- 1The International Graduate Program of Veterinary Science and Technology, Faculty of Veterinary Science, Chulalongkorn University, Bangkok, Thailand
- 2Center of Excellence in Fish Infectious Diseases, Department of Veterinary Microbiology, Faculty of Veterinary Science, Chulalongkorn University, Bangkok, Thailand
- 3Department of Food, Agriculture and Bioresources, Aquaculture and Aquatic Resources Management Program, Asian Institute of Technology, School of Environment, Klong Luang, Thailand
- 4Department of Aquaculture, Faculty of Fisheries, Kasetsart University, Bangkok, Thailand
- 5Center of Excellence in Aquatic Animal Health Management, Faculty of Fisheries, Kasetsart University, Bangkok, Thailand
The present study describes a simultaneous infection of a novel Chlamydia-like organism (CLO) with a Myxozoa parasite, Henneguya sp. in snakeskin gourami Trichopodus pectoralis in Thailand. A new CLO is proposed “Candidatus Piscichlamydia trichopodus” (CPT) based on 16S rRNA phylogenetic analysis. Systemic intracellular CPT infection was confirmed by histological examination, in situ hybridization, PCR assay, and sequencing of 16S rRNA. This novel pathogen belongs to the order Chlamydiales but differs in certain aspects from other species. The histopathological changes associated with CPT infection were different from the typical pathological lesions of epitheliocystis caused by previously known CLO. Unlike other CLO, CPT localized in the connective tissue rather than in the epithelial cells and formed smaller clumps of intracellular bacteria that stained dark blue with hematoxylin. On the other hand, typical myxospores of the genus Henneguya with tails were observed in the gill sections. Infection with Henneguya sp. resulted in extensive destruction of the gill filaments, most likely leading to respiratory distress. Due to the frequency of co-infections and the unavailability of culture methods for CLO and Henneguya sp., it was difficult to determine which pathogens were directly responsible for the associated mortality. However, co-infections may increase the negative impact on the host and the severity of the disease. Given the commercial importance of the snakeskin gourami and its significant aquaculture potential, the findings of this study are important for further studies on disease prevention.
Introduction
Snakeskin gourami, Trichopodus pectoralis, is native to Southeast Asia and commonly found in the Mekong and Chao Phraya basins of Cambodia, Thailand, Southern Vietnam, and Laos (1). In Thailand, the snakeskin gourami is a highly economic species and has become one of the five most important freshwater species in aquaculture (2, 3). Increased incidence of parasitic and bacterial diseases is one of the major obstacles to the farming of this species (4). However, studies on the occurrence of diseases in T. pectoralis are still very scarce (5). The pathogen fauna of this species is poorly understood and may contain pathogens that have not yet been described in the literature.
Myxosporeans are diverse and widespread parasites that cause severe economic damage to fish worldwide (6–8). The genus Henneguya includes more than 200 species and is one of the most diverse myxosporean genera in the family Myxobolidae (8). Morphologically, this genus is distinguished from the other genera of the family Myxobolidae by its elongated myxospores, which consist of two shell valves, each with a caudal projection, a usually binucleate sporoplasm, and two apical polar capsules (6, 9, 10). Some species of the genus Henneguya are responsible for diseases leading to high mortality rates, but most species are thought to have little or no negative impact on fish health (6, 11). Infection from Henneguya sp. usually occurs in the gills and is characterized by the presence of cyst-like structures on the gill filaments (6, 7, 12). Infection can devastate fish populations when the parasites multiply in high densities on the gills and leads to respiratory failure, especially in juvenile fish (13, 14). Other commonly known pathogens that cause gill cysts in fish are bacteria from the order Chlamydiales. These bacteria typically cause epithelial cysts in the skin and gills called epitheliocystis (15–17). However, it is noteworthy that different Chlamydia-like organisms (CLOs) have been found to have different pathology upon infection, possibly depending on the chlamydial species, the infected host, and the affected tissue (18). To date, unavailability of culture techniques for chlamydial pathogens has been a major obstacle for in vitro studies (15, 18).
In the present study, we described for the first time a systemic pathology caused by a novel Chlamydia-like organism, Candidatus Piscichlamydia trichopodus (order Chlamydiales) and a gill parasite Henneguya sp. (Myxosporea, Myxobolidae) infecting the same fish population. Pathogen characterization was performedbased on molecular analyses with detailed histopathological observations and confirmation by in situ hybridization (ISH).
Materials and Methods
Fish and Case History
In March 2021, higher than average mortality was observed in snakeskin gourami fingerlings in two nursery ponds in Suphan Buri province, central Thailand. History entails that snakeskin gourami fingerlings (0.3 g ± 0.05) reared by traditional methods (19) were purchased from a hatchery. Male and female broodstock were naturally mated in an earthen pond. Eggs were spawned in natural bubble nests made by male gourami in the same pond. Offspring were harvested at the size of 3.0 cm (weight 0.2–0.3 g) and 150,000 fish were delivered to the two ponds mentioned above. The fish were kept in a 5 m2 net in the pond and fed daily with a commercial pellet diet containing 28% protein (Betagro, Thailand), administered at a rate of 3% of body weight.
Gross Necropsy and Histopathology
Representative 10 fingerlings were collected on day 6 after disease onset for further examination approved by the Institutional Animal Care and Use Committee of Kasetsart University (Approval ID: ACKU63-FIS-009). After euthanasia with clove oil (150 ppm/l), fresh skin mucus and gill samples were collected for microscopic examination. Bacteriological examination of brain, liver and kidney tissue samples was performed by streaking on tryptic soy agar and brain-heart infusion agar (BHIA) (Himedia, India) and incubation at 28°C for 48 h. Samples from whole body fish (n = 5) and detached gills (n = 10) were fixed in 10% neutral buffered formalin and processed routinely for histology. Paraffin-embedded gills were sectioned at 5 μm, stained with haematoxylin and eosin and examined under a light microscope. The morphology of the spore and polar capsules were used to make a tentative genus classification of myxospores in this study, as previously described by Lom and Arthur (6, 9) and Fiala et al. (20).
DNA Extraction, 16S rRNA Amplification, and Sequencing
Genomic DNA was isolated from infected gills using the Tissue Genomic DNA Mini kit (Geneaid, Taiwan) according to the manufacturer's instructions. The presence of chlamydial DNA was first examined using primers 16SIGF (5′-CGGCGTGGATGAGGCAT-3′) and 16SIGR (5′-TCAGTCCCAGTGTTGGC-3′) described in the previous study (21). All positive samples were subjected to further PCR with Chlamydiales-specific primers 16SIGF (5′-CGGCGTGGATGAGGCAT-3′) and 806R (5′-GGAC TACCAGGGTATCTAAT-3′) according to Relman (22). PCR amplification reaction and cycling conditions for these assays were as previously described by Sood et al. (23) and Draghi et al. (24). The expected amplicons of the first and second PCR methods were 300 and 766 bp, respectively. Amplified PCR products (766 bp) from each fish were isolated using NucleoSpin™ Gel PCR Clean-up Kit (Fisher Scientific, Sweden) according to the manufacturer's protocol and then submitted for sequencing service (U2Bio, Thailand).
Phylogenetic Analyses
A BLASTn query against available nucleotide sequences was deposited in the GenBank database (www.ncbi.nlm.nih.gov) to determine taxonomic identity. The closest known relatives and several sequences from related species of Chlamydia-like organisms were obtained from the NCBI database and used for phylogenetic analysis. The phylogenetic tree was constructed using the neighbor-joining method with 1,000 bootstraps after multiple alignments against the closely related Chlamydia bacteria using ClustalW in MEGA X version 10.2.4 (25). To root the tree, sequences from a Betaproteobacterium, Candidatus Branchiomonas cysticola (Accession number JQ723599.1), were used as outgroup.
In situ Hybridization
To confirm localization of CPT in the infected tissues, in situ hybridization (ISH) with a CLO-specific probe was performed on tissues from five representative diseased fish. A 766 bp probe was produced firstly by PCR using DNA extracted from infected fish as DNA template and the primers 16SIGF and 806R (22). The product was labeled with digoxigenin (DIG) using a commercial PCR DIG labeling mixture (Roche Molecular Biochemicals, Germany) according to the manufacturer's instructions. In situ hybridization was performed as previously described by Dinh-Hung et al. (26) with some modifications. Briefly, unstained 4-μm sections on HistoGrip-coated slides (Fisher Scientific, Sweden) were deparaffinized 2 times for 5 min in xylene and then rehydrated through a graded series of ethanol and distilled water. After rapid treatment with cold acetic acid for 20 s and washing in distilled water, each section was covered with prehybridization buffer [4 × SSC contain 50% (v/v) deionized formamide] for at least 10 min at 37°C. The probe was diluted in hybridization buffer [50% deionized formamide, 50% dextran sulfate, 50 × Denhardt's solution (Sigma-Aldrich, Germany), 20 × SSC, 10 mg per ml salmon sperm DNA (Invitrogen, USA)], heated to 95°C for 10 min, and then cooled on ice. The specific probe was added to the slides, then covered with coverslips and incubated overnight at 42°C in a humidity chamber. For control slides, no probe was added to the hybridization solution. After hybridization, slides were washed in a series of graded sodium citrate solutions for 5 min in 2 × SSC at room temperature (RT), 15 min in 2 × SSC (37°C), 15 min in 1 × SSC (37°C), 30 min in 0.5 × SSC (37°C), and then equilibrated for 5 min in buffer I (1 M Tris-HCl, 1.5 M NaCl, pH 7.5). The tissue sections were then blocked with blocking solution buffer II (containing 0.1% Triton X-100 and 2% normal sheep serum) at room temperature for 30 min before being covered with anti-digoxigenin, Fab fragments (Roche Molecular Biochemicals, Germany, diluted 1:500 in buffer II) for 1 h at 45°C. After washing twice for 10 min each with buffer I, sections were treated for 10 min in buffer III (100 mM Tris-HCl, 1.5 M NaCl, 50 mM MgCl2, pH 9.5). Signals were developed using the BCIP/NBT substrate, followed by counterstaining with nuclear fast red. The slides were then mounted, observed and photographed under a digital microscope.
Results
Initial Diagnosis
Mortality was recorded on the second day after the fry were introduced. Daily mortality recorded from 1 to 5 days after onset of disease (dao) were 20, 50, 150, 200, and 400 fish, respectively. The infected fish exhibited lethargy, fish gasping at the water surface, and loss of appetite followed by mortality. Disease diagnosis carried out in the field on 6 dao showed no external lesions on the body surface of the fish (Figure 1A). Wet-mount examination of the skin mucus and gills showed no external parasites, although the formation of several characteristic cysts in the gill filaments was prevalent (Figure 1B). This observation initially led us to a preliminary misdiagnosis as an “epitheliocystis” case. At 3 dao, sea salt was added daily to the pond (total 200 kg), but no reduction in mortality was observed. Subsequently, oxytetracycline (OTC) (200 mg/g active ingredient, Pharmatech, Thailand) was continuously administered to the fish via feed admixture (5 g OTC per 1 kg of feed) for 7 days. After treatment with OTC, there was a significant decrease in daily mortality of 150, 50, and 16 fish at 7, 8, and 9 dao, respectively. No mortality (0 dead fish) was observed after treatment with OTC for 4 days (10 dao).
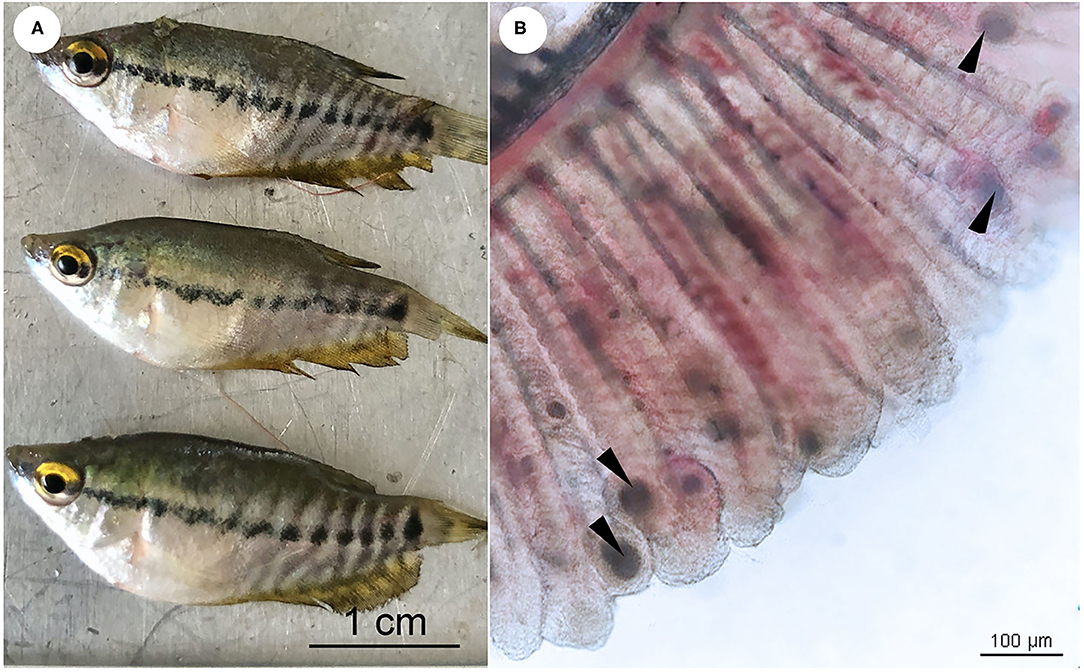
Figure 1. (A) Snakeskin gourami (Trichopodus pectoralis), no external lesions on the body surface of the fish. (B) Wet mount preparations of infected fish gill showing numerous morphological characteristics of “cysts” (arrowheads). Scale bars are shown in the pictures.
Histopathology and in situ Hybridization
Histological examination revealed colonization by intracellular bacteria in several organs, including the gill filaments (Figure 2A.1), submucosa of the intestine (Figure 2B.1), and caudal fin tissue (Figure 2C.1). Dense, roundish to oval intracellular bacteria with a great affinity for connective tissue rather than epithelial cells were observed (Figures 2A.2–C.2). Apparently, the bacteria infected the primary gill filaments rather than the secondary gill filaments. In particular, the cartilaginous junctions between primary and secondary gill filaments were apparently more susceptible to infection than others, resulting in the separation of the two components (Figure 2A.2). Similar changes were also observed at the cartilaginous junction of the caudal fin (data not shown). With respect to ISH, the DIG-labeled probe was specifically bound to intracellular bacterial foci (Figures 2A.3–C.3), whereas no binding signal was observed in tissue sections incubated with no probe (Figures 2A.4–C.4). Furthermore, no culturable bacteria were isolated from the internal organs on nutrient agar plates as well as on brain-heart infusion agar plates even after 48 h of incubation.
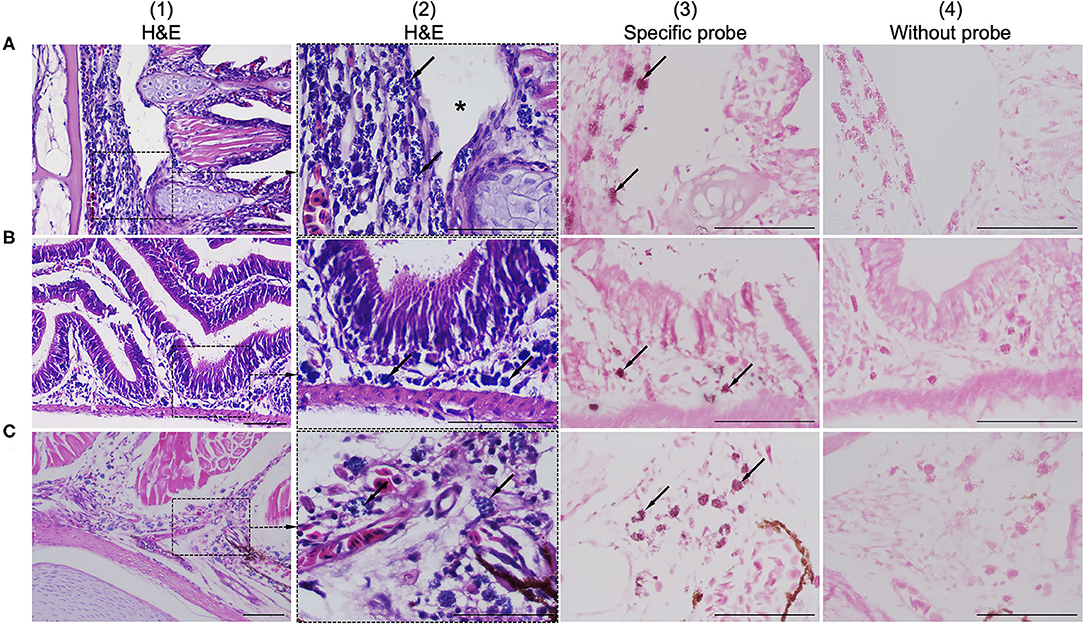
Figure 2. Comparison of consecutive gill sections of infected fish stained with H&E (A.1–C.1; A.2–C.2), ISH with a specific probe (A.3–C.3) and ISH without probe (A.4–C.4) as control. Infected fish showed the presence of a novel intracellular bacterium in the gill (A.1), intestinal submucosa (B.1), and caudal/tail fin (C.1). Higher magnification showed that the connective tissue was more susceptible to infection than others due to colonization by dense, roundish to oval, blue-stained intracellular bacteria (arrows in A.2–C.2). The bacteria were observed near the cartilaginous junctions of the primary and secondary gill filaments, resulting in disruption of the tissue junction (asterisk in A.2). ISH positive reactivity of intracellular bacterial foci is indicated by distinct dark signals (arrows in A.3–C.3). Scale bar = 50 μm.
Interestingly, histological analysis showed that the “cysts” found in the infected fish were not epitheliocystis, as tentatively diagnosed and later identified as plasmodia of a myxosporean. The presence of numerous plasmodia was observed on the gill filaments (Figure 3A). Myxospores and plasmodia demonstrated asynchronous development with young round plasmodia encased in a wall of epithelial cells of the gill filaments (Figure 3B). As the plasmodium grew, the envelope ruptured and released myxospores into the adjacent tissue (Figure 3C). The myxospores exhibited typical features of the genus Henneguya: two equal polar capsules, sporoplasm at the posterior pole of the spore, and two long, superimposed caudal processes (Figure 3D). Histological analysis of the infected gills of Henneguya showed that the plasmodia caused severe distortion of the lamellar structure and obstruction of the gills by compression of the cysts (Figures 4A–C). The plasmodia occupied the part extent of the gill lamellae and produced marked dilatation and discrete epithelial hyperplasia (Figures 4D,E). The extensive dilatation of the infected lamellae caused displacement and deformation of the adjacent lamellae. As the plasmodia grew, they compressed the adjacent tissue and caused tissue necrosis in the infected area (Figures 4E,F).
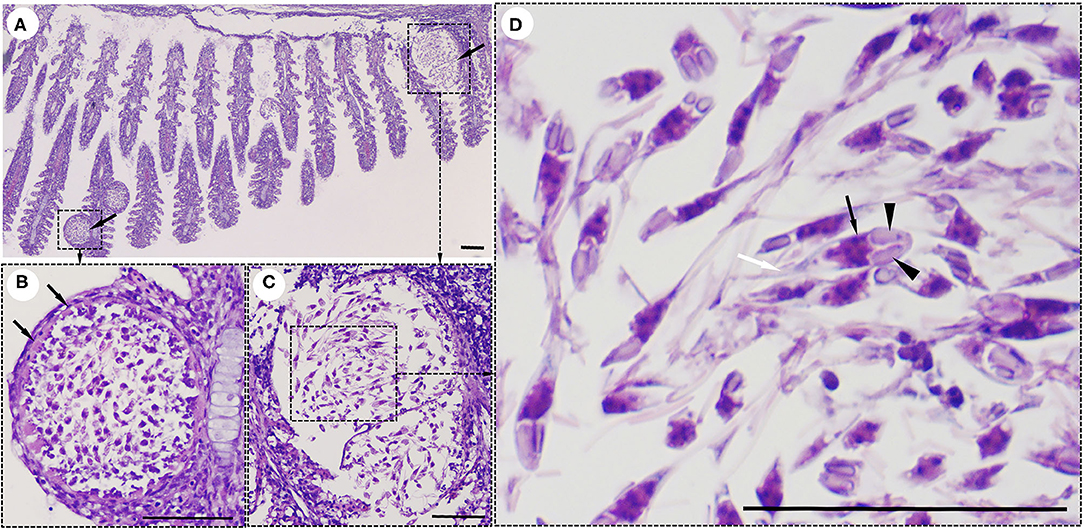
Figure 3. Histological lesions in the gills of the snakeskin gourami (Trichopodus pectoralis) infected with Henneguya sp. (A) Plasmodia (arrows) showing different developmental stages. (B) Young plasmodium was roundish and encased in a wall of epithelial cells (arrows). Immature myxospores were located in the periphery of the plasmodia and mature myxospores in the center. (C) A grown plasmodium ruptured the envelope. (D) The myxospores have typical features of a Henneguya sp. including two equal polar caps (arrowheads), sporoplasm at the posterior pole of the spore (black arrow), and two long, superimposed caudal processes (white arrow). Slides were stained with hematoxylin and eosin (H&E), scale bar = 50 μm.
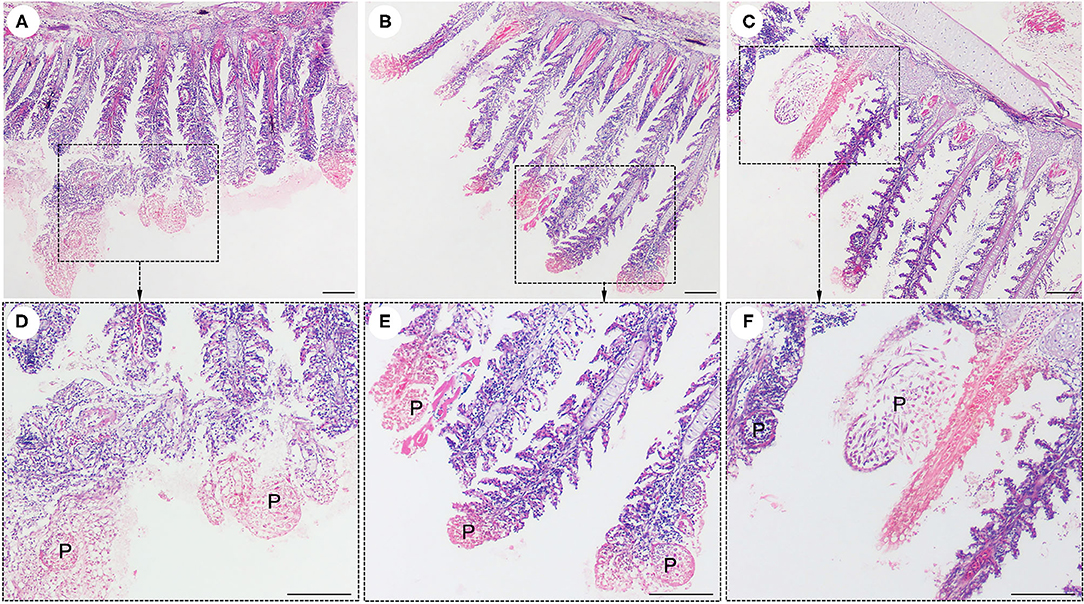
Figure 4. Histological lesions in the gills of the snakeskin gourami (Trichopodus pectoralis) infected with Henneguya sp. The plasmodia (P) caused severe distortion of the lamellar structure and obstruction of the gills by compression of the cysts (A–C). Higher magnification indicated plasmodia occupied part of the gill lamellae and caused marked dilation and discrete epithelial hyperplasia (D,E). The plasmodia grew and compressed the adjacent tissue and caused tissue necrosis in the infected area (E,F). Slides were stained with hematoxylin and eosin (H&E), scale bar = 100 μm.
Molecular Analyses
All collected samples (n = 4) were positive after repeated PCR amplification of the 16S rRNA gene of Chlamydiales (Supplementary Figure 1). Multiple sequence alignment of the partial 16S rRNA gene sequence of the T. pectoralis chlamydial pathogen from 4 representative infected fish, showed that the sequences were identical, and the consensus sequence (766 bp) was deposited in GenBank under accession number MW832782. BLAST-n search of consensus sequence in the NCBI database revealed closest sequence similarity (93.5%) with the partial 16S rRNA gene of a non-cultured bacterium (Accession number LN612734.1) obtained from a case of gill disease in Mediterranean Sea bream, followed by Candidatus Piscichlamydia sp. (92.3%) (Accession Number KY380090.1), which is associated with epitheliocystis infections in cyprinids. These bacteria formed a clade as an unclassified family belonging to the genus Candidatus Piscichlamydia within the order Chlamydiales. The accession numbers and taxonomic identities, as well as the origin of the organisms included in this phylogenetic analysis, are shown in Figure 5. This phylogenetic analysis confirmed that the bacterial pathogen in the case of the snakeskin gourami separated in a unique branch, representing a novel species, proposed name “Candidatus Piscichlamydia trichopodus”, a new member of the order Chlamydiales.
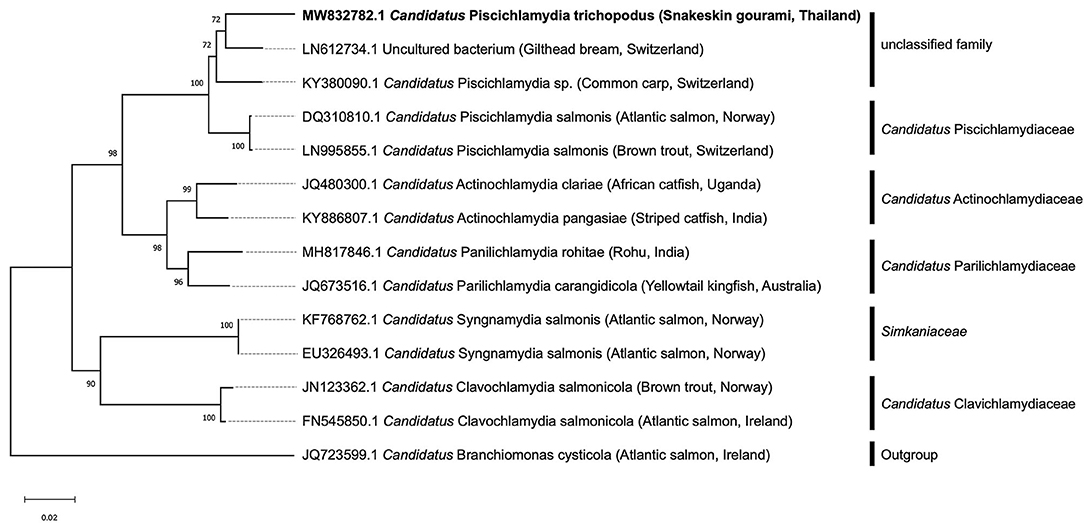
Figure 5. Phylogenetic tree was constructed based on the partial 16S rRNA sequence (766 bp) of the snakeskin gourami (Trichopodus pectoralis) from this study (MW832782) and closely related species. The accession numbers and taxonomic identities, as well as the host origin of the organisms included in this phylogenetic analysis, are shown. Candidatus Branchiomonas cysticola was selected as the outgroup. The tree was constructed using the neighbor-joining method. The scale bar represents 0.02—nucleotide substitution per site, while the number at the node of the tree indicates the bootstrap value in percent.
Discussion
Members of the order Chlamydiales are a diverse group of Gram-negative, obligate intracellular bacteria that are distributed worldwide and cause a wide variety of diseases in humans, livestock, domestic animals, wildlife, and exotic species (18, 27, 28). Chlamydia-like organisms (CLOs), commonly found in aquatic environments, have been identified as causing disease in at least 90 fish species in both freshwater and marine environments (16–18). A common feature of these bacteria is the infection of epithelial cells of fish, causing typical lesions in the form of epitheliocystis (15–18, 29, 30). Epitheliocystis as a result of CLOs infection have been described in several farmed fishes, including common carp Cyprinus carpio (31), red seabream Pagrus major (15), African catfish Clarias gariepinus (32), yellowtail kingfish Seriola lalandi (33), striped trumpeter Latris lineata (34) barramundi Lates calcarifer (35), striped catfish Pangasius hypophthalmus (36), and rohu Labeo rohita (23). In contrast to previous studies, our result showed that the histopathological changes associated with CPT infection revealed massive intracellular colonization but not obvious as epitheliocystis. Interestingly, the microbial pathogen in this study likely shares similarities with the CLO pathogen that causes systemic microbial disease in the Dungeness crab, Cancer magister (37). Both pathogens show systemic infection with numerous colonies of the organism having strong affinity for connective tissue and connective tissue cells, while rarely infecting epithelial cells. Several previous studies reported that CLOs can infect other cell types, including mucous cells in common carp Cyprinus carpio L. (38), pillar cells in tiger puffer Takifugu rubripes (39), macrophages in brown bullhead Ictalurus nebulosus (40) and chloride cells in Atlantic salmon Salmo salar (41). These studies have also indicated that epitheliocystis due to CLO is typical but not always observed. Moreover, the localization of CPT near the damaged cartilaginous tissue suggests that these bacteria may require cartilage for their metabolism. This histopathological feature could be considered in the presumptive diagnosis of this new pathogen. It is increasingly recognized that these pathogens are actually diverse in their morphology, e.g., the morphology of CLO organisms in the epithelial cysts and their staining characteristics, as well as the morphology of their capsules and their location in fish tissue (42–44). This is the first detection of CLO in snakeskin gourami and may represent another Chlamydia that is not associated with epitheliocystis. Since CLO pathogens cannot be distinguished by morphology or conventional culture methods, molecular methods are widely used to detect and characterize the causative pathogen. Apart from histopathological lesion of the bacterial foci, CLO infection was confirmed by ISH and specific PCR assay. Although the short 16S rRNA signature sequence detected in infected tissue is not ideal for detailed phylogenetic analysis, it is unique. Our sequence showed only a distant similarity of 93.5 and 92.3%, respectively, with a query coverage of 98% to the uncultured bacterium from gilthead sea bream and Candidatus Piscichlamydia sp. associated with epitheliocystis infections in cyprinids (30). There bacteria formed a clade as an unclassified family belonging to the genus Candidatus Piscichlamydia within the order Chlamydiales and also shared >90% sequence similarity with other members of the family Candidatus Piscichlamydiaceae, suggesting that these pathogens belong to the same family (21) or are closely related. Since the causative agent shared <95% similarity with other previously reported Chlamydia-like 16S rRNA sequences, the sequenced bacteria are new to the order Chlamydiales (21). These results highlight the wide genetic diversity within this bacterial group and are consistent with previous findings (29) that each new fish host indicates the existence of a phylogenetically distinct and novel Chlamydia infection.
In the present case, the diagnosis was challenging due to mixed infection of CPT along with a Henneguya sp. parasite in the affected gill tissues. The classification of Henneguya sp. was tentatively based on a unique morphological characteristic of its spore with caudal appendage (6, 9, 20, 45). Accurate identification of species therefore requires a combination of systematic morphological descriptions and sequencing of the small subunit ribosomal RNA gene (SSU) for phylogenetic analysis (10, 46). Previous studies have described that Henneguya sp. has the characteristic morphological feature of two equal polar capsules, sporoplasm on the posterior pole, and 2 independent caudal processes, which distinguishes it from the other genera of the family Myxobolidae (7, 9, 10, 47). The species of the genus Henneguya interact with the gill structures of fish in different manners, resulting in varying degrees of disease (11, 12, 48). The clinical signs noted by the farmer in the snakeskin gourami, particularly lethargy, gasping for oxygen at the water surface, and loss of appetite, are comparable with those previously documented in fish affected by Henneguya sp. (49–51). The most common histological lesions found in this case were distortion of the lamellar structure and obstructions of the gills due to compression of the cysts, which most likely caused respiratory distress and contributed to the observed mortality. Histopathological features similar to those described in this study have been observed in previous studies (52–54), in which parasitism by Henneguya sp. resulted in marked dilatation and discrete epithelial hyperplasia, and continued growth of the parasitic cyst resulted in tissue necrosis in the surrounding infected area. The observation of areas of cystic lesions associated with tissue necrosis caused by the compression of cysts in the epithelial cells of the gill lamellae in this study has also been reported in previous studies (55). The growth of Henneguya plasmodia results in a displacement and distortion of the lamellar structures, possibly adversely affecting gas exchange and associated with mortality in the fish population.
Unfortunately, due to the occurrence of co-infections and the unavailability of culture methods for fish CLOs, it was not possible to determine which pathogens were directly responsible for the associated mortality. Interestingly, the disease is sensitive to tetracyclines, since the antibiotic treatment with oxytetracycline in the field was effective, hence we assumed that the disease might have been caused primarily by the CLO. As previously reported by Goodwin et al. (56), OTC significantly reduced mortality due to chlamydial infection and supported this treatment regimen for future outbreaks. The cause and pathogenesis of this dual infection remain speculative and await the development of a culture technique and isolation of the Chlamydia-like organism before further in vitro studies. It is unclear whether Henneguya sp. and CPT has the potential of causing a significant impact on snakeskin gourami aquaculture. Given the commercial importance of the snakeskin gourami Trichopodus pectoralis and its great aquaculture potential, the results of this study highlight the need of follow up investigations on ultrastructural morphology, host range, prevalence, risk factors for disease, and mode of transmission. This could lead to a better understanding of the pathogen's biology and disease epidemiology for development of effective control measures.
In conclusion, the presence of pathogenic potential of mixed infection of a novel intracellular CLO (Candidatus Piscichlamydia trichopodus) and a gill parasite Henneguya sp. in snakeskin gourami in Thailand is reported for the first time. This study expands the knowledge of the pathology of snakeskin gourami, an important fish species in Asian aquaculture, and contributes to initial understanding of diseases in this fish species.
Data Availability Statement
The datasets presented in this study can be found in online repositories. The names of the repository/repositories and accession number(s) can be found in the article/Supplementary Material.
Ethics Statement
The animal study was reviewed and approved by the Institutional Animal Care and Use Committee of Kasetsart University (Approval ID: ACKU63-FIS-009).
Author Contributions
PK and HD: involved in conceptualization. ND-H, CS, SN, and PK: underwent investigation. SN: resources. ND-H, HD, and PK: performed formal analysis and wrote the original draft. ND-H, HD, CS, CR, SN, PS, SC, and PK: reviewed and edited. PK: one of the lead authors and was deceased prior to the submission of this manuscript. All authors have read and agreed to the current version of the manuscript.
Funding
This work was carried out with support from the Center of Excellence in Aquatic Animal Health Management, Faculty of Fisheries, Kasetsart University.
Conflict of Interest
The authors declare that the research was conducted in the absence of any commercial or financial relationships that could be construed as a potential conflict of interest.
Publisher's Note
All claims expressed in this article are solely those of the authors and do not necessarily represent those of their affiliated organizations, or those of the publisher, the editors and the reviewers. Any product that may be evaluated in this article, or claim that may be made by its manufacturer, is not guaranteed or endorsed by the publisher.
Supplementary Material
The Supplementary Material for this article can be found online at: https://www.frontiersin.org/articles/10.3389/fvets.2022.847977/full#supplementary-material
Supplementary Figure S1. Confirmation of PCR test results under agarose gel electrophoresis of samples from four representative fish. Lanes 1 and 6 were amplified without DNA template using primer set 1 and 2, respectively, as negative controls. Lanes 2, 3, 4, and 5 were amplified with the DNA template from primer set 1 (300 bp). Lanes 7, 8, 9, and 10 were amplified with the DNA template from primer set 2 (766 bp). Lane M was a DNA marker (Himedia, India).
References
1. Paepke H. The nomenclature of Trichopodus pectoralis Regan, 1910; Trichopus cantoris Sauvage, 1884 and Osphronemus saigonensis Borodin, 1930 (Teleostei: Peciformes: Osphronemidae). Vertebr Zool. (2009) 59:49–56.
2. FAO. Fishery and Aquaculture Statistics. Global Aquaculture Production 1950-2019 (FishstatJ). Rome: FAO Fisheries Division. Available online: http://www.fao.org/fishery/countrysector/naso_thailand/en (accessed June 19, 2021).
3. DOF. Statistics of Freshwater Aquaculture Production. Fisheries Development Policy and Strategy Division No. 8/2018. (2016). Bangkok: Department of Fisheries, Ministry of Agriculture and Cooperatives (2018).
4. Kanchan C, Imjai P, Kanchan N, Chaiyara A, Panchai K. Occurrence of parasitic and bacterial diseases in Thai freshwater fish. J Agric Crop Resh. (2020) 8:210–4. doi: 10.33495/jacr_v8i10.20.168
5. U.S Fish & Wildlife Service. Snakeskin Gourami (Trichopodus pectoralis) Ecological Risk Screening Summary. (2019). Available online: https://www.fws.gov/fisheries/ans/erss/uncertainrisk/ERSS-Trichopodus-pectoralis_Final.pdf (accessed June 19, 2021).
6. Lom J, Dyková I. Myxozoan genera: definition and notes on taxonomy, life-cycle terminology and pathogenic species. Folia Parasitol. (2006) 53:1–36. doi: 10.14411/fp.2006.001
7. Molnár K. Site preference of fish myxosporeans in the gill. Dis Aquat Organ. (2002) 48:197–207. doi: 10.3354/dao048197
8. Eiras JC, Adriano EA. A checklist of new species of Henneguya Thélohan, 1892 (Myxozoa: Myxosporea, Myxobolidae) described between 2002 and 2012. Syst Parasitol. (2012) 83:95–104. doi: 10.1007/s11230-012-9374-7
9. Lom J, Arthur JR A. guideline for the preparation of species descriptions in Myxosporea. J Fish Dis. (1989) 12:151–6. doi: 10.1111/j.1365-2761.1989.tb00287.x
10. Fiala I, Bartošová-Sojková P, Whipps CM. Classification and Phylogenetics of Myxozoa. In: Myxozoan Evolution, Ecology and Development, eds. Okamura B, Gruhl A, Bartholomew JL. Springer International Publishing. (2015), p. 85–110.
11. Kent ML, Andree KB, Bartholomew JL, El-Matbouli M, Desser SS, Devlin RH, et al. Recent advances in our knowledge of the Myxozoa. J Eukaryot Microbiol. (2001) 48:395–413. doi: 10.1111/j.1550-7408.2001.tb00173.x
12. Dykova I, Lom J. Histopathological changes in fish gills infected with myxosporidian parasites of the genus Henneguya. J Fish Biol. (1978) 12:197–202. doi: 10.1111/j.1095-8649.1978.tb04165.x
13. Whitaker JW, Pote LMW, Hanson LA. Assay to detect the actinospore and myxospore stages of proliferative gill disease in oligochaetes and pond water. N Am J Aquac. (2005) 67:133–7. doi: 10.1577/A03-059.1
14. Haaparanta A, Valtonen ET, Hoffmann RW. Pathogenicity and seasonal occurrence of Henneguya-creplini (Protozoa, Myxosporea) on the gills of perch Perca-Fluviatilis in central Finland. Dis Aquat Organ. (1994) 20:15–22. doi: 10.3354/dao020015
15. Nowak BF, LaPatra SE. Epitheliocystis in fish. J Fish Dis. (2006) 29:573–88. doi: 10.1111/j.1365-2761.2006.00747.x
16. Blandford MI, Taylor-Brown A, Schlacher TA, Nowak B, Polkinghorne A. Epitheliocystis in fish: an emerging aquaculture disease with a global impact. Transbound Emerg Dis. (2018) 65:1436–46. doi: 10.1111/tbed.12908
17. Pawlikowska-Warych M, Deptula W. Characteristics of Chlamydia-like organisms pathogenic to fish. J Appl Genet. (2016) 57:135–41. doi: 10.1007/s13353-015-0303-8
18. Borel N, Polkinghorne A, Pospischil A. A review on chlamydial diseases in animals: still a challenge for pathologists? Vet Pathol. (2018) 55:374–90. doi: 10.1177/0300985817751218
19. Yoonpundh R, Little D. Trends in the farming of the snakeskin gourami (Trichogaster pectoralis) in Thailand. Naga, The WorldFish Center. (1997) 20:18–20.
20. Fiala I, Bartošová-Sojková P, Okamura B, Hartikaine H. Adaptive radiation and evolution within the myxozoa. In: Myxozoan Evolution, Ecology and Development, editors. Okamura B, Gruhl A, Bartholomew JL. Springer International Publishing. (2015). p. 69–84. doi: 10.1007/978-3-319-14753-6_4
21. Everett KDE, Bush RM, Andersen AA. Emended description of the order Chlamydiales, proposal of Parachlamydiaceae fam. nov and Simkaniaceae fam nov, each containing one monotypic genus, revised taxonomy of the family Chlamydiaceae, including a new genus and five new species, and standards for the identification of organisms. Int J Syst Bacteriol. (1999) 49:415–40. doi: 10.1099/00207713-49-2-415
22. Relman D Universal bacterial 16S rRNA amplification and sequencing. In: Persing DH, Smith TF, Tenover FC, White TJ, editors. Diagnostic Molecular Biology, Principles and Applications. (1993). p. 489–95.
23. Sood N, Pradhan PK, Verma DK, Gupta S, Ravindra Dev AK, et al. Epitheliocystis in rohu Labeo rohita (Hamilton, 1822) is caused by novel Chlamydiales. Aquaculture. (2019) 505:539–43. doi: 10.1016/j.aquaculture.2019.03.007
24. Draghi AII, Popov VL, Kahl MM, Stanton JB, Brown CC, Tsongalis GJ, et al. Characterization of “Candidatus piscichlamydia salmonis” (order Chlamydiales), a chlamydia-like bacterium associated with epitheliocystis in farmed Atlantic salmon (Salmo salar). J Clin Microbiol. (2004) 42:5286–97. doi: 10.1128/JCM.42.11.5286-5297.2004
25. Kumar S, Stecher G, Li M, Knyaz C, Tamura K. MEGA X molecular evolutionary genetics analysis across computing platforms. Mol Biol Evol. (2018) 35:1547–9. doi: 10.1093/molbev/msy096
26. Dinh-Hung N, Sangpo P, Kruangkum T, Kayansamruaj P, Rung-Ruangkijkrai T, Senapin S., et al. Dissecting the localization of Tilapia tilapinevirus in the brain of the experimentally infected Nile tilapia, Oreochromis niloticus (L). J Fish Dis. (2021) 44:1053–64. doi: 10.1111/jfd.13367
27. Corsaro D, Valassina M, Venditti D. Increasing diversity within Chlamydiae. Crit Rev Microbiol. (2003) 29:37–78. doi: 10.1080/713610404
28. Horn M. Chlamydiae as symbionts in eukaryotes. Annu Rev Microbiol. (2008) 62:113–31. doi: 10.1146/annurev.micro.62.081307.162818
29. Stride MC, Polkinghorne A, Nowak BF. Chlamydial infections of fish: diverse pathogens and emerging causes of disease in aquaculture species. Vet Microbiol. (2014) 170:19–27. doi: 10.1016/j.vetmic.2014.01.022
30. Sellyei B, Molnár K, Székely C. Diverse Chlamydia-like agents associated with epitheliocystis infection in two cyprinid fish species, the common carp (Cyprinus carpio L.) and the gibel carp (Carassius auratus gibelio L.). Acta Vet Hung. (2017) 65:29–40. doi: 10.1556/004.2017.003
31. Kim DJ, Park JH, Seok SH, Cho SA, Baek MW, Lee HY, et al. Epitheliocystis in carp (Cyprinus carpio) in South Korea. J Vet Med Sci. (2005) 67:119–20. doi: 10.1292/jvms.67.119
32. Steigen A, Nylund A, Karlsbakk E, Akoll P, Fiksdal IU, Nylund S, et al. ‘Cand. Actinochlamydia clariaeh gen nov, sp nov, a unique intracellular bacterium causing epitheliocystis in catfish (Clarias gariepinus) in Uganda. PLoS ONE. (2013) 8:e66840. doi: 10.1371/journal.pone.0066840
33. Stride MC, Polkinghorne A, Miller TL, Groff JM, Lapatra SE, Nowak BF. Molecular characterization of “Candidatus Parilichlamydia carangidicola,” a novel Chlamydia-like epitheliocystis agent in yellowtail kingfish, Seriola lalandi (Valenciennes), and the proposal of a new family, “Candidatus Parilichlamydiaceae” fam. nov (order Chlamydiales). Appl Environ Microbiol. (2013) 79:1590–7. doi: 10.1128/AEM.02899-12
34. Stride MC, Polkinghorne A, Miller TL, Nowak BF. Molecular characterization of “Candidatus Similichlamydia latridicola” gen. nov, sp nov (Chlamydiales: “Chlamydial Parilichlamydiaceaeary a novel Chlamydia-like epitheliocystis agent in the striped trumpeter, Latris lineata (Forster). Appl Environ Microbiol. (2013) 79:4914–914 doi: 10.1128/AEM.00746-13
35. Stride MC, Polkinghorne A, Powell MD, Nowak BF. “Candidatus Similichlamydia laticola”, a novel Chlamydia-like agent of epitheliocystis in seven consecutive cohorts of farmed Australian barramundi, Lates calcarifer (Bloch). PLoS ONE. (2013) 8:e82889. doi: 10.1371/journal.pone.0082889
36. Sood N, Pradhan PK, Verma DK, Yadav MK, Ravindra Dev AK, et al. Candidatus Actinochlamydia pangasiae sp nov (Chlamydiales, Actinochlamydiaceae), a bacterium associated with epitheliocystis in Pangasianodon hypophthalmus. J Fish Dis. (2018) 41:281–90. doi: 10.1111/jfd.12711
37. Sparks AK, Morado JF, Hawkes JW. A systemic microbial disease in the Dungeness crab, Cancer magister, caused by a Chlamydia-like organism. J Invertebr Pathol. (1985) 45:204–17. doi: 10.1016/0022-2011(85)90010-2
38. Paperna I, Alves de Matos AP. The developmental cycle of epitheliocystis in carp, Cyprinus carpio L. J Fish Dis. (1984) 7:137–47. doi: 10.1111/j.1365-2761.1984.tb00916.x
39. Miyazaki T, Fujimaki Y, Hatai K. A light and electron microscopic study on epitheliocystis disease in cultured fishes. Nippon Suisan Gakkaishi. (1986) 52:199–202. doi: 10.2331/suisan.52.199
40. Desser S, Paterson W, Steinhagen D. Ultrastructural observations on the causative agent of epitheliocystis in the brown bullhead, Ictalurus nebulosus Lesueur, from Ontario and a comparison with the Chlamydiae of higher vertebrates. J Fish Dis. (1988) 11:453–60. doi: 10.1111/j.1365-2761.1988.tb00744.x
41. Nylund A, Kvenseth AM, Isdal E. A morphological study of the epitheliocystis agent in farmed Atlantic Salmon. J Aquat Anim Health. (1998) 10:43–55. doi: 10.1577/1548-8667(1998)010<0043:AMSOTE>2.0.CO;2
42. Work TM, Rameyer RA, Takata G, Kent ML. Protozoal and epitheliocystis-like infections in the introduced bluestripe snapper Lutjanus kasmira in Hawaii. Dis Aquat Organ. (2003) 57:59–66. doi: 10.3354/dao057059
43. Crespo S, Zarza C, Padrós F, Marín de Mateo M. Epitheliocystis agents in sea bream Sparus aurata: morphological evidence for two distinct Chlamydia-like developmental cycles. Dis Aquat Organ. (1999) 37:61–72. doi: 10.3354/dao037061
44. Guevara Soto M, Vaughan L, Segner H, Wahli T, Vidondo B, Schmidt-Posthaus H. Epitheliocystis distribution and dharacterization in brown trout (Salmo trutta) from the headwaters of two major European rivers, the Rhine and Rhone. Front Physiol. (2016) 7:131. doi: 10.3389/fphys.2016.00131
45. Atkinson S, Bartošová-Sojková P, Whipps C. Approaches for characterizing myxozoan species. In: Okamura B, Gruhl A, Bartholomew JL, editors. Myxozoan Evolution, Ecology and Development, editors. Switzerlan: Springer International Publishing (2015). p. 111–23.
46. Liu Y, Lövy A, Gu Z, Fiala I. Phylogeny of Myxobolidae (Myxozoa) and the evolution of myxospore appendages in the Myxobolus clade. Int J Parasitol. (2019) 48:523–30. doi: 10.1016/j.ijpara.2019.02.009
47. Eiras JC. Synopsis of the species of the genus Henneguya Thélohan, 1892 (Myxozoa: myxosporea: myxobolidae. Syst Parasitol. (2002) 52:43–54. doi: 10.1023/A:1015016312195
48. Molnár K, Eszterbauer E. Specificity of infection sites in vertebrate hosts. In: Okamura B, Gruhl A, Bartholomew JL, editors. Myxozoan Evolution, Ecology and Development. Springer International Publishing (2015). p. 295–313.
49. Sabri DM, Eissa IAM, Danasoury MA, Khouraiba HM. Prevalence of Henneguya branchialis in Catfish (Clarias gariepinus) in Ismailia, Egypt. Int J Agric Biol. (2010) 12:897–900.
50. Videira M, Velasco M, Malcher CS, Santos P, Matos P, Matos E. An outbreak of myxozoan parasites in farmed freshwater fish Colossoma macropomum (Cuvier, 1818) (Characidae, Serrasalminae) in the Amazon region, Brazil. Aquac. (2016) 3:31–4. doi: 10.1016/j.aqrep.2015.11.004
51. Stilwell JM, Camus AC, Leary JH, Khoo LH, Griffin MJ. Pathologic changes associated with respiratory compromise and morbidity due to massive interlamellar Henneguya exilis infection in Channel × Blue Hybrid Catfish. J Parasitol. (2019) 105:686–92. doi: 10.1645/19-28
52. Özer A, Özkan H, Gürkanli CT, Yurakhno V, Çiftçi Y. Morphology, histology and phylogeny of Henneguya sinova sp. nov (Myxobolidae: Myxozoa) infecting gills of Parablennius tentacularis in the Black Sea, Turkey. Dis Aquat Organ. (2016) 118:207–15. doi: 10.3354/dao02968
53. Úngari LP, Vieira DHMD, da Silva RJ, Santos ALQ, de Azevedo RK, O'Dwyer LH, et al. new myxozoan species Henneguya unitaeniata sp. nov (Cnidaria: Myxosporea) on gills of Hoplerythrinus unitaeniatus from Mato Grosso State, Brazil. Parasitol Res. (2019) 118:3327–36. doi: 10.1007/s00436-019-06533-1
54. Yokoyama H, Itoh N, Tanaka S. Henneguya pagri n. sp (Myxozoa: Myxosporea) causing cardiac henneguyosis in red sea bream, Pagrus major (Temminck & Schlegel). J Fish Dis. (2005) 28:479–87. doi: 10.1111/j.1365-2761.2005.00655.x
55. Figueredo RTA, de Oliveira JEF, Vilhena MPSP, Berredo J, dos Santos WJP, Matos E, et al. Henneguyosis in gills of Metynnis hypsauchen: an Amazon freshwater fish. J Parasit Dis. (2020) 44:213–20. doi: 10.1007/s12639-019-01183-7
Keywords: Chlamydiales, Chlamydia-like organism, Henneguya sp., snakeskin gourami, Candidatus Piscichlamydia trichopodus, 16S rRNA
Citation: Dinh-Hung N, Dong HT, Soontara C, Rodkhum C, Nimitkul S, Srisapoome P, Kayansamruaj P and Chatchaiphan S (2022) Co-infection of Candidatus Piscichlamydia Trichopodus (Order Chlamydiales) and Henneguya sp. (Myxosporea, Myxobolidae) in Snakeskin Gourami Trichopodus pectoralis (Regan 1910). Front. Vet. Sci. 9:847977. doi: 10.3389/fvets.2022.847977
Received: 03 January 2022; Accepted: 03 February 2022;
Published: 09 March 2022.
Edited by:
Valentina Virginia Ebani, University of Pisa, ItalyReviewed by:
Dieter Steinhagen, University of Veterinary Medicine Hannover, GermanyLuis Garcia Prieto, Universidad Nacional Autónoma de México, Mexico
Copyright © 2022 Dinh-Hung, Dong, Soontara, Rodkhum, Nimitkul, Srisapoome, Kayansamruaj and Chatchaiphan. This is an open-access article distributed under the terms of the Creative Commons Attribution License (CC BY). The use, distribution or reproduction in other forums is permitted, provided the original author(s) and the copyright owner(s) are credited and that the original publication in this journal is cited, in accordance with accepted academic practice. No use, distribution or reproduction is permitted which does not comply with these terms.
*Correspondence: Pattanapon Kayansamruaj, cGF0dGFuYXBvbi5rQGt1LnRo; Satid Chatchaiphan, c2F0aWQuY2hAa3UudGg=
†Deceased