- 1Department of Small Animal Medicine and Surgery, University of Veterinary Medicine, Hannover, Germany
- 2Institute for Laboratory Animal Science, Hannover Medical School, Hannover, Germany
- 3BrainCheck.Pet® – Tierärztliche Praxis für Epilepsie, Sachsenstraße, Mannheim, Germany
- 4Department of Clinical Science and Services, Royal Veterinary College, Hatfield, United Kingdom
Epilepsy is the most common chronic neurological disease in humans and dogs. Epilepsy is thought to be caused by an imbalance of excitatory and inhibitory neurotransmission. Intact neurotransmitters are transported from the central nervous system to the periphery, from where they are subsequently excreted through the urine. In human medicine, non-invasive urinary neurotransmitter analysis is used to manage psychological diseases, but not as yet for epilepsy. The current study aimed to investigate if urinary neurotransmitter profiles differ between dogs with epilepsy and healthy controls. A total of 223 urine samples were analysed from 63 dogs diagnosed with idiopathic epilepsy and 127 control dogs without epilepsy. The quantification of nine urinary neurotransmitters was performed utilising mass spectrometry technology. A significant difference between urinary neurotransmitter levels (glycine, serotonin, norepinephrine/epinephrine ratio, ɤ-aminobutyric acid/glutamate ratio) of dogs diagnosed with idiopathic epilepsy and the control group was found, when sex and neutering status were accounted for. Furthermore, an influence of antiseizure drug treatment upon the urinary neurotransmitter profile of serotonin and ɤ-aminobutyric acid concentration was revealed. This study demonstrated that the imbalances in the neurotransmitter system that causes epileptic seizures also leads to altered neurotransmitter elimination in the urine of affected dogs. Urinary neurotransmitters have the potential to serve as valuable biomarkers for diagnostics and treatment monitoring in canine epilepsy. However, more research on this topic needs to be undertaken to understand better the association between neurotransmitter deviations in the brain and urine neurotransmitter concentrations in dogs with idiopathic epilepsy.
Introduction
Dogs and humans are affected by naturally occurring epilepsy, a complex brain disorder characterised by a predisposition to experience recurring seizure events (1–3). It is one of the most common chronic neurological diseases in both species, with many shared clinical and epidemiological characteristics (4–6). Around two-thirds of the affected dogs and half of the human patients do not become seizure free, despite pharmacological treatment (7, 8). Persistent uncontrollable seizures are a health concern increasing mortality, causing psychological and physical stress, and culminating in a negative impact on the overall quality of life (9, 10).
Epileptic seizures are initiated by abnormally excessive or synchronous neuronal activity in the cerebral cortex or hippocampus of the brain (11). The exact pathogenesis of this process has not yet been solved. However, a contributing factor to the underlying pathophysiology of seizures may arise from the imbalance of excitatory and inhibitory neurotransmission, caused by neurotransmitter and receptor alterations (12, 13).
In the past decades, evidence in humans and primates has suggested that seizures were correlated to altered neurotransmitter concentrations of glutamate, ɤ-aminobutyric acid (GABA) and serotonin, which were measured in the extracellular fluid, cerebrospinal fluid (CSF) and serum (14–17). A deviating neurotransmitter composition, caused by a dysfunctional neurotransmitter metabolism in humans, can also result in seizures and other neurological signs (18).
Emerging seizures can also be linked to changes in neurotransmitter receptors. In earlier studies, the altered GABA or dopamine (DA) receptor density was accompanied by seizures or seizure susceptibility in human patients and rodents (19–22). Changes in receptor function, such as binding potential or endogenous activity of glutamate, GABA or serotonin receptors were found in humans suffering from temporal lobe epilepsy (23–27). Additionally, a divergent composition of glutamate or GABA receptor subunits has also been associated with recurring seizures in animal models and humans (28–31). Those detected subunit compositions were similar to those of the more excitatory immature brain and facilitated further seizures and epileptogenesis (32).
In the central nervous system (CNS), glutamate is the major excitatory neurotransmitter, whereas GABA is the primary inhibitory counterpart (33, 34). The equilibrium of these two neurotransmitters maintains the balance of cell excitability. The aforementioned alterations that affect one or both of these neurotransmitters are likely to elicit a shift to arousal in the brain, followed by seizures (13). The neurotransmitter serotonin is known for its anticonvulsant properties and regulation of mood and cognition (35, 36). Therefore, disturbances in the serotonergic system are assumed to evoke seizures and promote frequently developed neurobehavioural/-psychiatric comorbidities associated with epilepsy in dogs and humans (37–43).
In the body, intact neurotransmitters of the CNS are transported through the blood-brain barrier (BBB) to the peripheral systemic circulation, from where they are primarily excreted through the kidney into urine (44–47). The neurobiological basis of this process is poorly understood. It is substrate-specific and can vary for each neurotransmitter (48). However, several animal studies demonstrated an association between central and peripheral neurotransmitter output into the urine (49–52). Moreover, positive correlating neurotransmitter concentrations of serine, glycine and norepinephrine (NE) between the CSF, blood and urine in dogs were recently revealed, emphasising a connection (53).
In human medicine, non-invasive urinary neurotransmitter analysis is used to manage medical conditions such as depression and attention-deficit hyperactivity disorder (ADHD) (54–57). Patients affected by depressive and anxiety symptoms showed increased urinary catecholamines, like NE and epinephrine (E) (58–60). Suicide attempts in depression were strongly associated with urinary excreted DA, even greater than the CSF concentration (61). ADHD symptoms correlated with alterations of the urinary catecholamines NE and E, and a decrease in urinary phenylethylamine (PEA), which is linked to inattentiveness (62–66).
Urinary neurotransmitter analysis is not as yet used for epilepsy management in either humans or dogs, to the authors' knowledge. However, promising evidence was provided in a recent study, indicating altered urinary neurotransmitter patterns associated with the treatment efficacy of medium-chained triglyceride (MCT) oil in drug-resistant canine epilepsy (67). Intake of MCT oil increased urinary GABA concentration in dogs with IE. Also, the GABA/glutamate ratio changed significantly by decreased glutamate levels compared to GABA levels in dogs affected by epilepsy. Furthermore, non-responders without a reduction in seizure frequency below 50% excreted higher glutamate, histamine and serotonin levels in their urine (67).
This study investigated whether urinary neurotransmitter profiles differ between dogs with epilepsy and non-epileptic controls. We hypothesised that urinary neurotransmitter analysis could provide a non-invasive diagnostic tool, where characteristic neurotransmitter deviations can serve as valuable biomarkers in epilepsy research and clinical management.
Materials and Methods
Sample Acquisition
In this multicentre study a total of 223 urine samples were collected from 190 privately owned dogs (both sexes; mixed or pure breed) and divided into two cohorts. From the first cohort, 96 urine samples from 63 subjects with idiopathic epilepsy (IE) were obtained. Dogs in the IE cohort had no acute or chronic diseases of the gastrointestinal tract, kidney, liver or heart failure. They met at least the requirements of Tier I (n = 15) confidence level of the International Veterinary Epilepsy Task Force (IVETF) for the diagnosis of IE, however, most dogs met Tier II (n = 48). Two adjustments to IVETF criterion were applied, as long as magnetic resonance imaging was unremarkable: firstly, abnormalities in the interictal neurological examination caused by antiseizure drug (ASD) treatment were tolerated and secondly, the maximum age at seizure onset was increased to 12 years (68). Samples were collected and analysed as part of three former epilepsy studies, between October 2012 and September 2017, at international study sites: Queen Mother Hospital for Animals, Royal Veterinary College, London, UK (RVC) (n = 59: 29 paired samples collected from the same individual at certain study stages; 30 unpaired); University of Veterinary Medicine Hannover, Hannover, Germany (TiHo) (n = 3, paired); University of Helsinki, Helsinki, Finland (n = 1, paired) (69–72).
The second cohort was a control group of 127 healthy dogs. All control cohort subjects were at least 1 year of age, did not receive any medication and had no chronic diseases. One hundred (n = 100) of the second cohort samples were collected from dogs owned by TiHo staff and students, between January and June 2020. The remaining 27 samples were obtained from healthy control dogs at the RVC study site.
To avoid bias of the study results, the dogs were not fed milk products, fruits and vegetables 48 h before sample acquisition. Exposition to strenuous exercise was also avoided for 24 h before sampling. Bitches were not in heat during the collection process. The urine samples were collected via the free catch method. The first or second void of morning urine from the fasting dog (preferable midstream) was used for urinary neurotransmitter analysis. Samples were transferred into a tube containing a preservative to ensure sample stability (50 mg oxalic acid/10 ml urine), followed by an immediate transport to the TiHo laboratory. Samples from the other study sites (London, Helsinki) were collected as part of an enrolment or study visit for epilepsy trials with MCT, epilepsy behaviour studies or from healthy controls of the previously mentioned studies and were directly cooled on ice (69–72).
Sample Preparation and Analysis
Samples were aliquoted and quickly frozen at the different study site laboratories. They were stored at −80°C for at least 4–6 h prior to shipment. The preserved urine samples were continuously frozen and shipped on dry ice for external analysis of neurotransmitter concentrations to “Doctor's Data,” St. Charles, IL, USA. Nine urinary neurotransmitter levels (serotonin, histamine, glycine, phenylethylamine, DA, E, NE, glutamate, GABA) were quantified utilising High-performance liquid chromatography triple-quadrupole mass spectrometry/mass spectrometry (HPLC-QqQ MS/MS) technology. In addition, creatinine levels were measured by Enzymatic Colorimetric—Kinetic Jaffé method. Those were used as a reference value to determine urine concentrations and to evaluate neurotransmitter levels relative to creatinine levels. The applied neurotransmitter screening method is usually utilised in human patients. In previous canine studies, the method was used multiple times and the archived data revealed biologically reasonable results for this species as well (53, 67).
Statistical Analysis
Statistical analyses were performed with the R software (v4.0.3) to test the hypothesis, that there is a difference between the urinary neurotransmitter excretion of dogs affected by epilepsy and a healthy control group (H1) (73). Additionally, whether the urinary neurotransmitter excretion of dogs with epilepsy is affected by ASD administration was explored by comparing neurotransmitter levels of ASD- treated and untreated dogs with epilepsy (H2). First, data were log10-transformed to compensate for wide ranges. Then, the transformed data were tested against the hypothesis of normal distribution using Shapiro–Wilk's test. Finally, in the case of normally distributed data, group comparisons were analysed with an unpaired two-sided two-sample t-test. When data did not follow a normal distribution, the Wilcoxon-Mann-Whitney test was used in the analysis. Next, multiple group comparisons were analysed with a one-way analysis of variance (ANOVA) to find between-factor differences. Finally, a Games-Howell post-hoc test with the Holm correction for multiple comparisons was used to analyse multiple group contrasts and compensate for potential heteroscedasticity. If multiple group data did not follow a normal distribution, the Kruskal-Wallis test was used. Results were considered significant at the following p-value thresholds: p ≤ 0.05 (*), p ≤ 0.01 (**), p ≤ 0.001(***), and p ≤ 0.0001 (****).
Results
Study Population
For the current study, 223 urine samples from 190 dogs of more than 21 breeds were collected, including the following: Australian Shepherd (n = 5), Beagle (n = 6), Belgian Shepherd (n =2 ), Bernese Mountain Dog (n = 1), Border Collie (n = 4), Chihuahua (n = 2), Dachshund (n = 3), Boxer (n = 1), German Shepherd (n = 4), French Bulldog (n = 1), Golden Retriever (n = 2), Havanese (n = 1), Jack Russell Terrier (n = 1), Labrador (n = 2), Vizsla (n = 3), Maltese (n = 1), Poodle (n = 2), Rhodesian Ridgeback (n = 1), Siberian Husky (n = 2), cross breeds (n = 46) and other breeds (n = 43). For n = 57 dogs, no information of their breed was available. The study population consisted of n = 89 males, of which n = 32 were intact, n = 57 were neutered, and n = 98 females, of which n = 37 were intact and n = 61 were neutered. For three dogs, the gender status is not available. The dogs had a mean age of 5.31 (±SD 3.41) years and weighed a mean of 20.08 (±SD 12.25) kg. Of the n = 63 dogs with IE, n = 42 were treated with phenobarbital (66.67%) and n = 27 were treated with potassium bromide (42.86%), of which 26 received potassium bromide additional to the administered phenobarbital, and one dog was solely treated with potassium bromide. Forty-four (n = 44) dogs in the IE cohort received additional ASD treatment in addition to or instead of the aforementioned ASDs (69.84%): levetiracetam (n = 16 chronically; n = 3 pulse therapy) (74), imepitoin (n = 6), Gabapentin (n = 2), rectal diazepam rescue therapy (n = 7), MCT oil (n = 36), cannabidiol oil (n = 2), coconut oil (n = 1). Seven (n = 7) dogs with IE did not receive ASD treatment at the time of sample acquisition and for n = 6 dogs no treatment data were available. Thirty-three (n = 33) (52.38%) of the affected dogs had at least three generalised seizures in the past 3 months before study enrolment. They were chronically treated with at least one ASD without improving seizure frequency. Seven (n = 7) dogs (11.11%) of the IE cohort were seizure free during the past 3 months before sample collection. For n = 23 dogs, the seizure frequency was not accessible.
Neurotransmitter Analysis
A significant difference between urinary neurotransmitter levels of dogs diagnosed with IE and the control group was revealed, when sex and neutering status were accounted for (Figures 1, 2). Urinary glycine (p ≤ 0.05, Figure 1A) and serotonin concentration (p ≤ 0.001, Figure 1B) were significantly increased in dogs with IE. Whereas, the NE/E ratio (p ≤ 0.05, Figure 1C) and the GABA/glutamate ratio (p ≤ 0.01, Figure 1D) was significantly decreased in dogs with epilepsy. The sex and neutering status of the dogs substantially affected the urinary neurotransmitter excretion (Figure 2). Glycine concentration was significantly increased in neutered females with epilepsy (p ≤ 0.05, Figure 2A). The serotonin concentration was significantly increased in intact males (p ≤ 0.01), neutered males (p ≤ 0.001) and neutered females (p ≤ 0.001) with epilepsy (Figure 2B). The NE/E ratio was significantly decreased in neutered females (p ≤ 0.05, Figure 2C) with epilepsy. The GABA/glutamate ratio was significantly reduced in intact males (p ≤ 0.05) and neutered females (p ≤ 0.05) with epilepsy (Figure 2D). Finally, an influence of ASD treatment on urinary neurotransmitter excretion was observed in dogs with epilepsy (Figure 3). Treatment significantly increased serotonin concentration in dogs with epilepsy compared to untreated dogs (p ≤ 0.05, Figure 3A), to an even higher level as in healthy controls (p Holm-corrected = 1.02e-12, Figure 3B). GABA concentration was significantly decreased in untreated dogs with epilepsy compared to those treated dogs with IE (p ≤ 0.05, Figure 3C). The ASD treatment increased GABA concentration and increased it to a similar level to healthy control dogs (p Holm-corrected = 0.607, Figure 3D). For the remaining urinary neurotransmitters (histamine, PEA, DA, E, NE, glutamate) no statistically significant differences between the two cohorts or an ASD treatment effect were identified (Supplementary Table 1).
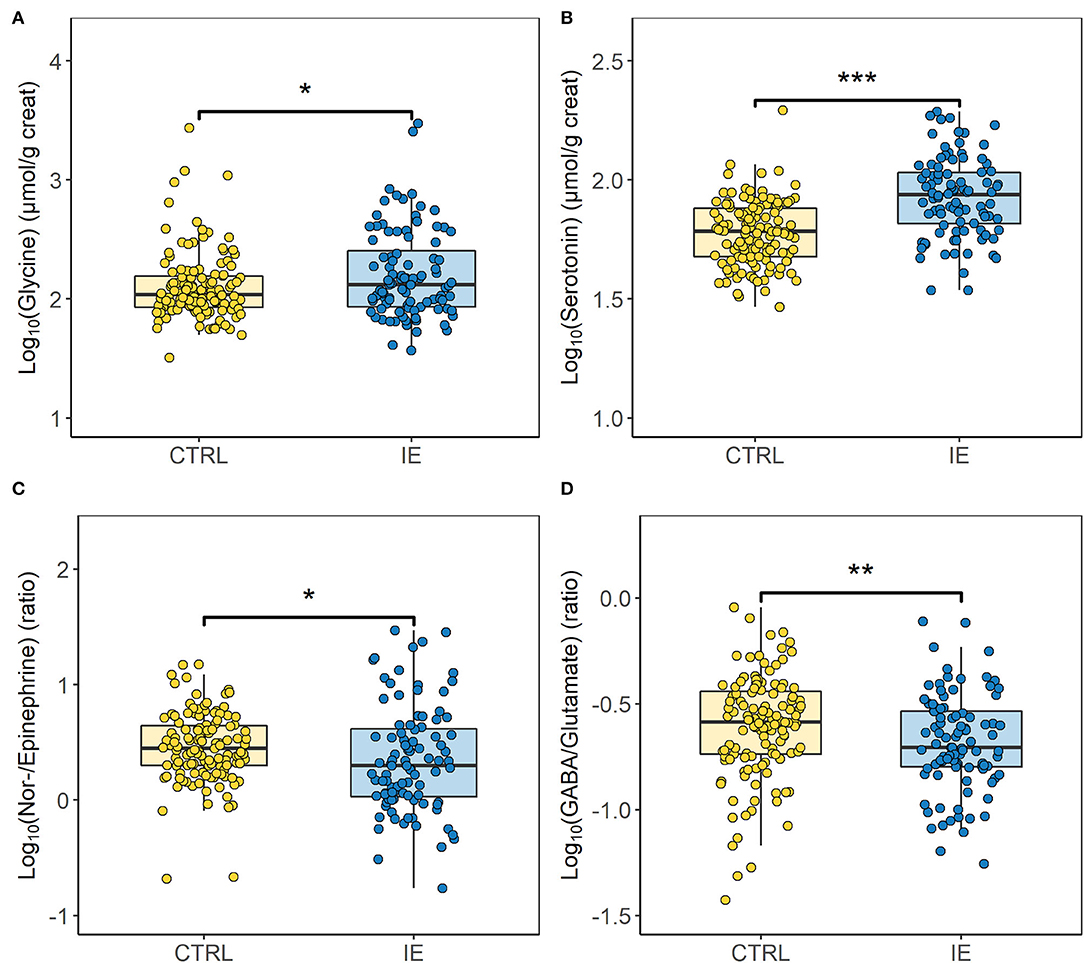
Figure 1. Differences in the urinary neurotransmitter profile between dogs with idiopathic epilepsy (IE) (n = 63 dogs/96 samples) and the healthy control group (CTRL) (n = 127 dogs/127 samples). Figure shows an increase of urinary (A) glycine (p ≤ 0.05) and (B) serotonin (p ≤ 0.001) concentrations and a decrease of the (C) norepinephrine/epinephrine ratio (p ≤ 0.05) and the (D) ɤ-aminobutyric acid (GABA)/glutamate ratio (p ≤ 0.01) in dogs affected by idiopathic epilepsy. Data are presented as box-and-whisker plots (the median is represented by the central line, the 25th and the 75th percentile represent the lower and upper limit, and the length of the whiskers represent the 1.5 multiple of the interquartile range). Unpaired two-sided two-sample t-test and Wilcoxon–Mann–Whitney test were used to compare the urinary neurotransmitter excretion between dogs with epilepsy and the healthy control group. *p ≤ 0.05, **p ≤ 0.01 and ***p ≤ 0.001.
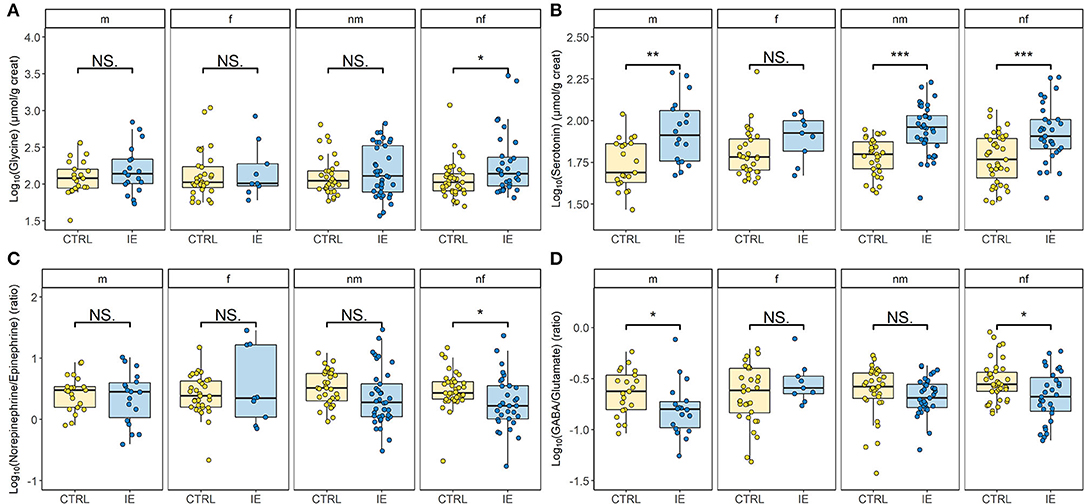
Figure 2. Effects of the sex and neutering status on the urinary neurotransmitter profile of dogs with idiopathic epilepsy (IE) compared to the healthy control group (CTRL). (A) Glycine levels differ significantly in neutered females (nf) (p ≤ 0.05) (IE: n = 22 dogs/31 samples vs. CTRL: n = 39 dogs/39 samples). (B) Serotonin levels differ significantly in intact males (m) (p ≤ 0.01) (IE: n = 11 dogs/18 samples vs. CTRL n = 21 dogs/21 samples), neutered males (nm) (p ≤ 0.001) (IE: n = 24 dogs/37 samples vs. CTRL n = 33 dogs/33 samples) and neutered females (p ≤ 0.001) (IE: n = 22 dogs/31 samples vs. CTRL n = 39 dogs/39 samples). (C) The norepinephrine/epinephrine ratio differs significantly in neutered females (p ≤ 0.05) (IE: n = 22 dogs/31 samples vs. CTRL n = 39 dogs/39 samples). (D) The ɤ-aminobutyric acid (GABA)/glutamate ratio differs significantly in intact males (p ≤ 0.05) (IE: n = 11 dogs/18 samples vs. CTRL n = 21 dogs/21 samples) and neutered females (p ≤ 0.05) (IE: n = 22 dogs/31 samples vs. CTRL n = 39 dogs/39 samples). In intact females (f) no significant difference (NS.) of all analysed neurotransmitter between the groups was found (IE: n = 5 dogs/9 samples vs. CTRL n = 21 dogs/21 samples). Data are presented as box-and-whisker plots (the median is represented by the central line, the 25th and the 75th percentile represent the lower and upper limit, and the length of the whiskers represent the 1.5 multiple of the interquartile range). Unpaired two-sided two-sample t-test and Wilcoxon–Mann–Whitney test were used to compare the urinary neurotransmitter excretion between dogs with epilepsy and the healthy control group. *p ≤ 0.05, **p ≤ 0.01 and ***p ≤ 0.001.
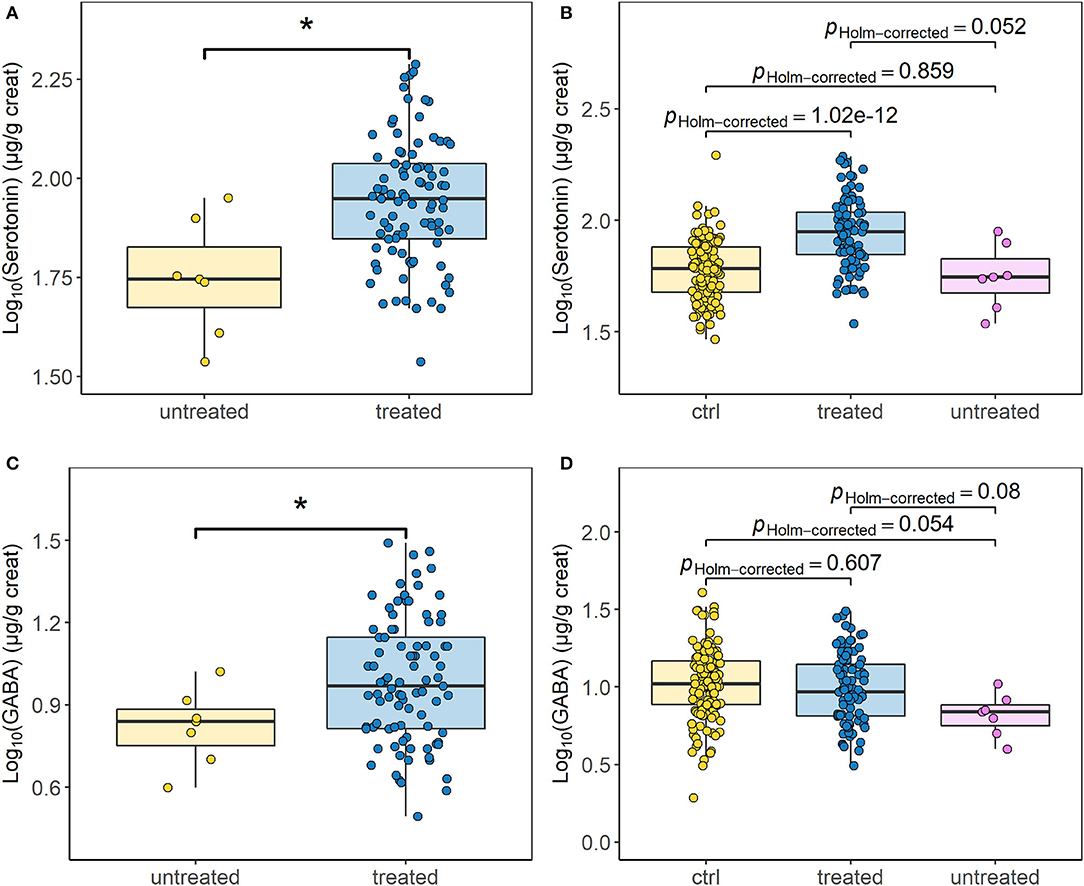
Figure 3. Influence of the antiseizure drug treatment on the urinary neurotransmitter profile. (A) Urinary serotonin concentration compared between treated (n = 56 dogs/89 samples) and untreated dogs (n = 7 dogs/7 samples) affected by idiopathic epilepsy (p ≤ 0.05). (B) Multiple group comparison of the urinary serotonin excretion between treated (n = 56 dogs/89 samples) and untreated dogs (n = 7 dogs/7 samples) with idiopathic epilepsy and the healthy control group (ctrl) (n = 127 dogs/127 samples). (C) Urinary ɤ-aminobutyric acid (GABA) concentration compared between treated (n = 56 dogs/89 samples) and untreated dogs (n = 7 dogs/7 samples) with idiopathic epilepsy (p ≤ 0.05). (D) Multiple comparisons of the urinary ɤ-aminobutyric acid excretion between treated (n = 56 dogs/89 samples) and untreated dogs (n = 7 dogs/7 samples) with idiopathic epilepsy and the healthy control group (n = 127 dogs/127 samples). Data are presented as box-and-whisker plots (the median is represented by the central line, the 25th and the 75th percentile represent the lower and upper limit, and the length of the whiskers represent the 1.5 multiple of the interquartile range). Unpaired two-sided two-sample t-test and Wilcoxon–Mann–Whitney test were used for group comparison. For multiple group comparisons a one-way analysis of variance (ANOVA) and a Games-Howell post-hoc test with the Holm correction was utilised. *p ≤ 0.05.
Discussion
The objective of this study was to evaluate the suitability of urinary neurotransmitter analysis as a non-invasive diagnostic tool, where characteristic neurotransmitter deviations serve as valuable biomarkers for canine IE. It was hypothesised that urinary neurotransmitter profiles differ between dogs with epilepsy and healthy controls (H1). Sex and neutering status substantially affected urinary neurotransmitter excretion. In the present study, urinary neurotransmitter patterns were significantly altered in dogs with IE, when sex and neutering status were accounted for, which confirmed the first hypothesis of our study. Urinary glycine and serotonin concentration were significantly increased in dogs with IE, whereas the GABA/glutamate ratio and the NE/E ratio was significantly decreased. Additionally, it was hypothesised that the urinary neurotransmitter excretion of dogs with epilepsy was affected by administered ASD, with hypothesised differences between ASD-treated dogs compared to untreated dogs with epilepsy (H2). Results demonstrated that ASD treatment increased GABA concentration in dogs with epilepsy to the level seen in the healthy control population.
Glycine serves primarily as an inhibitory neurotransmitter in the CNS (75). It generally improves mood, mental performance and memory skills (76, 77). However, elevated levels can compromise cognitive processing and provoke seizures (78, 79). In humans, a rare inherited error of glycine metabolism, called non-ketotic hyperglycinemia, causes an excessive accumulation of this neurotransmitter in the body, particularly in the nervous system (80). Clinical signs of this disease include refractory seizures, hyperactivity and in adults cognitive impairment (79, 80). Affected patients also excrete high levels of glycine in their urine (80). Non-ketotic hyperglycinemia and epilepsy are two different diseases, however, parallels in clinical signs exist. The most prominent clinical sign of human and canine epilepsy are recurrent seizures. Cognitive impairments and hyperactivity are often associated as well (81–87). The elevated urinary glycine levels in dogs with IE found in the current study are another similarity. The results indicating, increased glycine concentration might be a contributing factor inducing seizures and associated cognitive impairment, as well as hyperactivity in affected patients. However, elevated urinary glycine in dogs with epilepsy found in this study, should be differentiated from the massively increased concentrations in human patients with non-ketotic hyperglycinemia. Further studies are needed to evaluate whether urinary glycine can serve as a potential biomarker in canine epilepsy, too. It must be considered, that for laboratory diagnosis of non-ketotic hyperglycinemia CSF and serum glycine concentrations are determined. In a previous study canine glycine levels correlated between CSF, serum and urine, suggesting non-invasive urinary neurotransmitter analysis as a good option for glycine screening in dogs (53). A treatment effect of the ASDs (phenobarbital and potassium bromide), which might have caused the detected glycine increase, was not revealed in this study. For valproate, an anticonvulsive drug administered in human medicine, an elevating effect on urine and plasma glycine levels exists (88). To the authors' knowledge, such an effect is not known from first and second-line drugs (phenobarbital and potassium bromide) authorised for canine epilepsy treatment.
Urinary serotonin levels were increased in dogs with IE, compared to dogs without epilepsy and were substantially affected by their sex and neutering status. These findings match those of a recent study in which urinary serotonin excretion was altered after ovariohysterectomy in bitches (89). Serotonin plays a role in regulating sleep, appetite and mood (36). Grouping the data into treated and untreated epileptic dogs revealed that untreated dogs with epilepsy excreted significantly lower urinary serotonin levels than ASD-treated dogs with epilepsy or healthy control dogs. Decreased serotonin concentrations are related to the pathogenesis of various psychiatric and neurological disorders (41). Alterations in the serotonergic system can lower the seizure threshold and are associated with frequently co-occurring neurobehavioural comorbidities (35, 37). Psychological conditions/behavioural abnormalities are commonly treated with selective serotonin reuptake inhibitors (SSRIs) in humans and dogs (90, 91). However, drug manuals suggest that SSRIs are contraindicated in dogs with epilepsy or a history of seizures (92). In contrast, the International League Against Epilepsy and experimental data suggest SSRIs to be of low risk to patients with a history of seizures or epilepsy, indicating that they can be cautiously used for the treatment of anxiety in some epilepsy patients (93). Some experimental data even exists that SSRIs might be anticonvulsive. For example, the SSRI fluoxetine is effective in dogs with fly catching syndrome, a condition which has been considered by some as limbic epilepsy, but others as a compulsive behavioural disorder (94). In the current study, ASD treatment significantly increased the serotonin concentration in dogs with epilepsy compared to untreated dogs, to an even higher level than in healthy controls dogs. Elevated serotonin levels can be an amplifying and beneficial effect of ASD treatment, due to the protective properties of serotonin against seizures. Increased serotonin concentration may also improve associated neurobehavioural disorders in affected dogs, without prescribing contraindicated SSRIs, however, further studies are required to explore this potential positive effect.
The current study also revealed a diminished GABA/glutamate ratio in dogs with IE, which reflects low GABA levels or high glutamate levels in the examined urine samples, respectively. Both neurotransmitters are amino acids with contradictory effects on the body. GABA acts as the primary inhibitory neurotransmitter, while glutamate is the major excitatory counterpart in the CNS (33, 34). These findings in the urine potentially mirror neurotransmitter alterations in the epileptic brain. Furthermore, low urinary GABA concentrations in drug naïve dogs, compared to treated dogs with IE and healthy controls were shown. Dogs with IE who received ASD treatment excreted a higher urinary GABA concentration, which was almost at the same level as healthy controls. These findings reflect the expected lower GABA concentration in untreated epilepsy and corroborate a treatment effect, which may have corrected the GABA values up to the healthy controls state. However, the ASD administered in this study (phenobarbital and potassium bromide) are not known to directly influence GABA concentrations. Their anticonvulsant effect is mediated by other action mechanisms, including GABA receptor interactions (95, 96). Ultimately, however, acute and chronic phenobarbital treatment reduce brain GABA levels (97). Why urinary GABA levels behave differently requires further research.
The NE/E ratio was found to be decreased in dogs affected by IE, representing low NE levels or high E levels in the examined urine samples, respectively. These monoamine neurotransmitters are catecholamines and act receptor-binding-dependent either as excitatory or inhibitory stimulants in the CNS (98, 99). NE is known for its anticonvulsant properties in epilepsy, even though it can also be proconvulsive under certain circumstances (13, 100–102). Reduced NE levels of dogs with IE compared to healthy controls in the presented study corroborate the generally anticonvulsive effect of this neurotransmitter. The lack of NE might contribute to epileptogenesis and induction of seizures in the examined dogs. Furthermore, NE affects cognition, attention and memory ability (103). The noradrenergic system changes cause various neuropsychiatric and -degenerative disorders, such as Alzheimer's disease and ADHD in humans (103–105). As aforementioned, canine epilepsy can be associated with cognitive impairments (72, 82–84). The low NE concentrations detected in this study might contribute to the development of those impairments. These findings consistent with those of decreased NE levels assessed in the brain of human patients with Alzheimer's disease, which were correlated with the degree of cognitive impairment (106). Another comorbidity of human epilepsy is ADHD, with behavioural similarities also documented in canine epilepsy patients (86, 87). Previous research has indicated that ADHD can be associated with imbalances in the noradrenergic and dopaminergic systems (105, 107). Several studies reported a correlation between ADHD in children and altered urinary catecholamine excretion (56, 63, 108). Pliszka et al. detected elevated urinary excretion of NE metabolites in children with ADHD compared to healthy controls and increased urinary E excretion when ADHD was accompanied by anxiety (62). Anxiety disorders are also common in human and canine epilepsy (39, 109). A relationship between increased anxiety and exaggerated stress response of the neuroendocrine system haven been previously described (60, 110). E regulates many important body functions and is substantially involved in stress response (99). Elevated E concentrations in dogs with IE may have caused the identified deviation in the urinary NE/E ratio and may also be responsible for the co-occurring anxiety in canine epilepsy. Finally, sleep disturbances are often associated with epilepsy in humans (111). They are assumed to occur in dogs as well, although evaluation remains difficult (112). In former studies, poor sleep quality and disordered sleep were linked to a profuse activation of the sympathetic nervous system, resulting in increased nocturnal serum catecholamine levels (113, 114). As a comorbidity of epilepsy, altered sleeping patterns might also have existed in the canine participants of this study. This may have caused elevated nocturnal E levels, which were excreted and detected in the analysed morning urine. Overall, the evidence presented indicates that alterations in the NE/E ratio of dogs with IE in the current study may be associated with the development of seizures and common comorbidities, such as sleep disturbances, ADHD- and anxiety-like behaviour. The results of this study suggest a potential role of the nor-/adrenergic pathway alterations in canine epilepsy and neurobehavioural comorbidities.
A few limitations of the present study should be noted. First, the multicentred sample acquisition enabled a large sample size of urine from participants with IE and healthy controls, however, variability in sample collection and storage may have impacted results. The number of untreated dogs with IE concerning the total study population of dogs with epilepsy was small, therefore, caution must be applied, as findings referring to this population might not be representative. Another limitation of this study is that a direct correlation between CNS and urinary neurotransmitters levels has only been shown to a limited extent in previous research. Finally, despite us finding differences, these might not be clinically discriminatory and only be considered as a monitoring tool. Future studies are needed to identify for which patient these changes are clinically relevant.
Numerous factors can influence the eliminated urinary neurotransmitter concentrations. Neurotransmitter passage from the CNS to the periphery is regulated by the BBB, being formed by specific endothelial cells, through which the transport differs for each substrate (48). For glycine a non-carrier-mediated process for BBB crossing is assumed in rats, whereas for dogs no significant transfer through the BBB could be shown so far (115, 116). Serotonin is shuttled via a serotonin transporter, which enables a bidirectional permeation through the luminal membrane of the endothelial cells, but only unidirectional transport to the brain on the abluminal side (117, 118). GABA can cross the abluminal endothelial membrane through a transport system and a luminal membrane passage is presumed as well, even though the transporter has not yet been identified (45, 119). Glutamate can also pass the BBB across the abluminal side via several transporters into the endothelial cells, from where a bidirectional luminal transport is possible (44, 120). NE is shuttled via an abluminal transporter out of the brain into the endothelial cells (117, 121). E is proven to be assimilated into endothelial cells, however the exact process remains elusive (122). BBB function might be altered during seizure and the neurotransmitter could pass more readily. The presented evidence emphasises that the neurotransmitter transfer through the BBB is still not completely revealed. In the interpretation of the current study results, substrate-specific permeability and transport directions of the respective neurotransmitters through the BBB endothelial cells, should be considered.
After crossing the BBB, the neurotransmitters circulate in the bloodstream, from which they are subsequently eliminated by the kidneys into the urine (48). Renal excretion of monoamine neurotransmitters is affected by two mechanisms: glomerular ultrafiltration from the arterial blood and active reabsorption and secretion through specific transporters (48, 52, 123, 124). All participating dogs had normal renal function and were not affected by renal diseases. Nevertheless, the above-mentioned processes can modulate the detected amount of urinary excreted neurotransmitters in healthy dogs as well and this might have affected the acquired results of this study.
Another impact on urinary neurotransmitter levels could arise from additional synthesis outside the CNS. Neurotransmitters are also produced in the peripheral nervous system (PNS), as in serotonin secreting enterochromaffin cells of the enteric nervous system or in norepinephrine producing renal nerves (125–127). Even bacteria, hosted in the body as microbiota, are capable of synthesising neuroactive molecules by themselves (e.g., GABA), or regulating their host's neurotransmitter metabolism (e.g., serotonin), resulting in a modified overall neurotransmitter pool (128–131). Moreover, neurotransmitters are additively produced in many other body organs beside the nervous system, such as the pancreas (e.g., GABA), the adrenal glands (e.g., NE, E, DA) and the kidneys (e.g., GABA, E, NE) (132–137). Ingesting nutritional sources of neurotransmitters or their precursors, can have an ancillary influence on the neurotransmitter pool of the body (128, 138, 139). To minimise this external impact, dairy products, fruits and vegetables were not fed before sample acquisition. However, the dog's personal standard diet, containing meat and seafood as neurotransmitter sources, might also have influenced their urinary neurotransmitter concentration on an individual level (128). It is assumed, that animals and processed foods contain more stable levels of neurotransmitters, than the avoided plants, which might have reduced the individual variety (128). Moreover, endogenous mechanisms of the body, as inactivating enzymes, intestinal metabolism and certain barriers, limiting the effect of nutritional neurotransmitter and as well their urinary excretion (128). Anyhow, nutrition is an important factor for the neurotransmitter metabolism of the body. In further studies it needs to be addresses to which extent dietary factors influence the canine urinary neurotransmitter excretion.
Although the mentioned factors influence on the urinary neurotransmitter concentration, former studies revealed an association between central and peripheral neurotransmitter excretion into the urine. In early studies labelled NE was injected into the cisterna magna of dogs, followed by detection in their blood and fast metabolite excretion via the urine (140). Following these findings, a more recent study showed positively correlating neurotransmitter concentrations of serine, glycine and NE mirrored in three canine body fluids: CSF, blood and urine (53). Orally administered serotonin substrates in rats enhanced the serotonergic activity in the CNS and urinary serotonin levels, indicating a shared regulation mechanism (49). In another study injecting a neurotoxic compound into rat brains induced diminished DA levels in their brain and urine (50). Furthermore, a relationship between urinary excreted neurotransmitters and psychological disorders in humans has been identified. Elevated concentrations of urinary catecholamines, such as NE and E were associated with depression and anxiety (58–60). Urinary excreted DA correlated with suicide attempts in depressed patients even stronger than the CSF levels (61). In addition to the presented evidence, a crosstalk between the CNS and PNS was demonstrated in different studies, further strengthening the central and peripheral neurotransmitter association (141–143).
In human medicine rare inherited disorders exist, which are causing seizures and are also associated with neurotransmitter alterations in the body (144). Disorders of the pyridoxine metabolism evoke ASD resistant seizures in neonates, responding to administered pyridoxine (pyridoxine dependency) or in rare cases solely to its active form, pyridoxal phosphate (pyridoxal phosphate dependency) (144–146). Pyridoxal phosphate is involved in neurotransmitters metabolism of glutamate, GABA and glycine, but the contribution to the epilepsy remains controversial (146–148). Cerebral folate deficiency manifests with late infantile onset seizures and is treatable with folate acid supplementation (149). It is caused by folate transport or metabolism disorders, resulting in low CNS folate concentrations, which can be accompanied with a peripheral folate deficiency (149, 150). Folate is required in the neurotransmitter metabolism of glycine and its influence on serotonin and catecholamine homeostasis is discussed (144, 151, 152). Further inherited neurotransmitter disorders associated with seizures are succinic semialdehyde dehydrogenase (SSADH) deficiency (GABA metabolism disorder), aromatic L-amino acid decarboxylase (AADC) deficiency (dopamine/serotonin synthesising enzyme disorder, seizures are described but uncommon) and the aforementioned non-ketotic hyperglycinemia (glycine metabolism disorder) (80, 153–156). These disorders are not known in dogs so far. However, in canine IE the underlying cause of seizures remains unknown, which might generate a heterogenous group with different yet undiscovered diseases (1). The dogs of the current study met all requirements of the IVETF for the diagnosis of IE (Tier I/Tier II), but the mentioned human disorders require specific diagnostic screening and are not included in these clinical work-up guidelines (68). It is possible that similar inherited metabolic disorders exist in canine patients undetected, evoking seizures and altered neurotransmitter concentration. Nevertheless, in the mentioned human diseases additional abnormalities in the neurological examination/brain imaging are present, which are exclusion criteria of canine IE (Tier I/Tier II) and are therefore considered unlikely (144, 146, 150, 155). The exact underlying pathophysiology of seizures, and the associated urinary neurotransmitter alterations revealed in this study, remains to be elucidated further in the future. The current study can only be seen as a starting point.
Imbalances in the neurotransmitter system that cause epileptic seizures also lead to altered neurotransmitter elimination in the urine of affected dogs and, therefore, can serve as valuable biomarkers in epilepsy. Urinary neurotransmitter analysis with its non-invasive collection technique offers a major advantage over determining neurotransmitters from other body fluids (e.g., CSF, serum). Recent evidence revealed an association between urinary neurotransmitter patterns and treatment efficacy in drug-resistant dogs with IE, suggesting a benefit of utilising this diagnostic tool, particularly in epilepsy patients (67). In the future, neurotransmitter analysis could allow for a better understanding of the underlying pathomechanisms of epilepsy. These biomarkers may indicate specific subtypes of epilepsy in this heterogeneous disease, associated with pharmacoresistance. Applied in a clinical setting, the non-invasive urinary neurotransmitter analysis could be used for individual treatment monitoring and customised adjustments of therapeutic interventions in canine or even human epilepsy.
Conferences
Preliminary results of the current study were presented at following conferences: 33rd ESVN -ECVN Symposium 2021; 30. Jahrestagung der Fachgruppe “Innere Medizin und klinische Labordiagnostik” der DVG (InnLab) 2022 (awarded with the 1st poster prize).
Data Availability Statement
The datasets used and/or analysed within the current study are available as Supplementary Material, further inquiries can be directed to the corresponding author.
Ethics Statement
Written informed consent was obtained from all owners. The study was conducted following the guidelines of the University of Veterinary Medicine Hannover and approved by the thesis committee of the University. In addition, data and urine samples from multiple epilepsy studies were used. These studies were approved by the local Ethics and Welfare Group (EWG) (URN 2011 1132, URN 2016 1558 and URN 2017 1743-2).
Author Contributions
TS participated in the planning of the study, carried out the main practical work, the recruitment and the sample acquisition of the control group samples in Hannover, interpreted the results, and drafted the manuscript. BB, TL, SH, RP, and HV provided clinical and laboratory data of the dogs with idiopathic epilepsy of former epilepsy trials and studies. HV designed and coordinated the study. SM supported sample acquisition. SM and HV made essential contributions to the conception and acquisition of data. ST performed the statistical analysis and wrote sections of the manuscript. ST, BB, SH, NM, RP, and HV critically reviewed and edited the manuscript for important intellectual content. All authors contributed to the manuscript revision, read and approved the final manuscript.
Funding
This work was financially supported by the Biotechnology and Biological Sciences Research Council (BBSRC, grant code BB/P001874/1). This Open Access publication was funded by the Deutsche Forschungsgemeinschaft (DFG, German Research Foundation) within the programme LE 824/10-1 “Open Access Publication Costs” and University of Veterinary Medicine Hannover, Foundation.
Conflict of Interest
BB was employed by company BrainCheck.Pet®.
The remaining authors declare that the research was conducted in the absence of any commercial or financial relationships that could be construed as a potential conflict of interest.
Publisher's Note
All claims expressed in this article are solely those of the authors and do not necessarily represent those of their affiliated organizations, or those of the publisher, the editors and the reviewers. Any product that may be evaluated in this article, or claim that may be made by its manufacturer, is not guaranteed or endorsed by the publisher.
Acknowledgments
The authors thank the Biotechnology and Biological Sciences Research Council [BBSRC, (www.bbsrc.ac.uk), grant code BB/P001874/1] for their financial support to HV, RP, and TL. Further they would like to thank the University of Helsinki for providing the urinary neurotransmitter analysis data of their epilepsy trials. The authors also thank Doctor's data for the sample analysis. Finally, special thanks go to the participation of the dog owners and the dogs providing the canine urine samples for this study.
Supplementary Material
The Supplementary Material for this article can be found online at: https://www.frontiersin.org/articles/10.3389/fvets.2022.893013/full#supplementary-material
Abbreviations
GABA, ɤ-aminobutyric acid; CSF, cerebrospinal fluid; DA, dopamine; CNS, central nervous system; BBB, blood-brain barrier; NE, norepinephrine; ADHD, attention-deficit hyperactivity disorder; E, epinephrine; PEA, phenylethylamine; MCT, medium-chained triglyceride; IE, idiopathic epilepsy; IVETF, International Veterinary Epilepsy Task Force; ASD, antiseizure drug; RVC, Royal Veterinary College; TiHo, University of Veterinary Medicine Hannover; HPLC-QqQ MS/MS, High-performance liquid chromatography triple-quadrupole mass spectrometry/mass spectrometry; ANOVA, analysis of variance; SSRIs: selective serotonin reuptake inhibitors, ; peripheral nervous system, ; SSADH, succinic semialdehyde dehydrogenase, AADC, aromatic L-amino acid decarboxylase.
References
1. Berendt M, Farquhar RG, Mandigers PJ, Pakozdy A, Bhatti SF, De Risio L, et al. International veterinary epilepsy task force consensus report on epilepsy definition, classification and terminology in companion animals. BMC Vet Res. (2015) 11:182. doi: 10.1186/s12917-015-0461-2
2. Fisher RS, Acevedo C, Arzimanoglou A, Bogacz A, Cross JH, Elger CE, et al. Ilae official report: a practical clinical definition of epilepsy. Epilepsia. (2014) 55:475–82. doi: 10.1111/epi.12550
3. Patterson EE. Canine epilepsy: an underutilized model. Ilar J. (2014) 55:182–6. doi: 10.1093/ilar/ilu021
4. Kearsley-Fleet L, O'Neill DG, Volk HA, Church DB, Brodbelt DC. Prevalence and risk factors for canine epilepsy of unknown origin in the UK. Vet Rec. (2013) 172:338. doi: 10.1136/vr.101133
5. Sirven JI. Epilepsy: a spectrum disorder. Cold Spring Harb Perspect Med. (2015) 5:a022848. doi: 10.1101/cshperspect.a022848
6. Licht BG, Licht MH, Harper KM, Lin S, Curtin JJ, Hyson LL, et al. Clinical presentations of naturally occurring canine seizures: similarities to human seizures. Epilepsy Behav. (2002) 3:460–70. doi: 10.1016/S1525-5050(02)00523-1
7. Packer RMA, Shihab NK, Torres BB, Volk HA. Responses to successive anti-epileptic drugs in canine idiopathic epilepsy. Vet Rec. (2015) 176:203. doi: 10.1136/vr.102934
8. Kwan P, Brodie MJ. Early identification of refractory epilepsy. N Engl J Med. (2000) 342:314–9. doi: 10.1056/NEJM200002033420503
9. Berendt M, Gredal H, Ersboll AK, Alving J. Premature death, risk factors, and life patterns in dogs with epilepsy. J Vet Intern Med. (2007) 21:754–9. doi: 10.1111/j.1939-1676.2007.tb03017.x
10. Laxer KD, Trinka E, Hirsch LJ, Cendes F, Langfitt J, Delanty N, et al. The consequences of refractory epilepsy and its treatment. Epilepsy Behav. (2014) 37:59–70. doi: 10.1016/j.yebeh.2014.05.031
11. Fisher RS, Boas WvE, Blume W, Elger C, Genton P, Lee P, et al. Epileptic seizures and epilepsy: definitions proposed by the international league against epilepsy (ilae) and the international bureau for epilepsy (Ibe). Epilepsia. (2005) 46:470–2. doi: 10.1111/j.0013-9580.2005.66104.x
12. Flynn S, Babi MA. 12 - Anticonvulsants. In: Dowd FJ, Johnson BS, Mariotti AJ, editors. Pharmacology and Therapeutics for Dentistry (Seventh Edition). St. Louis: Mosby (2017). p. 176–92.
13. Akyuz E, Polat AK, Eroglu E, Kullu I, Angelopoulou E, Paudel YN. Revisiting the role of neurotransmitters in epilepsy: an updated review. Life Sci. (2021) 265:118826. doi: 10.1016/j.lfs.2020.118826
14. Lloyd KG, Scatton B, Voltz C, Bryere P, Valin A, Naquet R. Cerebrospinal fluid amino acid and monoamine metabolite levels of Papio Papio: correlation with photosensitivity. Brain Res. (1986) 363:390–4. doi: 10.1016/0006-8993(86)91030-9
15. Cavus I, Pan JW, Hetherington HP, Abi-Saab W, Zaveri HP, Vives KP, et al. Decreased hippocampal volume on MRI is associated with increased extracellular glutamate in epilepsy patients. Epilepsia. (2008) 49:1358–66. doi: 10.1111/j.1528-1167.2008.01603.x
16. During MJ, Spencer DD. Extracellular hippocampal glutamate and spontaneous seizure in the conscious human brain. Lancet. (1993) 341:1607–10. doi: 10.1016/0140-6736(93)90754-5
17. Murugesan A, Rani MRS, Hampson J, Zonjy B, Lacuey N, Faingold CL, et al. Serum serotonin levels in patients with epileptic seizures. Epilepsia. (2018) 59:e91–7. doi: 10.1111/epi.14198
18. Mercimek-Mahmutoglu S, Sidky S, Hyland K, Patel J, Donner EJ, Logan W, et al. Prevalence of inherited neurotransmitter disorders in patients with movement disorders and epilepsy: a retrospective cohort study. Orphanet J Rare Dis. (2015) 10:12. doi: 10.1186/s13023-015-0234-9
19. Olsen RW, Wamsley JK, McCabe RT, Lee RJ, Lomax P. Benzodiazepine/Gamma-aminobutyric acid receptor deficit in the midbrain of the seizure-susceptible gerbil. Proc Natl Acad Sci USA. (1985) 82:6701–5. doi: 10.1073/pnas.82.19.6701
20. Horton RW, Prestwich SA, Meldrum BS. Gamma-aminobutyric acid and benzodiazepine binding sites in audiogenic seizure-susceptible mice. J Neurochem. (1982) 39:864–70. doi: 10.1111/j.1471-4159.1982.tb07972.x
21. Johnson EW, de Lanerolle NC, Kim JH, Sundaresan S, Spencer DD, Mattson RH, et al. “Central” and “Peripheral” benzodiazepine receptors: opposite changes in human epileptogenic tissue. Neurology. (1992) 42:811–5. doi: 10.1212/wnl.42.4.811
22. Birioukova LM, Sitnikova EY, Kulikov MA, Raevsky VV. Compensatory changes in the brain dopaminergic system of Wag/Rij rats genetically predisposed to absence epilepsy. Bull Exp Biol Med. (2016) 161:662–5. doi: 10.1007/s10517-016-3480-5
23. McDonald JW, Garofalo EA, Hood T, Sackellares JC, Gilman S, McKeever PE, et al. Altered excitatory and inhibitory amino acid receptor binding in hippocampus of patients with temporal lobe epilepsy. Ann Neurol. (1991) 29:529–41. doi: 10.1002/ana.410290513
24. Toczek MT, Carson RE, Lang L, Ma Y, Spanaki MV, Der MG, et al. Pet imaging of 5-Ht1a receptor binding in patients with temporal lobe epilepsy. Neurology. (2003) 60:749–56. doi: 10.1212/01.WNL.0000049930.93113.20
25. Savic I, Lindström P, Gulyás B, Halldin C, Andrée B, Farde L. Limbic reductions of 5-Ht1a receptor binding in human temporal lobe epilepsy. Neurology. (2004) 62:1343–51. doi: 10.1212/01.WNL.0000123696.98166.AF
26. Merlet I, Ostrowsky K, Costes N, Ryvlin P, Isnard J, Faillenot I, et al. 5-Ht1a receptor binding and intracerebral activity in temporal lobe epilepsy: an [18f]Mppf-pet study. Brain. (2004) 127(Pt 4):900–13. doi: 10.1093/brain/awh109
27. Banerjee J, Banerjee Dixit A, Tripathi M, Sarkar C, Gupta YK, Chandra PS. Enhanced endogenous activation of Nmda receptors in pyramidal neurons of hippocampal tissues from patients with mesial temporal lobe epilepsy: a mechanism of hyper excitation. Epilepsy Res. (2015) 117:11–6. doi: 10.1016/j.eplepsyres.2015.08.007
28. Kang JQ. Defects at the crossroads of Gabaergic signaling in generalized genetic epilepsies. Epilepsy Res. (2017) 137:9–18. doi: 10.1016/j.eplepsyres.2017.08.013
29. Brooks-Kayal AR, Shumate MD, Jin H, Rikhter TY, Coulter DA. Selective changes in single cell Gaba(a) receptor subunit expression and function in temporal lobe epilepsy. Nat Med. (1998) 4:1166–72. doi: 10.1038/2661
30. Crino PB, Duhaime AC, Baltuch G, White R. Differential expression of glutamate and Gaba-a receptor subunit mrna in cortical dysplasia. Neurology. (2001) 56:906–13. doi: 10.1212/WNL.56.7.906
31. Talos DM, Kwiatkowski DJ, Cordero K, Black PM, Jensen FE. Cell-specific alterations of glutamate receptor expression in tuberous sclerosis complex cortical tubers. Ann Neurol. (2008) 63:454–65. doi: 10.1002/ana.21342
32. Sánchez Fernández I, Loddenkemper T. Subunit composition of neurotransmitter receptors in the immature and in the epileptic brain. Biomed Res Int. (2014) 2014:301950. doi: 10.1155/2014/301950
33. Danbolt NC, Furness DN, Zhou Y. Neuronal vs glial glutamate uptake: resolving the conundrum. Neurochem Int. (2016) 98:29–45. doi: 10.1016/j.neuint.2016.05.009
34. Schwartz RD. The Gabaa receptor-gated ion channel: biochemical and pharmacological studies of structure and function. Biochem Pharmacol. (1988) 37:3369–75. doi: 10.1016/0006-2952(88)90684-3
35. Buchanan GF, Murray NM, Hajek MA, Richerson GB. Serotonin neurones have anti-convulsant effects and reduce seizure-induced mortality. J Physiol. (2014) 592:4395–410. doi: 10.1113/jphysiol.2014.277574
36. Jenkins TA, Nguyen JC, Polglaze KE, Bertrand PP. Influence of tryptophan and serotonin on mood and cognition with a possible role of the gut-brain axis. Nutrients. (2016) 8:56. doi: 10.3390/nu8010056
37. Richerson GB, Buchanan GF. The serotonin axis: shared mechanisms in seizures, depression, and sudep. Epilepsia. (2011) 52(Suppl. 1):28–38. doi: 10.1111/j.1528-1167.2010.02908.x
38. Kanner AM. Epilepsy, suicidal behaviour, and depression: do they share common pathogenic mechanisms? Lancet Neurol. (2006) 5:107–8. doi: 10.1016/S1474-4422(06)70331-3
39. Shihab N, Bowen J, Volk HA. Behavioral changes in dogs associated with the development of idiopathic epilepsy. Epilepsy Behav. (2011) 21:160–7. doi: 10.1016/j.yebeh.2011.03.018
40. Jobe PC. Common pathogenic mechanisms between depression and epilepsy: an experimental perspective. Epilepsy Behav. (2003) 4:14–24. doi: 10.1016/j.yebeh.2003.08.020
41. Roth BL, Xia Z. Molecular and cellular mechanisms for the polarized sorting of serotonin receptors: relevance for genesis and treatment of psychosis. Crit Rev Neurobiol. (2004) 16:229–36. doi: 10.1615/CritRevNeurobiol.v16.i4.10
42. Rosado B, García-Belenguer S, León M, Chacón G, Villegas A, Palacio J. Blood concentrations of serotonin, cortisol and dehydroepiandrosterone in aggressive dogs. Applied Anim Behav Sci. (2010) 123:124–30. doi: 10.1016/j.applanim.2010.01.009
43. Amat M, Le Brech S, Camps T, Torrente C, Mariotti VM, Ruiz JL, et al. Differences in serotonin serum concentration between aggressive english cocker spaniels and aggressive dogs of other breeds. J Vet Behav. (2013) 8:19–25. doi: 10.1016/j.jveb.2012.04.003
44. Hawkins RA, O'Kane RL, Simpson IA, Viña JR. Structure of the blood–brain barrier and its role in the transport of amino acids. J Nutr. (2006) 136:218S−26. doi: 10.1093/jn/136.1.218S
45. Kakee A, Takanaga H, Terasaki T, Naito M, Tsuruo T, Sugiyama Y. Efflux of a suppressive neurotransmitter, Gaba, across the blood-brain barrier. J Neurochem. (2001) 79:110–8. doi: 10.1046/j.1471-4159.2001.00540.x
46. Amara SG, Kuhar MJ. Neurotransmitter transporters: recent progress. Annu Rev Neurosci. (1993) 16:73–93. doi: 10.1146/annurev.ne.16.030193.000445
47. Moleman P, Tulen JHM, Blankestijn PJ, Man in 't Veld AJ, Boomsma F. Urinary excretion of catecholamines and their metabolites in relation to circulating catecholamines: six-hour infusion of epinephrine and norepinephrine in healthy volunteers. Arch Gen Psychiatry. (1992) 49:568–72. doi: 10.1001/archpsyc.1992.01820070062009
48. Marc DT, Ailts JW, Campeau DC, Bull MJ, Olson KL. Neurotransmitters excreted in the urine as biomarkers of nervous system activity: validity and clinical applicability. Neurosci Biobehav Rev. (2011) 35:635–44. doi: 10.1016/j.neubiorev.2010.07.007
49. Lynn-Bullock CP, Welshhans K, Pallas SL, Katz PS. The Effect of Oral 5-Htp administration on 5-Htp and 5-Ht immunoreactivity in monoaminergic brain regions of rats. J Chem Neuroanat. (2004) 27:129–38. doi: 10.1016/j.jchemneu.2004.02.003
50. Chekhonin VP, Baklaushev VP, Kogan BM, Savchenko EA, Lebedev SV, Man'kovskaya IV, et al. Catecholamines and their metabolites in the brain and urine of rats with experimental Parkinson's disease. Bull Exp Biol Med. (2000) 130:805–9. doi: 10.1007/BF02766101
51. Matsuda F, Hayashi T, Naruse H. A Study on the origins of urinary serotonin and tryptamine. Tokai J Exp Clin Med. (1991) 16:245–52.
52. Graefe KH, Friedgen B, Wölfel R, Bossle F, Russ H, Schömig E. 1,1'-Diisopropyl-2,4'-cyanine (disprocynium24), a potent uptake2 blocker, inhibits the renal excretion of catecholamines. Naunyn-Schmiedebergs Arch Pharmacol. (1997) 356:115–25. doi: 10.1007/PL00005018
53. Meller S, Hildebrandt R, Gramer M, Richter Assencio F, Volk HA. Comparison of neurotransmitters concentration in canine cerebrospinal fluid, blood, and urine samples measured via highperformance liquid chromatography. In Proceedings of the 33rd On-line Symposium ESVN-ECVN 2021 September 17–18. J Vet Intern Med. (2021) 36:300–52. doi: 10.1111/jvim.16332
54. Delahanty DL, Nugent NR, Christopher NC, Walsh M. Initial urinary epinephrine and cortisol levels predict acute Ptsd symptoms in child trauma victims. Psychoneuroendocrinology. (2005) 30:121–8. doi: 10.1016/j.psyneuen.2004.06.004
55. Otte C, Neylan TC, Pipkin SS, Browner WS, Whooley MA. Depressive symptoms and 24-hour urinary norepinephrine excretion levels in patients with coronary disease: findings from the heart and soul study. Am J Psychiatry. (2005) 162:2139–45. doi: 10.1176/appi.ajp.162.11.2139
56. Anderson GM, Dover MA, Yang BP, Holahan JM, Shaywitz SE, Marchione KE, et al. Adrenomedullary function during cognitive testing in attention-deficit/hyperactivity disorder. J Am Acad Child Adolesc Psychiatry. (2000) 39:635–43. doi: 10.1097/00004583-200005000-00018
57. Murdoch RD, Youlten LJ, Williams AJ, Howland K. Plasma concentrations and urinary excretion of histamine after inhalation and subcutaneous injection of histamine. Br. J. Clin. Pharmacol. (1993) 35:171–7. doi: 10.1111/j.1365-2125.1993.tb05682.x
58. Roy A, Linnoila M, Karoum F, Pickar D. Relative activity of metabolic pathways for norepinephrine in endogenous depression. Acta Psychiatr Scand. (1986) 73:624–8. doi: 10.1111/j.1600-0447.1986.tb02734.x
59. Koslow SH, Maas JW, Bowden CL, Davis JM, Hanin I, Javaid J. Csf and urinary biogenic amines and metabolites in depression and mania. a controlled, univariate analysis. Arch Gen Psychiatry. (1983) 40:999–1010. doi: 10.1001/archpsyc.1983.01790080081011
60. Hughes JW, Watkins L, Blumenthal JA, Kuhn C, Sherwood A. Depression and anxiety symptoms are related to increased 24-hour urinary norepinephrine excretion among healthy middle-aged women. J Psychosom Res. (2004) 57:353–8. doi: 10.1016/S0022-3999(04)00064-9
61. Roy A, Pollack S. Are cerebrospinal fluid or urinary monoamine metabolite measures stronger correlates of suicidal behavior in depression? Neuropsychobiology. (1994) 29:164–7. doi: 10.1159/000119081
62. Pliszka SR, Maas JW, Javors MA, Rogeness GA, Baker J. Urinary Catecholamines in attention-deficit hyperactivity disorder with and without comorbid anxiety. J Am Acad Child Adolesc Psychiatry. (1994) 33:1165–73. doi: 10.1097/00004583-199410000-00012
63. Hanna GL, Ornitz EM, Hariharan M. Urinary catecholamine excretion and behavioral differences in Adhd and normal boys. J Child Adolesc Psychopharmacol. (1996) 6:63–73. doi: 10.1089/cap.1996.6.63
64. Baker GB, Bornstein RA, Rouget AC, Ashton SE, van Muyden JC, Coutts RT. Phenylethylaminergic mechanisms in attention-deficit disorder. Biol Psychiatry. (1991) 29:15–22. doi: 10.1016/0006-3223(91)90207-3
65. Kusaga A, Yamashita Y, Koeda T, Hiratani M, Kaneko M, Yamada S, et al. Increased urine phenylethylamine after methylphenidate treatment in children with Adhd. Ann Neurol. (2002) 52:372–4. doi: 10.1002/ana.10302
66. Berry MD. Mammalian central nervous system trace amines. Pharmacologic amphetamines, physiologic neuromodulators. J Neurochem. (2004) 90:257–71. doi: 10.1111/j.1471-4159.2004.02501.x
67. Berk BA, Law TH, Wessmann A, Bathen-Nöthen A, Knebel A, Tipold A, et al. Neurotransmitter concentrations in urine associated with the consumption of a medium-chain triglyceride (MCT) supplement in dogs with idiopathic epilepsy. Proceedings of the 32nd Symposium ESVN-ECVN; 2019 September 12–14; Wroclaw, Poland. J Vet Intern Med. (2020) 34:2990–3057. doi: 10.1111/jvim.15875
68. De Risio L, Bhatti S, Munana K, Penderis J, Stein V, Tipold A, et al. International veterinary epilepsy task force consensus proposal: diagnostic approach to epilepsy in dogs. BMC Vet Res. (2015) 11:148. doi: 10.1186/s12917-015-0462-1
69. Berk BA, Law TH, Packer RMA, Wessmann A, Bathen-Nöthen A, Jokinen TS, et al. A multicenter randomized controlled trial of medium-chain triglyceride dietary supplementation on epilepsy in dogs. J Vet Intern Med. (2020) 34:1248–59. doi: 10.1111/jvim.15756
70. Packer RMA, Law TH, Davies E, Zanghi B, Pan Y, Volk HA. Effects of a ketogenic diet on Adhd-like behavior in dogs with idiopathic epilepsy. Epilepsy Behav. (2016) 55:62–8. doi: 10.1016/j.yebeh.2015.11.014
71. Law TH, Davies ES, Pan Y, Zanghi B, Want E, Volk HA. A randomised trial of a medium-chain tag diet as treatment for dogs with idiopathic epilepsy. Br J Nutr. (2015) 114:1438–47. doi: 10.1017/S000711451500313X
72. Hobbs SL, Law TH, Volk HA, Younis C, Casey RA, Packer RMA. Impact of canine epilepsy on judgement and attention biases. Sci Rep. (2020) 10:17719. doi: 10.1038/s41598-020-74777-4
73. R Core Team. R: A Language Environment for Statistical Computing. R Foundation for Statistical Computing. Vienna, Austria (2020). Available online at: https://www.R-project.org/ (accessed February 23, 2022).
74. Packer RM, Nye G, Porter SE, Volk HA. Assessment into the usage of levetiracetam in a canine epilepsy clinic. BMC Vet Res. (2015) 11:25. doi: 10.1186/s12917-015-0340-x
75. Hernandes MS, Troncone LR. Glycine as a neurotransmitter in the forebrain: a short review. J Neural Transm. (2009) 116:1551–60. doi: 10.1007/s00702-009-0326-6
76. File SE, Fluck E, Fernandes C. Beneficial effects of glycine (Bioglycin) on memory and attention in young and middle-aged adults. J Clin Psychopharmacol. (1999) 19:506–12. doi: 10.1097/00004714-199912000-00004
77. Strzelecki D, Kropiwnicki P, Rabe-Jabłońska J. [Augmentation of antipsychotics with glycine may ameliorate depressive and extrapyramidal symptoms in schizophrenic patients–a preliminary 10-week open-label study]. Psychiatr Pol. (2013) 47:609–20.
78. Almannai M, El-Hattab AW. Inborn errors of metabolism with seizures: defects of glycine and serine metabolism and cofactor-related disorders. Pediatr Clin North Am. (2018) 65:279–99. doi: 10.1016/j.pcl.2017.11.007
79. Percy A. Nonketotic hyperglycinemia in adults: anticipating the unexpected. Neurology. (2005) 64:1105. doi: 10.1212/WNL.64.7.1105
80. Krawiec C, Goyal A. Nonketotic Hyperglycinemia. Statpearls. Treasure Island, FL: StatPearls Publishing LLC (2021).
81. Motamedi G, Meador K. Epilepsy and cognition. Epilepsy Behav. (2003) 4(Suppl. 2):S25–38. doi: 10.1016/j.yebeh.2003.07.004
82. Packer RMA, McGreevy PD, Pergande A, Volk HA. Negative effects of epilepsy and antiepileptic drugs on the trainability of dogs with naturally occurring idiopathic epilepsy. Appl Anim Behav Sci. (2018) 200:106–13. doi: 10.1016/j.applanim.2017.11.008
83. Packer RMA, McGreevy PD, Salvin HE, Valenzuela MJ, Chaplin CM, Volk HA. Cognitive dysfunction in naturally occurring canine idiopathic epilepsy. PLoS ONE. (2018) 13:e0192182. doi: 10.1371/journal.pone.0192182
84. Winter J, Packer RMA, Volk HA. Preliminary assessment of cognitive impairments in canine idiopathic epilepsy. Vet Rec. (2018) 182:633. doi: 10.1136/vr.104603
85. Berk BA, Packer RMA, Law TH, Wessmann A, Bathen-Nöthen A, Jokinen TS, et al. Medium-chain triglycerides dietary supplement improves cognitive abilities in canine epilepsy. Epilepsy Behav. (2021) 114(Pt A):107608. doi: 10.1016/j.yebeh.2020.107608
86. Jokinen TS, Tiira K, Metsahonkala L, Seppala EH, Hielm-Bjorkman A, Lohi H, et al. Behavioral abnormalities in Lagotto Romagnolo dogs with a history of benign familial juvenile epilepsy: a long-term follow-up study. J Vet Intern Med. (2015) 29:1081–7. doi: 10.1111/jvim.12611
87. Thome-Souza S, Kuczynski E, Assumpção F Jr, Rzezak P, Fuentes D, Fiore L, et al. Which factors may play a pivotal role on determining the type of psychiatric disorder in children and adolescents with epilepsy? Epilepsy Behav. (2004) 5:988–94. doi: 10.1016/j.yebeh.2004.09.001
89. Hydbring-Sandberg E, Larsson E, Madej A, Höglund OV. Short-term effect of ovariohysterectomy on urine serotonin, cortisol, testosterone and progesterone in bitches. BMC Res Notes. (2021) 14:265. doi: 10.1186/s13104-021-05680-y
90. DeFilippis M, Wagner KD. Management of treatment-resistant depression in children and adolescents. Paediatr Drugs. (2014) 16:353–61. doi: 10.1007/s40272-014-0088-y
91. Simpson BS, Landsberg GM, Reisner IR, Ciribassi JJ, Horwitz D, Houpt KA, et al. Effects of reconcile (Fluoxetine) chewable tablets plus behavior management for canine separation anxiety. Vet Ther. (2007) 8:18–31.
92. Ramsey I. Bsava Small Animal Formulary. 8th ed. Gloucester: BSAVA Small Animal Veterinary Association (2014). p. 166.
93. Watson F, Rusbridge C, Packer RMA, Casey RA, Heath S, Volk HA. A review of treatment options for behavioural manifestations of clinical anxiety as a comorbidity in dogs with idiopathic epilepsy. Vet J. (2018) 238:1–9. doi: 10.1016/j.tvjl.2018.06.001
94. Wrzosek M, Płonek M, Nicpoń J, Cizinauskas S, Pakozdy A. Retrospective multicenter evaluation of the “fly-catching syndrome” in 24 dogs: Eeg, baer, Mri, Csf findings and response to antiepileptic and antidepressant treatment. Epilepsy Behav. (2015) 53:184–9. doi: 10.1016/j.yebeh.2015.10.013
95. Meldrum BS, Rogawski MA. Molecular targets for antiepileptic drug development. Neurotherapeutics. (2007) 4:18–61. doi: 10.1016/j.nurt.2006.11.010
96. Rogawski MA, Löscher W. The neurobiology of antiepileptic drugs. Nat Rev Neurosci. (2004) 5:553–64. doi: 10.1038/nrn1430
97. Aoki K, Kuroiwa Y. Effect of acute and chronic phenobarbital treatment on Gaba and other amino acids contents in seven regions of the rat brain. J Pharmacobio Dyn. (1982) 5:88–96. doi: 10.1248/bpb1978.5.88
98. Hussain LS, Reddy V, Maani CV. Physiology, Noradrenergic Synapse. Treasure Island: StatPearls (2020).
99. William Tank A, Lee Wong D. Peripheral and central effects of circulating catecholamines. Compreh Physiol. 5:1–15. doi: 10.1002/cphy.c140007
100. Giorgi FS, Pizzanelli C, Biagioni F, Murri L, Fornai F. The role of norepinephrine in epilepsy: from the bench to the bedside. Neurosci Biobehav Rev. (2004) 28:507–24. doi: 10.1016/j.neubiorev.2004.06.008
101. Ghasemi M, Mehranfard N. Mechanisms underlying anticonvulsant and proconvulsant actions of norepinephrine. Neuropharmacology. (2018) 137:297–308. doi: 10.1016/j.neuropharm.2018.05.015
102. Fitzgerald PJ. Is elevated norepinephrine an etiological factor in some cases of epilepsy? Seizure. (2010) 19:311–8. doi: 10.1016/j.seizure.2010.04.011
103. Borodovitsyna O, Flamini M, Chandler D. Noradrenergic modulation of cognition in health and disease. Neural Plast. (2017) 2017:6031478. doi: 10.1155/2017/6031478
104. Gannon M, Che P, Chen Y, Jiao K, Roberson ED, Wang Q. Noradrenergic dysfunction in Alzheimer's disease. Front Neurosci. (2015) 9:220. doi: 10.3389/fnins.2015.00220
105. Biederman J. Attention-deficit/hyperactivity disorder: a selective overview. Biol Psychiatry. (2005) 57:1215–20. doi: 10.1016/j.biopsych.2004.10.020
106. Matthews KL, Chen CP-H, Esiri MM, Keene J, Minger SL, Francis PT. Noradrenergic changes, aggressive behavior, and cognition in patients with dementia. Biol Psychiatry. (2002) 51:407–16. doi: 10.1016/S0006-3223(01)01235-5
107. Darcq E, Kieffer BL. Pi3k Signaling in the Locus coeruleus: a new molecular pathway for adhd research. EMBO Mol Med. (2015) 7:859–61. doi: 10.15252/emmm.201505266
108. Dvoráková M, Jezová D, Blazícek P, Trebatická J, Skodácek I, Suba J, et al. Urinary catecholamines in children with attention deficit hyperactivity disorder (Adhd): modulation by a polyphenolic extract from pine bark (Pycnogenol). Nutr Neurosci. (2007) 10:151–7. doi: 10.1080/09513590701565443
109. LaFrance WC, Kanner AM, Hermann B. Psychiatric comorbidities in epilepsy. Int Rev Neurobiol. (2008) 83:347–83. doi: 10.1016/S0074-7742(08)00020-2
110. Gerra G, Zaimovic A, Zambelli U, Timpano M, Reali N, Bernasconi S, et al. Neuroendocrine responses to psychological stress in adolescents with anxiety disorder. Neuropsychobiology. (2000) 42:82–92. doi: 10.1159/000026677
111. Bazil CW. Epilepsy and sleep disturbance. Epilepsy Behav. (2003) 4:39–45. doi: 10.1016/j.yebeh.2003.07.005
112. Forsgard JA, Metsahonkala L, Kiviranta AM, Cizinauskas S, Junnila JJT, Laitinen-Vapaavuori O, et al. Seizure-precipitating factors in dogs with idiopathic epilepsy. J Vet Intern Med. (2019) 33:701–7. doi: 10.1111/jvim.15402
113. Huang Y, Mai W, Hu Y, Wu Y, Song Y, Qiu R, et al. Poor sleep quality, stress status, and sympathetic nervous system activation in nondipping hypertension. Blood Pressure Monit. (2011) 16:117–23. doi: 10.1097/MBP.0b013e328346a8b4
114. Irwin M, Thompson J, Miller C, Gillin JC, Ziegler M. Effects of sleep and sleep deprivation on catecholamine and interleukin-2 levels in humans: clinical implications1. The J Clin Endocrinol Metab. (1999) 84:1979–85. doi: 10.1210/jcem.84.6.5788
115. Pollay M. Movement of glycine across the blood-brain barrier of the rabbit. J Neurobiol. (1976) 7:123–8. doi: 10.1002/neu.480070205
116. Yudilevich DL, De Rose N, Sepúlveda FV. Facilitated transport of amino acids through the blood-brain barrier of the dog studied in a single capillary circulation. Brain Res. (1972) 44:569–78. doi: 10.1016/0006-8993(72)90319-8
117. Wakayama K, Ohtsuki S, Takanaga H, Hosoya K, Terasaki T. Localization of norepinephrine and serotonin transporter in mouse brain capillary endothelial cells. Neurosci Res. (2002) 44:173–80. doi: 10.1016/S0168-0102(02)00120-7
118. Nakatani Y, Sato-Suzuki I, Tsujino N, Nakasato A, Seki Y, Fumoto M, et al. Augmented brain 5-Ht crosses the blood-brain barrier through the 5-Ht transporter in rat. Eur J Neurosci. (2008) 27:2466–72. doi: 10.1111/j.1460-9568.2008.06201.x
119. Takanaga H, Ohtsuki S, Hosoya K, Terasaki T. Gat2/Bgt-1 as a system responsible for the transport of gamma-aminobutyric acid at the mouse blood-brain barrier. J Cereb Blood Flow Metab. (2001) 21:1232–9. doi: 10.1097/00004647-200110000-00012
120. Arriza JL, Fairman WA, Wadiche JI, Murdoch GH, Kavanaugh MP, Amara SG. Functional comparisons of three glutamate transporter subtypes cloned from human motor cortex. J Neurosci. (1994) 14:5559–69. doi: 10.1523/JNEUROSCI.14-09-05559.1994
121. Ohtsuki S. New aspects of the blood-brain barrier transporters; its physiological roles in the central nervous system. Biol Pharm Bull. (2004) 27:1489–96. doi: 10.1248/bpb.27.1489
122. Hardebo JE, Owman C. Characterization of the in vitro uptake of monoamines into brain microvessels. Acta Physiol Scand. (1980) 108:223–9. doi: 10.1111/j.1748-1716.1980.tb06526.x
123. Koepsell H, Busch A, Gorboulev V, Arndt P. Structure and function of renal organic cation transporters. News Physiol Sci. (1998) 13:11–6. doi: 10.1152/physiologyonline.1998.13.1.11
124. Boren DR, Henry DP, Selkurt EE, Weinberger MH. Renal modulation of urinary catecholamine excretion during volume expansion in the dog. Hypertension. (1980) 2:383–9. doi: 10.1161/01.HYP.2.4.383
125. Spiller R. Serotonin and Gi clinical disorders. Neuropharmacology. (2008) 55:1072–80. doi: 10.1016/j.neuropharm.2008.07.016
126. Gershon MD. 5-Hydroxytryptamine (Serotonin) in the gastrointestinal tract. Curr Opin Endocrinol Diabetes Obes. (2013) 20:14–21. doi: 10.1097/MED.0b013e32835bc703
127. Buu NT, Duhaime J, Kuchel O. Handling of dopamine and dopamine sulfate by isolated perfused rat kidney. Am J Physiol. (1986) 250(6 Pt 2):F975–9. doi: 10.1152/ajprenal.1986.250.6.F975
128. Briguglio M, Dell'Osso B, Panzica G, Malgaroli A, Banfi G, Zanaboni Dina C, et al. Dietary neurotransmitters: a narrative review on current knowledge. Nutrients. (2018) 10:591. doi: 10.3390/nu10050591
129. O'Mahony SM, Clarke G, Borre YE, Dinan TG, Cryan JF. Serotonin, tryptophan metabolism and the brain-gut-microbiome axis. Behav Brain Res. (2015) 277:32–48. doi: 10.1016/j.bbr.2014.07.027
130. Mazzoli R, Pessione E. The neuro-endocrinological role of microbial glutamate and Gaba signaling. Front Microbiol. (2016) 7:1934. doi: 10.3389/fmicb.2016.01934
131. Barrett E, Ross RP, O'Toole PW, Fitzgerald GF, Stanton C. Γ-aminobutyric acid production by Culturable bacteria from the human intestine. J Appl Microbiol. (2012) 113:411–7. doi: 10.1111/j.1365-2672.2012.05344.x
132. Franklin IK, Wollheim CB. Gaba in the endocrine pancreas: its putative role as an islet cell paracrine-signalling molecule. J Gen Physiol. (2004) 123:185–90. doi: 10.1085/jgp.200409016
133. Pérez-Alvarez A, Hernández-Vivanco A, Albillos A. Past, present and future of human chromaffin cells: role in physiology and therapeutics. Cell Mol Neurobiol. (2010) 30:1407–15. doi: 10.1007/s10571-010-9582-0
134. Ziegler MG, Aung M, Kennedy B. Sources of human urinary epinephrine. Kidney Int. (1997) 51:324–7. doi: 10.1038/ki.1997.40
135. Ziegler MG, Kennedy B, Elayan H. Rat renal epinephrine synthesis. J Clin Invest. (1989) 84:1130–3. doi: 10.1172/JCI114276
136. Henry DP, Dentino M, Gibbs PS, Weinberger MH. Vascular compartmentalization of plasma norepinephrine in normal man: the relationships between venous and arterial norepinephrine concentration and the urinary excretion of norepinephrine. J Lab Clin Med. (1979) 94:429–37.
137. Takano K, Yatabe MS, Abe A, Suzuki Y, Sanada H, Watanabe T, et al. Characteristic expressions of Gaba receptors and Gaba producing/transporting molecules in rat kidney. PLoS ONE. (2014) 9:e105835. doi: 10.1371/journal.pone.0105835
138. Lieberman HR, Corkin S, Spring BJ, Wurtman RJ, Growdon JH. The effects of dietary neurotransmitter precursors on human behavior. Am J Clin Nutr. (1985) 42:366–70. doi: 10.1093/ajcn/42.2.366
139. Meyers S. Use of neurotransmitter precursors for treatment of depression. Altern Med Rev. (2000) 5:64–71.
140. Maas JW, Landis DH. A technique for assaying the kinetics of norepinephrine metabolism in the central nervous system in vivo. Psychosom Med. (1966) 28:247–56. doi: 10.1097/00006842-196605000-00005
141. Gallowitsch-Puerta M, Pavlov VA. Neuro-immune interactions via the cholinergic anti-inflammatory pathway. Life Sci. (2007) 80:2325–9. doi: 10.1016/j.lfs.2007.01.002
142. Lechin F, van der Dijs B. Central nervous system circuitry and peripheral neural sympathetic activity responsible for essential hypertension. Curr Neurovasc Res. (2006) 3:307–25. doi: 10.2174/156720206778792894
143. Mayer EA, Tillisch K, Bradesi S. Review article: modulation of the brain-gut axis as a therapeutic approach in gastrointestinal disease. Aliment Pharmacol Ther. (2006) 24:919–33. doi: 10.1111/j.1365-2036.2006.03078.x
144. Pearl PL, Capp PK, Novotny EJ, Gibson KM. Inherited disorders of neurotransmitters in children and adults. Clin Biochem. (2005) 38:1051–8. doi: 10.1016/j.clinbiochem.2005.09.012
145. Kuo MF, Wang HS. Pyridoxal phosphate-responsive epilepsy with resistance to pyridoxine. Pediatr Neurol. (2002) 26:146–7. doi: 10.1016/S0887-8994(01)00357-5
146. Baxter P. Pyridoxine-dependent and pyridoxine-responsive seizures. Dev Med Child Neurol. (2001) 43:416–20. doi: 10.1017/S0012162201000779
147. Wilson MP, Plecko B, Mills PB, Clayton PT. Disorders affecting vitamin B(6) metabolism. J Inherit Metab Dis. (2019) 42:629–46. doi: 10.1002/jimd.12060
148. Scriver CR, Whelan DT. Glutamic acid decarboxylase (Gad) in mammalian tissue outside the central nervous system, and its possible relevance to hereditary vitamin B6 dependency with seizures. Ann N Y Acad Sci. (1969) 166:83–96. doi: 10.1111/j.1749-6632.1969.tb54259.x
149. Pope S, Artuch R, Heales S, Rahman S. Cerebral folate deficiency: analytical tests and differential diagnosis. J Inherit Metab Dis. (2019) 42:655–72. doi: 10.1002/jimd.12092
150. Hyland K, Shoffner J, Heales SJ. Cerebral folate deficiency. J Inherited Metab Dis. (2010) 33:563–70. doi: 10.1007/s10545-010-9159-6
151. Kanmaz S, Simsek E, Yilmaz S, Durmaz A, Serin HM, Gokben S. Cerebral folate transporter deficiency: a potentially treatable neurometabolic disorder. Acta Neurol Belg. (2021). doi: 10.1007/s13760-021-01700-7 [Epub ahead of print].
152. Hansen FJ, Blau N. Cerebral folate deficiency: life-changing supplementation with folinic acid. Mol Gen Metab. (2005) 84:371–3. doi: 10.1016/j.ymgme.2004.12.001
153. Pearl PL, Shukla L, Theodore WH, Jakobs C, Michael Gibson K. Epilepsy in succinic semialdehyde dehydrogenase deficiency, a disorder of Gaba metabolism. Brain Dev. (2011) 33:796–805. doi: 10.1016/j.braindev.2011.04.013
154. Drasbek KR, Vardya I, Delenclos M, Gibson KM, Jensen K. Ssadh deficiency leads to elevated extracellular Gaba levels and increased Gabaergic neurotransmission in the mouse cerebral cortex. J Inherit Metab Dis. (2008) 31:662–8. doi: 10.1007/s10545-008-0941-7
155. Fiumara A, Bräutigam C, Hyland K, Sharma R, Lagae L, Stoltenborg B, et al. Aromatic L-amino acid decarboxylase deficiency with hyperdopaminuria. Clinical and laboratory findings in response to different therapies. Neuropediatrics. (2002) 33:203–8. doi: 10.1055/s-2002-34497
Keywords: neurotransmitter, epilepsy, biomarker, urinary, canine
Citation: Schmidt T, Meller S, Talbot SR, Berk BA, Law TH, Hobbs SL, Meyerhoff N, Packer RMA and Volk HA (2022) Urinary Neurotransmitter Patterns Are Altered in Canine Epilepsy. Front. Vet. Sci. 9:893013. doi: 10.3389/fvets.2022.893013
Received: 09 March 2022; Accepted: 22 April 2022;
Published: 16 May 2022.
Edited by:
Daisuke Hasegawa, Nippon Veterinary and Life Science University, JapanReviewed by:
Shinji Tamura, Tamura Animal Clinic, JapanSam Long, Veterinary Referral Hospital, Australia
Adrian Sewell, Biocontrol, Germany
Copyright © 2022 Schmidt, Meller, Talbot, Berk, Law, Hobbs, Meyerhoff, Packer and Volk. This is an open-access article distributed under the terms of the Creative Commons Attribution License (CC BY). The use, distribution or reproduction in other forums is permitted, provided the original author(s) and the copyright owner(s) are credited and that the original publication in this journal is cited, in accordance with accepted academic practice. No use, distribution or reproduction is permitted which does not comply with these terms.
*Correspondence: Holger A. Volk, SG9sZ2VyLlZvbGtAdGloby1oYW5ub3Zlci5kZQ==