- 1Department of Paraclinical Sciences, Faculty of Veterinary Medicine, Norwegian University of Life Sciences, Ås, Norway
- 2College of Veterinary Medicine, Mekelle University, Mekelle, Ethiopia
- 3Production Animal Clinical Sciences, Faculty of Veterinary Medicine, Norwegian University of Life Sciences, Ås, Norway
- 4Department of Pathology and Parasitology, College of Veterinary Medicine and Agriculture, Addis Ababa University, Bishoftu, Ethiopia
The occurrence and species/genotypes of Cryptosporidium and Giardia duodenalis infecting young livestock in selected districts of Tigray, Ethiopia were investigated, along with risks associated with infection. A total of 757 faecal samples were collected from calves, lambs, and goat kids from four rural districts in Tigray, and also from calves in periurban Mekelle, Tigray's main city, and analysed for Cryptosporidium oocysts and Giardia cysts. Farmers answered questionnaires regarding potential risk factors at sample collection. Immunofluorescent antibody staining was used for parasite detection, and PCR at selected genes and sequencing of positive samples was used for molecular characterisation. The occurrence of Cryptosporidium infection was 10, 9, and 4% in calves, lambs, and goat kids, respectively; equivalent figures for Giardia infection were 39, 32, and 21%. Molecular characterisation of Cryptosporidium isolates revealed C. ubiquitum, subtype XIIa in all three host species; C. ryanae in calves and goat kids; C. andersoni and C. bovis were identified only in calves, and C. xiaoi was identified in lambs. For Giardia, Assemblage E predominated in all host species, but among calf isolates we also identified a few potentially zoonotic genotypes (assemblages A (AI) and Assemblage B). Periparturient care was shown to be a particularly relevant risk factor for infection, and infections were less likely to occur under extensive management systems. Our major findings were widespread occurrence of both parasites in livestock, and the apparent lack of the most common zoonotic species. Our results are discussed in relation to other relevant studies. As our study was conducted in Tigray, further investigation in different settings in Ethiopia could provide relevant information on transmission and zoonotic potential. In addition, given the dependency on healthy animals for the livelihoods of the population of Tigray, investigation of the effect of these common parasites on livestock productivity is important.
Introduction
In developing societies, the livelihoods of many people are dependent on livestock. In most parts of Africa, people and animals live in close proximity, and children have a substantial role in livestock-rearing activities (1). In low and middle-income countries, livestock, primarily cattle and small ruminants (sheep and goats), represent much more than food and nutrition (2) and are also used for income and employment, as well as a means of storing wealth. Livestock is also particularly valuable to women in Africa because it is among the few assets in many societies that women can own and manage themselves (3). Furthermore, livestock manure is used for fertiliser, as fuel for cooking, and for building materials, which are typically prepared by hand by women and children (4). However, this close association between people and their animals also provides the interface for the transmission of zoonotic pathogens (5).
Ethiopia has the largest livestock population in Africa. In Tigray, one of the regional states in northern Ethiopia, the livestock populations are estimated to be approximately 5 x 106 cattle, 2 x 106 sheep, and 5 x 106 goats (6).
There are three main livestock production systems in Ethiopia: extensive, intensive, and semi-intensive (7). In Tigray, the livestock system is usually extensive, with animals mostly kept in small groups in simple pens close to the farmer's home (personal observation). These animals are often under the care of younger family members, and communal grazing is common (7). Drinking water for ruminant livestock in grazing areas is mainly provided from untreated sources (personal observation). Feeding strategies use naturally available forage (pasture and browse), with farmers using crop residues and hay as supplements during the dry season (7).
The protozoan parasites Cryptosporidium (Phylum Apicomplexa) and Giardia (Phylum Fornicata) are extremely common in ruminants, with worldwide distribution (8, 9). Both parasites can cause gastrointestinal disease in infected hosts, sometimes leading to considerable economic losses, and both also have zoonotic potential (8, 10).
Cryptosporidium infection is an important cause of clinical morbidity, mortality, and associated loss of production in ruminant livestock, particularly in young, especially neonatal, animals (8). Clinical signs of cryptosporidiosis in calves include pasty to watery diarrhoea, sometimes accompanied by lethargy, inappetence, fever, dehydration, and poor body condition. Similarly, neonatal lambs and goat kids with cryptosporidiosis have pasty to watery, yellow foul-smelling diarrhoea, anorexia, depression and abdominal pain (11). Infection in older animals is usually subclinical, but can still have a negative effect on production, with a lower body condition score, slower growth rate, and lower carcass weight and dressing percentage at slaughter (11).
Giardiasis in livestock is also characterised by diarrhoea, weight loss, and malabsorption. Infection may also be subclinical, but still affect growth and productivity (9). Chronic Giardia infections tend to occur toward the end of the neonatal period in ruminants, but their importance as a cause of diarrhoea is still ambiguous (8).
Over 30 species and over 70 genotypes of Cryptosporidium have been identified, some of which are host specific (12, 13). The four major Cryptosporidium spp. that infect cattle throughout the world are C. parvum, C. bovis, C. ryanae, and C. andersoni, of which C. parvum and C. andersoni are the two species that have been most associated with clinical disease in cattle (14). In sheep and goats, the most common species identified are C. parvum, C. ubiquitum, and C. xiaoi, and all three can be responsible for mild to severe diarrhoea and mortality (9, 11).
The zoonotic potential of Cryptosporidium depends on the species / genotype (11). C. parvum (subtypes IIa and IId) is the dominant zoonotic species in cattle and humans. Although information is scarce, some evidence suggests that sheep and goats can also be important as reservoirs for the zoonotic transmission of Cryptosporidium, particularly C. parvum (15, 16).
Giardia duodenalis is a species complex with eight distinct assemblages or genotypes, with some degree of host specificity (17–19). Globally, sub-assemblage AI is predominantly found in livestock and to a lesser extent in humans, whereas in most parts of the world, AII is mainly found in humans and a minor proportion in animals. In contrast, AIII has exclusively been found in animals, mainly in wildlife (17). Sub-assemblages AI, AII, BIII, and BIV are considered potentially zoonotic (17).
Among Giardia infections in domestic ruminants (cattle, goats, sheep) globally, including in Africa, Assemblage E is the predominant species reported and is not usually considered zoonotic (20, 21). However, Assemblages A (AI and AII) and B (BIV) have been reported in goats, cattle, and other animal species, both domesticated and wild, from various parts of Africa (18).
Although livestock infections with Cryptosporidium and Giardia have been reported from many parts of Ethiopia (Table 1), all published studies are from Addis Ababa and Oromia region (120 km distance from Addis Ababa) and very few studies included molecular characterisation (species and subtypes of Cryptosporidium or Assemblages of Giardia). The only published article on the molecular study of Cryptosporidium and Giardia in lambs from Addis Ababa and its surroundings reported C. ubiquitum and Assemblage E Giardia (21).
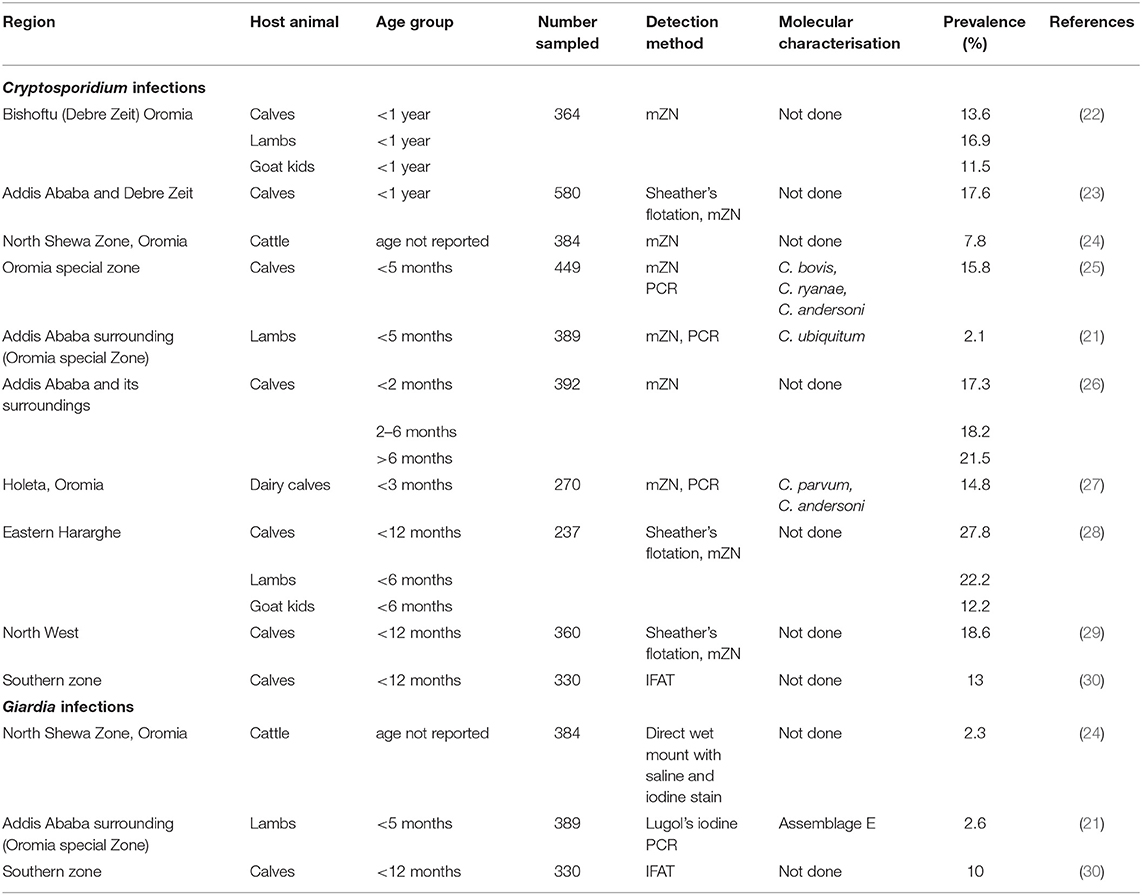
Table 1. Studies from Ethiopia on Cryptosporidium and Giardia infections in ruminant livestock, with detection methods and prevalence data.
The epidemiology, impact, and zoonotic potential of these parasites in livestock in Ethiopia are therefore poorly understood. This is partly because most previous studies in livestock were based solely on light microscopy, using modified Ziehl-Neelsen (mZN) staining (23, 24). This method has poor sensitivity and specificity and cannot be used to identify species (and subtypes) or genotypes. Thus, knowledge on the epidemiology of Cryptosporidium and Giardia in young livestock in Ethiopia would be highly valuable, particularly from regions beyond the area in the vicinity of the capital, Addis Ababa, and also regarding zoonotic (public health) aspects.
This need underpinned the objectives of this study, which were specifically: i) to determine the occurrence of Cryptosporidium and Giardia infection in dairy calves, lambs, and goat kids in Tigray, Northern Ethiopia; ii) to identify the species (and subtypes) of Cryptosporidium and Giardia duodenalis genotypes in infected dairy calves, lambs, and goat kids, and thereby determine the zoonotic potential; and iii) to identify risk factors associated with the infection of livestock with these parasites.
Materials and Methods
Study Areas
The study was carried out between October 2018 and January 2019 in four districts of Tigray, Ethiopia; these are: Enderta (south east zone of the region), Kilte Awulaelo (east), Hintalo Wejirat (south east zone of the region), and Raya Azebo (south zone). Detailed information about these districts can be found in Kifleyohannes et al. (31). In addition, in February 2020, calf samples from urban farms were collected from Mekelle, the capital city of Tigray.
Study Design and Sample Size Determination
A cross-sectional study design, based on a convenience sample rather than randomised sampling, was used to determine the occurrence in livestock from these four districts of Tigray by analysing faecal samples collected from calves, lambs, and goat kids. Sample size was determined by using expected prevalence of 14.8 % for Cryptosporidium and 2.3 % for Giardia infections in calves (24, 27), 16.9 % for Cryptosporidium and 2.6 % for Giardia infections in lambs (21, 22), and 11.5 % for Cryptosporidium in goat kids (22) using the formula given by Thrusfield (32). To calculate the sample size, 5 % absolute precision and 95 % confidence intervals were used for each species.
The calf samples from Mekelle were collected on an availability basis, with input from the city's animal production and health experts regarding where young calves were available for sampling within Mekelle.
Study Animals
Animals were targeted through a door-to-door survey in all selected villages (tabia) at the specified study sites, and all homesteads within each village were contacted. Across the four districts, faecal samples were collected from 208 calves, 270 lambs, and 248 goat kids, all of which were ≤ 6 months of age and kept under intensive, semi-intensive, or extensive management systems. Of the calves, 65 (31%) were ≤ 8 weeks of age, and of these 21 (32%) were under 3 weeks of age.
For calves in Mekelle city, a total of 31 faecal samples were collected from 12 urban farms (two cooperative farms and 10 private farms). All calves were aged ≤ 3 months.
Information was recorded on animal age, sex, management system, faecal characteristics (consistency, colour, presence or absence of mucus), cleanliness of the pen and perianal area. For calves, the breed (local or crossbreed) was also recorded.
Sample Collection and Analysis for Cryptosporidium Oocysts and Giardia Cysts
Approximately 5 g of faeces were collected directly from the rectum of each animal and placed into sampling bottles. The samples were preserved by mixing in 2.5 % potassium dichromate and then refrigerated at 4°C at the Parasitology Laboratory, at the College of Veterinary Medicine, Mekelle University (Mekelle, Ethiopia) before shipping to Norway. Potassium dichromate was removed from the faeces by repeated washings at the Parasitology Laboratory, Faculty of Veterinary Medicine, at the Norwegian University of Life Sciences (Oslo, Norway).
The subsamples were then homogenised, concentrated by centrifugation, and approximately 20 μl of the resulting pellet was placed on a microscope slide using a plastic loop. The samples were air-dried and then fixed with methanol before being analysed by using immunofluorescent antibody test (IFAT), with staining with 15 μl of fluorescein isothiocyanate (FITC)-labelled monoclonal antibody (Aqua-Glo, Waterborne Inc, NO, USA) for the detection of Cryptosporidium or Giardia (oo)cysts. After incubation of the samples and antibody at 37°C for 20 min, 10 μl of 4', 6' diamidino-2-phenylindole (DAPI) was added. After rinsing the samples, a drop of 1,4 diazabicyclo (2.2.2) octane (DABCO) anti-fade mounting medium was added and a coverslip placed over the sample. The stained samples were screened immediately after staining using a fluorescence microscope equipped with appropriate filters (FITC and DAPI) and Nomarski optics.
The entire slide was scanned under the FITC filter and, for presumptive (oo)cysts based on morphometry and staining, the morphology and intactness were checked by light microscopy with Nomarksi optics, as well as visualisation of DAPI staining of nuclei under the DAPI filter. A semi-quantitative measure of infection intensity was determined based on the number of cysts/oocysts per field of view at the objective 20x according to Utaaker, (33): +1 1–9 (oo)cysts, +2 10–50 (oo)cysts, +3 51–100 (oo)cysts, +4 >100 (oo)cysts.
DNA Isolation
The samples containing higher numbers of (oo)cysts or where (oo)cysts were positive for DAPI (and therefore containing nuclei), were preferentially selected for DNA extraction. DNA was isolated using the DNeasy PowerSoil Kit protocol (Qiagen, Oslo, Norway), with slight modifications. The faecal pellet (250 μl) and 60 μl of the lysis solution (solution C1) were added to the PowerBead Tubes and vortexed together to mix. This was then subjected to bead beating to release the DNA by breaking the (oo)cysts walls using a FastPrep-24 5G (MP Biomedicals) in two cycles of 4 m/s for 60 s with a 45 s pause between the cycles. Finally, the DNA was eluted in 50 μl of the elution solution (solution C6) and stored at −20°C. Studies by Elwin et al. (34, 35) reported that the bead-beating approach is effective for obtaining the DNA of Cryptosporidium and Giardia from human stools.
Polymerase Chain Reaction and Sequencing
For samples positive for Cryptosporidium, primers targeting sections of the SSU-rRNA gene and gp60 gene for C. ubiquitum were used for sequence investigation by conventional PCR. Four genes were targeted for genotyping investigations of the Giardia-positive samples: glutamate dehydrogenase (GDH) gene, beta-giardin (BG) gene, triosephosphate isomerase (TPI) gene, and SSU (rRNA) gene. In addition, three genes were used to subtyping Assemblage A samples: DNA repair and recombination gene (RHP26), high cysteine membrane gene (HCMP), and NEK kinase gene. Assemblage B subtyping were performed using the following three genes, 6-phosphogluconate dehydrogenase (pdg), Hypothetical protein, and Phosphorylase B kinase gamma catalytic chain (phkg2). Primers and reaction cycles, as well as other details, for all PCR used in this study are provided in Supplementary File 1.
The PCR products were examined after separation on a 2% agarose gel, stained with SYBERsafe DNA gel stain and visualised under UV illumination. A 100 bp ready-to-use DNA ladder (Thermo Scientific) was used for fragment size determination. Purification of the positive products was carried out using either ExoSAP-IT PCR product clean-up reagent (Thermofisher Scientific) or PureLink Quick Gel extraction and PCR purification Combo kit (Thermo Fisher Scientific) and sent to a commercial company (Eurofins Genomics, Germany) for sequencing in both directions. Sequences were checked using Geneious Prime software, contigs made, and compared with sequences already deposited in GenBank using the Basic Local Alignment Search Tool (NCBI BLAST), along with a recently developed online tool, SSU and gp60 Cryptogenotyper for Cryptosporidium sequences (36).
Questionnaire Survey
A pre-tested questionnaire was used to collect data on the study animals, their owners, and management practises. The questionnaires were filled out during individual interviews with the animal owners by the first author, supported by colleagues from the College of Veterinary Medicine of Mekelle University, along with veterinarians and technicians at the study districts. Data on risk factors of Cryptosporidium and Giardia infections such as age, feeding method, source of drinking water, the occurrence of diarrhoea, pen cleanliness, etc. were collected (see Supplementary File 2 for questionnaire). The questionnaires were designed to contain mainly closed-ended questions.
Statistics
A database was created in Excel and analysed using STATA 15 software (STATA SE Corp, TX, USA) software. The Chi-square test and Fisher's exact test were used to evaluate the association between risk factors for Cryptosporidium and Giardia infection. Variables significant at P < 0.05 in the above tests were considered as candidates for a multivariable model. Subsequently, a backward stepwise logistic regression was used to identify possible predictors of the Cryptosporidium and Giardia infection out of the following variables: districts, periparturient care, weaning age, management system and breed. At each step, the variable with the highest p-value in the multivariable logistic regression model was removed before re-running the multivariable logistic regression model and these iterations of elimination were continued as long as the values of the Akaike information criterion (AIC) of the new models were decreasing. The confidence level was maintained at 95 % and P < 0.05 was considered for the significance level.
Results
Overview of Cryptosporidium and Giardia Infections in Calves, Lambs, and Goat Kids From the Four Districts
The overall combined occurrence of Cryptosporidium spp. and Giardia spp. in the four districts in all livestock (calves, lambs, and goat kids) were 8 % (56/726) and 30 % (220/726), respectively. Investigation of associations between the occurrence of infection and livestock species showed statistically significant differences for both Cryptosporidium (P = 0.024) and Giardia (P = 0.001). The highest occurrence of Cryptosporidium was observed in calves and the lowest in goat kids, and the same pattern was found with Giardia.
Statistically significant differences (P = 0.015) were found between the occurrences of Cryptosporidium in the four districts: the highest occurrence was in Enderta (11 %) and the lowest occurrence was found in Hintalo Wejirat (3 %). No statistically significant difference was observed between the presence of Giardia in the four districts.
The intensity of Cryptosporidium infection between livestock species was as shown in Figure 1, with most infected animals shedding relatively low numbers of oocysts (+1). The intensity of infection in Giardia-infected animals varied between livestock species. Although most infected animals shed only low quantities of cysts (+1), relatively high Giardia-cyst counts were recorded in several calves.
The occurrence of Giardia infection among the three species of the study animals was compared in different age groups of animals using the chi-square test. Calves and lambs ≤ 8-weeks were more likely to have Giardia infection than calves and lambs of >8 weeks, whereas the opposite was seen for goat kids; goat kids over 8 weeks were more likely to be infected with Giardia than goat kids of 8 weeks and younger (Table 2). Although Cryptosporidium infections tended to occur more often within the younger age group for all livestock species, no clear age-related associations were identified.
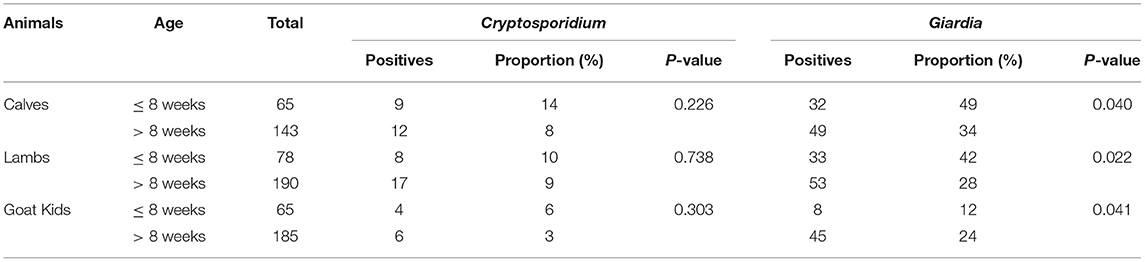
Table 2. Comparison of the occurrences of Cryptosporidium and Giardia infections between different age groups of calves, lambs, and goat kids.
Cryptosporidium and Giardia Infections in Calves by District and Different Management Systems
The Chi-square test showed a significant difference in Cryptosporidium infection in calves in all four districts. The occurrence of infection in the calves of Kilte Awulaelo district (21%) was significantly higher than that in the other districts; see Supplementary File 3 (Supplementary Table 1). The occurrence of infection was significantly higher in crossbreed calves (33 %) than in local breed calves (7%); see Supplementary File 3 (Supplementary Table 1).
However, when multivariable logistic regression analysis was used, only management system and periparturient care were found to be significant. Calves in an intensive management system were more likely to be infected with Cryptosporidium than calves in an extensive management system (OR = 7.8, 95% CI = 2.46–24.49: P = 0.001). Calves that stayed in the same barn with cows after birth were at higher risk of Cryptosporidium infection than calves that stayed in their pen alone or with other calves (OR = 3.2, 95% CI = 1.02–10.08; P = 0.04).
The occurrence of Giardia infection in calves was similar among the four districts and with respect to their periparturient care, weaning age, type of feed supplement, type of management system, and breeds see Supplementary File 3 (Supplementary Table 2).
In calves in urban farms in Mekelle city, out of 31 calf faecal samples collected from 12 urban farms, 13% contained Cryptosporidium spp. oocysts and 35% contained Giardia cysts.
Molecular Characterisation of Cryptosporidium and Giardia From Calf Infections
Of the 21 Cryptosporidium-positive samples from the four districts, species determination was attempted on 15, but only nine were successfully amplified and sequenced. Additionally, of the four Cryptosporidium-positive calf samples from Mekelle city, molecular characterisation was successful for three. Accordingly, C. andersoni (n = 1), C. bovis (n = 3), C. ryanae (n = 7), and C. ubiquitum (n = 1) were identified from the four districts, whereas only C. andersoni and C. ryanae were identified in the urban farms. The C. ubiquitum sample was subtyped and found to belong to the XIIa family.
Of the 81 Giardia-positive calf samples from the four districts, 31 were selected for molecular characterisation, of which 18 provided good sequence results; 14 were Assemblage E, three were Assemblage A, and one was Assemblage B. Subtyping the Assemblage A isolates revealed that two were AI and one could not be subtyped. Subtyping of the Assemblage B using multilocus sequence typing showed a close relationship with Algerian samples (37). Out of 11 Giardia-positive calf samples from urban farms, molecular characterisation was successful for six and all were Assemblage E.
Representative sequences have been deposited in GenBank and can be found under Accession numbers OK336067, OK336072-OK336079, OK336082-83 and OK358701-OK358702 (Cryptosporidium) and OK523961-OK523964, OK523969-OK523975, OK626272-OK626274, OK626276-OK626277 (Giardia).
Cryptosporidium Infection in Lambs and Goat Kids by District and Different Management Systems
Based on the Chi-square test, the occurrence of Cryptosporidium infection in lambs showed significant differences among the four districts. The occurrence of infection in lambs in the Enderta district (16%) was significantly higher than in the other districts; see Supplementary File 3 (Supplementary Table 3). However, such differences were not observed in goat kids among different districts. The only significant difference was that the occurrence of Cryptosporidium in goat kids housed with adults and other animals (9%) was higher than in goat kids kept with does in their pen (1.3%) see Supplementary File 3 (Supplementary Table 4). Lambs kept under a semi-intensive management system had a significantly higher occurrence of Cryptosporidium infection (19%) than lambs reared under an extensive management system (7%); see Supplementary File 3 (Supplementary Table 3).
However, analysis using multivariable logistic regression only showed a significantly higher occurrence of Cryptosporidium infection in lambs housed with adults and other animals than lambs kept with ewes in their pen (OR = 3.7, 95% CI = 1.12–11.19; P = 0.020). Lambs from Enderta were more likely to be infected with Cryptosporidium infection than lambs from Kilte Awulaelo and Hintalo Wejirat (OR = 5.1, 95% CI =1.07–23.88; P = 0.040). Lambs from Raya Azebo were more likely to be infected with Cryptosporidium infection than lambs from Kilte Awulaelo and Hintalo Wejirat (OR = 6.1, 95% CI = 1.14–32.4; P = 0.035).
Giardia Infection in Lambs and Goat Kids by District and Different Management Systems
Analysis using Chi-square test showed that the occurrence of Giardia infection in lambs was similar among the four districts, and not affected by age at colostrum feeding and type of feed supplement. However, lambs, but not goat kids, kept under semi-intensive management had a significantly higher occurrence of Giardia infection (44%) than lambs reared in extensive management (29%); see Supplementary File 3 (Supplementary Tables 5, 6). Multivariable logistic regression revealed that lambs housed with ewes and other animals were more likely to be infected with Giardia than lambs kept with ewes in their pen (OR = 2.7, 95% C = 1.42–5.21; P = 0.002).
Molecular Characterisation of Cryptosporidium and Giardia From Lamb Infections
Out of 93 lamb faecal samples positive for Cryptosporidium oocysts and/or Giardia cysts, DNA was extracted from 20. Of the 25 Cryptosporidium-positive lamb samples, only seven were selected for molecular characterisation. For five of them, amplification was successful and resulted in good sequences; three were identified as C. ubiquitum and two as C. xiaoi. All three C. ubiquitum isolates were subtyped as belonging to the XIIa family.
Of the 86 Giardia-positive lamb samples, most were very light infections containing few cysts; however, 17 were selected for molecular characterisation, and amplification and sequencing were successful for 12 of these. All the samples were found to be Assemblage E.
Representative sequences have been deposited in GenBank and can be found under Accession numbers OK336068-OK336070, OK336084-OK336087, OK358703-OK358704 (Cryptosporidium) and OK523965-OK523966, OK523976, OK626275 (Giardia).
Molecular Characterisation of Cryptosporidium and Giardia From Goat Kid Infections
Out of 61 goat kid faecal samples positive for Cryptosporidium oocysts and/or Giardia cysts, DNA was extracted from 11. Of the three Cryptosporidium-positive goat-kid samples selected for molecular characterisation, DNA from two of them was amplified and sequenced successfully and revealed C. ryanae and C. ubiquitum. The latter was subsequently identified as the XIIa subtype.
Of 10 Giardia-positive goat kids samples selected for molecular characterisation, only six samples provided good sequence results at one or more genes; all were identified as Assemblage E.
Representative sequences have been deposited in GenBank and can be found under Accession numbers OK336071, OK336080-81 and OK358705-06 (Cryptosporidium) and OK523967-OK523968, OK523977-OK523978 (Giardia).
Discussion
Cryptosporidium and Giardia Infections in Calves, Lambs, and Goat Kids
The main finding of this study is that both parasites occur widely and frequently within livestock in rural Tigray, with a significantly higher occurrence in calves than small ruminants, particularly goat kids. The low infection in goat kids may reflect that they are browsers, and thus the likelihood of ingesting the transmission stages of these parasites from the ground is lower than for the other grazing species (38). Another important finding was that in the four study districts, Cryptosporidium infection of livestock occurred significantly less frequently than Giardia infection and a similar pattern was seen among calves from the urban farms in Mekelle. However, for calves, more variables had significant association with Cryptosporidium infection than with Giardia infection. This might be attributed to the robustness of Cryptosporidium and survival capability to stay in the environment and contaminate the barn. In addition, in the study areas, although intensive management and cross breeds were reported by some farmers concerning calves, for lambs and goat kids only local breeds were reported, with extensive management almost exclusively predominating, and intensive management not reported at all. This is in line with a report by Vermeulen et al. (39), who found that intensively managed calves are the dominant source of oocysts compared with other livestock species. Although Giardia cysts are robust and can survive well in aquatic environments, they are relatively fragile compared with Cryptosporidium oocysts and are unlikely to survive for prolonged periods in the animal houses (40).
Unlike in our study, other studies of livestock in Ethiopia for both parasites have tended to find higher prevalences of Cryptosporidium infection than Giardia (see Table 1). However, most other studies focused only on Cryptosporidium, and generally report higher prevalences than found in our investigation. Differences in occurrence for both parasites should be expected between studies, due to variations in management practises, transmission possibilities, host susceptibilities, and other parameters. Of particular relevance may be that most of the samples in our study were from extensively managed animals, but in some of the other studies from Ethiopia the animals were intensively managed [e.g., 22, 28]. Intensive management may promote transmission, due to closer contact between infected animals and build-up of oocyst contamination within a restricted environment.
However, it also seems likely that methodological specificities and sensitivities may be of relevance. Most other studies from Ethiopia used mZN for detection of Cryptosporidium (21, 24, 27), which is less sensitive and less specific than the IFAT technique used in our study. A study from Tanzania on Cryptosporidium in calves (41) found that misidentification occurred when mZN was used, and commented that false-positive results may occur when mZN is the sole detection method used.
There are only three published reports from Ethiopia on the prevalence of Giardia in livestock (Table 1), and our results describe a considerably higher prevalence than all the previous studies. Methodological differences may also play a role here, as a less sensitive method (light microscopy with Lugol's iodine staining) was used in one study for identifying Giardia infection in lambs (21). However, another study that investigated Giardia shedding in calves in southern Ethiopia (30) used IFAT, but also found a much lower prevalence (10%; see Table 1).
Although both infections were found in all species of livestock in all districts investigated, and occurrences were similar for Giardia among districts, for Cryptosporidium the occurrences varied significantly according to district. That we found the highest occurrence of Cryptosporidium infection in Enderta followed by Kilte Awulaelo, is interesting as surveys of fresh produce and water in all four districts found contamination with these parasites in these two districts only (31, 42). This might suggest that this parasite is circulating more successfully among animals and in the environment in these two districts.
Most animals included in our study had relatively low intensities of infection. Most studies from different parts of the world do not report the intensity of infection. The low levels of intensity of infection found in our study may reflect that the majority of samples were collected from extensive management systems where trickle infections, with concomitant build-up of immunity, may be more likely to occur than abrupt exposure to a massive infectious dose.
The prevalence of Cryptosporidium infection was similar in the different age groups of animals investigated in our study, although there was a trend that the occurrence was higher within the younger age group for all livestock species. In a report from Nigeria, young calves (<3 months old) had higher infection rates of Cryptosporidium than calves > 3 months old (43).
The occurrence of Giardia infection was higher in calves and lambs ≤ 8weeks old than older calves and lambs suggesting that the infection rates decline as the age of the animals increases. Similar results have been reported in other countries. In Canada and China the prevalence of Giardia infection was greater in calves and lambs than adults (44, 45) and this is mostly consistent with our findings. However, we found that Giardia infection in goat kids was significantly higher in the age group > 8 weeks than ≤ 8 weeks old.
Compared with Giardia cyst excretion from calves and lambs, relatively few cysts were found in most Giardia-positive goat kids. This low shedding of Giardia cysts from infected goat kids might be related to goats being selective browsers, and therefore less exposed to infectious cysts.
Risk Factors for Cryptosporidium and Giardia Infections in Calves, Lambs, and Goat Kids
We found that Cryptosporidium infection occurred more often in crossbreed calves than local breeds. The potential effect of cattle breed on Cryptosporidium infection has been previously discussed (13). In a study from Malaysia (46), it was found that Mafriwal cattle (Sahiwal × Friesian crosses) and Jersey × Friesian crosses had higher rates of infection. This could also be due to the different management system, as crossbreed calves tended to be intensively managed compared with local breeds. Higher rates of Cryptosporidium infection in lambs under semi-intensive systems probably reflects the relatively high confinement in semi-intensive management, which results in greater crowding and a more contaminated environment than in farms using an extensive system. No significant difference was observed in goat kids under both management systems in our study.
Calves housed with cows had a significantly higher Cryptosporidium infection than calves housed in their pen alone or with other calves in our study; this finding is similar to those reported by Manyazewal et al. (27). This strong association between periparturient care and Cryptosporidium infection in calves is probably associated with the level of hygiene when calves are kept alone or with other animals. This suggestion is supported by Abebe et al. (23), who reported a substantial connexion between Cryptosporidium infection and the hygienic status of dairy animals and their farms. Similarly, Ayele et al. (29) stated that poor hygiene increases the infection rate and spread of Cryptosporidium among animals. This is probably because dirty and muddy farms may create favourable conditions for the presence or survival of Cryptosporidium oocysts. This increases the likelihood of feed and water contamination, which, in turn, might favour exposure of calves to Cryptosporidium oocysts. We found similar results for ewes and lambs and for goat kids and does housed together in a single pen, and, again, a likely explanation is close contact between the animals and contamination, along with less exposure to inactivation pressures such as desiccation and UV-irradiation (13).
Molecular Characterisation of Cryptosporidium and Giardia in Calves, Lambs, and Goat Kids
Perhaps the most important finding of this cross-sectional study is that, despite the close contact between animals and humans, the main zoonotic species and subtypes of Cryptosporidium and Giardia were not detected in any of the animal samples. However, species such as C. andersoni and C. ubiquitum, which are usually considered to have limited zoonotic potential (47, 48), were detected. Similarly, the Giardia assemblages identified were mostly non-zoonotic Assemblage E, but a few Giardia isolates detected in calves were of the potentially zoonotic Assemblages A and B.
Globally, four species of Cryptosporidium occur commonly in cattle: C. parvum, C. bovis, C. ryanae and C. andersoni (14). However, the majority of studies from Africa have indicated that C. parvum occurs relatively infrequently in cattle here (13). Nevertheless, one study from Egypt reported all the common Cryptosporidium spp., except for C. andersoni (49), and, in Ethiopia, all four common species have been reported in calves from the Oromia region by Wegayehu et al. (25) and Manyazewal et al. (27). Our findings seem more similar to those from a study from Nigeria that reported all species of Cryptosporidium from calves, except C. parvum (43). It could be argued that C. parvum was not detected in calves in our study, as it is more common in very young calves. Of the 65 calves ≤ 8 weeks old included in our study, the age range was from 4 days, with 21 ≤ 3 weeks of age. Interestingly, our findings also differ from other reports from Africa, as we also detected C. ubiquitum, subtype XIIa in cattle. C. ubiquitum is considered an emerging zoonotic pathogen, with a wide geographic distribution and broad host range (47). However, to the best of our knowledge, there is only a single report of human infection with C. ubiquitum in Africa, with just one child from Nigeria reported to be infected with C. ubiquitum (then described as cervine genotype) among a survey of 692 children, of whom 134 were considered to be infected with Cryptosporidium (50). Thus, it appears that zoonotic transmission of this species of Cryptosporidium is not well established in Africa; reasons for this are discussed in the review article of Robertson et al. (13).
Globally, the most common species of Cryptosporidium identified in sheep are C. ubiquitum and C. xiaoi, although in some European countries C. parvum is also prevalent in sheep (51). The report by Robertson et al. (13), that C. parvum occurs relatively infrequently in African small ruminants, with C. xiaoi and C. ubiquitum predominating, is in line with our findings.
In goats, the most common Cryptosporidium spp. identified from different parts of the world are C. ubiquitum, C. xiaoi, and C. parvum (9, 52, 53). However, in our study only C. ryanae and C. ubiquitum were detected in goat kids. A recent review of Cryptosporidium in goats (38), reported that in studies from different parts of the world (Europe, Asia, Africa, South America, and Oceania) where C. ubiquitum has been reported, only subtype-XIIa has been detected (where subtyping has been conducted) and we also found this sub-type.
The Giardia assemblages identified in this study indicated that calves mainly carried Assemblage E infections and all Giardia isolates from lambs and goat kids belonged to Assemblage E. This Assemblage, which is generally not considered zoonotic, is usually found in cattle, sheep, goats, and pigs (9, 17). However, we did identify a few potentially zoonotic Assemblages in calves, with three Assemblage A isolates and one Assemblage B isolate, and consistent results among the different genetic loci amplified by PCR. Our finding of Assemblage B in calves is rather unusual, but Sprong et al. (17) also noted a very low percentage of Assemblage B in cattle. Our case could, potentially, indicate carriage rather than infection. Subtyping of two Assemblage A isolates revealed AI, which is mainly found in animals (17).
Conclusion
Our study found a widespread occurrence of Cryptosporidium and Giardia in livestock in Tigray, Ethiopia. Five Cryptosporidium species, namely C. bovis, C. ryanae, C. andersoni, C. xiaoi, and C. ubiquitum, were identified in calves, lambs, and goat kids, with C. ryanae and C, ubiquitum being the predominant species. The zoonotic species, C. parvum, was not detected in any of the animal samples. Similarly, the majority of the Giardia assemblages detected in the samples were Assemblage E.
Periparturient care seemed to be particularly important regarding these infections in livestock, with crowding among calves, lambs, and goat kids, particularly mixing with adults and/or other animals, increasing the vulnerability to infection with both these parasites. Similarly, if the management system was more intensive, then the likelihood of infection was greater.
Conducting further research from different locations and settings in Ethiopia could provide relevant information regarding transmission dynamics for both parasites and the potential for zoonotic transmission. It would also be important to ascertain the effect that these parasites have on the productivity of the livestock, given that the farmers are dependent on healthy animals for their livelihoods.
Data Availability Statement
The datasets presented in this study can be found in online repositories. The names of the repository/repositories and accession number(s) can be found at: https://www.ncbi.nlm.nih.gov/genbank/, OK336067, OK336072-OK336079, OK336082-83, OK358701-OK358702; OK523961-OK523964, OK523969-OK523975, OK626272-OK626274, OK626276-OK626277, OK336068-OK336070, OK336084-OK336087, OK358703-OK358704, OK523965-OK523966, OK523976, OK626275, OK336071, OK336080-81, OK358705-06, OK523967-OK523968 and OK523977-OK523978.
Ethics Statement
The animal study was reviewed and approved by the Regional Committee for Medical and Health Research Ethics, South East; REK, SE, case number 2018/1279 C and the National Research Ethics Review Committee in Ethiopia (Ref. No: MoSHE/RD/144/1095/19). Written informed consent was obtained from the owners for the participation of their animals in this study.
Author Contributions
TK, AN, JD, GT, and LR: conceptualization, methodology, and writing-review and editing. LR: validation. TK, AN, JD, and LR: formal analysis. TK: investigation, data curation, and writing original draft preparation. TK and LR: visualization and funding acquisition. LR, AN, JD, and GT: supervision. LR: project administration. All authors have read and agreed to the published version of the manuscript.
Funding
This study was funded by the Norwegian Ministry of Foreign Affairs through the MU-NMBU Institutional Collaboration Phase IV, Improved Livelihood of the Rural Communities in the Arid Highlands of Northern Ethiopia.
Conflict of Interest
The authors declare that the research was conducted in the absence of any commercial or financial relationships that could be construed as a potential conflict of interest.
Publisher's Note
All claims expressed in this article are solely those of the authors and do not necessarily represent those of their affiliated organizations, or those of the publisher, the editors and the reviewers. Any product that may be evaluated in this article, or claim that may be made by its manufacturer, is not guaranteed or endorsed by the publisher.
Acknowledgments
We are very grateful to all participants involved in this study. We are also grateful for project support from Kristoffer Tysnes and statistical analysis support from Gebreyohans Tesfaye Gebregiwergis, respectively. In addition, we are grateful to Kjersti Selstad Utaaker for assistance with the analytical methodology. We would also like to thank Habtamu Taddele, Yohannes Hagos, Biruk Mekonnen, Tadesse Teferi, and Haftay Abraha for their support and assistance during the sample collection. Finally, we express our gratitude to all drivers, district veterinarians, and animal owners who helped during sample collection.
Supplementary Material
The Supplementary Material for this article can be found online at: https://www.frontiersin.org/articles/10.3389/fvets.2021.825940/full#supplementary-material
References
1. Swanepoel F, Stroebel A, Moyo S. The Role of Livestock in Developing Communities: Enhancing Multifunctionality. South Africa: UFS and CTA (2010). p. 2.
2. Bettencourt EMV, Tilman M, Narciso V, da Silva Carvalho ML, de Sousa Henriques PD. The livestock roles in the wellbeing of rural communities of Timor-Leste. Rev Econ e Sociol Rural. (2015) 53:S063–80. doi: 10.1590/1234-56781806-94790053s01005
3. Sanginga PC, Njuki J, Waithanji E. Women, livestock ownership and markets: Bridging the gender gap in Eastern and Southern Africa. Improving the design and delivery of gender outcomes in livestock research for development in Africa. New York: Routledge (2013). p. 2.
4. Tegegne A. Urban livestock production and gender in addis ababa, Ethiopia. Urban Agric Mag. (2002) 30–1.
5. Chen D, Mechlowitz K, Li X, Schaefer N, Havelaar AH, McKune SL. Benefits and risks of smallholder livestock production on child nutrition in low-and middle-income countries. Front Nutr. (2021) 8:836. doi: 10.3389/fnut.2021.751686
6. CSA (Central Statistical Agency). Agricultural Sample Survey 2020 / 2021 (2003 E.C.) II. Report on livestock and livestock characteristics (Private Peasant Holdings), CSA, Statistical Bulletin 589. Addis Ababa, Ethiopia (2020). p. 5–46.
7. Makkar HPS, Addonizio E, Gizachew L. Charcterization of feeding systems in Ethiopia with a focus on dry areas. Broadening Horizons. (2018) 51:1–9.
8. O'Handley RM, Olson ME. Giardiasis and cryptosporidiosis in ruminants. Vet Clin North Am - Food Anim Pract. (2006) 22:623–43. doi: 10.1016/j.cvfa.2006.07.002
9. Santin M. Cryptosporidium and Giardia in ruminants. Vet Clin North Am-Food Anim Pract. (2020) 36:223–38. doi: 10.1016/j.cvfa.2019.11.005
10. Shaw HJ, Innes EA, Morrison LJ, Katzer F, Wells B. Long-term production effects of clinical cryptosporidiosis in neonatal calves. Int J Parasitol. (2020) 50:371–6. doi: 10.1016/j.ijpara.2020.03.002
11. Robertson LJ, Bjorkman C, Axen C, Fayer R. Cryptosporidiosis in Farmed Animals. In: Caccio SM, Widmer G, editors. Cryptosporidium: Parasite and Disease. Wien, Springer (2014). p. 149–80.
12. de Lucio A, Bailo B, Aguilera M, Cardona GA, Fernández-Crespo JC, Carmena D. No molecular epidemiological evidence supporting household transmission of zoonotic Giardia duodenalis and Cryptosporidium spp. from pet dogs and cats in the province of Álava, Northern Spain. Acta Trop. (2017) 170:48–56. doi: 10.1016/j.actatropica.2017.02.024
13. Robertson LJ, Johansen ØH, Kifleyohannes T, Efunshile AM, Terefe G. Cryptosporidium infections in Africa—how important is zoonotic transmission? a review of the evidence. Front Vet Sci. (2020) 7. doi: 10.3389/fvets.2020.575881
14. Thomson S, Hamilton CA, Hope JC, Katzer F, Mabbott NA, Morrison LJ, et al. Bovine cryptosporidiosis: impact, host-parasite interaction and control strategies. Vet Res. (2017) 48:42. doi: 10.1186/s13567-017-0447-0
15. Guo Y. Li, N, Ryan, U, Feng, Y, Xiao, L. Small ruminants and zoonotic cryptosporidiosis. Parasitol Res. (2021) 120:4189–98. doi: 10.1007/s00436-021-07116-9
16. Robertson LJ. Giardia and Cryptosporidium infections in sheep and goats: A review of the potential for transmission to humans via environmental contamination. Epidemiol Infect. (2009) 137:913–21. doi: 10.1017/S0950268809002295
17. Sprong H, Cacciò SM, Van Der Giessen JWB. Identification of zoonotic genotypes of Giardia duodenalis. PLoS Negl Trop Dis. (2009) 3:1–12. doi: 10.1371/journal.pntd.0000558
18. Lyu Z, Shao J, Xue M, Ye Q, Chen B, Qin Y, Wen J. A new species of Giardia künstler, 1882 (Sarcomastigophora: Hexamitidae) in hamsters. Parasit Vectors. (2018) 11. doi: 10.1186/s13071-018-2786-8
19. Thompson RCA, Ash A. Molecular epidemiology of Giardia and Cryptosporidium infections-what's new? Infect genet evol. (2019) 75:103951. doi: 10.1016/j.meegid.2019.103951
20. Squire SA, Ryan U. Cryptosporidium and Giardia in Africa: current and future challenges. Parasit Vectors. (2017) 10:195. doi: 10.1186/s13071-017-2111-y
21. Wegayehu T, Karim MR Li J, Adamu H, Erko B, Zhang L, Tilahun G. Prevalence and genetic characterization of Cryptosporidium species and Giardia duodenalis in lambs in Oromia Special Zone, Central Ethiopia. BMC Vet Res. (2017) 13:22. doi: 10.1186/s12917-016-0916-0
22. Ayana D, Alemu B. Cryptosporidiosis in calves, lambs and goat kids in Bishoftu, Oromia Regional State, Ethiopia. African J Basic Appl Sci. (2015) 7:233–9. doi: 10.5829/idosi.ajbas.2015.7.5.95160
23. Abebe R, Wossene A, Kumsa B. An epidemiological study of Cryptosporidium infection in dairy calves on selected dairy farms of central Ethiopia. Revue de Médecine Véterinaire. (2008) 159:107–11.
24. Wegayehu T, Adamu H, Petros B. Prevalence of Giardia duodenalis and Cryptosporidium species infections among children and cattle in North Shewa Zone, Ethiopia. BMC Infect Dis. (2013) 13:419. doi: 10.1186/1471-2334-13-419
25. Wegayehu T, Karim MR, Anberber M, Adamu H, Erko B, Zhang L, Tilahun G. Prevalence and genetic characterization of Cryptosporidium species in dairy calves in central Ethiopia. PLoS ONE. (2016) 11. doi: 10.1371/journal.pone.0154647
26. Manyazewal A, Francesca S, Pal M, Gezahegn M, Tesfaye M, Lucy M, et al. (2018). Prevalence, risk factors and molecular characterization of Cryptosporidium infection in cattle in Addis Ababa and its environs, Ethiopia. Vet Parasitol Reg Stud Reports. 13:79–84. doi: 10.1016/j.vprsr.2018.03.005
27. Manyazewal A, Francesca S, Gezahegn M, Getachew T. Cryptosporidium infection in dairy cattle calves and its public health significance in central Ethiopia. J Adv Vet Res. (2017) 7:59–65.
28. Regassa A, Gizaw O, Abunna F, Abebe R, Beyene D, Megersa B, et al. Cryptosporidium in calves, lambs and kids at Haramaya, eastern Ethiopia. Ethiop Vet J. (2013) 17:81. doi: 10.4314/evj.v17i1.7
29. Ayele A, Seyoum Z, Leta S. Cryptosporidium infection in bovine calves: Prevalence and potential risk factors in northwest Ethiopia. BMC Res Notes. (2018) 11:1–6. doi: 10.1186/s13104-018-3219-7
30. Hailu M, Asmare K, Gebremedhin EZ, Sheferaw D, Gizaw D, Di Marco V, et al. Cryptosporidium and Giardia infections in dairy calves in southern Ethiopia. Parasite Epidemiol Control. (2020) 10:e00155. doi: 10.1016/j.parepi.2020.e00155
31. Kifleyohannes T, Debenham JJ, Robertson LJ. Is fresh produce in Tigray, Ethiopia a potential transmission vehicle for Cryptosporidium and Giardia? Foods. (2021) 10:1979. doi: 10.3390/foods10091979
33. Utaaker KS. Intestinal protozoan parasites in Northern India–investigations on transmission routes (Doctoral dissertation). Oslo, Norway: Norwegian University of Life Sciences (2018).
34. Elwin K, Fairclough HV, Hadfield SJ, Chalmers RM. Giardia duodenalis typing from stools: A comparison of three approaches to extracting DNA, and validation of a probe-based real-time PCR typing assay. J Med Microbiol. (2013) 63:38–44. doi: 10.1099/jmm.0.066050-0
35. Elwin K, Robinson G, Hadfield SJ, Fairclough HV, Iturriza-Gómara M, Chalmers RM, et al. Comparison of two approaches to extracting Cryptosporidium DNA from human stools as measured by a real-time PCR assay. J Microbiol Methods. (2012) 89:38–40. doi: 10.1016/j.mimet.2012.02.006
36. Yanta CA, Bessonov K, Robinson G, Troell K, Guy RA. CryptoGenotyper: a new bioinformatics tool for rapid Cryptosporidium identification. Food Waterborne Parasitol. (2021) 23:e00115. doi: 10.1016/j.fawpar.2021.e00115
37. Seabolt MH, Konstantinidis KT, Roellig DM. Hidden diversity within common protozoan parasites as revealed by a novel genomotyping scheme. Appl Environ Microbiol. (2021) 87:e02275–20. doi: 10.1128/AEM.02275-20
38. Utaaker KS, Chaudhary S, Kifleyohannes T, Robertson LJ. Global Goat! Is the expanding goat population an important reservoir of Cryptosporidium? Front Vet Sci. (2021) 8. doi: 10.3389/fvets.2021.648500
39. Vermeulen LC, Benders J, Medema G, Hofstra N. Global Cryptosporidium loads from livestock manure. Environ Sci Technol. (2017) 51:8663–71. doi: 10.1021/acs.est.7b00452
40. Utaaker KS, Skjerve E, Robertson LJ. Keeping it cool: Survival of Giardia cysts and Cryptosporidium oocysts on lettuce leaves. Int J Food Microbiol. (2017) 255:51–7. doi: 10.1016/j.ijfoodmicro.2017.05.009
41. Chang'a JS, Robertson LJ, Mtambo MMA, Mdegela RH, Løken T, Reksen O. Unexpected results from large-scale cryptosporidiosis screening study in calves in Tanzania. Ann Trop Med Parasitol. (2011) 105:513–9. doi: 10.1179/2047773211Y.0000000007
42. Kifleyohannes T, Robertson LJ. Preliminary insights regarding water as a transmission vehicle for Cryptosporidium and Giardia in Tigray, Ethiopia. Food Waterborne Parasitol. (2020) 19. doi: 10.1016/j.fawpar.2020.e00073
43. Maikai BV, Umoh JU, Kwaga JKP, Lawal IA, Maikai VA, Cama V, et al. Molecular characterization of Cryptosporidium spp. in native breeds of cattle in Kaduna State. Nigeria Vet Parasitol. (2011) 178:241–5. doi: 10.1016/j.vetpar.2010.12.048
44. Ralston BJ, McAllister TA, Olson ME. Prevalence and infection pattern of naturally acquired giardiasis and cryptosporidiosis in range beef calves and their dams. Vet Parasitol. (2003) 114:113–22. doi: 10.1016/S0304-4017(03)00134-1
45. Wang H, Qi M, Zhang K, Li J, Huang J, Ning C, et al. Prevalence and genotyping of Giardia duodenalis isolated from sheep in Henan Province, central China. Infect Genet Evol. (2016) 39:330–5. doi: 10.1016/j.meegid.2016.02.006
46. Abdullah DA, Ola-Fadunsin SD, Ruviniyia K, Gimba FI, Chandrawathani P, Lim YAL, et al. Molecular detection and epidemiological risk factors associated with Cryptosporidium infection among cattle in Peninsular Malaysia. Food Waterborne Parasitol. (2019) 14:e00035. doi: 10.1016/j.fawpar.2019.e00035
47. Li N, Xiao L, Alderisio K, Elwin K, Cebelinski E, Chalmers R, et al. Subtyping Cryptosporidium ubiquitum, a zoonotic pathogen emerging in humans. Emerg Infect Dis. (2014) 20:217–24. doi: 10.3201/eid2002.121797
48. Zahedi A, Paparini A, Jian F, Robertson I, Ryan U. Public health significance of zoonotic Cryptosporidium species in wildlife: critical insights into better drinking water management. Int J Parasitol Parasites Wildl. (2016) 5:88–109. doi: 10.1016/j.ijppaw.2015.12.001
49. Helmy YA, Krücken J, Nöckler K, von Samson-Himmelstjerna G, Zessin KH. Molecular epidemiology of Cryptosporidium in livestock animals and humans in the Ismailia province of Egypt. Vet Parasitol. (2013) 193:15–24. doi: 10.1016/j.vetpar.2012.12.015
50. Molloy SF, Tanner CJ, Kirwan P, Asaolu SO, Smith H V, Nichols RAB, et al. Sporadic Cryptosporidium infection in Nigerian children: Risk factors with species identification. Epidemiol Infect. (2011) 139:946–54. doi: 10.1017/S0950268810001998
51. Ye J, Xiao L, Wang Y, Wang L, Amer S, Roellig DM, et al. Periparturient transmission of cryptosporidium xiaoi from ewes to lambs. Vet Parasitol. (2013) 197:627–33. doi: 10.1016/j.vetpar.2013.07.021
52. Majeed QAH, El-Azazy OME, Abdou NEMI, Al-Aal ZA, El-Kabbany AI, Tahrani LMA, et al. Epidemiological observations on cryptosporidiosis and molecular characterization of Cryptosporidium spp. in sheep and goats in Kuwait. Parasitol Res. (2018) 117:1631–6. doi: 10.1007/s00436-018-5847-1
Keywords: Cryptosporidium, Ethiopia, Giardia, livestock, risk factor
Citation: Kifleyohannes T, Nødtvedt A, Debenham JJ, Terefe G and Robertson LJ (2022) Cryptosporidium and Giardia in Livestock in Tigray, Northern Ethiopia and Associated Risk Factors for Infection: A Cross-Sectional Study. Front. Vet. Sci. 8:825940. doi: 10.3389/fvets.2021.825940
Received: 30 November 2021; Accepted: 21 December 2021;
Published: 14 January 2022.
Edited by:
Alessia Libera Gazzonis, University of Milan, ItalyReviewed by:
Arwid Daugschies, Leipzig University, GermanyJan Slapeta, The University of Sydney, Australia
Copyright © 2022 Kifleyohannes, Nødtvedt, Debenham, Terefe and Robertson. This is an open-access article distributed under the terms of the Creative Commons Attribution License (CC BY). The use, distribution or reproduction in other forums is permitted, provided the original author(s) and the copyright owner(s) are credited and that the original publication in this journal is cited, in accordance with accepted academic practice. No use, distribution or reproduction is permitted which does not comply with these terms.
*Correspondence: Tsegabirhan Kifleyohannes, dHNlZ2FiaXJoYW4ua3lvaGFubmVzLnRlc2FtYUBubWJ1Lm5v; dHNlZ2FiaXJoYW4xQGdtYWlsLmNvbQ==