- 1CIRAD, UMR ASTRE, Montpellier, France
- 2ASTRE, Univ Montpellier, CIRAD, INRAE, Montpellier, France
- 3French Establishment for Fighting Zoonoses (ELIZ), Malzéville, France
Crimean–Congo hemorrhagic fever (CCHF) is a viral zoonotic disease resulting in hemorrhagic syndrome in humans. Its causative agent is naturally transmitted by ticks to non-human vertebrate hosts within an enzootic sylvatic cycle. Ticks are considered biological vectors, as well as reservoirs for CCHF virus (CCHFV), as they are able to maintain the virus for several months or even years and to transmit CCHFV to other ticks. Although animals are not symptomatic, some of them can sufficiently replicate the virus, becoming a source of infection for ticks as well as humans through direct contact with contaminated body fluids. The recent emergence of CCHF in Spain indicates that tick–human interaction rates promoting virus transmission are changing and lead to the emergence of CCHF. In other European countries such as France, the presence of one of its main tick vectors and the detection of antibodies targeting CCHFV in animals, at least in Corsica and in the absence of human cases, suggest that CCHFV could be spreading silently. In this review, we study the CCHFV epidemiological cycle as hypothesized in the French local context and select the most likely parameters that may influence virus transmission among tick vectors and non-human vertebrate hosts. For this, a total of 1,035 articles dating from 1957 to 2021 were selected for data extraction. This study made it possible to identify the tick species that seem to be the best candidate vectors of CCHFV in France, but also to highlight the importance of the abundance and composition of local host communities on vectors' infection prevalence. Regarding the presumed transmission cycle involving Hyalomma marginatum, as it might exist in France, at least in Corsica, it is assumed that tick vectors are still weakly infected and the probability of disease emergence in humans remains low. The likelihood of factors that may modify this equilibrium is discussed.
Introduction
Arboviruses are viruses transmitted by arthropod vectors to different hosts, which are susceptible to cause diseases threatening public and animal health (1). One of these viruses is considered as high risk of emergence in Europe is the Crimean–Congo hemorrhagic fever virus (CCHFV).
Crimean–Congo Hemorrhagic Fever was first described in 1944–1945 (2) in Russian soldiers and peasants who were exposed to ticks in Crimea and in 1956 in Congo (3). This zoonotic disease remains asymptomatic in animals while causing an acute and potentially fatal infection in humans (4). After an incubation period of 2–9 days, fever, progressive lassitude, and behavioral changes can occur, but these symptoms may be mild and go unnoticed or misdiagnosed. In the second phase, some patients (10–40%) develop intracranial and intraperitoneal bleeding, as well as a petechial rash, leading to coma or death (5, 6). Infection in humans usually occurs via a tick bite or from contact with contaminated body fluids of infected livestock or human patients (7). In nature, CCHFV is transmitted through an enzootic tick–non-human vertebrate–tick sylvatic cycle. Ticks, mostly of the Hyalomma genus, are considered biological vectors, as well as reservoirs for CCHFV, as they are able to maintain the virus for several months or even years. They are also able to transmit CCHFV from one generation to the next (vertical transmission), from one development stage to the other (transovarial transmission), from males to females during copulation (sexual transmission), or from one tick to other ticks feeding closely on a same non-viremic host (cofeeding) (8). Although vertebrate animals are not symptomatic, they can replicate the virus and be a source of infection for both ticks and humans (9), at least during viremia, which is described to last no more than 7–10 days (10). Currently, CCHF is recognized as endemic or potentially endemic in multiple areas of the world throughout Africa, Asia, and Middle East (11). In Europe and in the Mediterranean Basin, it is considered as an emerging disease, with epidemics observed in Turkey since 2002, regular cases in some countries of Balkans, and occasional autochthonous cases recently reported in Spain, as well as one case in Greece (12, 13). As CCHFV has been described in multiple tick and vertebrate species, it circulates on several continents according to the distribution of its tick vectors and different sedentary or migratory vertebrate hosts, which are amplifiers of virus and/or ticks (14). With global warming, the distribution of vectors is changing, potentially modifying CCHFV circulation and increasing the risk of virus emergence in new geographic areas (15). Indeed, the establishment of one of the CCHFV tick vectors, Hyalomma marginatum, was recently demonstrated in the mainland of France while it has been already reported in southern Corsica island for 50 years (16, 17). Given the human cases of CCHF recently reported in Spain and the regular exchanges of bulls, horses, and wildlife with this neighboring country, CCHFV circulation was questioned in France.
In areas potentially at risk for CCHFV introduction and circulation, serological surveillance of sensitive domestic animals is a rational system for the early detection of virus circulation (18, 19). It is even more relevant that a large panel of vertebrate animals can host Hyalomma ticks and CCHFV, and can develop CCHFV antibodies persisting for several years (10, 20). Sheep are known to sufficiently replicate CCHFV and to show high seroprevalence in some countries (21, 22), and are therefore considered suitable sentinels for monitoring and detecting outbreaks/circulation in new and non-endemic areas (18). Cattle are one of the common domestic hosts for the adult stage of Hyalomma ticks and could also be appropriate sentinels for determining at-risk areas for CCHFV circulation since they produce antibodies upon first contact with the virus (23). In France, a preliminary serological study on cattle and small ruminants was carried out in Corsica between 2014 and 2016. Seropositivity of 13% in cattle and 2–3% in small ruminants was observed and the presence of specific neutralizing antibodies was confirmed by plaque reduction neutralization test (23). This suggests that CCHFV already circulates in Corsica, although CCHFV remains to be isolated and genotyped to confirm its presence and phylogenetic origin. This study also highlighted a spatial hotspot of high seroprevalence in the northwest of the island (23). No data is currently available for mainland France but investigations are in progress to determine the epidemiological status of the continental France. Assuming that CCHFV is already circulating at least in Corsica but maybe more widely on the French territory, as any animals must be infected by CCHFV to develop an immune response, the seroprevalence measured can reflect the rate of CCHFV infection in animal vertebrates and can be a proxy for estimating the intensity of CCHFV transmission among the enzootic natural cycle.
To maximize early detection of arbovirus emergence in non-endemic areas, surveillance efforts should target areas where circulation is most likely, and thus identifying these potentially emerging hotspots is a major challenge (24). Determining ecological conditions leading to more or less transmission improves our ability to predict risks (24). Regarding CCHF, there have been several epidemiological and modeling studies examining such factors influencing virus introduction and spread, at the regional or country level (15, 25–29). However, the epidemiology of CCHF is complex and territory dependent, with host–vector–pathogen interactions that can vary from one socio–ecosystem to another (30). If CCHFV is confirmed to circulate in France (apart from ongoing serological and entomological investigation), questions arise regarding the factors responsible for its local amplification and diffusion, to explain the observed occurrence of CCHFV antibodies in ruminants without any reported human cases.
This study aims to review the literature in its entirety concerning CCHF, to revisit the CCHF epidemiological cycle as hypothesized in the French local context and select the most likely parameters that may influence the virus transmission among tick vectors and non-human vertebrate hosts. Hypotheses are also made on other possible not-yet-confirmed factors that may be specific to our French socio–ecosystem.
Available Bibliographic Resources
In 2018, Dereli and Kayser conducted an overview of scientific publications in the CCHF field, to identify new research strategies to control the virus. For our current review, we used the same search databases that produced the highest number of resources in the previous survey, namely, PubMed, Scopus and ScienceDirect, and the same keywords “Crimean–Congo Hemorrhagic Fever” under different orthographies. We framed our searches from the 1940s, the first mentions of articles dedicated to CCHF according to the previous study, to December 2021. By combining the three databases, we obtained 1994 articles (Figure 1), with an average of 123 new articles per year since 2015, the last date included in the previous study. This is in line with the reported increase of publications since the massive CCHF human outbreaks in Turkey in 2002, with an average of 100 articles per year since 2011 (31).
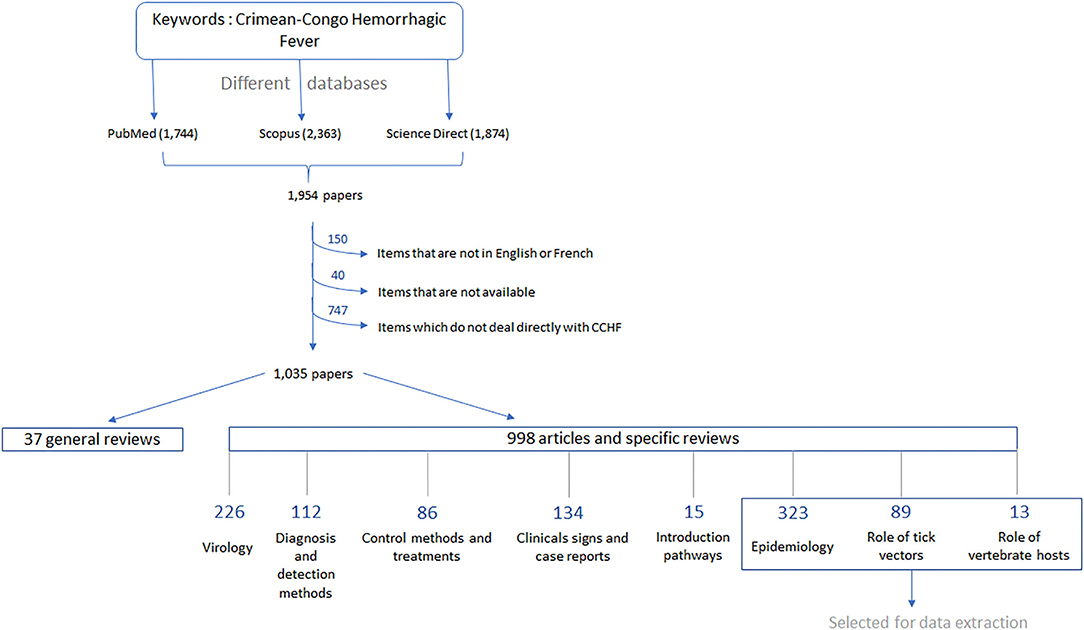
Figure 1. Workflow summarizing the steps taken to classify the different articles in the literature and to select the categories of interest for our study.
Bibliographic resources for which the content was neither available in French nor in English (i.e., written in Russian, Serbian, Turkish, or Japanese) were excluded, as we could not easily exploit them. We also removed articles that did not deal directly with CCHF, as no data could be extracted. Original studies, reviews, and some reports were included in this study. There was also one book dedicated to CCHF that was considered as a sum of several publications. In the end, a total of 1,035 resources, dating from 1947 to 2021, were selected for data extraction and were sorted based on their titles, abstracts, and keywords into a few categories (Figure 1). For the purpose of our review, we directly removed those related to CCHFV virology number = 226, CCHF diagnosis and detection methods (32), CCHF symptoms in the form of case reports number = 134, as well as CCHF control and treatment (33) as they were not useful for the data extraction. As we focused on the enzootic circulation of CCHFV, we also removed the articles studying introduction pathways (15). For our analysis, we kept general reviews on many aspects of CCHF (34) and those related to CCHF epidemiology number = 323, the role of ticks as CCHFV vectors (35), and the role of vertebrate animals in CCHF (13). Concerning epidemiology, we considered any studies related to the frequency and distribution of CCHFV in humans and animals, as well as the factors influencing its occurrence. This included the large number of serological surveys conducted in many different countries, serving as the basis for the first investigations on CCHF.
Publications serving specifically as information resources for data extraction were referenced as Supplementary Material. For each topic, we first considered the existing reviews as they were expected to synthetize all available data prior to their publication date. To assess their exhaustiveness and relevance, we systematically referred to part of the original cited articles, and then completed or updated data with lacking or newly published information. Regarding tick vectors and vertebrate hosts that should be considered in the CCHFV transmission cycle, we considered the historical review on the epidemiology of CCHF in Asia, Europe, and Africa published by Hoogstraal (14), as well as two specific articles, written by Turell (36) and Nalca and Whitehouse (37). More recently, Gargili et al. (8) provided an update of field and laboratory studies that have contributed to demonstrate vector competence and capacity of different tick species and genera for CCHFV. At the same time, Spengler et al. (10) proposed a systematic review of experimental CCHFV infection studies, assessing the role of many vertebrate animals in CCHFV amplification and transmission.
Some data not directly extracted from our reviewing process were also necessary to complete our understanding on tick vectors and vertebrate hosts in the French context. Concerning host preferences of potential CCHFV tick vectors, especially those of H. marginatum, we did not review all the available literature, as it was not the main purpose of our study. However, we made preliminary estimations based on the relative mean infestation rates and the relative proportions of ticks per host species, using what we considered to be reference articles, providing comparable and relevant data for our geographical zone of interest. For host densities in France and the capacity of hosts to spread, we used census and predictive maps available from the French Biodiversity Office (OFB), data archives from major European projects working on the prediction of vector-borne disease transmission (EDENext and Pale–Blue), as well as specific scientific articles. All the related publications or sources of these “extra” data were referenced in Supplementary Material.
Assessment of French Tick Vectors and Reservoirs of CCHFV
Although the involvement of Hyalomma ticks as main vectors and reservoirs of CCHFV is confirmed and quite well-documented, the role of the other tick genera in CCHFV replication and transmission is not as clear. The epidemiological status of ticks is all the more difficult to assess, as detecting the viral genome, or even isolating the virus in a tick does not mean that the latter is able to maintain and replicate the virus, especially when collected directly on viremic animals from which it ingested the virus during a blood meal. In addition, being a competent vector for a virus (i.e., the ability to become infected during a blood meal, replicate the virus, and transmit the virus to another host through a subsequent bite) does not necessarily result in efficient transmission in nature because several other parameters, such as vector density and longevity, feeding rate, and trophic preferences of vectors, as well as the infectious period and susceptibility of vertebrate hosts, are involved in what is called vectorial capacity (36). As a consequence, replication of CCHFV in the tick throughout its development cycle but also the additional tick-to-tick transmission pathways (which characterize the ability of ticks to serve as reservoirs) are crucial in CCHF epidemiology. This is especially true when the likelihood of being infected from viremic animal hosts is low and when it is necessary for ticks to maintain CCHFV infection during periods such as winter in temperate regions such as France that limit active virus transmission (8). In addition, differential tick biology may impact their vector and reservoir roles. In Ixodid ticks, there is one larval, one nymphal, and one adult stage, which each require a bloodmeal from vertebrate hosts before molting or reproducing (38). Ticks known or suspected as CCHFV vectors can range from one-host species (where each parasitic stage feeds on the same host) to three-host species (where each parasitic stage feeds on different hosts), which impact the opportunity for a tick to become infected from a viremic animal and retransmit the virus during its lifetime (14, 39).
Tick species that have been found to be infected at least once by CCHFV and are present in France are indicated in Table 1 and related references are indicated in Supplementary Data 1. According to several criteria shown in Table 1, we were able to rank ticks according to their presupposed importance as CCHFV vectors within the enzootic transmission cycle in the French local context. We did not consider tick species that are not present in France, although they could be introduced punctually (e.g., through migratory birds for H. rufipes or imported ruminant for H. anatolicum) (16, 40), and may be responsible for CCHFV introduction, they cannot establish viable populations allowing persistent local virus transmission. Concerning H. lusitanicum, it apparently likely transmits CCHFV in Spain. Indeed, CCHFV has been mostly detected in such tick species compared to H. marginatum, and red deer on which adult stages likely engorge have been found 3 times more CCHFV seropositive than cattle in Spanish areas where CCHFV circulates locally (34). Unfortunately, there is still no experimental data to confirm its vector competence. In France, H. lusitanicum was historically reported in south–western part of the territory, as well as in Camargue at the east (20, 41). For the last 60 years, no new reports of H. lusitanicum has been referenced. Based on many field observations recorded in an extensive synthesis, Morel (42) described this species as clearly dependent on rabbit populations although Valcárcel (43) recently modulated this assertion. With the quasi-extinction of wild rabbits in France due to myxomatosis epizooties in the 1950s (44), we consequently assumed that this tick species has disappeared from the territory or at least drastically declined to remain anecdotal through residual small populations. Without any updates of presence, we thus decided not to include this tick species into Table 1, although keeping in mind its epidemiological importance elsewhere.
As a result, and based on our bibliographic review, it appears that H. marginatum seems to be the best “candidate” for the transmission of CCHFV in France. It succeeded experimentally in all pathways of transmission, and is often associated with CCHF human cases in other endemic countries, and presents bioecological features favoring engorgement on potential CCHFV amplifier hosts at immature and adult stages. Its abundance in Corsica and its recent establishment in the southern mainland (16, 43, 45), in addition with the detection of CCHFV antibodies in Corsican cattle (23), make it a very suitable vector with the risk of CCHFV circulation. Dermacentor marginatus, which is autochthonous in the South of France (41, 46) and which adults stages can also engorge on CCHFV amplifier hosts would also be a suitable vector. Although its ability for transovarial transmission from infected females to subsequent generations was demonstrated once in the 1970s (47), no further assays have been conducted since this date and evidence of CCHFV genome detection in ticks remains scarce compared to H. marginatum. In the east of the Mediterranean Basin, where CCHFV circulates at high levels, several studies did not detect CCHFV in D. marginatus, whereas the virus was amplified from other tick species collected on the same animals (48–51). However, D. marginatus ticks feeding on wild boars were found to be infected in CCHF endemic areas in Spain, without determining the infectious status of animals (34). In Turkey, some specimens collected from wild boars were also found infected but this was attributed to their association with Hyalomma infected ticks on the same host (52). Therefore, further investigation is required to definitively confirm or deny D. marginatus as a CCHFV vector; however, its role as a secondary vector cannot be excluded at this stage. Apart from these two species, no other ticks present in France presented virological or bioecological criteria to be considered as potential CCHFV vectors. Historically, Hyalomma scupense has been described in western France and has been recently identified in Corsica (45, 53). Although it could be assumed to be a competent as any Hyalomma tick species, the fact that it infests the same cattle host throughout its development cycle (one-host tick) and cannot transmit CCHFV to its progeny (14) does not make this tick species a suitable vector of CCHFV. Regarding Rh. bursa, Gargili et al. (8) suggested that its bioecology could be favorable for CCHFV transmission but in absence of the relevant virological evidence, we did not consider this species as a suitable vector, despite its abundance in the South of France (17, 41, 54).
Assessment of French Vertebrate Animals as Hosts of CCHFV and Tick Vectors
We considered that vertebrate animals can contribute to CCHFV transmission within the natural enzootic cycle through three major abilities: (i) by sufficiently replicating CCHFV to be able to infect tick vectors during a blood meal, (ii) by amplifying tick vector populations and providing sufficient naive ticks to be infected, and (iii) by introducing CCHFV or CCHFV-infected or uninfected tick vectors in free geographical areas through long-distance movements. Regarding the community of vertebrate hosts to be considered in a potential CCHFV enzootic cycle in the French context, we decided to focus on those infested by H. marginatum as it was identified to be the best current “candidate” vector to locally transmit CCHFV.
Although data are scarce (Supplementary Data 2), the monitoring of virus kinetics in experimentally infected animals is the only relevant method to assess the role of vertebrate hosts as CCHFV amplifiers. Conversely, many field investigations are based on serological tests measuring antibodies targeting CCHFV as this is very useful for early detection and provides relevant information on transmission levels (14). However, some animal species, such as horses, can develop the same levels of antibodies as any other animals although their viremia remains insufficient to allow the infection of new naive ticks to maintain transmission through blood feeding (10). As a consequence, we consider that serology likely reflects the exposure of vertebrate hosts to CCHFV-infected tick vectors, depending either on tick bite frequency or on tick infection rate. As stated above, cattle and small ruminants have often been reported as the most sensitive indicators of low-level CCHFV circulation, as they seem to be highly infested by CCHFV tick vectors (55, 56). However, this can vary according to the geographical region and the local CCHFV transmission cycle, and does not predict their ability as CCHFV amplifiers. Another limiting aspect of using field serological surveys is the difficulty to compare results, as many different techniques with distinct sensitivity and specificity were used over the years and between studies (55). As viremia is short in any animal, reports of direct CCHFV detection in field animal samples remain too scarce to be used to assess their relative role in CCHFV replication. The few symptoms in animals, when present, are not informative on their role as CCHFV amplifiers since they do not seem to be correlated with viremia (10).
Regarding the ability of vertebrate animals to amplify tick vector populations, this mainly depends on host preferences and abundance of tick vectors, but also on the availability of vertebrate hosts for ticks. Theories are very extensive on host preferences in ticks, ranging from the necessity for ticks to be specialist and co-evolve with their hosts, and consequently their environment (57), to their likely adaptation to abiotic conditions and specific habitats resulting in host preferences (58). This question remains difficult to assess, as experimental studies providing practical measures are few to none. For H. marginatum, only one study tested the efficiency of its development cycle according to its blood feeding on three different small vertebrates (mice, guinea pig, and white rabbit), which led to a laboratory animal model useful for CCHFV transmission experiments (59). Two field surveys were also conducted to test the effect of host species and host abundances on population densities of H. aegyptium and H. lusitanicum (60, 61). For the latter, it was shown that the experimental removal of hares from the environment was significantly correlated to a lower abundance of H. lusitanicum, although it did not prevent its presence. Based on such partial results, field data reporting infestation rates of several host species by H. marginatum or relative proportions of H. marginatum among all tick species found on different hosts may be a proxy to assess host preferences of this tick species. These studies are numerous, at least for common domestic hosts such as cattle, small ruminants, and horses (Supplementary Data 2). However, scientists have usually investigated ticks from livestock because of its economic importance and the confirmed side effects of ticks and tick-borne infections on productivity. The same phenomenon can be observed on emblematic wild vertebrates and this may bias comparisons between vertebrate hosts. For H. marginatum, only one study is available on its presence on a striped field mouse (Apodemus agrarius) in Bulgaria (62). However, because rodents are frequently examined for ticks as they are reservoirs for Lyme Borreliosis and ick-borne encephalitis causative agents, we can assume that H. marginatum never, or extremely rarely, parasitizes rodents, as suggested by Hoogstraal (14). Another limitation of using field data on tick collections from hosts to assess host preferences is the apparent heterogeneity and incongruences between geographical regions, as it is observed for example for cattle and small ruminants in Romania compared to Turkey, and even within Turkey (52, 63, 64). This could be due to either distinct abilities of ecoclimatic zones to host H. marginatum, which may result in various tick densities, or distinct host communities available for this “generalist” tick. This last case suggests that some ticks could adapt their host preferences according to local contexts and thus we should remain very cautious when interpreting tick data collection from the field.
Finally, to assess the role of hosts as spreaders of CCHFV or CCHFV tick vectors, the most important information is their capacity to move long distances, as well as the duration of tick attachment on the host for blood feeding. As many vertebrate animals show short CCHFV viremia, the probability of movement during viremia is very low; they are therefore more likely to spread ticks infected with CCHFV than to directly spread the virus via physical contacts with contaminated fluids. Hard ticks at all development stages, remain attached to their host during blood feeding for ~10 days, until they become completely engorged (65). However, this duration may increase if the tick species in question reduces its free-living phases, like H. marginatum for which larval and nymphal stages engorge on the same small vertebrate for an average of 14–21 days without falling to the ground for larval molting (20, 59, 66). Although some authors do not support this assumption (10), this period largely covers the duration of Trans-Mediterranean bird migrations, known as major hosts for immature stages (67, 68). Additionally, the commercial trade of livestock may result in long-distance movements of ruminants, potentially infested by CCHFV-infected tick vectors, as their external parasitic status is not necessarily controlled for importation (69). While domestic animal trade is common between European countries, inter alia, from Spain where CCHFV is known to circulate among domestic ungulates, importation of livestock from non-European CCHF-endemic areas into Europe remains rare (70). Occasional wildlife trans locations, such as the reintroduction of the Spanish ibex (Capra hircus) into the transboundary Pyrenees mountains, remain infrequent and highly monitored; however, this can be considered a practice at-risk since CCHFV antibodies have been detected in Spanish Ibex (71). In addition, the international trade of game species for the hunting industry can represent a potential source of introduction into new territories (72). Wild rabbit and hare imports have been reported from Spain since the 1970s to restock French hunting reserves and palliate the disappearance of native species due to myxomatosis and rabbit hemorrhagic disease (73, 74).
Based on the different criteria discussed above, Tables 2A,B, presents an assessment of the different vertebrate hosts of H. marginatum for their role in CCHFV circulation within the natural enzootic transmission cycle (reference articles are indicated in Supplementary Data 2). Voluntarily, we did not include exotic species, present in zoos or other public reception structures as their presence remains sufficiently rare to not significantly change transmission levels. This is the case for camels that are assumed to be good CCHFV amplifiers and can be parasitized by Hyalomma ticks (75), ostriches (Struthio camelus), African starling (Lamprotornis sp.), and red-beaked hornbills (Tockus erythrorynchus), the latter three representing the only bird species able to develop sufficient CCHFV viremia to infect ticks (76, 77). In the case of ostriches, they were also able to contaminate humans by direct contact in African slaughterhouses (78). However, such animals are not naturally and endemically found in France.
Considering Tables 2A,B, lagomorphs, especially hares, seem to play a major role as CCHFV amplifiers, as well as being a preferred host with birds for immature stages of H. marginatum. According to Hoogstraal (14), hares would be the vertebrate hosts mainly involved in the circulation of CCHFV in endemic areas. French populations of hares are stable but very heterogeneous depending on the habitat (74, 79), while wild rabbits remain rare despite repopulation actions. Likewise, some families of birds, especially those feeding on the ground and that can be easily infested by hunting ticks such as H. marginatum, are reported as important hosts for immature stages. In Cyprus and Spain, resident blackbirds and rooks showed very high infestation rates (80, 81). Their role as tick population amplifiers is all the more reason to consider these birds, as they are largely distributed in many diverse habitats of France. In addition, some representatives of these families are migrating birds connecting African and European CCHF endemic areas to France and their ability for spreading ticks potentially infected by CCHFV should be pointed out. Indeed, the recent tick collections conducted in the South of France have reported the presence of immature stages of H. marginatum, but also H. rufipes that is only established in Africa, from migrating Eurasian Blackcaps (Sylvia atricapilla), dunnocks (Prunella modularis), and European robins (Erithacus rubecula) (16). However, most birds are considered refractory to CCHFV and may not act as CCHFV sources (10). Considering adult stages of H. marginatum, the main hosts are domestic ungulates, especially horses and to a lesser extent cattle. Horses are frequent in the South of France whereas cattle farming remains rare, except in Corsica where H. marginatum is highly abundant (17) and in humid pastures of Camargue for bull rearing where H. marginatum cannot develop (82). Both species may be involved in long-distance tick spreading due to international trade, national seasonal transhumance, or even equestrian competitions. However, only cattle have been reported as good CCHFV amplifiers whereas horses cannot be sufficiently viremic to infect ticks (10). Sheep were also demonstrated to efficiently replicate CCHFV but they are very rarely infested by H. marginatum, at least in France (17). The role of wild ungulates remains partially unknown and deserves further investigation, although red deer (Cervus elaphus) has been reported to be an important host for the Spanish tick vector H. lusitanicum and are suspected to be efficient CCHFV amplifiers (34). However, this tick species is apparently absent from France and red deer populations are not abundant in the French area colonized by H. marginatum and seem to be exceptionally infested by this tick (34).
The Presupposed CCHFV Transmission Cycle in France: Importance of Tick Vectors and Vertebrate Host Communities
Few articles describe the entire CCHFV transmission cycle (Supplementary Data 3). Those that exist are mostly dealing with mathematic modeling used to describe generic transmission cycles or surveys that focus on disease epidemiology in some endemic areas, mainly eastern ones. Other publications addressing CCHFV transmission pathways usually focus on one aspect of the viral cycle, such as the role of tick vectors, the status of vertebrate hosts or potential routes for virus transmission. Among the proposed cycles, generic ones that are deliberately simplified to model reality, cannot reflect the total diversity of tick species and vertebrate animals interacting in CCHFV transmission. For example, in West African Sahelian regions, H. truncatum has been considered to be the main vector of CCHFV for humans. However, some “helpers” may locally favor virus transmission and long-term persistence (39). As only 17% of CCHFV transovarial transmission and <1% of CCFV cofeeding is reported in H. truncatum (83), adult ticks may likely become infected at the immature stage through feeding on viremic lagomorphs. However, such small vertebrates are scarce in the region and cannot allow frequent infection in ticks. Fortunately, another tick H. rufipes, present at similar latitudes, was demonstrated as a possible vector of CCHFV, at least within the enzootic cycle; thanks to a large infestation of immature stages on the abundant red-beaked hornbill, the only bird able to amplify CCHFV and replace “traditional” lagomorph amplifiers (76). In southern Sudanese regions where Hyalomma ticks are scarce, Amblyomma variegatum, another tick with a large diversity of vertebrate hosts was also demonstrated to participate in CCHFV transmission. This tick species has a diversity of vertebrate hosts, including “traditional” and abundant cattle amplifiers, parasitized by each developmental stage of this tick species. Its anthropophilic behavior may also make it a good candidate for CCHFV transmission to humans (39). The above example clearly shows the risk of biased predictions when oversimplifying such complex virus epidemiological cycles or adapting previously described cycles to another geographical area, as tick and vertebrate host communities may differ and change the dynamics and levels of virus transmission. Regarding the recent emergence of CCHF in Spain, one could not have predicted that the virus would likely circulate within red deer living in forested areas and that the tick vector would be H. lusitanicum, rather than H. marginatum, the latter being more widely distributed within the country and commonly considered as the main CCHFV vector in southern Europe and northern Mediterranean countries (34, 84).
Considering the assessment of ticks and vertebrate hosts in CCHFV transmission detailed above, the pre-supposed enzootic CCHFV transmission cycle in France can be mapped as illustrated in Figure 2. In our state of knowledge on vector competence and French tick distribution, H. marginatum is considered the only “serious” local candidate for CCHFV transmission. As we hypothesized that H. lusitanicum may be absent or only present in residual populations on our territory, it seems unable to maintain a sustainable and perennial transmission of CCHFV. In the South of France, immature stages of H. marginatum may likely engorge on birds, which are much more numerous in the environment than lagomorphs, though both animal classes are considered preferred hosts. However, as birds are refractory to CCHFV, the probability for ticks to become infected at immature stages and the resulting ability of emerging adults to further infect large ungulates through biting are low. This might be however modulated if cofeeding on birds may occur, but transmission rates using this pathway seem to remain low (85) and we have no specific data for H. marginatum. Moreover, adults of H. marginatum mainly parasitize horses, which are both the preferred hosts and also abundant in the south of France, but cannot develop sufficient viremia to infect ticks. Except if cofeeding may also occur on horses, their role for CCHFV transmission seems to be low to null. Blood feeding on confirmed good CCHFV amplifiers, such as cattle or sheep, remains infrequent or rare and therefore cannot strongly participate in increasing infection level in the tick vector. Regarding wild ungulates, as stated above, only red deer was assumed as good CCHFV amplifiers, although they remain quasi-absent from southern French areas where H. marginatum is established, and are considered unlikely hosts for this tick. Without any more information about the ability of other wild ungulates, especially wild boar, to participate in CCHFV replication or in the amplification of Hyalomma tick populations, their role cannot yet be fully considered. In the current state of our knowledge and our assumptions on CCHFV circulation in France, opportunities for H. marginatum ticks to become infected and to infect new animals would be rare, at any development stage, even if transovarial transmission and cofeeding are partially efficient. Therefore, the risk for humans to be contaminated through either tick bite or direct contact with animals' body fluids can currently be estimated as low. This prediction is based on the theoretical concept of the “dilution effect,” developed by LoGiudice et al. (86) for Lyme disease and emphasizes the impact of host biodiversity and community composition of ecosystems on their functions. The authors identified “dilution hosts” as animals characterized by high tick burden, low reservoir competence, and high population density, as could be the case of horses and/or birds in the French context of CCHFV transmission. Therefore when the host community is less diverse and mostly composed by “dilution hosts,” a “generalist” tick such as H. marginatum is likely to engorge on these hosts and show low infection prevalence (86). This could partially explain the detection of antibodies targeting CCHFV in French domestic ungulates, yet the lack of CCHFV detection in tick and the lack of CCHF human case reports, for example. However, we cannot omit other possible explanations, such as the existence of low virulent CCHFV strains resulting in particularly few (or no) hemorrhagic symptoms, or CCHFV-like viral strains that may be able to cause immune cross-reaction in domestic ungulates but would be too divergent to be detected in ticks using specific CCHFV molecular amplification methods. In addition, although we estimate the risk of CCHF emergence in France as low considering its presumed local transmission cycle between animals and ticks, this situation is not static and is likely to change under climate and global changes, as observed in Spain (69).
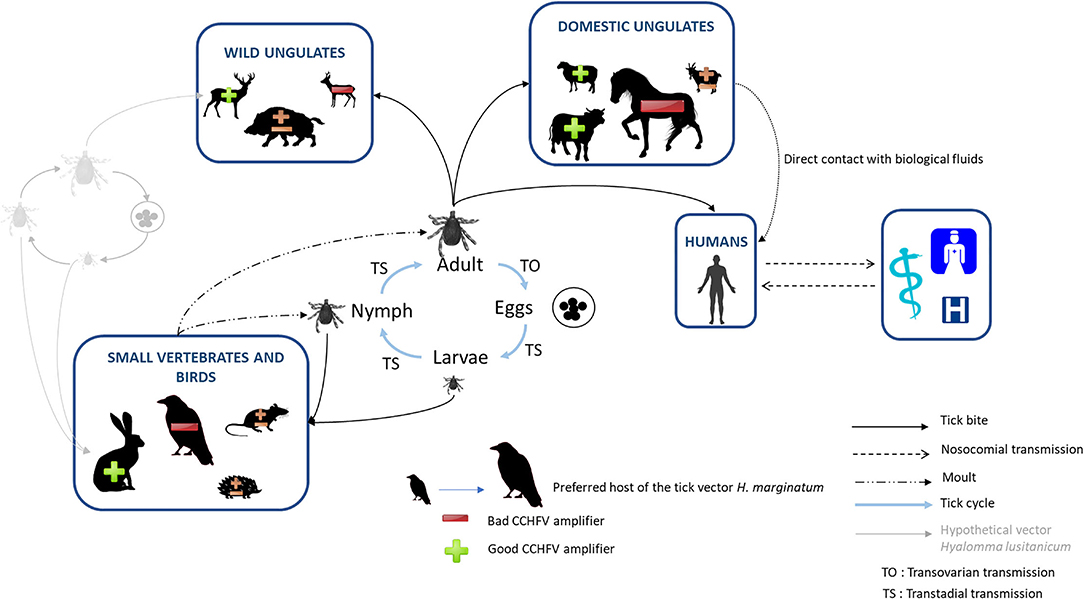
Figure 2. Presumed enzootic transmission cycle of CCHFV in France, involving the candidate tick vector H. marginatum and its different vertebrate hosts. Animals are represented in different sizes according to relative host preferences of H. marginatum. The ability of these animals to replicate CCHFV is indicated by “+” for good CCHFV amplifiers and “–” for bad CCHFV amplifiers.
Factors That May Promote CCHFV Enzootic Transmission in France: Intrinsic Sensitivity and Modulation of Exposure
In the literature, some articles do refer to risk factors involved in CCHFV transmission. Among the 58 articles referenced in our review as epidemiological studies investigating risk factors for the transmission of CCHFV, 34 looked at factors favoring human contamination (usually measured by the incidence of disease cases or the seroprevalence of CCHFV antibodies in humans) and 24 referred to biotic or abiotic factors impacting the natural circulation of CCHFV among vertebrate animals. Based on the latter publications, we were able to schematize the effects of the major factors cited, either on the exposure of vertebrate hosts to CCHFV tick vectors or the prevalence of CCHFV infection among tick vectors. We determined which factors have proximal or ultimate effects and interact together, and we only considered factors in Figure 3 that could make sense within the local French context of CCHFV enzootic transmission.
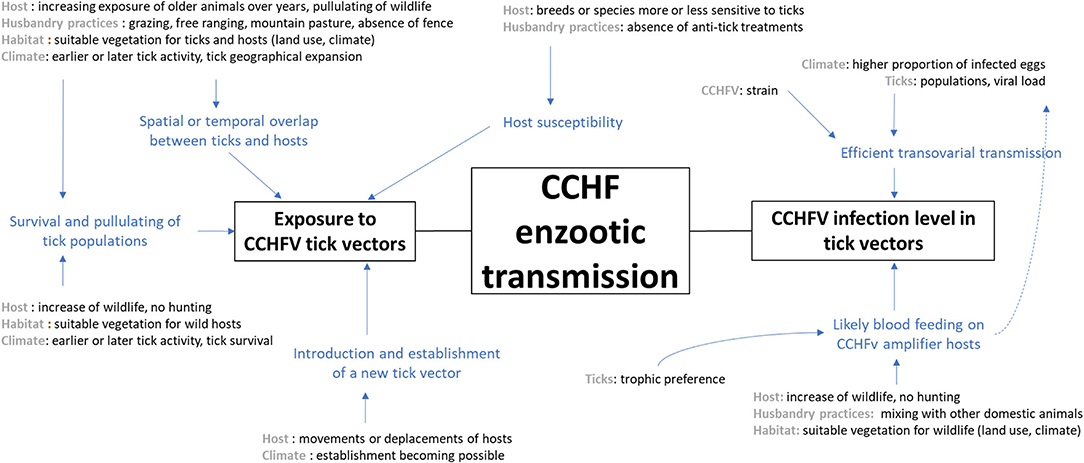
Figure 3. Scheme representing factors that may impact either the exposure of animals to tick vectors or the CCHFV infection level of tick vectors, which could finally influence the CCHFV enzootic transmission in France.
Several studies conducted in Africa and Asia have shown that CCHF seroprevalence significantly increases with the animal age, either in cattle, small ruminants, and camels (33, 35, 87–97). This correlation was mainly attributed to an increasing probability of an animal to be exposed to infected tick vectors, contract CCHFV, and develop an immune response in relation with increasing age (33). Such an additive effect of age seems obvious as antibodies are assumed to persist for a long period in animals, up to several years (98). An additional potential age-linked relevant factor for exposure to CCHFV is variation in tick burden associated with body size, physiological age-linked variations, e.g., immune competence, and behavioral patterns (99). However, other parameters may explain such patterns, such as diverse breeding practices. In Sudan, when assessing age as a risk factor for cattle CCHFV seroprevalence, the authors showed that calves started to get infected after the age of 2 years, as this was when animals were released to pasture and therefore became exposed to infected ticks and subsequently to CCHFV infection (35, 89). In Mauritania, this hypothesis of overexposure in older animals has been also proposed to explain an apparent higher seroprevalence in camels than in cattle or small ruminants, as camels are bred for longer periods of time (95). Although we agree that the age effect may be a confounding factor with animal species, most ticks present host preferences that could result in differential infestation rates according to the vertebrate species. In addition, tick vectors and host species may be not homogeneously distributed, with some vertebrates being over-represented locally and therefore more infested by the tick vectors in question, present in the same zone. The investigation of both age and species effects in a serological survey conducted on small ruminants from Pakistan confirmed significant differences of CCHFV seroprevalence between goat and sheep that were not due to age, as both species are typically kept no more than a few years before slaughtering (88).
Regarding other intrinsic host-related risk factors, significant differences in CCHFV seroprevalence were also detected on several occasions depending on the breed of the animals. In Sudanese cattle, the highest rates of infection have been observed in cross breeds, compared to endogenous ones, as they seemed to be very susceptible to tick infestation (89). Relative resistance of native breeds to tick infestation has often been described in relation to distinct patterns of transmission for several tick-borne pathogens (100–102). However, concerning CCHFV, significant differences were not systematically highlighted, even within the same country (35), and in some cases the tendency was just the opposite (88). The effect of breeds was also reported in camels from Sudan but each breed was actually representative of one locality of the country and may likely reflect local tick exposure (90). In cattle, differences in husbandry practices may also occur depending on the breed, as these breeds do not show the same aptitudes for production. Production systems have their own organizational constraints. Consequently, practices related to animal movements can modify the exposure of animals to CCHFV tick vectors, as discussed in the following. This aspect should be investigated especially in French areas, like Corsica, where traditional cattle breeds are maintained and, suckling farming with free-ranging has become popular and widespread. Moreover, parasite resistance may be influenced by cross-breeding—between the Corsican breed and Limousine, Aubrac, or Charolaise—that some farmers do to increase their production compared to the Corsican breed adapted to harsh scrublands and supposed to be more resistant to ticks.
Apart from the breed, sex as another host-related risk factor was also tested in several studies and its effect was mostly insignificant on the CCHFV seroprevalence (88, 91, 96, 97). The only exception was for cattle from Malawi and South Africa where CCHFV seroprevalence was higher in females than males, although this cannot be attributed to intrinsic resistance in females against ticks (33, 87). In these countries, female cattle are raised mainly for breeding purposes, meaning they spend more time in the fields grazing and therefore have an increased risk of tick exposure. Males, however, are used for drought power and stay away from pastures longer than females and young cattle, particularly during the rainy season when crop cultivation is at its peak and ticks are active (33). In two studies conducted on cattle from Sudan, animal body condition was also tested in relation to CCHFV seroprevalence but its effect was insignificant to the assumption that poor body conditions may invoke higher susceptibility of CCHFV infection in animals (35, 89). Conversely, the possibility that CCHFV infection induces a poorer body condition in infected animal is considered anecdotal, since no clinical signs have been detected in animals to date.
As stated above, husbandry practices, such as feeding systems, can be important factors impacting the exposure of animals to tick vectors and thus there exists the risk for CCHFV transmission. A few studies conducted in Africa and Asia reported higher CCHF seroprevalence in animals grazing in pastures than those fed on trough (87, 88), and even higher prevalence in nomadic herds covering long distances (89). In Iran, Lotfollahzadeh et al. (93) also pointed out vegetation where grazing took place as an additional factor that may favor the presence and abundance of ticks and thus exposure to CCHFV vectors. In the South of France, extensive farming systems where animals are highly exposed to ticks do exist, as observed for cattle rearing in Camargue. However, these areas are of wet habitats and have been demonstrated as unsuitable for the establishment of the H. marginatum tick vector (103). Extensive farming can also be temporary as mostly reported in Corsica where most cattle are free-ranging in suitable scrubs during spring and summer, corresponding to the activity period of the adult stages of H. marginatum. Another husbandry practice that was reported as an important factor modifying the risk of CCHFV transmission is the use of acaricide treatments on animals, as they decrease the infestation load and the susceptibility of animals to ticks and subsequently the probability of CCHFV transmission. This phenomenon was reported in cattle from Pakistan (88) and was considered a reliable preventive strategy when animals from Sudan were introduced into Saudi Arabia (104). This was clearly linked to the tick burden of animals, which was also measured in some studies (33, 35, 87–91). Conversely, some studies reported insignificant or opposite effects of acaricide treatments or tick control actions but a little information was provided on the chemicals and methods used to assess their reliability (35, 87, 89). Although tick infestation rates may be lower in France than those observed in tropical or subtropical regions, some farmers use acaricides or insecticides, punctually, the latter targeting flies, or anthelmintics with ivermectine that can be indirectly active on ticks. These practices should be tested as factors influencing the exposure of animals to CCHFV tick vectors. Finally, several other husbandry practices, such as the flock size, the import of animals into farms, the slaughtering of animals on farms, or the contact with other farms, have been tested in some studies but remained insignificant (87, 88). Two factors captured our attention as they seemed relevant within the French context and could impact not only the exposure of animals to tick vectors but also the level of infection in tick vectors are as follows: (1) The presence of other domestic animals on farms and (2) the possible contact with wildlife (87, 88). As stated above regarding the importance of the host community composition for tick vectors, changes may occur in CCHFV prevalence among tick vectors depending on the probability of these ticks to feed on other viremic and infectious animals reared in conjunction with cattle. In the South of France where H. marginatum has been established, sheep farming and the use of horses for cultivation were previously considered traditional practices. However, farmers have progressively diversified their activities since the 1960s by including suckling cattle for meat production and dairy goats; all these animal species presenting differential abilities to replicate CCHFV and amplify tick populations. Similarly, spatial overlap between cattle and wildlife, such as lagomorphs, birds, or wild boars in absence of fences or due to free-range farming, as observed in Corsica, may change the probability for tick vectors to become infected with CCHFV.
However, apart from the husbandry practices, contact between cattle and wildlife can also result from changes in land use (21). For example, CCHFV emergence in Turkey since 2002 has been mainly attributed to the local increase of hares' abundance, which highly amplified populations of H. marginatum and enhanced their infection with CCHFV. This was mainly due to security issues resulting in the temporary prohibition of hunting and agricultural activities, followed by the reopening of these areas after several years with the sudden exposure of humans to highly infectious ticks (105). This was also concomitant to higher spring temperatures over several successive years within the country that accelerated the development cycle of the tick vector H. marginatum, decreased its density-independent mortality, and thus increased populations available to become infected (106). Kulichenko in the Russian Federation (106) and Vescio in Bulgaria (107) also mentioned climate as a main factor impacting the development of CCHFV tick vectors and thus local virus circulation. Climate can also increase tick search activity and host parasitic load, which promotes virus circulation (24). In a deterministic model considering the total development cycle of H. marginatum and each CCHFV transmission pathway between ticks and vertebrate hosts, Estrada-Pena and collaborators (108) showed that increased temperature, especially in late summer, allows for increased oviposition events in females before unsuitable autumn conditions, which increases survival probability of developing eggs. However, he also demonstrated that a key factor for CCHFV transmission by H. marginatum was a climate-independent biological parameter intrinsic to the tick vector, namely the rate of CCHFV transovarial transmission (108). Although few studies described the vector competence of H. marginatum for CCHFV, including tick-to-tick transmission processes such as transovarial transmission, it is assumed that this ability depends on both the tick population/species and the viral strain, as it has been well-described for other arboviruses (109, 110). Nevertheless, if CCHFV transovarial transmission is efficient, better egg survival due to higher temperature is predicted to result in a higher proportion of infected eggs. Consequently, it is possible to assert that climate change may impact both the exposure of animals to tick populations and also the level of CCHFV infection in the tick vectors. Climate change is also likely to expand the geographical range of H. marginatum northward in the Mediterranean Basin, with ticks recently being found in southwestern Europe (32, 111), as well as contribute to concomitant geographical spread of CCHFV from neighboring endemic areas. Indeed, Hyalomma ticks prefer warm summers, relatively mild winters, and reduced precipitation, which is becoming normal under the current climate change in this region (21, 112). Successful establishment of new CCHFV tick vectors in France such as H. lusitanicum or H. rufipes, due to the combined climate change and introduction events, may also occur. In relation to global warming, habitats can also change and become more or less suitable for both the survival of CCHFV tick vectors and the increased abundance of some wild mammal species, such as wild boars (113). In southern Europe, including the South of France, specific categories of xerophilous land covers such as shrubs, grasslands, and herbaceous vegetation may expand under climate change and favor the establishment of H. marginatum (103) among locally abundant small vertebrates population such as hares and rabbits (74). In addition, modifications in land use and husbandry practices, as a consequence of either climate or global changes (e.g., land conversion to pastures through deforestation), can contribute to an increase of open areas. Inversely, habitat reforestation due to agriculture decline can contribute to a higher abundance of wild ungulates populations, while fragmented habitats with wooded areas can increase their movement and the transportation of adult ticks to the new environments (30, 107). It appears that the environmental conditions are the ultimate factors that impact all the pre-cited factors.
Conclusion
France seems to be still an apparently free-disease area for CCHF, as there have never been any reports of human autochthonous cases and the virus has never been detected in ticks. However, CCHFV antibodies have been detected in domestic ruminants in Corsica Island, suggesting local transmission of the virus at least in this part of France. In this work, we have shown that H. marginatum seems to be the best candidate for this transmission, as its distribution has been increasing in the south of France, for some years, under climate changes. The known vertebrate hosts of this tick are present and numerous locally, which also enhanced its establishment. Some of these hosts such as lagomorphs or cattle are considered as good CCHFV amplifiers while others like birds or horses are not. Although the circulation of the virus seems to be possible as shown by serological evidence in cattle, the trophic preference of H. marginatum and the availability of hosts may impact the probability for H. marginatum tick vectors to become infected though what is called “the dilution effect” and therefore to efficiently transmit CCHFV. The natural enzootic transmission of CCHFV between ticks and non-human vertebrate hosts, commonly measured by seroprevalence in animals, depends on either the exposure of such animals to tick vectors or the level of infection of such ticks. These two parameters can be impacted by various factors related to the hosts, husbandry practices, habitat, and climate.
Data Availability Statement
The original contributions presented in the study are included in the article/Supplementary Material, further inquiries can be directed to the corresponding author/s.
Author Contributions
CB: bibliographic review, formal analysis, writing the original draft, and reviewing and editing the manuscript. LV: bibliographic review, formal analysis, conceptualization, reviewing and editing the manuscript, supervision, and funding acquisition. PH and VG: conceptualization and reviewing and editing the manuscript. MTB: bibliographic review and review and editing of the manuscript. FJ: scientific support and review and editing. MB and BC: funding acquisition and reviewing and editing the manuscript. All authors contributed to the article and approved the submitted version.
Funding
The authors thank the funders who made this work possible: French Ministry of Agriculture—General Directorate for Food (DGAl, grant agreement: SPA17 n◦0079-E), European Funds for Regional Development (FEDER, Grand-Est), Monitoring Outbreaks for Disease surveillance in a data science context project (MOOD project, EU H2020, grant agreement ID: 874850), French Establishment for Fighting Zoonoses (ELIZ) and the Association Nationale Recherche Technologie (ANRT, grant agreement No.: 2019-1145).
Conflict of Interest
The authors declare that the research was conducted in the absence of any commercial or financial relationships that could be construed as a potential conflict of interest.
Publisher's Note
All claims expressed in this article are solely those of the authors and do not necessarily represent those of their affiliated organizations, or those of the publisher, the editors and the reviewers. Any product that may be evaluated in this article, or claim that may be made by its manufacturer, is not guaranteed or endorsed by the publisher.
Acknowledgments
We acknowledge the working group Risk analysis for human and animal health related to Hyalomma ticks of French Agency for Food, Environmental and Occupational Health and Safety (ANSES) to which one of our co-authors participate by carrying the bibliographic search on Hyalomma ticks' vector competence. In addition, we thank Léa Souq, first year master degree student for her bibliographic work on H. marginatum hosts. We would also like to thank Iyonna Zortmann for her careful proofreading of the language.
Supplementary Material
The Supplementary Material for this article can be found online at: https://www.frontiersin.org/articles/10.3389/fvets.2022.932304/full#supplementary-material
References
1. Papa A. Emerging arboviruses of medical importance in the Mediterranean region. J Clin Virol. (2019) 115:5–10. doi: 10.1016/j.jcv.2019.03.007
2. Ergönül Ö. Crimean-Congo haemorrhagic fever. Lancet Infect Dis. (2006) 6:203–14. doi: 10.1016/S1473-3099(06)70435-2
3. Khan JA, Rehman S, Fisher-Hoch SP, Mirza S, Khurshid M, McCormick JB. Crimean congo-haemorrhagic fever treated with oral ribavirin. Lancet. (1995) 346:472–5. doi: 10.1016/S0140-6736(95)91323-8
4. Öncü S. Crimean-Congo hemorrhagic fever: an overview. Virol Sin. (2013) 28:193–201. doi: 10.1007/s12250-013-3327-4
5. Yaqub T, Oneeb M, Mukhtar N, Tahir Z, Shahid F, Subhan S, et al. Crimean–Congo Haemorrhagic Fever: case study analysis of a sporadic outbreak from Chakwal, Pakistan. Zoonoses Public Health. (2019) 66:871–3. doi: 10.1111/zph.12623
6. WHO. Crimean-Congo Haemorrhagic Fever. (2013). Available online at: https://www.who.int/news-room/fact-sheets/detail/crimean-congo-haemorrhagic-fever (accessed November 19, 2019).
7. Mardani M, Keshtkar-Jahromi M. Crimean-Congo hemorrhagic fever. Arch Iran Med. (2007) 10:204–14. Available online at: https://www.sid.ir/en/journal/ViewPaper.aspx?id=64251
8. Gargili A, Estrada-Peña A, Spengler JR, Lukashev A, Nuttall PA, Bente DA. The role of ticks in the maintenance and transmission of Crimean-Congo hemorrhagic fever virus: a review of published field and laboratory studies. Antiviral Res. (2017) 144:93–119. doi: 10.1016/j.antiviral.2017.05.010
9. Spengler JR, Estrada-Peña A. Host preferences support the prominent role of Hyalomma ticks in the ecology of Crimean-Congo hemorrhagic fever. PLoS Negl Trop Dis. (2018) 12:e0006248. doi: 10.1371/journal.pntd.0006248
10. Spengler JR, Estrada-Peña A, Garrison AR, Schmaljohn C, Spiropoulou CF, Bergeron É, et al. Chronological review of experimental infection studies of the role of wild animals and livestock in the maintenance and transmission of Crimean-Congo hemorrhagic fever virus. Antiviral Res. (2016) 135:31–47. doi: 10.1016/j.antiviral.2016.09.013
11. Nasirian H. Crimean-Congo hemorrhagic fever (CCHF) seroprevalence: a systematic review and meta-analysis. Acta Trop. (2019) 196:102–20. doi: 10.1016/j.actatropica.2019.05.019
12. Stavropoulou E, Troillet N. Fièvre hémorragique de Crimée-Congo : une maladie virale émergente en Europe. Rev Med Suisse. (2018) 14:1786–9. doi: 10.53738/REVMED.2018.14.622.1786
13. Papa A, Sidira P, Larichev V, Gavrilova L, Kuzmina K, Mousavi-Jazi M, et al. Crimean-congo hemorrhagic fever virus, Greece. Emerg Infect Dis. (2014) 20:288–90. doi: 10.3201/eid2002.130690
14. Hoogstraal H. The epidemiology of tick-borne Crimean-Congo hemorrhagic fever in Asia, Europe, and Africa. J Med Entomol. (1979) 15:307–417. doi: 10.1093/jmedent/15.4.307
15. Estrada-Peña A, Zatansever Z, Gargili A, Aktas M, Uzun R, Ergonul O, et al. Modeling the spatial distribution of crimean-congo hemorrhagic fever outbreaks in Turkey. Vect Borne Zoonot Dis. (2007) 7:667–78. doi: 10.1089/vbz.2007.0134
16. Vial L, Stachurski F, Leblond A, Huber K, Vourc'h G, René-Martellet M, et al. Strong evidence for the presence of the tick Hyalomma marginatum Koch, 1844 in southern continental France. Ticks Tick Borne Dis. (2016) 7:1162–7. doi: 10.1016/j.ttbdis.2016.08.002
17. Grech-Angelini S, Stachurski F, Lancelot R, Boissier J, Allienne J-F, Marco S, et al. Ticks (Acari: Ixodidae) infesting cattle and some other domestic and wild hosts on the French Mediterranean island of Corsica. Parasit Vect. (2016) 9:582. doi: 10.1186/s13071-016-1876-8
18. De Liberato C, Frontoso R, Magliano A, Montemaggiori A, Autorino GL, Sala M, et al. Monitoring for the possible introduction of Crimean-Congo haemorrhagic fever virus in Italy based on tick sampling on migratory birds and serological survey of sheep flocks. Prev Vet Med. (2018) 149:47–52. doi: 10.1016/j.prevetmed.2017.10.014
19. Schuster I, Chaintoutis SC, Dovas CI, Groschup MH, Mertens M. Detection of Crimean-Congo hemorrhagic fever virus-specific IgG antibodies in ruminants residing in Central and Western Macedonia, Greece. Ticks Tick Borne Dis. (2017) 8:494–8. doi: 10.1016/j.ttbdis.2017.02.009
20. Morel PC. Les Tiques d'Afrique et du Bassin méditerranéen. Bull Académie Vét Fr. (2003) 1–1342. doi: 10.4267/2042/66807
21. Mertens M, Schmidt K, Ozkul A, Groschup MH. The impact of Crimean-Congo hemorrhagic fever virus on public health. Antiviral Res. (2013) 98:248–60. doi: 10.1016/j.antiviral.2013.02.007
22. Christova I, Panayotova E, Groschup MH, Trifonova I, Tchakarova S, Sas MA. High seroprevalence for Crimean–Congo haemorrhagic fever virus in ruminants in the absence of reported human cases in many regions of Bulgaria. Exp Appl Acarol. (2018) 75:227–34. doi: 10.1007/s10493-018-0258-7
23. Grech-Angelini S, Lancelot R, Ferraris O, Peyrefitte CN, Vachiery N, Pédarrieu A, et al. Crimean-Congo hemorrhagic fever virus antibodies among livestock on Corsica, France, 2014–2016. Emerg Infect Dis. (2020) 26:1041–4. doi: 10.3201/10.3201/eid2605.191465
24. Esser HJ, Mögling R, Cleton NB, van der Jeugd H, Sprong H, Stroo A, et al. Risk factors associated with sustained circulation of six zoonotic arboviruses: a systematic review for selection of surveillance sites in non-endemic areas. Parasit Vect. (2019) 12:265. doi: 10.1186/s13071-019-3515-7
25. Hoch T, Breton E, Josse M, Deniz A, Guven E, Vatansever Z. Identifying main drivers and testing control strategies for CCHFV spread. Exp Appl Acarol. (2016) 68:347–59. doi: 10.1007/s10493-015-9937-9
26. Hoch T, Breton E, Vatansever Z. Dynamic modeling of Crimean Congo Hemorrhagic Fever Virus (CCHFV) spread to test control strategies. J Med Entomol. (2018) 55:1124–32. doi: 10.1093/jme/tjy035
27. Ak Ç, Ergönül Ö, Gönen M. A prospective prediction tool for understanding Crimean–Congo haemorrhagic fever dynamics in Turkey. Clin Microbiol Infect. (2019) 26:123.e1–7. doi: 10.1016/j.cmi.2019.05.006
28. Goldfarb LG, Chumakov MP, Myskin AA, Kondratenko VF, Reznikova OY. An epidemiological model of Crimean hemorrhagic fever. Am J Trop Med Hyg. (1980) 29:260–4. doi: 10.4269/ajtmh.1980.29.260
29. Switkes J, Nannyonga B, Mugisha JYT, Nakakawa J. A mathematical model for Crimean-Congo haemorrhagic fever: tick-borne dynamics with conferred host immunity. J Biol Dyn. (2016) 10:59–70. doi: 10.1080/17513758.2015.1102976
30. Estrada-Peña A, Jameson L, Medlock J, Vatansever Z, Tishkova F. Unraveling the ecological complexities of tick-associated Crimean-Congo hemorrhagic fever virus transmission: a gap analysis for the Western Palearctic. Vect Borne Zoon Dis. (2012) 12:743–52. doi: 10.1089/vbz.2011.0767
31. Dereli AS, Kayser V. Crimean–Congo hemorrhagic fever: a bibliometric assessment of the literature. J Infect Public Health. (2018) 11:147–8. doi: 10.1016/j.jiph.2017.06.010
32. Andersen LK, Davis MDP. Climate change and the epidemiology of selected tick-borne and mosquito-borne diseases: update from the International Society of Dermatology Climate Change Task Force. Int J Dermatol. (2017) 56:252–9. doi: 10.1111/ijd.13438
33. Phonera MC, Simuunza MC, Kainga H, Ndebe J, Chembensofu M, Chatanga E, et al. Seroprevalence and risk factors of Crimean-Congo hemorrhagic fever in cattle of smallholder farmers in central Malawi. Pathog Basel Switz. (2021) 10:1613. doi: 10.3390/pathogens10121613
34. Moraga-Fernández A, Ruiz-Fons F, Habela MA, Royo-Hernández L, Calero-Bernal R, Gortazar C, et al. Detection of new Crimean–Congo haemorrhagic fever virus genotypes in ticks feeding on deer and wild boar, Spain. Transbound Emerg Dis. (2021) 68:993–1000. doi: 10.1111/tbed.13756
35. Ibrahim AM, Adam IA, Osman BT, Aradaib IE. Epidemiological survey of Crimean Congo hemorrhagic fever virus in cattle in East Darfur State, Sudan. Ticks Tick Borne Dis. (2015) 6:439–44. doi: 10.1016/j.ttbdis.2015.03.002
36. Turell MJ. Role of ticks in the transmission of crimean-congo hemorrhagic fever virus. In: Ergonul O, Whitehouse CA, editors. Crimean-Congo Hemorrhagic Fever: A Global Perspective. Dordrecht: Springer (2007). p. 143–54. doi: 10.1007/978-1-4020-6106-6_12
37. Nalca A, Whitehouse C. Crimean-Congo hemorrhagic fever virus infection among animals. In: Crimean-Congo Hemorrhagic Fever: A Global Perspective. Amsterdam: Springer (2007). p. 155–65. doi: 10.1007/978-1-4020-6106-6_13
38. Boulanger N, McCoy KD, (eds),. Tiques et maladies à tiques : Biologie, écologie évolutive, épidémiologie. Marseille: IRD Éditions (2017). p. 336–8. Available online at: http://books.openedition.org/irdeditions/9001 (accessed April 24, 2022).
39. Camicas J-L, Cornet J-P, Gonzalez J-P, Wilson ML, Adam F, Zeller HG. La fièvre hémorragique de Crimée-Congo au Sénégal : dernières données sur l'écologie du virus CCHF. (1994) 6 p.
40. Khan AS, Maupin GO, Rollin PE, Noor AM, Shurie HH, Shalabi AG, et al. An outbreak of Crimean-Congo hemorrhagic fever in the United Arab Emirates, 1994-1995. Am J Trop Med Hyg. (1997) 57:519–25. doi: 10.4269/ajtmh.1997.57.519
41. Rageau J. Répartition géographique et rôle pathogène des tiques (acariens : Argasidae et Ixodidae) en France. (1972) 13 p.
42. Morel P-C. Les Hyalomma (Acariens, Ixodidæ) de France. Ann Parasitol Hum Comparée. (1959) 34:552–5. doi: 10.1051/parasite/1959344552
43. Valcárcel F, González J, González MG, Sánchez M, Tercero JM, Elhachimi L, et al. Comparative ecology of Hyalomma lusitanicum and Hyalomma marginatum Koch, 1844 (Acarina: Ixodidae). Insects. (2020) 11:303. doi: 10.3390/insects11050303
44. Giban J, Barthélémy J, Aubry J. L'épizootie de myxomatose en France chez le lapin de garenne: (Oryctolagus cuniculus L.). 13 p.
45. Macaigne F, Perez-Eid C. Hyalomma scupense Schulze, 1919 (Acarina, Ixodoidea) tique autochtone du Sud-Ouest de la France. Ann Parasitol Hum Comparée. (1993) 68:199–200. doi: 10.1051/parasite/1993684199
46. Cicculli V, Oscar M, Casabianca F, Villechenaud N, Charrel R, de Lamballerie X, et al. Molecular detection of spotted-fever group rickettsiae in ticks collected from domestic and wild animals in Corsica, France. Pathog Basel Switz. (2019) 8:E138. doi: 10.3390/pathogens8030138
47. Kondratenko V, Blagoveschchenskaya N, Butenko A, Vyshnivetskaya L, Zarubina L, Milyutin V, et al. Results of virological investigation of ixodid ticks in Crimean hemorrhagic fever focus in Rostov Oblast. Crime Hemorr Fever. (1970) 10:29–35.
48. Sherifi K, Cadar D, Muji S, Robaj A, Ahmeti S, Jakupi X, et al. Crimean-Congo hemorrhagic fever virus Clades V and VI (Europe 1 and 2) in Ticks in Kosovo, 2012. PLoS Negl Trop Dis. (2014) 8:e3168. doi: 10.1371/journal.pntd.0003168
49. Gevorgyan H, Grigoryan GG, Atoyan HA, Rukhkyan M, Hakobyan A, Zakaryan H, et al. Evidence of Crimean-Congo haemorrhagic fever virus occurrence in ixodidae ticks of Armenia. J Arthropod-Borne Dis. (2019) 13:9–16. doi: 10.18502/jad.v13i1.928
50. Yaser SA, Sadegh C, Zakkyeh T, Hassan V, Maryam M, Ali OM, et al. Crimean–Congo hemorrhagic fever: a molecular survey on hard ticks (Ixodidae) in Yazd province, Iran. Asian Pac J Trop Med. (2011) 4:61–3. doi: 10.1016/S1995-7645(11)60034-5
51. Yesilbag K, Aydin L, Dincer E, Alpay G, Girisgin AO, Tuncer P, et al. Tick survey and detection of Crimean-Congo hemorrhagic fever virus in tick species from a non-endemic area, South Marmara region, Turkey. Exp Appl Acarol. (2013) 60:253–61. doi: 10.1007/s10493-012-9642-x
52. Orkun Ö, Karaer Z, Çakmak A, Nalbantoglu S. Crimean-Congo hemorrhagic fever virus in ticks in Turkey: a broad range tick surveillance study. Infect Genet Evol. (2017) 52:59–66. doi: 10.1016/j.meegid.2017.04.017
53. Grech-Angelini S, Stachurski F, Lancelot R, Boissier J, Allienne J-F, Gharbi M, et al. First report of the tick Hyalomma scupense (natural vector of bovine tropical theileriosis) on the French Mediterranean island of Corsica. Vet Parasitol. (2016) 216:33–7. doi: 10.1016/j.vetpar.2015.11.015
54. Rocafort-Ferrer G, Leblond A, Joulié A, René-Martellet M, Sandoz A, Poux V, et al. Molecular assessment of Theileria equi and Babesia caballi prevalence in horses and ticks on horses in southeastern France. Parasitol Res. (2022) 121:999–1008. doi: 10.1007/s00436-022-07441-7
55. Spengler JR, Bergeron É, Rollin PE. Seroepidemiological Studies of Crimean-Congo hemorrhagic fever virus in domestic and wild animals. PLoS Negl Trop Dis. (2016) 10:e0004210. doi: 10.1371/journal.pntd.0004210
56. Schuster I, Mertens M, Mrenoshki S, Staubach C, Mertens C, Brüning F, et al. Sheep and goats as indicator animals for the circulation of CCHFV in the environment. Exp Appl Acarol. (2016) 68:337–46. doi: 10.1007/s10493-015-9996-y
57. Hoogstraal H, Aeschlimann A. Tick-host specificity. Bull Société Entomol Suisse. (1982) 55:5–32.
58. Klompen JSH, Black WC, Keirans JE, Oliver JH. Evolution of ticks. Annu Rev Entomol. (1996) 41:141–61. doi: 10.1146/annurev.en.41.010196.001041
59. Gargili A, Thangamani S, Bente D. Influence of laboratory animal hosts on the life cycle of Hyalomma marginatum and implications for an in vivo transmission model for Crimean-Congo hemorrhagic fever virus. Front Cell Infect Microbiol. (2013) 3:39. doi: 10.3389/fcimb.2013.00039
60. Tiar G, Tiar-Saadi M, Benyacoub S, Rouag R, Široký P. The dependence of Hyalomma aegyptium on its tortoise host Testudo graeca in Algeria. Med Vet Entomol. (2016) 30:351–9. doi: 10.1111/mve.12175
61. Valcárcel F, González J, Tercero-Jaime JM, Olmeda AS. The effect of excluding ungulates on the abundance of ixodid ticks on wild rabbit (Oryctolagus cuniculus). Exp Appl Acarol. (2017) 72:439–47. doi: 10.1007/s10493-017-0166-2
62. Kanchev K, Kamenov Y, Atanassova I, Davidova R, Tomov R. Parasitic alien terrestrial arthropods on small mammals in Northeast and South Bulgaria. Bulg J Agric Sci Bulg. (2012) 18:965–70. Available online at: https://www.agrojournal.org/18/06-20-12.pdf
63. Ionita M, Mitrea IL, Pfister K, Hamel D, Silaghi C. Molecular evidence for bacterial and protozoan pathogens in hard ticks from Romania. Vet Parasitol. (2013) 196:71–6. doi: 10.1016/j.vetpar.2013.01.016
64. Koc S, Aydin L, Cetin H. Tick species (Acari: Ixodida) in Antalya City, Turkey: species diversity and seasonal activity. Parasitol Res. (2015) 114:2581–6. doi: 10.1007/s00436-015-4462-7
65. James GH, Dinulos M. Infestations and bites. In: Habif's Clinical Dermatology. (2021). Available online at: https://www.clinicalkey.com/#!/content/book/3-s2.0-B9780323612692000150 (accessed April 11, 202).
66. Elhachimi L, Valcárcel F, Olmeda AS, Elasatey S, Khattat SE, Daminet S, et al. Rearing of Hyalomma marginatum (Acarina: Ixodidae) under laboratory conditions in Morocco. Exp Appl Acarol. (2021) 84:785–94. doi: 10.1007/s10493-021-00641-3
67. Pascucci I, Di Domenico M, Capobianco Dondona G, Di Gennaro A, Polci A, Capobianco Dondona A, et al. Assessing the role of migratory birds in the introduction of ticks and tick-borne pathogens from African countries: An Italian experience. Ticks Tick-Borne Dis. (2019) 10:101–272. doi: 10.1016/j.ttbdis.2019.101272
68. Grard G, Drexler JF, Fair J, Muyembe J-J, Wolfe ND, Drosten C, et al. Re-emergence of Crimean-Congo hemorrhagic fever virus in Central Africa. PLoS Negl Trop Dis. (2011) 5:e1350. doi: 10.1371/journal.pntd.0001350
69. Portillo A, Palomar AM, Santibáñez P, Oteo JA. Epidemiological Aspects of Crimean-Congo Hemorrhagic Fever in Western Europe: what about the Future? Microorganisms. (2021) 9:649. doi: 10.3390/microorganisms9030649
70. Spengler JR, Bergeron É, Spiropoulou CF. Crimean-Congo hemorrhagic fever and expansion from endemic regions. Curr Opin Virol. (2019) 34:70–8. doi: 10.1016/j.coviro.2018.12.002
71. Espunyes J, Cabezón O, Pailler-García L, Dias-Alves A, Lobato-Bailón L, Marco I, et al. Hotspot of Crimean-Congo hemorrhagic fever virus seropositivity in wildlife, Northeastern Spain. Emerg Infect Dis. (2021) 27:2480–4. doi: 10.3201/eid2709.211105
72. Hars J, Rossi S, Faure E, Taconet A-E, Gay P, Landelle P, et al. Risques sanitaires liés à l'importation de gibier sauvage d'élevage et de repeuplement [French]. (2015) 4.
73. González-Redondo P, Sánchez-Martínez R. Characterisation of wild rabbit commercial game farms in Spain. World Rabbit Sci. (2014) 22:51. doi: 10.4995/wrs.2014.1213
74. Letty J, Olivier A, Tatin L, Galaup O. Lièvre France. In: Atlas des mammifères sauvages de France volume 2. Paris: Publications scientifiques du Muséum national d'Histoire naturelle (2021).
75. Nabeth P, Cheikh DO, Lo B, Faye O, Vall IOM, Niang M, et al. Crimean-Congo hemorrhagic fever, Mauritania. Emerg Infect Dis. (2004) 10:2143–9. doi: 10.3201/eid1012.040535
76. Zeller HG, Cornet JP, Camicas JL. Crimean-Congo haemorrhagic fever virus infection in birds: field investigations in Senegal. Res Virol. (1994) 145:105–9. doi: 10.1016/S0923-2516(07)80012-4
77. Swanepoel R, Leman PA, Burt FJ, Jardine J, Verwoerd DJ, Capua I, et al. Experimental infection of ostriches with Crimean–Congo haemorrhagic fever virus. Epidemiol Infect. (1998) 121:427–32. doi: 10.1017/S0950268898001344
78. Capua I. Crimean-Congo haemorrhagic fever in ostriches: a public health risk for countries of the European Union? Avian Pathol. (1998) 27:117–20. doi: 10.1080/03079459808419311
80. Kaiser MN, Hoogstraal H, Watson GE. Ticks (Ixodoidea) on migrating birds in Cyprus, fall 1967 and spring 1968, and epidemiological considerations*. Bull Entomol Res. (1974) 64:97–110. doi: 10.1017/S0007485300027024
81. Palomar AM, Portillo A, Mazuelas D, Roncero L, Arizaga J, Crespo A, et al. Molecular analysis of Crimean-Congo hemorrhagic fever virus and Rickettsia in Hyalomma marginatum ticks removed from patients (Spain) and birds (Spain and Morocco), 2009–2015. Ticks Tick Borne Dis. (2016) 7:983–7. doi: 10.1016/j.ttbdis.2016.05.004
83. Wilson ML, Gonzalez J-P, Cornet J-P, Camicas J-L. Transmission of crimean-congo haemorrhagic fever virus from experimentally infected sheep to hyalomma truncatum ticks. Res Virol. (1991) 142:395–404. doi: 10.1016/0923-2516(91)90007-P
84. Crimean-Congo Hemorrhagic F. 2011-2015. Emerg Infect Dis. (2019) 25:1177–84. doi: 10.3201/eid2506.180877
85. Gordon SW, Linthicum KJ, Moulton JR. Transmission of Crimean-Congo hemorrhagic fever virus in two species of Hyalomma ticks from infected adults to cofeeding immature forms. Am J Trop Med Hyg. (1993) 48:576–80. doi: 10.4269/ajtmh.1993.48.576
86. LoGiudice K, Ostfeld RS, Schmidt KA, Keesing F. The ecology of infectious disease: effects of host diversity and community composition on Lyme disease risk. Proc Natl Acad Sci USA. (2003) 100:567–71. doi: 10.1073/pnas.0233733100
87. Msimang V, Weyer J, le Roux C, Kemp A, Burt FJ, Tempia S, et al. Risk factors associated with exposure to Crimean-Congo haemorrhagic fever virus in animal workers and cattle, and molecular detection in ticks, South Africa. PLoS Negl Trop Dis. (2021) 15:e0009384. doi: 10.1371/journal.pntd.0009384
88. Kasi KK, Sas MA, Sauter-Louis C, von Arnim F, Gethmann JM, Schulz A, et al. Epidemiological investigations of Crimean-Congo haemorrhagic fever virus infection in sheep and goats in Balochistan, Pakistan. Ticks Tick-Borne Dis. (2020) 11:101324. doi: 10.1016/j.ttbdis.2019.101324
89. Adam IA, Mahmoud MAM, Aradaib IE. A seroepidemiological survey of Crimean Congo hemorrhagic fever among cattle in North Kordufan State, Sudan. Virol J. (2013) 10:178. doi: 10.1186/1743-422X-10-178
90. Suliman HM, Adam IA, Saeed SI, Abdelaziz SA, Haroun EM, Aradaib IE. Crimean Congo hemorrhagic fever among the one-humped camel (Camelus dromedaries) in Central Sudan. Virol J. (2017) 14:147. doi: 10.1186/s12985-017-0816-3
91. Balinandi S, von Brömssen C, Tumusiime A, Kyondo J, Kwon H, Monteil VM, et al. Serological and molecular study of Crimean-Congo Hemorrhagic Fever Virus in cattle from selected districts in Uganda. J Virol Methods. (2021) 290:114075. doi: 10.1016/j.jviromet.2021.114075
92. Guilherme JM, Gonella-Legall C, Legall F, Nakoume E, Vincent J. Seroprevalence of five arboviruses in Zebu cattle in the Central African Republic. Trans R Soc Trop Med Hyg. (1996) 90:31–3. doi: 10.1016/S0035-9203(96)90468-X
93. Lotfollahzadeh S, Nikbakht Boroujeni GHR, Mokhber Dezfouli MR, Bokaei S. A serosurvey of Crimean-Congo haemorrhagic fever virus in dairy cattle in iran: serosurvey of Crimean-Congo haemorrhagic fever virus in dairy cattle. Zoonoses Public Health. (2011) 58:54–9. doi: 10.1111/j.1863-2378.2009.01269.x
94. Ozan E, Ozkul A. Investigation of Crimean-Congo hemorrhagic fever virus in ruminant species slaughtered in several endemic provinces in Turkey. Arch Virol. (2020) 165:1759–67. doi: 10.1007/s00705-020-04665-9
95. Schulz A, Barry Y, Stoek F, Pickin MJ, Ba A, Chitimia-Dobler L, et al. Detection of crimean-congo hemorrhagic fever virus in blood-fed hyalomma ticks collected from mauritanian livestock. Parasit Vectors. (2021) 14:342. doi: 10.1186/s13071-021-04819-x
96. Wilson M, Desoutter D, Gonzalez J-P, Camicas J-L, Guillaud ML, LeGuenno B. Distribution of Crimean-Congo hemorrhagic fever viral antibody in senegal: environmental and vectorial correlates. Am J Trop Med Hyg. (1990) 43:557–66. doi: 10.4269/ajtmh.1990.43.557
97. Zohaib A, Saqib M, Athar MA, Hussain MH, Sial A-R, Tayyab MH, et al. Crimean-Congo hemorrhagic fever virus in humans and livestock, Pakistan, 2015–2017. Emerg Infect Dis. (2020) 26:5. doi: 10.3201/eid2604.191154
98. Burt FJ, Leman PA, Abbott JC, Swanepoel R. Serodiagnosis of Crimean-Congo haemorrhagic fever. Epidemiol Infect. (1994) 113:551–62. doi: 10.1017/S0950268800068576
99. Vor T, Kiffner C, Hagedorn P, Niedrig M, Rühe F. Tick burden on European roe deer (Capreolus capreolus). Exp Appl Acarol. (2010) 51:405–17. doi: 10.1007/s10493-010-9337-0
100. Tabor AE, Ali A, Rehman G, Rocha Garcia G, Zangirolamo AF, Malardo T, et al. Cattle tick Rhipicephalus microplus-host interface: a review of resistant and susceptible host responses. Front Cell Infect Microbiol. (2017) 7:506. doi: 10.3389/fcimb.2017.00506
101. Yessinou RE, Adoligbe C, Akpo Y, Adinci J, Youssao Abdou Karim I, Farougou S. Sensitivity of different cattle breeds to the infestation of cattle ticks Amblyomma variegatum, Rhipicephalus microplus, and Hyalomma spp. on the Natural Pastures of Opkara Farm, Benin. J Parasitol Res. (2018) 2018:1–9. doi: 10.1155/2018/2570940
102. Nwachukwu EN, Ogbu CC, Edozie C, Oke UK, Ojewola GS, Ekumankama OO. Incidence of tick infestation in mixed breeding herd of indigenous cattle in a rainforest agro-ecological zone. Livestock Res Rural Develop. (2020) 32. Available online at: http://www.lrrd.org/lrrd32/1/cosch32004.html (accessed June 29, 2022).
103. Bah MT, Grosbois V, Stachurski F, Muñoz F, Duhayon M, Rakotoarivony I, et al. The Crimean-Congo haemorrhagic fever tick vector Hyalomma marginatum in the south of France: Modelling its distribution and determination of factors influencing its establishment in a newly invaded area. Transbound Emerg Dis. (2021). doi: 10.1111/tbed.14578 [Epub ahead of print].
104. Hassanein KM, El-Azazy OME, Yousef HM. Detection of Crimean-Congo haemorrhagic fever virus antibodies in humans and imported livestock in Saudi Arabia. Trans R Soc Trop Med Hyg. (1997) 91:536–7. doi: 10.1016/S0035-9203(97)90014-6
105. Jameson LJ, Ramadani N, Medlock JM. Possible drivers of Crimean-Congo hemorrhagic fever virus transmission in Kosova. Vect Borne Zoon Dis. (2012) 12:753–7. doi: 10.1089/vbz.2011.0773
106. Kulichenko AN, Prislegina DA. Climatic prerequisites for changing activity in the natural Crimean-Congo hemorrhagic fever focus in the South of the Russian Federation. Russ J Infect Immun. (2019) 9:162–72. doi: 10.15789/2220-7619-2019-1-162-172
107. Vescio FM, Busani L, Mughini-Gras L, Khoury C, Avellis L, Taseva E, et al. Environmental correlates of crimean-congo haemorrhagic fever incidence in Bulgaria. BMC Public Health. (2012) 12:1116. doi: 10.1186/1471-2458-12-1116
108. Estrada-Peña A, Ruiz-Fons F, Acevedo P, Gortazar C, de la Fuente J. Factors driving the circulation and possible expansion of Crimean-Congo haemorrhagic fever virus in the western Palearctic. J Appl Microbiol. (2013) 114:278–86. doi: 10.1111/jam.12039
109. Ciota AT, Bialosuknia SM, Zink SD, Brecher M, Ehrbar DJ, Morrissette MN, et al. Effects of Zika virus strain and aedes mosquito species on vector competence. Emerg Infect Dis. (2017) 23:1110–7. doi: 10.3201/eid2307.161633
110. Pereira De Oliveira R, Hutet E, Lancelot R, Paboeuf F, Duhayon M, Boinas F, et al. Differential vector competence of Ornithodoros soft ticks for African swine fever virus: what if it involves more than just crossing organic barriers in ticks? Parasit Vect. (2020) 13:618. doi: 10.1186/s13071-020-04497-1
111. Gale P, Stephenson B, Brouwer A, Martinez M, de la Torre A, Bosch J, et al. Impact of climate change on risk of incursion of Crimean-Congo haemorrhagic fever virus in livestock in Europe through migratory birds. J Appl Microbiol. (2012) 112:246–57. doi: 10.1111/j.1365-2672.2011.05203.x
112. Dreshaj S, Ahmeti S, Ramadani N, Dreshaj G, Humolli I, Dedushaj I. Current situation of Crimean-Congo hemorrhagic fever in Southeastern Europe and neighboring countries: a public health risk for the European Union? Travel Med Infect Dis. (2016) 14:81–91. doi: 10.1016/j.tmaid.2016.03.012
Keywords: CCHF, Hyalomma tick vectors, vertebrate host communities, viral transmission cycle, France
Citation: Bernard C, Holzmuller P, Bah MT, Bastien M, Combes B, Jori F, Grosbois V and Vial L (2022) Systematic Review on Crimean–Congo Hemorrhagic Fever Enzootic Cycle and Factors Favoring Virus Transmission: Special Focus on France, an Apparently Free-Disease Area in Europe. Front. Vet. Sci. 9:932304. doi: 10.3389/fvets.2022.932304
Received: 29 April 2022; Accepted: 15 June 2022;
Published: 19 July 2022.
Edited by:
Sara Moutailler, Agence Nationale de Sécurité Sanitaire de l'Alimentation, de l'Environnement et du Travail (ANSES), FranceReviewed by:
Francisco Ruiz-Fons, Spanish National Research Council (CSIC), SpainAli Mirazimi, Karolinska University Hospital, Sweden
Copyright © 2022 Bernard, Holzmuller, Bah, Bastien, Combes, Jori, Grosbois and Vial. This is an open-access article distributed under the terms of the Creative Commons Attribution License (CC BY). The use, distribution or reproduction in other forums is permitted, provided the original author(s) and the copyright owner(s) are credited and that the original publication in this journal is cited, in accordance with accepted academic practice. No use, distribution or reproduction is permitted which does not comply with these terms.
*Correspondence: Célia Bernard, Y2VsaWEuYmVybmFyZEBjaXJhZC5mcg==