Rfc expression exhibited a decreasing trend with the increase in dietary FA supplementation (21, 22). In the present study, there was no change in Pcft expression levels in the experimental groups, although a tendency for reduced Pcft expression was observed, which concurs with the findings of Jing et al. (22). There was a significant increase in the duodenal Rfc expression levels and a significant decrease in the jejunal Rfc expression levels of hens in the FA1 group compared with those of hens in the other treatment groups, indicating that Rfc expression might be regulated by FA concentration in the intestines. Folate oversupplementation led to down-regulation of intestinal folate uptake in rat, which was caused by down-regulating the expressions of RFC and PCFT in the protein level (37). Under the folate oversupplemented condition, the uptake of folic acid by Caco-2 and HK-2 cell was specifically lower than the folate-sufficient condition, which was associated with the decrease in mRNA levels of RFC, PCFT, and FOLR, and also the decrease in protein level of RFC and the activity of the RFC promoter (38). We speculate that, Rfc, Pcft, and Folr transcription processes and their protein levels may also be regulated in chicken intestine, and the down-regulation of these genes would contribute to a lower folic acid absorption efficiency.
Although apical uptake transporters have been extensively explored, the molecular entities involved in basolateral efflux transport and reverse transport to the lumen are yet to be identified in chickens. ABCC3 is involved in the serosal efflux of FA and leucovorin, as well as 5-MTHF in mice (39), and the down- and up-regulation of ABCC3 expression can influence 2,008 human ovarian cells' folate homeostasis (40). Although duodenal Abcc3 expression was not different between treatment groups, it showed an increasing trend with the increase in dietary FA supplementation, indicating that folate transfer from the intestinal epithelial cells into the bloodstream might be regulated to maintain body folate homeostasis. Jejunal and ileal Abcc3 expression decreased in the FA1 group and then increased in the FA6 and FA24 groups, which may indicate that priority may be given to meet body need when the diet folate is short. Efflux may increase to maintain folate homeostasis when the diet folate is in excess. Ileal Abcc2 expression decreased in treatment groups indicating that there was a balance of folate concentration between intestinal lumen and epithelial cells. Further studies should pursue this.
The DHFR-mediated dihydrofolate reduction can be inhibited by its own substrate folic acid (41) and its production (6s)-5,6,7,8-tetrahydrofolate (42). Duodenal DHFR activity was reported to be lower in birds fed diets supplemented with 10 mg/kg FA than in birds in the control group (36). In the present study, duodenal and ileal Dhfr expression decreased with the increase in dietary FA supplementation, which might be associated with a decrease in DHFR activity.
In summary, FA transport and transfer from the intestinal lumen into enterocytes, and then into the bloodstream, are strictly regulated. It can be concluded that feeding excessively high levels of FA can cause large amounts of unabsorbed FA or its degradants to flow into the cecum of laying hens.
Effect of Folic Acid Supplementation on the Cecum Chyme Microbiota in Laying Hens
Cecal microbiota plays an important role in chicken health, growth performance (43), and egg production (44). Although unabsorbed FA or its degradants flowed into the cecum in the experimental groups, our data suggest that there was no change in the microbial diversity due to FA supplementation. This outcome might be associated with the minor effect of FA supplementation on egg production performance (Appendix Table 1).
Excess consumption of folate by humans can significantly increase the relative abundance of Faecalibacterium, Subdoligranulum, Alistipes, Haemophilus, Desulfovibrio, Prevotella, Odoribacterium, Dialister, and Akkermansia, and significantly decrease the relative abundance of Lachnospiraceae, and Erysipelatoclostridium at the genus level (45). In the present study, the relative abundance of Alistipes, Akkermansia, Barnesiella, Perlucidibaca, and Blvii28 were positively correlated with FA supplementation, whereas the relative abundance of Faecalibacterium, Fusobacterium, Campylobacter, Butyricicoccus, Aeriscardovia, and Megamonas were negatively correlated. The abundance of Prevotella exhibited a quadratic pattern, peaking at 1 mg/kg diet (FA1 group) and decreasing at higher supplementation levels (FA6 and FA24 groups). These discrepancies might be attributed to differences in intestinal microbiota between humans and chickens. Additionally, Escherichia coli and Salmonella are major pathogen in poultry industry (46–48), the biochemical modulation of folate may potentially help the host cope with those challenging situations (49) and increase the host survival (50). Nevertheless, they were not detected by 16S sequencing in the present study, and a further study is required.
Compared with FA0, the relative abundance of TM7, Rs-045 groups in TM7, BS11 groups in Bacteroidetes, Campylobacter, Megamonas, and Fusobacterium were decreased in the cecum of hens fed FA-supplemented diets, which might indicate that these bacterial classes were sensitivity to FA. TM7 is associated with oral inflammation, and its abundance increases in the patient's mouth with gingivitis severity and periodontal disease (51). Fusobacterium is a proinflammatory bacterium. In colorectal cancer tumors, Fusobacterium species are over-represented and a significant co-occurrence was observed for Fusobacterium, Leptotrichia, and Campylobacter species, which was associated with the Interleukin-8 gene and over-expression of other host genes (52). There was a high Fusobacterium abundance in 53-week-old hens with poor egg production, indicating the negative effects of Fusobacterium on chicken health (53). Campylobacter spp. are associated with the gastrointestinal tract of several animals, and Campylobacter jejuni and C. coli cause ~90% of human campylobacteriosis cases (foodborne bacterial gastroenteritis causing bloody diarrhea, fever, and abdominal pain). Chickens are natural hosts for Campylobacter, and chicken meat and eggs are the most important sources of human campylobacteriosis (54). Campylobacter is a commensal microorganism without any obvious clinical signs; however, oral uptake of C. jejuni can significantly affect nutrient absorption, intestinal epithelial barrier activity, and immune reaction, resulting in decreased weight gain in seemingly healthy chickens (55). The BS11 groups in Bacteroidetes participates in multiple pathways for fermenting hemicellulose monomeric sugars to short-chain fatty acids (56). Megamonas species are important propionate and acetate producers (57). In the present study, we found that BS11 and Megamonas were positively but weakly correlated with carbohydrate metabolism. Briefly, dietary FA supplementation can decrease the number of pathogenic bacteria in the intestines and the number of genera associated with short-chain fatty acid production.
There was a significant increase in the relative abundances of Verrucomicrobia, RFP12 family (within Verrucomicrobia), and Barnesiella (within Bacteroidetes), and a significant decrease in the relative abundance of Peptostreptococcaceae (within Firmicutes), Bifidobacteriaceae (within Actinobacteria), and Spirochaetaceae (within Spirochaetes) in the intestines of birds in the FA24 group compared to birds in the other treatment groups. The relative abundance of Akkermansia accounted for the highest proportion in Verrucomicrobia, and it was positively correlated with dietary FA supplementation. Zhang et al. (58) reported that chemical folate modulated the microbiota of rats by increasing the abundance of the genus Akkermansia, which further increased the relative abundance of the phylum Verrucomicrobia to the second most abundant phylum. Verrucomicrobia can improve glucose metabolism in animals (59), and have the potential to induce regulatory immunity (60). Akkermansia is an important microorganism because of its probiotic role, including thickening of the mucous layer, improving gut barrier function (61), enhancing glucose tolerance, reducing insulin resistance, modulating pathways involved in establishing homeostasis for basal metabolism, enhancing immune tolerance against commensal microbiota (62), and prolonging the life of mice from premature aging (63). Barnesiella is an effective immunomodulator that inhibits the colonization of pathogenic antibiotic-resistant bacteria in the gut (64), and promote the nutrient metabolism of chicken (65). Characterized Peptostreptococcaceae species are anaerobic bacteria that include pathogens associated with tissue infections and antibiotic resistance (66). Bifidobacteriaceae are considered to be the most important beneficial microbes in the gut (67), and some species in genus Bifidobacterium have the capacity for de novo folate biosynthesis, and are developed into probiotic products (68). FA supplementation of the laying hen diet at 24 mg/kg increased the relative abundance of beneficial bacteria and decreased the relative abundance of pathogenic bacteria, which were involved in the regulation of immunity. However, there was a decrease in the relative abundance of Bifidobacteriaceae, a beneficial bacterium, in the intestines of birds in the FA24 treatment group, this may suggest the folic acid supplementation associate with probiotics-Bifidobacteria The gene functions of microbiota in the intestines of birds in the FA24 group were significantly different from those of birds in the other treatment groups, indicating that 24 mg/kg FA supplementation may influence folate metabolism in laying hens. In conclusion, FA supplementation at 24 mg/kg was not suitable for laying hens.
According to the findings of our previous work, a suitable amount of dietary FA for folate-enriched egg production is 6 mg/kg of the diet. In this study, compared with the FA1 group, there was a significant decrease in the relative abundance of Prevotellaceae and Prevotella, and gene function associated with unclassified cellular processes and signaling in the intestines of hens in the FA6 group. Prevotellaceae are known degraders of intestinal mucus and can destroy this important intestinal barrier (69). This may indicate that dietary FA supplementation has little effect on the health and metabolism of laying hens for folate-enriched egg production from the microbial perspective.
Conclusions
Serum folate level of laying hens reached a plateau in the FA6 group, which was possibly associated with the regulation of the expression of genes involved in FA absorption in the intestine. Unabsorbed FA may enter the cecum when added in excess, leading to a decrease in the relative abundance of some pathogenic bacteria, especially in the FA24 group. The beneficial bacteria (Bifidobacteriaceae) decreased in FA24 group. Overall, FA supplementation at 6 mg/kg, which was selected for folate-enriched egg production, did not affect the health and metabolism of laying hens negatively.
Data Availability Statement
The datasets presented in this study can be found in online repositories. The names of the repository/repositories and accession number(s) can be found below: http://www.ncbi.nlm.nih.gov/bioproject/747060.
Ethics Statement
The animal study was reviewed and approved by Shanxi Agricultural University Animal Experiment Ethics Committee.
Author Contributions
YY: conceptualization, resources, supervision, project administration, and funding acquisition. YB: methodology, formal analysis, and writing—original draft preparation. RRL: investigation. XTW: date curation. RW and YB: writing—reviewing and editing. All authors have read and agreed to published version of the manuscript.
Funding
The present study was funded by the 1331 Key Discipline Construction of Engineering Animal Husbandry of Shanxi Province (J202011315) and the Research and Application of Healthy Functional Animal Products Production Technology (201703D211001-05).
Conflict of Interest
The authors declare that the research was conducted in the absence of any commercial or financial relationships that could be construed as a potential conflict of interest.
Publisher's Note
All claims expressed in this article are solely those of the authors and do not necessarily represent those of their affiliated organizations, or those of the publisher, the editors and the reviewers. Any product that may be evaluated in this article, or claim that may be made by its manufacturer, is not guaranteed or endorsed by the publisher.
Acknowledgments
We would like to thank relative personnel of Xingmin animal husbandry cooperative (Taigu, China) for guidance of feeding process, we would also like to thank Editage (www.editage.cn) for English language editing.
Supplementary Material
The Supplementary Material for this article can be found online at: https://www.frontiersin.org/articles/10.3389/fvets.2021.720851/full#supplementary-material
References
1. Nassim N, House JD. Recent developments in folate nutrition. Adv Food Nutr Res. (2018) 83:5. doi: 10.1016/bs.afnr.2017.12.006
2. Sukla KK, Nagar R, Raman R. Vitamin-B12 and folate deficiency, major contributing factors for anemia: a population based study. ESPEN J. (2014) 9:1. doi: 10.1016/j.clnme.2013.11.003
3. Boddie AM, Dedlow ER, Nackashi JA, Opalko FJ, Kauwell GP, Gregory III JF, et al. Folate absorption in women with a history of neural tube defect-affected pregnancy. Am J Clin Nutr. (2000) 72:154–8. doi: 10.1093/ajcn/72.1.154
4. Morris MS. Folate, homocysteine, and neurological function. Nutr Clin Care. (2002) 5:3. doi: 10.1046/j.1523-5408.2002.t01-1-00006.x
5. Mason JB, Tang SY. Folate status and colorectal cancer risk: a 2016 update. Mol Aspects Med. (2016) 53:73–9. doi: 10.1016/j.mam.2016.11.010
6. Sijilmassi O. Folic acid deficiency and vision: a review. Graefes Arch Clin Exp Ophthalmol. (2019) 257:8. doi: 10.1007/s00417-019-04304-3
7. Tuszyńska M. Folic acid—the occurrence and the role in human nutrition. Vegetable Crop Res Bull. (2012) 76:1. doi: 10.2478/v10032-012-0003-4
8. Witthöft C, Hefni M. Folic acid and folates: physiology and health effects. In: Caballero B, Finglas P, Toldrá F, editors. The Encyclopedia of Food and Health. Pittsburgh, PA: Academic Press (2016). p. 724–30.
9. Czarnowska M, Gujska E. Effect of freezing technology and storage conditions on folate content in selected vegetables. Plant Foods Hum Nutr. (2012) 67:4. doi: 10.1007/s11130-012-0312-2
10. Delchier N, Ringling C, Grandois JL, Aoudé-Werner D, Galland R, Georgé S, et al. Effects of industrial processing on folate content in green vegetables. Food Chem. (2013) 139:1–4. doi: 10.1016/j.foodchem.2013.01.067
11. Mills JM, Molloy A, Reynolds E. Do the benefits of folic acid fortification outweigh the risk of masking vitamin B12 deficiency? BMJ. (2018) 360:724. doi: 10.1136/bmj.k724
12. Patel KR, Sobczyńska-Malefora A. The adverse effects of an excessive folic acid intake. Eur J Clin Nutr. (2017) 71:2. doi: 10.1038/ejcn.2016.194
13. Selhub J, Rosenberg IH. Excessive folic acid intake and relation to adverse health outcome. Biochimie. (2016) 126:71–8. doi: 10.1016/j.biochi.2016.04.010
14. Altic L, McNulty H, Hoey L, McAnena L, Pentieva K. Validation of folate-enriched eggs as a functional food for improving folate intake in consumers. Nutrients. (2016) 8: 777. doi: 10.3390/nu8120777
16. Hebert K, House JD, Guenter W. Effect of dietary folic acid supplementation on egg folate content and the performance and folate status of two strains of laying hens. Poult Sci. (2005) 84:10. doi: 10.1093/ps/84.10.1533
17. Hoey L, McNulty H, McCann EME, McCracken KJ, Scott JM, Marc BB, et al. Laying hens can convert high doses of folic acid added to the feed into natural folates in eggs providing a novel source of food folate. Br J Nutr. (2009) 101:2. doi: 10.1017/S0007114508995647
18. House JD, O'Connor CP, Guenter W. Plasma homocysteine and glycine are sensitive indices of folate status in a rodent model of folate depletion and repletion. J Agric Food Chem. (2003) 51:15. doi: 10.1021/jf0341621
19. Tactacan GB. Characterization of factors influencing the regulation of dietary folic acid deposition in the eggs (PhD's thesis). University of Manitoba, Winnipeg, MB, Canada (2011).
20. Saini RK, Nile SH, Keum YS. Folates: chemistry, analysis, occurrence, biofortification and bioavailability. Food Res Int. (2016) 89:1. doi: 10.1016/j.foodres.2016.07.013
21. Jing M, Tactacan GB, Rodriguez-Lecompte JC, Kroeker A, House JD. Molecular cloning and tissue distribution of reduced folate carrier and effect of dietary folate supplementation on the expression of reduced folate carrier in laying hens. Poult Sci. (2009) 88:9. doi: 10.3382/ps.2009-00032
22. Jing M, Tactacan GB, Rodriguez-Lecompte JC, Kroeker A, House JD. Proton-coupled folate transporter (PCFT): molecular cloning, tissue expression patterns and the effects of dietary folate supplementation on mRNA expression in laying hens. Br Poult Sci. (2010) 51:5. doi: 10.1080/00071668.2010.508490
23. Visentin M, Diop-Bove N, Zhao R, Goldman ID. The intestinal absorption of folates. Annu Rev Physiol. (2014) 76:1. doi: 10.1146/annurev-physiol-020911-153251
24. Tactacan GB, Rodriguez-Lecompte JC, Karmin O, House JD. Functional characterization of folic acid transport in the intestine of the laying hen using the everted intestinal sac model. Poult Sci. (2011) 90:1. doi: 10.3382/ps.2010-01029
26. Borda-Molina D, Seifert J, Camarinha-Silva A. Current perspectives of the chicken gastrointestinal tract and its microbiome. Comput Struct Biotechnol J. (2018) 16. doi: 10.1016/j.csbj.2018.03.002
27. Svihus B, Choct M, Classen HL. Function and nutritional roles of the avian caeca: a review. Worlds Poult Sci J. (2013) 69:02. doi: 10.1017/S0043933913000287
28. Magnúsdóttir S, Ravcheev D, de Crécy-Lagard V, Thiele I. Systematic genome assessment of B-vitamin biosynthesis suggests co-operation among gut microbes. Front Genet. (2015) 6:148. doi: 10.3389/fgene.2015.00148
29. Caporaso JG, Kuczynski J, Stombaugh J, Bittinger K, Bushman FD, Costello EK, et al. QIIME allows analysis of high-throughput community sequencing data. Nat Methods. (2010) 7:5. doi: 10.1038/nmeth.f.303
30. Chen H, Jiang W. Application of high-throughput sequencing in understanding human oral microbiome related with health and disease. Front Microbiol. (2014) 5:508. doi: 10.3389/fmicb.2014.00508
31. Gill SR, Pop M, DeBoy RT, Eckburg PB, Turnbaugh PJ, Samuel BS, et al. Metagenomic analysis of the human distal gut microbiome. Science. (2006) 312:5778. doi: 10.1126/science.1124234
32. Magoc T, Salzberg SL. FLASH: fast length adjustment of short reads to improve genome assemblies. Bioinformatics. (2011) 27:21. doi: 10.1093/bioinformatics/btr507
33. Edgar RC. Search and clustering orders of magnitude faster than blast. Bioinformatics. (2010) 26:19. doi: 10.1093/bioinformatics/btq461
34. DeSantis TZ, Hugenholtz P, Larsen N, Rojas M, Brodie EL, Keller K, et al. Greengenes, a chimera-checked 16S rRNA gene database and workbench compatible with ARB. Appl Environ Microbiol. (2006) 72:7. doi: 10.1128/AEM.03006-05
35. Altschul SF, Madden TL, Schaffer AA, Zhang JH, Zhang Z, Miller W, et al. Gapped BLAST and PSI-BLAST: a new generation of protein database search programs. Nucleic Acids Res. (1997) 25:17. doi: 10.1093/nar/25.17.3389
36. Tactacan GB, Jing M, Thiessen S, Rodriguez-Lecompte JC, O'Connor DL, Guenter W, et al. Characterization of folate-dependent enzymes and indices of folate status in laying hens supplemented with folic acid or 5-methyltetrahydrofolate. Poult Sci. (2010) 89:4. doi: 10.3382/ps.2009-00417
37. Dev S, Wani NA, Kaur J. Regulatory mechanisms of intestinal folate uptake in a rat model of folate oversupplementation. Br J Nutr. (2011) 105:5. doi: 10.1017/S0007114510004538
38. Ashokkumar B, Mohammed ZM, Vaziri ND, Said HM. Effect of folate oversupplementation on folate uptake by human intestinal and renal epithelial cells. Am J Clin Nutr. (2007) 86:1. doi: 10.1093/ajcn/86.1.159
39. Kitamura Y, Kusuhara H, Sugiyama Y. Basolateral efflux mediated by multidrug resistance-associated protein 3 (mrp3/abcc3) facilitates intestinal absorption of folates in mouse. Pharm Res. (2010) 27:4. doi: 10.1007/s11095-009-0047-4
40. Hooijberg JH, Peters GJ, Assaraf YG, Kathmann I, Priest DG, Bunni MA, et al. The role of multidrug resistance proteins MRP1, MRP2, MRP3 in cellular folate homeostasis. Biochem Pharmacol. (2003) 65:5. doi: 10.1016/S0006-2952(02)01615-5
41. Kao TT, Wang KC, Chang WN, Lin CY, Chen BH, Wu HL, et al. Characterization and comparative studies of zebrafish and human recombinant dihydrofolate reductases-inhibition by folic acid and polyphenols. Drug Metab Dispos. (2008) 36:3. doi: 10.1124/dmd.107.019299
42. Cao HN, Gao M, Zhou HY, Skolnick J. The crystal structure of a tetrahydrofolate-bound dihydrofolate reductase reveals the origin of slow product release. Commun Biol. (2018) 1:1. doi: 10.1038/s42003-018-0236-y
43. Zhang S, Zhong G, Shao D, Wang Q, Hu Y, Wu TX, et al. Dietary supplementation with Bacillus subtilis promotes growth performance od broilers by altering the dominant microbial community. Poult Sci. (2021) 100:3. doi: 10.1016/j.psj.2020.12.032
44. Elokil AA, Magdy M, Melak S, Ishfaq H, Bhuiyan A, Cui L, et al. Faecal microbiome sequences in relation to the egg-laying performance of hens using amplicon-based metagenomic association analysis. Animal. (2020) 14:4. doi: 10.1017/S1751731119002428
45. Gurwara S, Ajami NJ, Jang A, Hessel FC, Chen L, Plew S, et al. Dietary nutrients involved in one-carbon metabolism and colonic mucosa-associated gut microbiome in individuals with an endoscopically normal colon. Nutrients. (2019) 11:613. doi: 10.3390/nu11030613
46. Tawyabur M, Islam MS, Sobur MA, Hossain MJ, Mahmud MM, Paul S, et al. Isolation and characterization of multidrug-resistant Escherichia coli and Salmonella spp. from healthy and diseased Turkeys. Antibiotics. (2020) 9:11. doi: 10.3390/antibiotics9110770
47. Shi SR, Wu S, Shen YR, Zhang S, Xiao YQ, He X, et al. Iron oxide nanozyme suppresses intracellular salmonella enteritidis growth and alleviates infection in vivo. Theranostics. (2018) 8:22. doi: 10.7150/thno.29303
48. Wu S, Shen YR, Zhang S, Xiao YQ, Shi SR. Salmonella interacts with autophagy to offense or defense. Front Microbiol. (2020) 11. doi: 10.3389/fmicb.2020.00721
49. Jing M, Munyaka PM, Tactacan GB, Rodriguez-Lecompte JC, House JD. Performance, serum biochemical responses, and gene expression of intestinal folate transporters of young and older laying hens in response to dietary folic acid supplementation and challenge with Escherichia coli lipopolysaccharide. Poult Sci. (2014) 93:1. doi: 10.3382/ps.2013-03384
50. Sivamaruthi BS, Balamurugan K. Impact of food and folate supplementation during Salmonella Typhi infection in Caenorhabditis elegans. J Proteins Proteomics. (2012) 3:1.
51. Bor B, Bedree JK, Shi W, McLean JS, He X. Saccharibacteria (TM7) in the human oral microbiome. J Dent Res. (2019) 98:5. doi: 10.1177/0022034519831671
52. Warren RL, Freeman DJ, Pleasance S, Watson P, Moore R, Cochrane K, et al. Co-occurrence of anaerobic bacteria in colorectal carcinomas. Microbiome. (2013) 1:1. doi: 10.1186/2049-2618-1-16
53. Kollarcikova M, Kubasova T, Karasova D, Crhanova M, Cejkova D, Sisak F, et al. Use of 16s rRNA gene sequencing for prediction of new opportunistic pathogens in chicken ileal and cecal microbiota. Poult Sci. (2019) 98:6. doi: 10.3382/ps/pey594
54. Soro AB, Whyte P, Bolton DJ, Tiwari BK. Strategies and novel technologies to control campylobacter in the poultry chain: a review. Compr Rev Food Sci Food Saf. (2020) 19:4. doi: 10.1111/1541-4337.12544
55. Awad WA, Hess C, Hess M. Re-thinking the chicken-Campylobacter Jejuni interaction: a review. Avian Pathol. (2018) 47:4. doi: 10.1080/03079457.2018.1475724
56. Solden LM, Hoyt DW, Collins WB, Plank JE, Daly RA, Hildebrand E, et al. New roles in hemicellulosic sugar fermentation for the uncultivated bacteroidetes family BS11. ISME J. (2017) 11:3. doi: 10.1038/ismej.2016.150
57. Polansky O, Sekelova Z, Faldynova M, Sebkova A, Sisak F, Rychlik I. Characterisation of the most important metabolic pathways and biological processes expressed in chicken caecal microbiota. Appl Environ Microbiol. (2015) 82:5. doi: 10.1128/AEM.03473-15
58. Zhang J, Cai DY, Yang M, Hao YJ, Zhu YH, Chen ZX, et al. Screening of folate-producing lactic acid bacteria and modulatory effects of folate-biofortified yogurt on gut dysbacteriosis of folate-deficient rats. Food Funct. (2020) 11:7. doi: 10.1039/D0FO00480D
59. Shin NR, Lee JC, Lee HY, Kim MS, Whon TW, Lee MS, et al. An increase in the akkermansia spp. population induced by metformin treatment improves glucose homeostasis in diet-induced obese mice. Gut. (2014) 63:5. doi: 10.1136/gutjnl-2012-303839
60. Lindenberg F, Krych L, Fielden J, Kot W, Frøkiær H, Galen GV, et al. Expression of immune regulatory genes correlate with the abundance of specific clostridiales and verrucomicrobia species in the equine ileum and cecum. Sci Rep. (2019) 9:12674. doi: 10.1038/s41598-019-49081-5
61. Fujisaka S, Usui I, Nawaz A, Igarashi Y, Okabe K, Furusawa Y, et al. Bofutsushosan improves gut barrier function with a bloom of Akkermansia Muciniphila and improves glucose metabolism in mice with diet-induced obesity. Sci Rep. (2020) 10:5544. doi: 10.1038/s41598-020-62506-w
62. Zhao F, Li CB. Characteristics of intestinal bacterium Akkermansia Muciniphila and the association with host health. Microbiol China. (2017) 44:6. doi: 10.13344/j.microbiol.china.160740
63. Bárcena C, Valdés-Mas R, Mayoral P, Garabaya C, Durand S, Rodríguez F, et al. Healthspan and lifespan extension by fecal microbiota transplantation into progeroid mice. Nat Med. (2019) 25:8. doi: 10.1038/s41591-019-0504-5
64. Ubeda C, Bucci V, Caballero S, Djukovic A, Toussaint NC, Equinda M, et al. Intestinal microbiota containing Barnesiella species cures vancomycin- resistant Enterococcus faecium colonization. Infect Immun. (2013) 81:3. doi: 10.1128/IAI.01197-12
65. Hu Y, Wang LD, Shao D, Wang Q, Wu YY, Han YM, et al. Selectived and reshaped early dominant microbial community in the cecum with similar proportions and better homogenization and species diversity due to organic acids as AGP alternatives mediate their effects on broilers growth. Front Microbiol. (2020) 10:2948. doi: 10.3389/fmicb.2019.02948
66. Murphy EC, Frick IM. Gram-positive anaerobic cocci-commensals and opportunistic pathogens. FEMS Micorbiol Rev. (2013) 37:4. doi: 10.1111/1574-6976.12005
67. Sekirov I, Russell SL, Antunes LCM, Finlay BB. Gut microbiota in health and disease. Physiol Rev. (2010) 90:3. doi: 10.1152/physrev.00045.2009
68. Andlid TA, D'Aimmo MR, Jastrebova J. Folate and Bifidobacteria. In: Mattarelli P, Biavati B, Holzapfel WH, Wood BJB, editors. The Bifidobacteria and Related Organisms: Biology, Taxonomy, Applications. Pittsburgh, PA: Academic Press (2018). p. 195–212.
Keywords: laying hen, folic acid, absorption, gut microbiota, functional prediction
Citation: Bai Y, Wang R, Yang Y, Li RR and Wu XT (2021) Folic Acid Absorption Characteristics and Effect on Cecal Microbiota of Laying Hens. Front. Vet. Sci. 8:720851. doi: 10.3389/fvets.2021.720851
Received: 05 June 2021; Accepted: 26 July 2021;
Published: 17 August 2021.
Edited by:
Shourong Shi, Poultry Institute, Chinese Academy of Agricultural Sciences, ChinaCopyright © 2021 Bai, Wang, Yang, Li and Wu. This is an open-access article distributed under the terms of the Creative Commons Attribution License (CC BY). The use, distribution or reproduction in other forums is permitted, provided the original author(s) and the copyright owner(s) are credited and that the original publication in this journal is cited, in accordance with accepted academic practice. No use, distribution or reproduction is permitted which does not comply with these terms.
*Correspondence: Yu Yang, MzQ1NjA1MjAzQHFxLmNvbQ==