- 1Section for Animal Welfare and Disease Control, Department of Veterinary and Animal Sciences, Copenhagen University, Copenhagen, Denmark
- 2Department of Parasitology and Parasitic Diseases, University of Agricultural Sciences and Veterinary Medicine of Cluj-Napoca, Cluj-Napoca, Romania
- 3Department of Virus and Microbiological Special Diagnostics, Statens Serum Institut, Copenhagen, Denmark
- 4Department of Biodiversity Conservation and Sustainable Use of Natural Resources, Danube Delta National Institute for Research and Development, Tulcea, Romania
Introduction: Mosquitoes either biologically or mechanically transmit various vector-borne pathogens affecting pigs. Mosquito species display a wide variety of host preference, as well as host attraction and behaviours. Mosquito species attraction rates to- and feeding rates on pigs or other potential hosts, as well as the seasonal abundance of the mosquito species affects their pathogen transmission potential.
Methods: We caught mosquitoes in experimental cages containing pigs situated in Romanian backyard farms. The host species of blood meals were identified with PCR and sequencing.
Results: High feeding preferences for pigs were observed in Aedes vexans (90%), Anopheles maculipennis (80%) and Culiseta annulata (72.7%). However, due to a high abundance in the traps, Culex pipiens/torrentium were responsible for 37.9% of all mosquito bites on pigs in the Romanian backyards, despite low feeding rates on pigs in the cages (18.6%). We also found that other predominantly ornithophilic mosquito species, as well as mosquitoes that are already carrying a blood meal from a different (mammalian) host, were attracted to backyard pigs or their enclosure.
Discussion: These results indicate that viraemic blood carrying, for instance, African swine fever virus, West-Nile virus or Japanese encephalitis virus could be introduced to these backyard pig farms and therefore cause an infection, either through subsequent feeding, via ingestion by the pig or by environmental contamination.
1. Introduction
Flying hematophagous insects that are known to feed on wild boar and domestic pigs have been described as vectors of a wide variety of pathogens (1–4), with some of these affecting pigs (Suidae) (5). In terms of vector-borne transmission of pathogens, two distinct mechanisms are described, namely biological- and mechanical transmission (6). With biological transmission, a pathogen is able to replicate within the body of a vector before it is delivered to a new host, whereas with mechanical transmission, the pathogen cannot replicate and is eventually digested or shed, thus requiring transmission to a new host while still infectious (6). An example of pathogens transmitted by biological vector transmission is Japanese encephalitis virus [mosquitoes (7)], while examples of pathogens transmitted by mechanical vector transmission are porcine reproductive and respiratory syndrome (8), Ross River Virus [mosquitoes (9)], Mycoplasma suis [mosquitoes and Stomoxys calcitrans (10)], and classical swine fever [mosquitoes (11), S. calcitrans (12), and tabanids (13)].
Mosquitoes can act as vectors between vertebrate species for pathogens of increasing societal concern. Mosquitoes infected with West-Nile virus (WNV) or Japanese encephalitis virus (JEV) after feeding on an infected bird can act as bridge-vectors to pigs (7, 14, 15), and pigs can then act as important amplification hosts for the virus (5). As different mosquito species can show varying host preferences (16), it is necessary to determine the host-preferences of each mosquito species to evaluate its transmission potential for various pathogens.
Hematophagous insects are potential mechanical vectors of African swine fever virus (ASFV) (17), and in the Baltic countries a seasonal pattern is observed in outbreaks in domestic pigs coinciding with the summer peak abundance pattern observed in many hematophagous insect species (18, 19). Various studies suggest that mechanical transmission by vectors can occur in an experimental setting (20), for instance through ingestion (21), environmental contamination (22) or subsequent blood feeding (23), and may occur in a natural setting (24, 25). Insect species frequently attracted to, and feeding on, pigs are particularly interesting when exploring the risk of introducing pathogens to domestic pig farms by contaminated insects. Other studies have indicated that ASFV contaminated trucks (26) or professional farm visitors (27) could be a source of ASFV introduction to commercial pig farms, although the exact transmission mechanism behind these two risk factors is not yet fully understood. From this, we can hypothesise that the introduction of viraemic blood into a pig farm through hematophagous insects, if carrying a sufficient viral load, could potentially cause an outbreak. It is therefore important to identify which hematophagous insect species would potentially feed on an ASFV infected pig host outside of a farm, before they could be attracted to domestic pigs and thus introduce virus into a pig farm.
Studies show that throughout the vector season in Europe (in Sweden, Italy and the Netherlands), Culex spp. are significantly more abundant than any other mosquito genus, particularly Culex pipiens, while Aedes spp. also takes up a significant proportion of the total number of mosquitoes throughout the year (28). Differences in overall abundance are important as, while host preferences can vary between species (29), it is not necessarily the species with the strongest preference towards a certain host that is the most relevant in terms of vector potential of a certain pathogen (14, 30).
Spread of pathogens by hematophagous insects could be influenced by differences in seasonal activity patterns, species abundance, locality, host-diversity or host-preference, insect species size, digestion as well as reproductive behaviour and strategies. We designed an experiment in Romania to quantify which species of mosquitoes are attracted to Romanian backyard pigs, which species take blood meals from these, and whether these observed feeding behaviours vary throughout the vector season. The findings from this experiment could provide more evidence for the introduction of pathogens in blood meals by flying vectors.
2. Methodology
We selected two backyard pig farm locations in Tulcea County (Somova and Sӑlcioara, Southeast Romania, GPS coordinates: 45°11′41“N 28°38′52“E & 44°47′57“N 28°53′49“E) and another two in Satu Mare County (Ambud and Odoreu, Northwest Romania, GPS coordinates: 47°45′59“N 22°56′22“E & 47°47′54“N 22°58′59“E) (Figure 1). We selected the specific localities based on availability and suitability for our experimental purposes, as well as there being ASFV outbreaks in these regions.
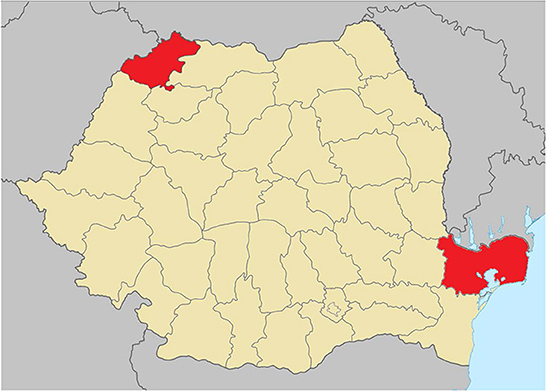
Figure 1. Map of Romania with Satu Mare County (locations Ambud and Odoreu) highlighted in red in the Northwest and Tulcea County (locations Somova and Salcioara) highlighted in red in the Southeast.
2.1. Cages and pigs
Domestic pigs were kept outside in modified dog puppy cages (~120 cm height × 160 cm length × 160 cm depth) fitted with fine stainless-steel mesh (mesh size: 0.6 mm) sheets on the walls and fine mesh on the roof. For this, we obtained an animal experiment ethical permit, registration number: 216, issued 12-06-2020 by the Bioethics Commission of USAMV Cluj-Napoca, Romania. We conducted animal care and maintenance in accordance with EU legislation on animal experimentation (EU Directive 2010/63/EU). We applied an entry-trap design, which allowed flying insects to fly into the cages through a ~5 cm cage-wide slit on two sides of the cages (Figure 2). In each of the 4 locations, we kept 2 pigs weighing between 7.5 and 10 kg each at the onset of their respective sampling period.
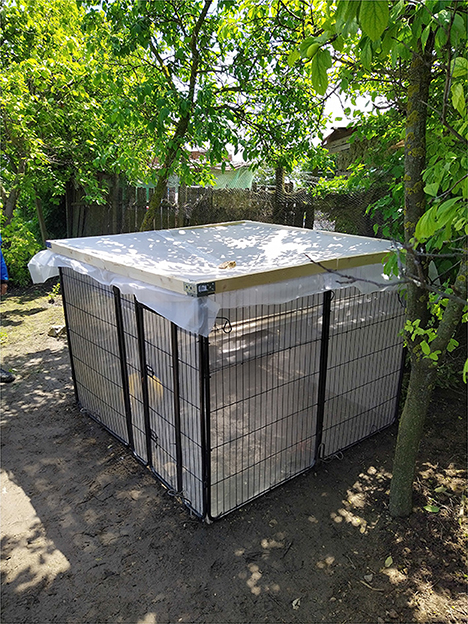
Figure 2. One of the pig cages used in the experiment (pigs not yet included). The entry trap slit can be seen on the front right panel. Collectors entered the cage through a small door, as can be seen on the front left panel, by carefully opening it and closing it immediately upon entry, so no insects would fly out.
2.2. BG-Pro traps
In each of the 4 locations, we also set up a BG-Pro mosquito trap (BioGents AG). We baited each of these traps with CO2 (0.5 kg of CO2 per 24 h) and BG-Lure mosquito attractants (which emit lactic- and caproic acid as well as ammonia). We placed the traps within the vicinity of the cages to catch mosquitoes continuously throughout the season, and we collected the mosquitoes once a week. These 7-day catches allowed us to quantify abundance of mosquitoes as well as correlate it to the proportion of mosquitoes with pig blood in mosquitoes collected from the pig cages.
2.3. Sampling periods
We carried out sampling during June to October 2021. For the cages, we aimed at 3 sampling periods of 4 weeks each, whereas the BG-Pro mosquito traps ran continuously throughout the study. For each sampling period, we replaced the pigs with new pigs, leading to 24 pigs in total during the study. The first collection period started on the 14th of June 2021 and ended on the 7th of July. In Satu Mare, the start of the second period was delayed due to logistical issues and the collection was extended beyond the original collection schedule for this period from the 16th of August until the 8th of September. In Tulcea, the second collection period started on the 1st of August and was also extended until the 8th of September to align with the collections in Satu Mare. The third collection period in both regions started on the 20th of September and ended on the 13th of October 2021.
2.4. Sampling procedure
We cleaned the cages once per week, after which we placed the mesh roofs on top of the cages, which indicated the start of that week's collection. We entered the cages 24 h later. Using aspirators, we collected all hematophagous insects present inside the cages and stored them at −20°C. Another 24 h later, we would repeat this process, after which we removed the roofs of the cages for the remainder of the week. For each of the four locations, we therefore had two 24 h consecutive collections of hematophagous insects from the cages per week for a total of 12 weeks.
2.5. Species identification
In the high containment laboratory (OIE3/4, BSL2) at Statens Serum Institut (Denmark), we identified each sample from the cages to species level using the Moskeytool identification tool (https://www.medilabsecure.com/moskeytool.html). We then visually screened each mosquito for the presence of a blood meal according to the Sella score (1–7) where Sella score 1 and 7 indicate no blood in the abdomen, and Sella score 2–6 indicates varying amounts of blood present (31).
Due to large numbers of mosquitoes collected in the BG-Pro traps, we subsampled collections when necessary and identified the mosquitoes only to genus level. We subsampled collections using a divider in a Petri dish until a single subsample contained <350 mosquitoes. We then extrapolated the results from the selected subsample to the original sample. We did not screen these mosquitoes any further or analyse them for the presence of blood meals.
2.6. Lab procedure
After species identification, we placed each mosquito with blood in the abdomen (Sella score between 2 and 6) individually in a 1.5 ml Eppendorf tube and added 1 ml MagNA Pure Lysis/Binding Buffer (Roche). We homogenised the samples using two 3 mm stainless steel beads (Dejay Distribution Ltd.) in a TissueLyser II (Qiagen) for 3 min at 25 Hz, after which we centrifuged the sample homogenates for 2 min at 10.000 RCF to collect supernatants for nucleic acid extraction. We purified the nucleic acids from the homogenised sample supernatants as previously described by Olesen et al. (32) and analysed for the presence of a porcine blood meal (i.e. MT-CYTB from Suidae) applying a TaqMan assay (33) using the CFX Opus Real-Time PCR System (BioRad). We determined a positive result (Suid blood present) in this qPCR by identification of the threshold cycle value (Cq) at which FAM dye emission appeared above background within 35 cycles.
Samples testing negative for porcine blood were tested using the TaqMan assay (No Cq value or Cq value above 35) for the presence of a mammalian blood meal (i.e. MT-CYTB of mammalian origin) using a Resolight (Roche) approach with primer sequences obtained from Andrejevic et al. (34), with minor modifications. The slightly modified primer sequences were 5′ GACGGCCAGTGAAACAGGATCCAACAACCC 3′ (forward) and 5′ GCTATGACCGGTGTAGTTGTCTGGGTCTCC 3′ (reverse). We performed the amplification using the CFX Opus Real-Time PCR System (Bio-Rad). We determined a positive result (mammalian blood present) in this qPCR by identification of the Cq value at which SYBR dye emission appeared above background within 30 cycles. We chose this threshold of 30 based on prior validation using templates of different mammalian, avian and invertebrate origin. During this validation, samples with Cq values above 30 were of avian and invertebrate origin.
We selected samples in which we detected a blood meal of mammalian origin, using the mammalian MT-CYB assay, for Sanger sequencing. For sequencing, we purified PCR products using the GeneJET PCR Purification Kit (Thermo Scientific) according to the manufacturer's instructions. We performed cycle sequencing of the PCR products using the Veriti™ 96-Well Thermal Cycler (Applied Biosystems). We performed the sequencing of the reverse and forward strand using 10 μM of the primers and the BigDye Terminator v. 1.1. Cycle Sequencing Kit (Applied BioSystems). For capillary electrophoresis, we purified the sequencing products using the SigmaSpin Post-Reaction columns (Sigma-Aldrich). We carried out capillary electrophoresis out using an ABI3500 Genetic Analyzer (Applied BioSystems). Ultimately, we analysed the results using Sequence Scanner Software v1.0 (Applied BioSystems) and the blood meal source identified using BLAST (https://blast.ncbi.nlm.nih.gov/Blast.cgi).
3. Results
In total, we identified 356 mosquitoes and 1 tabanid from the cages in the 4 locations. From the Satu Mare region, this includes 204 mosquitoes from Ambud and 96 mosquitoes from Odoreu, while from the Tulcea region this includes 38 mosquitoes and 1 tabanid samples from Sălcioara and 18 mosquito samples from Somova. Unfortunately, some of the samples from Tulcea turned unidentifiable upon arrival to the high containment laboratory, as unforeseen leakage of a number of sample containers in the same shipment necessitated use of decontamination (disinfection) upon arrival to the laboratory. This caused disinfectant to seep into some of the containers. This caused the mosquitoes inside 12 out of 20 (60%) 24 h collections from Sălcioara and 15 out of 20 (75%) 24 h collections from Somova to become unidentifiable, while it was still possible to count the numbers of mosquitoes in most collections excluding 6 collections from Somova, where we estimated the numbers visually.
From the Tulcea region (Somova and Sălcioara), and in particular Somova, we caught larger numbers of mosquitoes in the cages throughout the experiment, compared to the Satu Mare region (Ambud and Odoreu) (Figure 3). We can also see that mosquito activity likely already started prior to the start of the experiment due to the high numbers already observed in week 24. Activity gradually declined, particularly in the Tulcea region, until there were only more or less sporadic catches left after week 36.
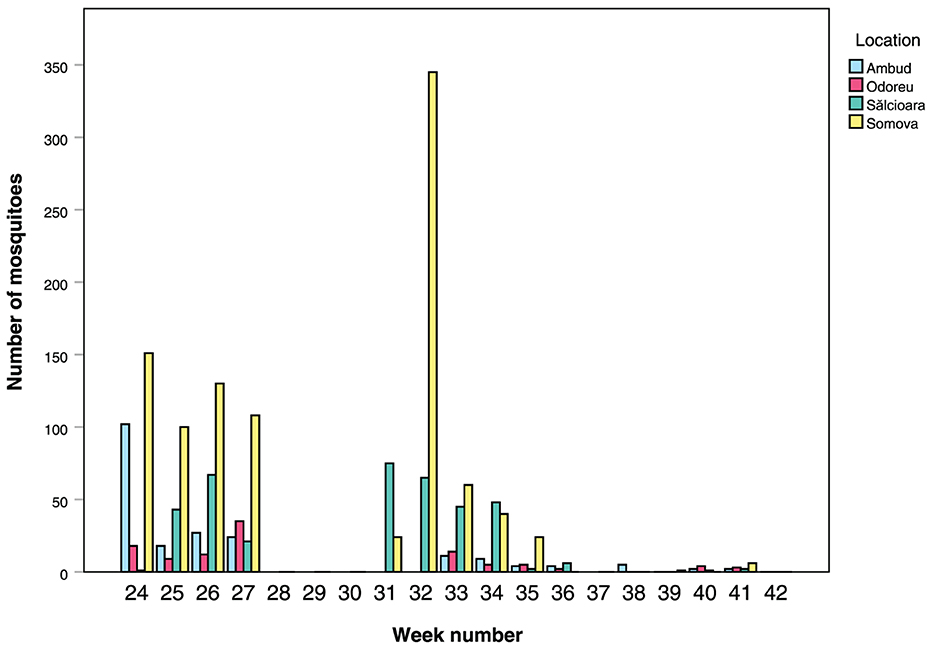
Figure 3. Number of mosquitoes caught inside the pig cages per week for all four locations. Note that there were no pigs inside the cages, and thus no collections, in week 28, 29, 30 (+ 31, 32 for Satu Mare's Ambud and Odoreu locations) and week 37.
3.1. Mosquito species feeding on pigs
Of the 356 mosquito (and 1 tabanid) samples that we successfully identified, a total of 130 showed visual traces of blood meals (Table 1). Of these, 116 (89.2%) tested positive for porcine blood (Cq values from 22.5 to 34.8 in the Suid assay). Upon testing for the presence of mammalian blood in the remaining 14 mosquito samples, one Aedes caspius caught in Sălcioara tested positive for bovine blood (Bos taurus) (Cq-value of 29 in the mammalian assay,). The remaining 13 samples tested negative for any mammalian blood in the analysis, despite the visual trace of blood. The one horsefly tested negative for any blood.
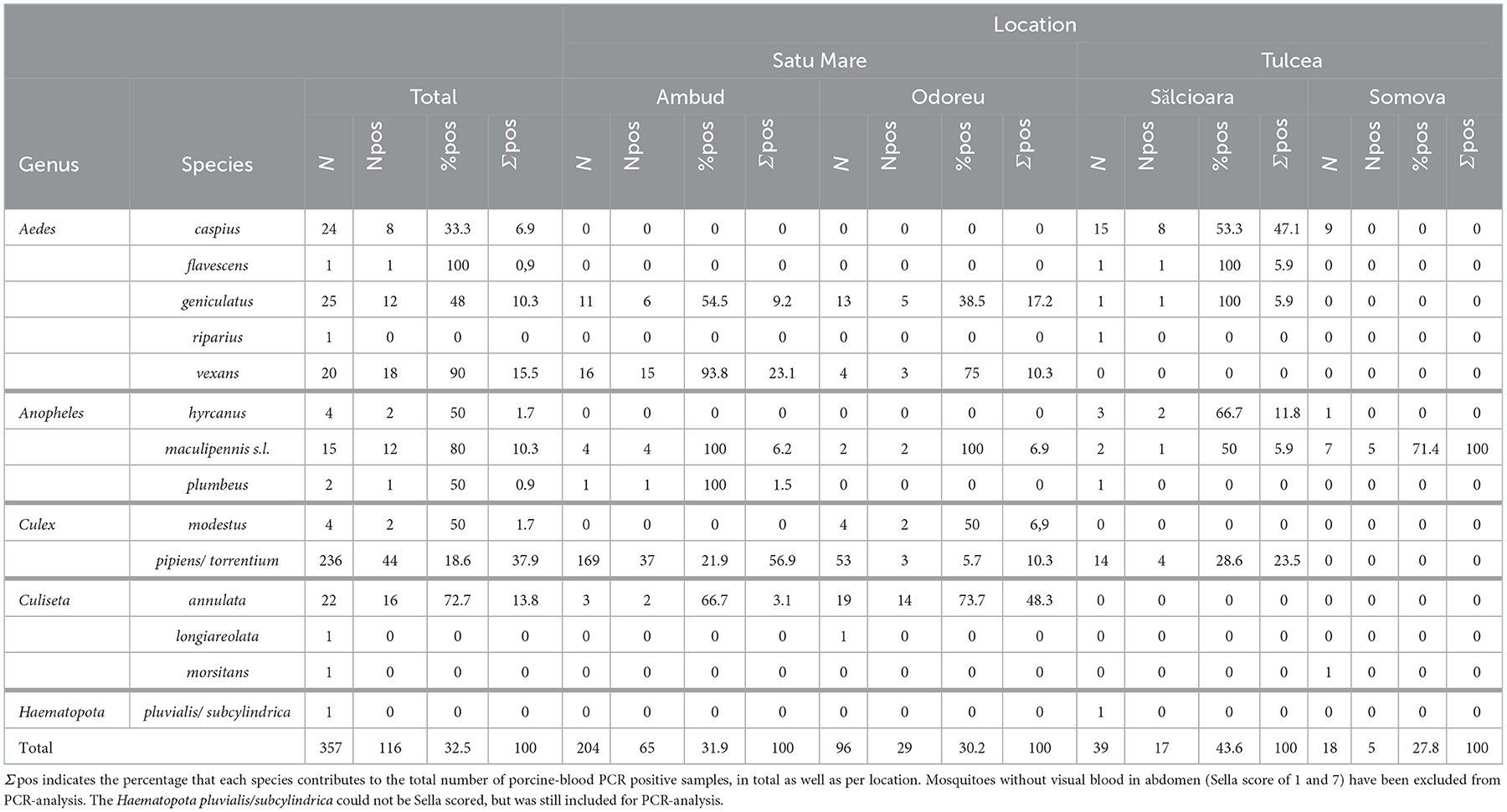
Table 1. Overview of the number of mosquitoes and horseflies (N) caught in the pig cages of each species in total and per location, as well as the number (Npos) and percentage (%pos) of porcine-blood PCR positive samples per species.
While only 18.6% of the collected Culex pipiens/torrentium carried a porcine blood meal (44 out 236), this species still constituted the largest number of mosquitoes carrying pig blood due to their high abundance (44 positive Culex pipiens/torrentium out of 116 positive mosquitoes in total, or 37.9%) (Table 1). Among the other species of which we caught at least five specimen, Aedes vexans (18 out of 20, i.e. 90 or 15.5% of all porcine blood meals), Anopheles maculipennis (12 out of 15, i.e. 80 or 10.3% of all porcine blood meals) and Culiseta annulata (16 out of 22, i.e. 72.7 or 13.8% of all porcine blood meals) showed the highest proportions feeding on domestic pig. These four species combined comprised 77.5% of all porcine blood meals. Other (non-sporadic, i.e. >5) species were Aedes geniculatus (12 out of 25, i.e. 48 or 10.3% of all porcine blood meals), and Aedes caspius (8 out of 24, i.e. 33.3 or 6.9% of all porcine blood meals). Note that Aedes caspius was only caught in cages from the Tulcea region.
3.2. Seasonality vs. proportion of mosquitoes with pig blood
We plotted the overall mosquito seasonal abundance data from our subsampled BG-Pro trap collections for each genera together with the number of mosquitoes with pig blood in the cages (Figure 4). Culex spp. was significantly more abundant than the other genera. Collections tended to be more numerous in Odoreu compared to Ambud for all genera. Overall, vector seasonal abundance increased the first 2 weeks from the onset of our BG-Pro trap collections and decreased towards the end of the collection period to almost zero. While peak activity in Ambud occurred in week 32 and 33 for all genera, both Aedes spp. and Culex spp. activity peaked in week 27 in Odoreu, while Culiseta spp. peak activity was later in week 31.
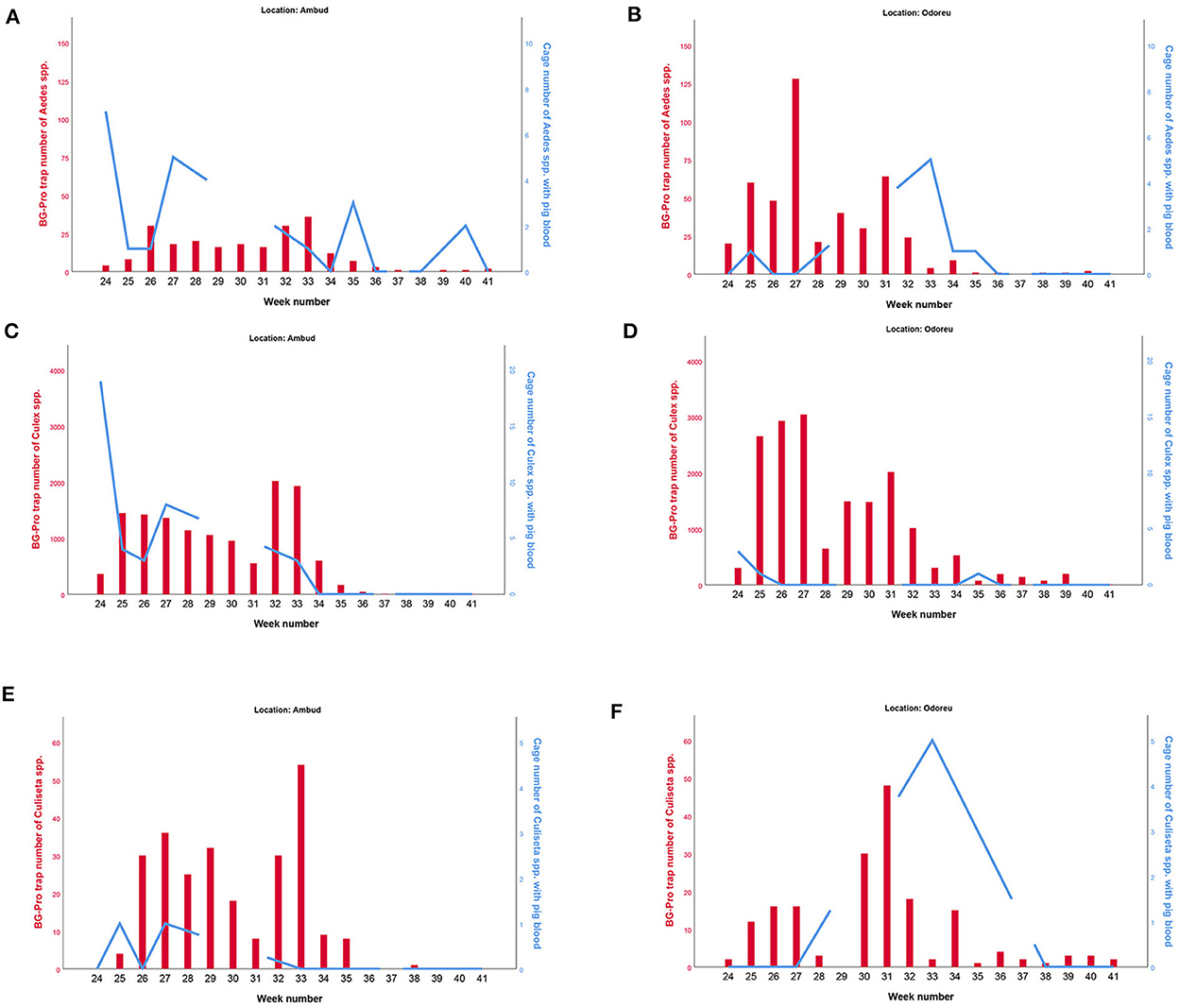
Figure 4. Seasonal abundance of the different genera of mosquitoes caught in our BG traps (red bars and the left Y-axis), as well as the number of mosquitoes with pig blood of the specific mosquito genera in our cages (blue lines and the right Y-axis). Aedes spp. is shown in (A,B), Culex spp. is shown in (C,D), Culiseta spp. is shown in (E,F). Ambud is shown in the left (A,C,E) and Odoreu is shown in the right (B,D,F), both locations are situated in northwestern Romania. Note that the proportion of mosquitoes with pig blood are based on the sum of all the individual species caught for each genera, and only porcine-blood PCR-positive cases are included. Left and right Y-axes scales are not identical, and axes scales between the different genera are not identical. Anopheles are not included as we had too few observations to make any meaningful plots.
For all four mosquito genera and for both Ambud and Odoreu, we tested for a correlation between the seasonal abundance (i.e. BG-Pro trap results) and the proportion of blood fed mosquitoes of that genus in our cages using Spearman's correlation test. None of these tests found a significant correlation between seasonal abundance and the proportion of mosquitoes having fed on pigs.
4. Discussion
Of the 356 successfully identified mosquitoes caught in the cages, 116 carried a porcine blood meal, while one Aedes caspius in Sălcioara carried a bovine blood meal (Bos taurus) and the remaining 239 did not carry any identifiable blood meals. The bovine blood meal finding indicates that blood from different hosts is occasionally introduced to backyard pig farms and that blood fed mosquitoes are still attracted to backyard pigs or their enclosures. Given this finding, we argue that a mosquito carrying an ASFV blood meal, obtained from a nearby infected domestic pig or wild boar, could potentially introduce ASFV to a farm. To cause an outbreak, virus transmission from the mosquito to domestic pigs is needed, for example by the mosquito taking a consecutive blood meal from the backyard pig, as has been shown possible in previous studies using S. calcitrans (23). Additional modes of transmission could for example be through the pig ingesting the mosquito, as has been shown sufficient for S. calcitrans (21), or environmental contamination of the pig enclosure. However, there are significant differences in the volume of a blood meal taken between potential vectors. S. calcitrans is known to take blood meals between 11.2 and 15.1 μl (35), while horseflies can take blood meals ranging from 20 μl (Chrysops spp.) to almost 700 μl (Tabanus attratus) (36), as they can consume more than their own weight in blood (37). Mosquito blood meals are significantly smaller, ranging, for example, between 1.3 μl and 5.4 μl in Anopheles stephensi (38), or 1.86–3.74 μl in Aedes aegypti (39). Previous studies have identified close proximity to outbreaks in domestic farms to be a risk factor for ASF occurrence in Romanian farms (27). Our findings support that mosquitoes do bring exogenous blood meals in close contact with backyard pigs.
Some mosquitoes that we caught inside the cages, are species known to be predominantly ornithophilic (i.e. Culiseta longiareolata and Culiseta morsitans) (Appendix I). These mosquitoes did not show any signs of blood meals. Conversely, some mosquitoes that we caught inside the cages are species known to be primarily ornithophilic (i.e. Culex pipiens/torrentium and Culiseta annulata) (Appendix I). Some of these mosquitoes from the pig cages did carry visual blood meals but tested negative for both porcine and mammalian blood. Catching these mosquitoes in the cages indicates that the predominantly ornithophilic mosquitoes are still attracted to the pigs or their enclosures, despite not feeding on the pigs. It also shows that the occasional ornithophilic species, despite already carrying a (non-mammalian) blood meal, are attracted to the pigs or their enclosures. Culex pipiens could therefore have taken a WNV or JEV viraemic blood meal before coming into contact with domestic pigs. The finding of these mosquito species next to pigs suggests that these mosquitoes may introduce JEV to pig stables without being biological vectors, which we argue could potentially still transmit the virus either by being ingested by a pig or through environmental contamination.
Despite having one of the lowest proportions of porcine-blood meals in the cages, the predominantly ornithophilic C. pipiens/torrentium still accounted for the highest number of the identified porcine blood meals (44 out of 116). This is due to their higher abundance compared to the other species caught (236 out of 356). Kilpatrick et al. (14) coined this phenomenon the Bridge Vector Paradigm. This paradigm describes the phenomenon where a potential vector is deemed unimportant for a particular pathogen from a qualitative perspective, conversely becomes a key vector species when studied in a quantitative manner. Their findings show that Culex pipiens and Culex restuans, two species previously considered unimportant in humans infections due to their predominantly ornithophilic feeding behaviour, are actually responsible for up to 80% of WNV infections in humans in the northeast United States due to their abundance compensating for the proportional feeding preference for mammalian blood. We argue that, assuming random selection of host species between individual C. pipiens/torrentium mosquitoes, the vast majority of C. pipiens/torrentium that take a second or third blood meals will at some point likely have fed on bird blood, which in turn could make them important bridge vectors for WNV and JEV. However, the abundance of C. pipiens/torrentium could be exacerbated due to the (semi-) urban settings we used for our collection, as C. pipiens is known to prefer (semi-) urban habitats over rural/wild habitats (40).
The use of C. pipiens/torrentium in this study, which is a combination of the C. pipiens complex (i.e. C. pipiens pipiens and C. pipiens molestus) and C. torrentium, was due to the females of these species/biotypes being very difficult to reliably distinguish (16). This however limits the conclusions that can be drawn from the study, as C. pipiens pipiens and C. pipiens molestus exhibit different feeding behaviours, with C. pipiens pipiens being primarily ornithophilic and C. pipiens molestus feeding on both birds and mammals (41), with humans representing 20% of blood meal hosts of C. pipiens molestus in Romania (42). Because of this difference, C. pipiens pipiens are believed to play only a minor role in the spread of arboviruses in Europe (16), although C. pipiens (in combination with C. restuans) are reported to account for over 80% of WNV infections in the north-eastern United States (14). As for C. pipiens and C. torrentium, C. pipiens is reported to be the dominant species in southern Europe, with C. torrentium rarely being reported. In northern Europe, C. torrentium is the dominant species while in central Europe similar proportions of C. pipiens and C. torrentium are observed (41). Nicolescu reported that, in Romania, C. pipiens s.l. is more abundant in urban areas compared to C. pipiens molestus, while in rural areas, they are abundant in animal shelters along with Anopheles spp. (43). Tiron et al. (42) however reported that, in southeastern Romania, C. pipiens pipiens has a preference for “green areas,” while C. pipiens molestus prefers human settings and animal farmland. Given the location of our study sites (i.e. animal shelters in rural southeastern Europe) and the described differences in feeding behaviour, the blood fed C. pipiens/torrentium in our study (44 out of 236 contained pig blood) are likely to C. pipiens molestus, although we cannot state this for certain. C. pipiens is considered the main vector of WNV in southern Romania, while Anopheles spp. could also play a role in rural environments (43). Aedes vexans, the second-most frequent feeder on pig blood caught in the cages (18 out of 20 contained pig blood), is a predominantly mammalophilic species most abundant around floodplains or lakes (16, 44), that can take multiple blood meals (16). Culiseta annulata, the third-most frequent feeder on pig blood caught in the cages, also displays a strong preference for mammals (16) and frequently enter animal enclosures or houses to feed on humans or domestic animals during the summer months (16).
While the vast majority of mosquitoes with visual signs of a blood meal also tested positive for porcine blood (89.1%), 14 samples (10.9%) did not. Besides one sample with Bos taurus blood, we were unable to state for certain that the remaining 13 samples did not carry blood of mammalian origin. Most likely, the blood meals within the remaining 13 samples were too degraded, the amount of blood within the samples were too low, or they were of non-mammalian origin.
We did not observe any clear correlation between the proportion of mosquitoes with pig blood (i.e. pig blood fed mosquitoes in the cages) and seasonal abundance (i.e. BG-Pro trap mosquitoes) of the various mosquito genera we caught (Figure 4). Throughout the sampling period, Culex spp. were significantly more abundant in the BG-Pro traps than the other genera ( = 92.3%, max = 99.2%, min = 60%). Other studies on mosquito abundance also indicate that Culex is the most abundant mosquito genus throughout Europe, particularly Culex pipiens (28).
We observed two seasonal peaks in Culex spp. (Figure 4) in both locations (Ambud and Odoreu). Although we cannot say what the vector activity was prior to week 24, personal communication with people at both locations tells us mosquito activity was very low before the onset of our collection period. Interestingly, for both Aedes spp. and Culex spp., the cage collections with the highest number of mosquitoes with pig blood occurred in the first week of the experiment (week 24). This was several weeks before the observed seasonal peak of these two genera in the BG-Pro traps (week 32 + 33). Furthermore, personal communication also tells us that the region was sprayed with insecticide from a helicopter right after our collection in week 27, from which it appears we can see the effect particularly in Odoreu. While the most abundant species of the Culex genus, Culex pipiens, is known to go through up to 7 generations in a single year (16), the insecticide sprayed over the region by helicopter following the collection in week 27 could also potentially explain the apparent double peaks.
Since insects were collected once every 24 h, after we fitted the roof on the cages, any mosquito taking a blood meal directly after the roof placement would have up to 24 h to digest the blood meal before collection. According to the digestion times given by Detinova et al. (31), a mosquito could digest enough blood within these 24 h to reach a Sella score of up to 4 (i.e. practically half full and half empty with dark blood). Interestingly, 25 out of 28 samples with a Sella score of 5, and 7 out of 9 with a Sella score of 6 tested positive for pig blood, which indicates that these blood meals either came from other pigs in the vicinity or were only partial/interrupted blood meals. It should be noted however, that the rate of blood meal digestion in mosquitoes, being poikilotherms, varies according to the temperature in the surrounding environment (45). We initially assumed that a higher Sella score (i.e. the blood meal being in a later state of digestion) would correlate to higher Cq-values for our PCR assays. However, a scatterplot comparing Cq-values to Sella scores provided an R2 correlation coefficient of 0.0004, indicating a negligible correlation between the two variables (data not shown).
Our findings contribute to our understanding of which mosquito species are relevant when studying vector-borne pathogens that involve pigs. Culex pipiens, despite not preferentially feeding on pigs, should be the mosquito species of primary focus when looking at mosquito-borne pathogens, due to its high abundance. Other mosquito species, that do not tend to feed on pigs, could still be relevant vectors in terms of the introduction of viraemic blood from other host species, such WNV, as these ornithophilic mosquito species are still attracted to pigs or their enclosures. Future studies are needed to assess, which specific pathogens are introduced by mosquitoes to backyard pigs or their enclosures, and in what quantities. It is also important to gain more insights into the flight distance of these blood fed mosquitoes, to understand the possible range of transmission from viraemic blood-carrying vectors.
Data availability statement
The raw data supporting the conclusions of this article will be made available by the authors, without undue reservation.
Ethics statement
The animal study was reviewed and approved by Bioethics Commission of USAMV Cluj-Napoca.
Author contributions
JS, AM, RB, LK, and AB: conceptualisation. JS, AM, OB, MM, and VA: field data collection. JS, AO, and TR: sample processing. JS: data analysis and draft writing. JS, RB, AM, AO, LK, AB, TR, MM, and VA: draft review. All authors contributed to the article and approved the submitted version.
Funding
This study was supported by the University of Copenhagen.
Acknowledgments
We would like to thank Emanuel Albu and Razvan Blaga for their assistance in collecting the samples. We thank Philippe Weissenberg, Noureddine Mechouk, Gheorghe Bucur, and Mircea Coroian for their logistical support and Fie Fisker Brønnum Christiansen for her help processing the samples in the laboratory.
Conflict of interest
The authors declare that the research was conducted in the absence of any commercial or financial relationships that could be construed as a potential conflict of interest.
Publisher's note
All claims expressed in this article are solely those of the authors and do not necessarily represent those of their affiliated organizations, or those of the publisher, the editors and the reviewers. Any product that may be evaluated in this article, or claim that may be made by its manufacturer, is not guaranteed or endorsed by the publisher.
Supplementary material
The Supplementary Material for this article can be found online at: https://www.frontiersin.org/articles/10.3389/fvets.2022.1046263/full#supplementary-material
References
1. Craigo JK, Montelaro RC. Equine infectious anemia virus. Evol Viruses. (2008) 167–74. doi: 10.1016/B978-012374410-4.00395-2
2. Vloet RPM, Vogels CBF, Koenraadt CJM, Pijlman GP, Eiden M, Gonzales JL, et al. Transmission of Rift Valley fever virus from European-breed lambs to Culex pipiens mosquitoes. PLoS Negl Trop Dis. (2017) 11:e0006145. doi: 10.1371/journal.pntd.0006145
3. Fischer EA, Boender GJ, Nodelijk G, De Koeijer AA, Van Roermund HJ. The transmission potential of Rift valley fever virus among livestock in the Netherlands: a modelling study. Vet Res. (2013) 44:58. doi: 10.1186/1297-9716-44-58
4. Mellor PS, Carpenter S, White DM. Bluetongue virus in the insect host. Bluetongue. (2009) 295:320. doi: 10.1016/B978-012369368-6.50018-6
5. Zimmerman JJ, Karriker LA, Ramirez A, Schwartz KJ, Stevenson GW, Zhang J. Diseases of Swine. Hoboken, NJ: Wiley-Blackwell (2019).
6. EFSA. Vector-borne Diseases. EFSA (2022). Available online at: https://www.efsa.europa.eu/en/topics/topic/vector-borne-diseases (accessed October 9, 2022).
7. Auerswald H, Maquart PO, Chevalier V, Boyer S. Mosquito vector competence for japanese encephalitis virus. Viruses. (2021) 13:1154. doi: 10.3390/v13061154
8. Otake S, Dee SA, Rossow KD, Moon RD, Pijoan C. Mechanical transmission of porcine reproductive and respiratory syndrome virus by mosquitoes, Aedes vexans (Meigen). Can J Vet Res. (2002) 66:191–5.
9. Harley D, Sleigh A, Ritchie S. Ross river virus transmission, infection, and disease: a cross-disciplinary review. Clin Microbiol Infect. (2001) 14:909–32. doi: 10.1128/CMR.14.4.909-932.2001
10. Prullage JB, Williams RE, Gaafar SM. On the transmissibility of Eperythrozoon suis by Stomoxys calcitrans and Aedes aegypti. Vet Parasitol. (1993) 50:125–35. doi: 10.1016/0304-4017(93)90013-D
11. Stewart WC, Carbrey EA, Jenney EW. Transmission of hog cholera virus by mosquitoes. Am J Vet Res. (1975) 36:611–4.
12. Morgan NO, Miller DM. Muscidae (diptera): experimental vectors of hog cholera virus. J Medi. (1976) 12:657–60. doi: 10.1093/jmedent/12.6.657
13. Tidwell MA, Dean WD, Tidwell MA, Combs GP, Anderson DW, Cowart WO, et al. Transmission of hog cholera virus by horseflies (Tabanidae: Diptera). Am J Vet Res. (1972) 33:615–22.
14. Kilpatrick AM, Kramer LD, Campbell SR, Alleyne EO, Dobson AP, Daszak P. West nile virus risk assessment and the bridge vector paradigm. Emerg Infect Dis. (2005) 11:425–9. doi: 10.3201/eid1103.040364
15. Molaei G, Andreadis TG. Identification of avian- and mammalian-derived bloodmeals in Aedes vexans and Culiseta melanura (Diptera: Culicidae) and its implication for West Nile virus transmission in connecticut, U.S.A. J Med Entomol. (2006) 43:1088–93. doi: 10.1093/jmedent/43.5.1088
16. Becker N, Petric D, Zgomba M, Boase C, Dahl C, Madon M, et al. Mosquitoes and Their Control. 2nd edition. Heidelberg; Dordrecht; London; New York, NY: Springer (2010). doi: 10.1007/978-3-540-92874-4
17. EFSA, Miteva A, Papanikolaou A, Gogin A, Boklund A, Bøtner A, et al. Epidemiological analyses of African swine fever in the European Union (November 2018 to October 2019). EFSA J. (2020) 18:5996. doi: 10.2903/j.efsa.2020.5996
18. Resh VH, Carde RT. Encyclopedia of Insects. Vol. 7. Amsterdam: Elsevier; Academic Press. (2015) 37–72p.
19. EFSA, Desmecht D, Gerbier G, Schmidt CG, Grigaliuniene V, Helyes G, et al. Epidemiological analysis of African swine fever in the European Union (September 2019 to August 2020). EFSA J. (2021) 19:6572. doi: 10.2903/j.efsa.2021.6572
20. Olesen AS, Hansen MF, Rasmussen TB, Belsham GJ, Bødker R, Bøtner A. Survival and localization of African swine fever virus in stable flies (Stomoxys calcitrans) after feeding on viremic blood using a membrane feeder. Vet Microbiol. (2018) 222:25–9. doi: 10.1016/j.vetmic.2018.06.010
21. Olesen AS, Lohse L, Hansen MF, Boklund A, Halasa T, Belsham GJ, et al. Infection of pigs with African swine fever virus via ingestion of stable flies (Stomoxys calcitrans). Transbound Emerg Dis. (2018) 65:1152–7. doi: 10.1111/tbed.12918
22. Olesen AS, Lohse L, Boklund A, Halasa T, Belsham GJ, Rasmussen TB, et al. Short time window for transmissibility of African swine fever virus from a contaminated environment. Transbound Emerg Dis. (2018) 65:1024–32. doi: 10.1111/tbed.12837
23. Mellor PS, Kitching RP, Wilkinson PJ. Mechanical transmission of capripox virus and African swine fever virus by Stomoxys calcitrans. Res Vet Sci. (1987) 43:109–12. doi: 10.1016/S0034-5288(18)30753-7
24. Balmoş OM, Supeanu A, Tamba P, Cazan CD, Ionică AM, Ungur A, et al. Entomological survey to study the possible involvement of arthropod vectors in the transmission of African swine fever virus in Romania. EFSA Supp Public. (2021) 18:6460E. doi: 10.2903/sp.efsa.2021.EN-6460
25. Mazur-Panasiuk N, Zmudzki J, Wozniakowski G. African swine fever virus - persistence in different environmental conditions and the possibility of its indirect transmission. J Vet Res. (2019) 63:303–10. doi: 10.2478/jvetres-2019-0058
26. Mur L, Martínez-López B, Sánchez-Vizcaíno JM. Risk of African swine fever introduction into the European Union through transport-associated routes: returning trucks and waste from international ships and planes. BMC Vet Res. (2012) 8:149. doi: 10.1186/1746-6148-8-149
27. Boklund A, Dhollander S, Vasile C, Abrahantes J, Bøtner A, Gogin A, et al. Risk factors for African swine fever incursion in Romanian domestic farms during (2019). Sci Rep. (2020) 10:10215. doi: 10.1038/s41598-020-66381-3
28. Möhlmann TWR, Wennergren U, Tälle M, Favia G, Damiani C, Bracchetti L, et al. Community analysis of the abundance and diversity of mosquito species (Diptera: Culicidae) in three European countries at different latitudes. Parasit Vect. (2017) 10:510. doi: 10.1186/s13071-017-2481-1
29. Shahhosseini N, Frederick C, Racine T, Kobinger GP, Wong G. Modeling host-feeding preference and molecular systematics of mosquitoes in different ecological niches in Canada. Acta Trop. (2021) 213:105734. doi: 10.1016/j.actatropica.2020.105734
30. Boyer S, Durand B, Yean S, Brengues C, Maquart PO, Fontenille D, et al. Host-feeding preference and diel activity of mosquito vectors of the Japanese encephalitis virus in rural Cambodia. Pathogens. (2021) 10:376. doi: 10.3390/pathogens10030376
31. Detinova TS. Age-Grouping Methods in Diptera of Medical Importance: With Special Reference to Some Vectors of Malaria. Geneva: WHO (1962). doi: 10.2307/3275215
32. Olesen AS, Lohse L, Boklund A, Halasa T, Gallardo C, Pejsak Z, et al. Transmission of African swine fever virus from infected pigs by direct contact and aerosol routes. Vet Microbiol. (2017) 211:92–102. doi: 10.1016/j.vetmic.2017.10.004
33. Forth JH. Standardisierung eines nicht-invasiven Beprobungssystems zur infektionsüberwachung bei wildschweinen (Sus scrofa). Ernst Moritz Arndt Universität Greifswald. (2015)
34. Andrejevic M, Markovic MK, Bursac B, Mihajlovic M, Tanasic V, Kecmanovic M, et al. Identification of a broad spectrum of mammalian and avian species using the short fragment of the mitochondrially encoded cytochrome b gene. For Sci Med Pathol. (2019) 15:169–77. doi: 10.1007/s12024-019-00096-4
35. Schowalter T, Klowden M. Blood Meal Size of the Stable Fly, Stomoxys calcitrans, Measured by the HICN Method. Vol. 39. Mosquito News. Athens, GA: Department of Entomology, University of Georgia, (1979).
36. Mullens BA. Horse flies and deer flies (Tabanidae). Med Vet Entomol. (2019) 3:327–43. doi: 10.1016/B978-0-12-814043-7.00016-9
37. Leprince DJ, Foil LD. Relationships among body size, blood meal size, egg volume, and egg production of Tabanus fuscicostatus (Diptera: Tabanidae). J Med Entomol. (1993) 30:865–71. doi: 10.1093/jmedent/30.5.865
38. Graumans W, Heutink R, Van Gemert GJ, Van De Vegte-Bolmer M, Bousema T, Collins KA. A mosquito feeding assay to examine plasmodium transmission to mosquitoes using small blood volumes in 3D printed nano-feeders. Parasit Vect. (2020) 13:401. doi: 10.1186/s13071-020-04269-x
39. Knaus RM, Foil LD, Issel CJ, Leprince DJ. Insect blood meal studies using radiosodium 24Na and 22Na. J Am Mosq Control Assoc. (1993) 9:264–8.
40. González MA, Prosser SW, Hernández-Triana LM, Alarcón-Elbal PM, Goiri F, López S, et al. Avian feeding preferences of Culex pipiens and Culiseta spp. Along an urban-to-wild gradient in Northern Spain. Front Ecol Evol. (2020) 8:568835. doi: 10.3389/fevo.2020.568835
41. Brugman VA, Hernández-Triana LM, Medlock JM, Fooks AR, Carpenter S, Johnson N. The role of Culex pipiens L. (Diptera: Culicidae) in virus transmission in Europe. Int J Environ Res Public Health. (2018) 15:389. doi: 10.3390/ijerph15020389
42. Tiron GV, Stancu IG, DInu S, Prioteasa FL, Falcuta E, Ceianu CS, et al. Characterization and host-feeding patterns of Culex pipiens s.l. Taxa in a West Nile virus-endemic area in Southeastern Romania. Vector Borne Zoonotic Dis. (2021) 21:713–9. doi: 10.1089/vbz.2020.2739
43. Nicolescu G. A general characterisation of the mosquito fauna (Diptera: Culicidae) in the epidemic area for West Nile vims in the south of Romania. Eur Mosq Bull. (1998) 2:13–8.
44. Kurucz K, Kepner A, Bosiljka K, Hederics D, Földes F, Zana B, et al. Blood-meal analysis and avian malaria screening of mosquitoes collected from human-inhabited areas in Hungary and Serbia. J Eur Mosq Control Assoc. (2018) 36: 3–13.
Keywords: blood meal, african swine fever virus, mechanical transmission, west-nile virus, japanese encephalitis virus, insect vectors
Citation: Stelder JJ, Mihalca AD, Olesen AS, Kjær LJ, Boklund AE, Rasmussen TB, Marinov M, Alexe V, Balmoş OM and Bødker R (2023) Potential mosquito vector attraction to- and feeding preferences for pigs in Romanian backyard farms. Front. Vet. Sci. 9:1046263. doi: 10.3389/fvets.2022.1046263
Received: 16 September 2022; Accepted: 08 December 2022;
Published: 04 January 2023.
Edited by:
Shao-Lun Zhai, Guangdong Academy of Agricultural Sciences, ChinaReviewed by:
Edward D. Walker, Michigan State University, United StatesJohn Bosco Keven, University of California, Irvine, United States
Copyright © 2023 Stelder, Mihalca, Olesen, Kjær, Boklund, Rasmussen, Marinov, Alexe, Balmoş and Bødker. This is an open-access article distributed under the terms of the Creative Commons Attribution License (CC BY). The use, distribution or reproduction in other forums is permitted, provided the original author(s) and the copyright owner(s) are credited and that the original publication in this journal is cited, in accordance with accepted academic practice. No use, distribution or reproduction is permitted which does not comply with these terms.
*Correspondence: Jonno Jorn Stelder, am9ubm8uc3RlbGRlckBzdW5kLmt1LmRr