- College of Animal Science, Anhui Science and Technology University, Chuzhou, China
Fc gamma receptor-mediated antibody-dependent enhancement (ADE) can promote virus invasion of target cells, sometimes exacerbating the severity of the disease. ADE may be an enormous hurdle to developing efficacious vaccines for certain human and animal viruses. ADE of porcine reproductive and respiratory syndrome virus (PRRSV) infection has been demonstrated in vivo and in vitro. However, the effect of PRRSV-ADE infection on the natural antiviral immunity of the host cells is yet to be well investigated. Specifically, whether the ADE of PRRSV infection affects the levels of type II (interferon-gamma, IFN-γ) and III (interferon-lambdas, IFN-λs) interferons (IFNs) remains unclear. In this study, our results showed that PRRSV significantly induced the secretion of IFN-γ, IFN-λ1, IFN-λ3, and IFN-λ4 in porcine alveolar macrophages (PAMs) in early infection, and weakly inhibited the production of IFN-γ, IFN-λ1, IFN-λ3, and IFN-λ4 in PAMs in late infection. Simultaneously, PRRSV infection significantly increased the transcription of interferon-stimulated gene 15 (ISG15), ISG56, and 2′, 5′-oligoadenylate synthetase 2 (OAS2) in PAMs. In addition, our results showed that PRRSV infection in PAMs via the ADE pathway not only significantly decreased the synthesis of IFN-γ, IFN-λ1, IFN-λ3, and IFN-λ4 but also significantly enhanced the generation of transforming growth factor-beta1 (TGF-β1). Our results also showed that the ADE of PRRSV infection significantly reduced the mRNAs of ISG15, ISG56, and OAS2 in PAMs. In conclusion, our studies indicated that PRRSV-ADE infection suppressed innate antiviral response by downregulating the levels of type II and III IFNs, hence facilitating viral replication in PAMs in vitro. The ADE mechanism demonstrated in the present study furthered our understanding of persistent pathogenesis following PRRSV infection mediated by antibodies.
Introduction
Porcine reproductive and respiratory syndrome (PRRS) is a highly contagious disease of pigs, which first emerged in North America in the late 1980s and subsequently in Western Europe in the early 1990s (1). PRRS is a significant threat to swine health. It is best characterized by severe respiratory disorders and high mortality in piglets, preterm birth, fetal death, late-term abortions, and mummified fetuses in pregnant sows, resulting in devastating economic losses to the modern pig industry worldwide for over three decades (2, 3). PRRS virus (PRRSV), as the etiological agent of this disease, is an enveloped virus containing one single-stranded positive RNA genome of ~15.4 kb in length. This virus, together with the equine arteritis virus, simian hemorrhagic fever virus, and mouse lactate dehydrogenase-elevating virus, belongs to the Arteriviridae family within the order Nidovirales (4). All PRRSV strains are grouped into two species: PRRSV-1 (European or type 1 genotype) and PRRSV-2 (North American or type 2 genotype). Interestingly, although both genotypes show only ~60% identity at the nucleotide levels of genomic sequences with a high degree of antigenic divergence, they lead to similar clinical manifestations in infected pigs (5, 6). Swine are the only known natural hosts of PRRSV. The porcine monocyte-macrophage lineage, particularly monocyte-derived inflammatory dendritic cells, and differentiated macrophages, such as porcine alveolar macrophages (PAMs), are the PRRSV principal permissive cells (7, 8). In addition, the immortalized monkey kidney epithelial cell line, Marc-145 cells, can be infected by PRRSV (9).
Antibody-dependent enhancement (ADE), an immunopathological phenomenon in which preexisting specific non- or sub-neutralizing antibodies enhance the entry and replication of the virus in the host cells, has been described for many viruses, including PRRSV (10). As early as 1993, Christianson et al. reported that PRRSV infectivity increased in fetuses by adding porcine serum containing PRRSV antibodies (11). Later, Yoon et al. directly revealed the presence of PRRSV-ADE in vivo by injecting swine with PRRSV-specific antibodies. The duration of viremia is more significant in pigs injected with sub-neutralizing anti-PRRSV immunoglobulin G (IgG) before the virus challenge than in control pigs injected with normal IgG (12). The prolonged duration of viremia and the isolation of the virus from the tissues of piglets with low maternal antibodies ulteriorly provide evidence of in vivo PRRSV-ADE activity (13). In vitro, the enhanced replication of PRRSV in the presence of sub-neutralizing specific antibodies against PRRSV has also been confirmed (14, 15). These studies show that ADE is likely to increase the severity of PRRS and the susceptibility to PRRSV in pigs with declining maternal antibodies for PRRSV or with low levels of specific antibodies induced by exposure to wild-type or vaccine virus strains. Currently, commercially available PRRS vaccines provide insufficient protection against PRRSV, especially emerging and heterologous field virus strains (16–18). The immunological effect of PRRS vaccines is impacted by multitudinous host factors, in which ADE may be one crucial factor (19, 20). Vaccine-induced enhancement of susceptibility to virus infection or aberrant viral pathogenesis is also a significant obstacle in developing certain paramyxo-, corona-, flavi-, and lentivirus vaccines (21, 22). Therefore, more effective prevention and control strategies for PRRSV are still needed. A clear understanding of the ADE event could prevent the disease.
The innate immune response is the first line of host protection against viral infections. The suppression of intracellular antiviral immune signals by the virus using the ADE mechanism plays a vital role in the pathogenesis of persistent infection of the disease (23–25). PRRSV modulates host innate antiviral immunity by modifying the expression patterns of crucial antiviral cytokines such as interferons (IFNs) (26, 27). However, the roles of PRRSV-ADE in the natural immune defense system of the host are not well understood. In this study, we assessed the effect of PRRSV-ADE infection on the production of type II (IFN-γ) and III (IFN-λ1, IFN-λ3, and IFN-λ4) IFNs, and surveyed the transcription expression of several critical downstream antiviral protein genes (interferon-stimulated gene 15, ISG15; ISG56; and 2′, 5′-oligoadenylate synthetase 2, OAS2). The current study suggests that PRRSV infection suppressed IFN-γ/λs antiviral responses via the antibody-dependent pathway in PAMs in vitro. These results would help facilitate the understanding of PRRSV-persistent pathogenesis and antiviral vaccination strategies.
Materials and methods
Cells
Marc-145 cells maintained in Dulbecco's modified Eagle's medium (Sangon Biotech, Shanghai, China) supplemented with 10% fetal calf serum (FCS) (Tianhang Biotech, Huzhou, China) were used to propagate PRRSV and titrate its 50% tissue culture infectious dose (TCID50) employing the Reed–Muench method. PAMs derived from 3 to 6-week-old PRRSV-negative piglets using lung lavage were cultivated in a Roswell Park Memorial Institute (RPMI)-1640 medium (Sangon Biotech) containing 10% FCS plus 100 μg/ml streptomycin and 100 U/ml penicillin (Sangon Biotech). These two cells were kept in a humidified atmosphere with 5% CO2 at 37°C before use.
Virus and antibodies
The type 2 PRRSV HeN-3 strain used in the current study was a kind gift from Prof. Pingan Xia of Henan Agricultural University. The inactivated purified HeN-3 virus particles were used to immunize pigs to produce PRRSV-positive sera (Enzyme-linked immunosorbent assay (ELISA) titer: 6400). PRRSV-negative serum samples were collected from PRRSV-negative healthy piglets. The IgG antibodies were depurated using diethyl-aminoethanol chromatography.
Preparation of infectious PRRSV–antibody complexes
Approximately 2000 TCID50/ml of PRRSV was fully incubated with an equal volume of 850 μg/ml of purified PRRSV-positive IgG (PPI) or PRRSV-negative IgG (PNI) for 1 h at 37°C to yield infectious virus–antibody complexes (PRRSV+PPI) or controls (PRRSV+PNI), respectively.
PRRSV or PRRSV-ADE infection in PAMs
Six hours before infection, PAMs were dispensed into 24-well plates (Sangon Biotech) at a density of 5 × 105 cells/well. After discarding the culture solutions and washing the cells gently three times with FCS-free RPMI-1640, 200 μl poly (I:C) (100 μg/ml) (Sigma, Missouri, USA), PRRSV (200 TCID50), PRRSV+PNI, or PRRSV+PPI was inoculated onto the cell monolayers at 37°C for 2 h. Then, the inoculum was removed, and 500 μl fresh growth media was added. The uninfected cells served as mock trials. The cells of each well and culture supernatants were harvested every 12 h post-infection for real-time RT-PCR (28), virus titration, relative quantitative RT-PCR, or ELISA assay.
Relative quantitative RT-PCR
Total RNA from PAMs was extracted using TRIzol reagent (Takara Bio, Beijing, China), and cDNAs were synthesized using RT reagent Kits (Takara Bio). Subsequently, the cDNAs were used for the amplification of relative quantitative RT-PCR. The primer pairs are described in Table 1. The PCR reaction volume was 20 μl and comprised 10 μl TB Green® Premix Ex Taq™ II (Tli RNaseH Plus) (Takara Bio), 2 μl forward and reverse primers (20 pmol/μl), 2 μl cDNA template, and 6 μl sterile double-distilled water. The relative quantitative RT-PCR was performed on a QuantStudio5 Real-Time PCR System (Applied Biosystems, Massachusetts, USA). The thermocycling conditions were 95°C for 5 min, 95°C for 5 s, and 60°C for 34 s with 40 cycles. The 2 method was adopted to analyze the quantification of the target genes.
ELISA assay
The protein concentrations of IFN-γ, IFN-λ1, IFN-λ3, IFN-λ4, and TGF-β1 in the cell culture supernatants were detected using commercial ELISA Kits according to the manufacturer's protocols. The ELISA Kits for IFN-γ or TGF-β1 were purchased from R&D Systems in the United States (Minnesota). MyBioSource Inc. (California, USA) provided the IFN-λ1, IFN-λ3, and IFN-λ4 ELISA Kits.
Statistical analysis
The statistical analyses were conducted using a two-way analysis of variance (ANOVA) followed by Bonferroni post-tests using GraphPad Prism 5.0 (GraphPad Software Inc.). A p-value of < 0.05 was considered significant.
Results
Presence of PRRSV-ADE activity in swine anti-PRRSV antibodies (IgGs)
To confirm whether swine anti-PRRSV specific antibodies mediated the ADE phenomenon, PRRSV-positive IgG (PPI) and PRRSV-negative IgG (PNI) originating from pigs were tested for the activity of ADE infection by comparing their ability to enhance PRRSV replication in PAMs. Approximately 850 μg/ml of the purified PPI or PNI was mixed with 2,000 TCID50/ml of PRRSV in equal volumes and incubated at 37°C for 1 h to accelerate the generation of infectious virus–antibody immune complexes (PRRSV+PPI) or control groups (PRRSV+PNI). The PAM cell monolayers seeded into 24-well plates were infected with PRRSV, PRRSV+PNI, or PRRSV+PPI for the indicated time points. The infected cell supernatants were collected for demonstration of the yields of the virus by measuring the RNA and TCID50 of PRRSV. The results are shown in Figure 1. Compared with the control group cells infected with PRRSV+PNI, clear and significant increases (p < 0.001) in both PRRSV RNA and TCID50 were observed in cell culture supernatants of PAMs infected with PRRSV+PPI for 12–72 h. The increases in viral RNA and TCID50 in PAMs mediated by PPI ranged from 18.18 to 38.88 folds and 14.39–34.22 folds, respectively. By contrast, the kinetics of viral infectivity in PRRSV +PNI and PRRSV alone infected cells were similar. These results suggest that PPI facilitates the proliferation of PRRSV in PAMs. Therefore, ADE activity exists in swine anti-PRRSV antibodies.
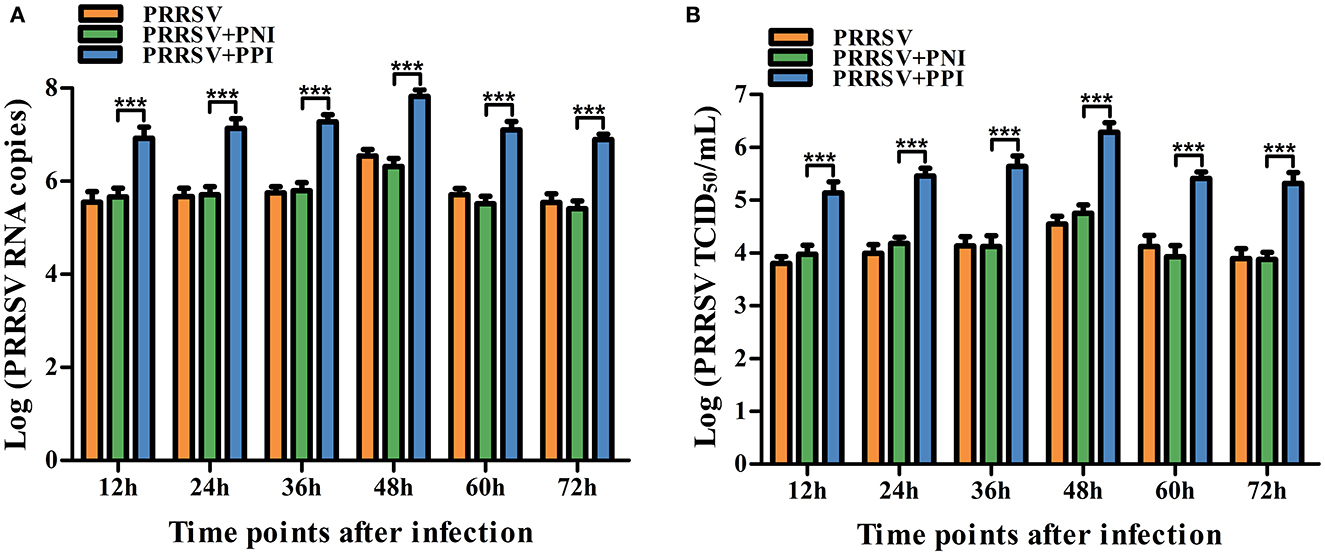
Figure 1. Detection of PRRSV-ADE activity in PAMs. PRRSV RNA (A) and TCID50 (B) in harvested culture supernatants of the PAM cells following PRRSV, PRRSV+PPI, or PRRSV+PNI infection were measured using real-time RT-PCR and viral titration experiments. The bars indicate the RNA copies or TCID50 titers of PRRSV. The error bars indicate the mean ± standard error of the mean (SEM) from three independent experiments. ***p < 0.001.
PRRSV induces IFN-γ/λs antiviral responses in PAMs
Several key innate immune cytokines (IFN-γ, IFN-λ1, IFN-λ3, IFN-λ4, and TGF-β1) and downstream antiviral protein genes (ISG15, ISG56, and OAS2) closely associated with viral replication were selected for post-PRRSV infection investigation to determine the effect of the virus on innate antiviral response in PAMs. In the case of PRRSV infection alone, the transcripts of IFN-γ, IFN-λ1, IFN-λ3, and IFN-λ4 in the infected PAM cells increased remarkably at 12–24 h postinfection and decreased slightly at 36–72 h postinfection (Figures 2A–D). Additionally, the protein concentrations of IFN-γ, IFN-λ1, IFN-λ3, and IFN-λ4 in the infected cell culture supernatants were significantly enhanced by PRRSV at 12–48 h postinfection, displaying rapid decline with the duration of virus infection (Figures 3A–D). Nevertheless, the data in Figures 2E, 3E show that the mRNA and protein concentration of TGF-β1 in the PAM cells increased observably in the case of infection with PRRSV for 12–72 h. Furthermore, relative quantitative analysis shows that the transcriptional levels of ISG15, ISG56, and OAS2 in cells infected with PRRSV for 12–60 h were signally heightened compared with the untreated mock control group cells (Figures 2F–H). These results suggest that PRRSV infection induces IFN-γ/λs antiviral responses in PAMs.
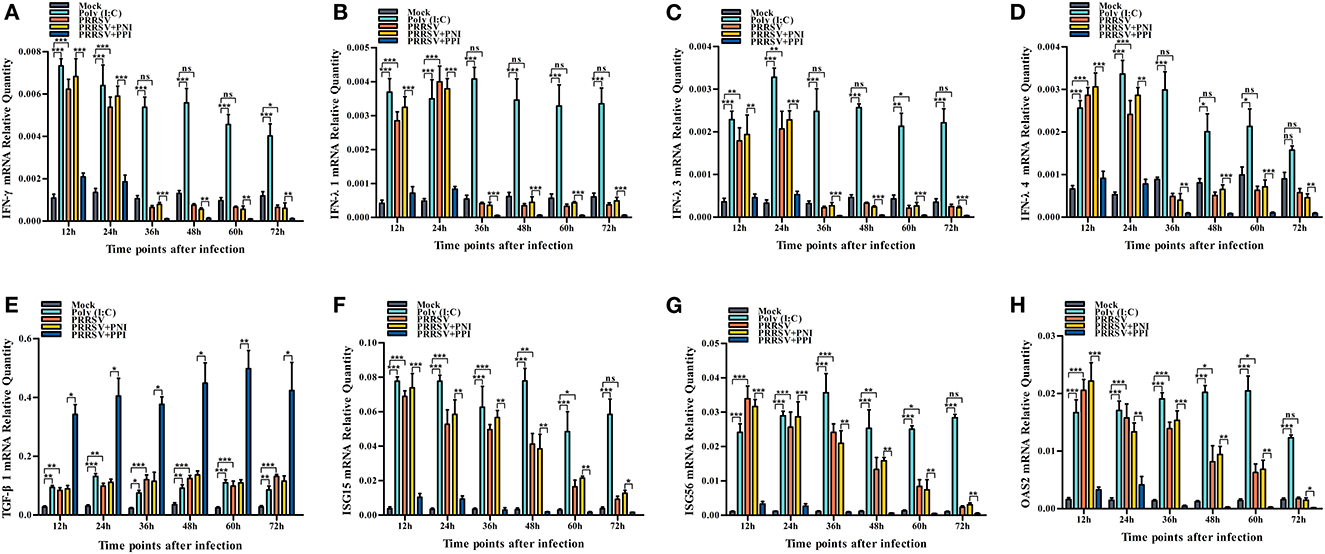
Figure 2. The effect of PRRSV or PRRSV-ADE on mRNAs of the innate immune cytokines and antiviral protein genes in PAMs. The mRNAs of the innate immune cytokines and antiviral protein genes in treated PAM cells were evaluated using relative quantitative RT-PCR. (A) IFN-γ mRNA, (B) IFN-λ1 mRNA, (C) IFN-λ3 mRNA, (D) IFN-λ4 mRNA, (E) TGF-β1 mRNA, (F) ISG15 mRNA, (G) ISG56 mRNA, and (H) OSA2 mRNA. The bars indicate the relative expression levels of mRNAs of the innate immune cytokines or antiviral protein genes. The error bars indicate the SEM from three independent experiments. ***p < 0.001, **p < 0.01, *p < 0.05, and ns, no significance.
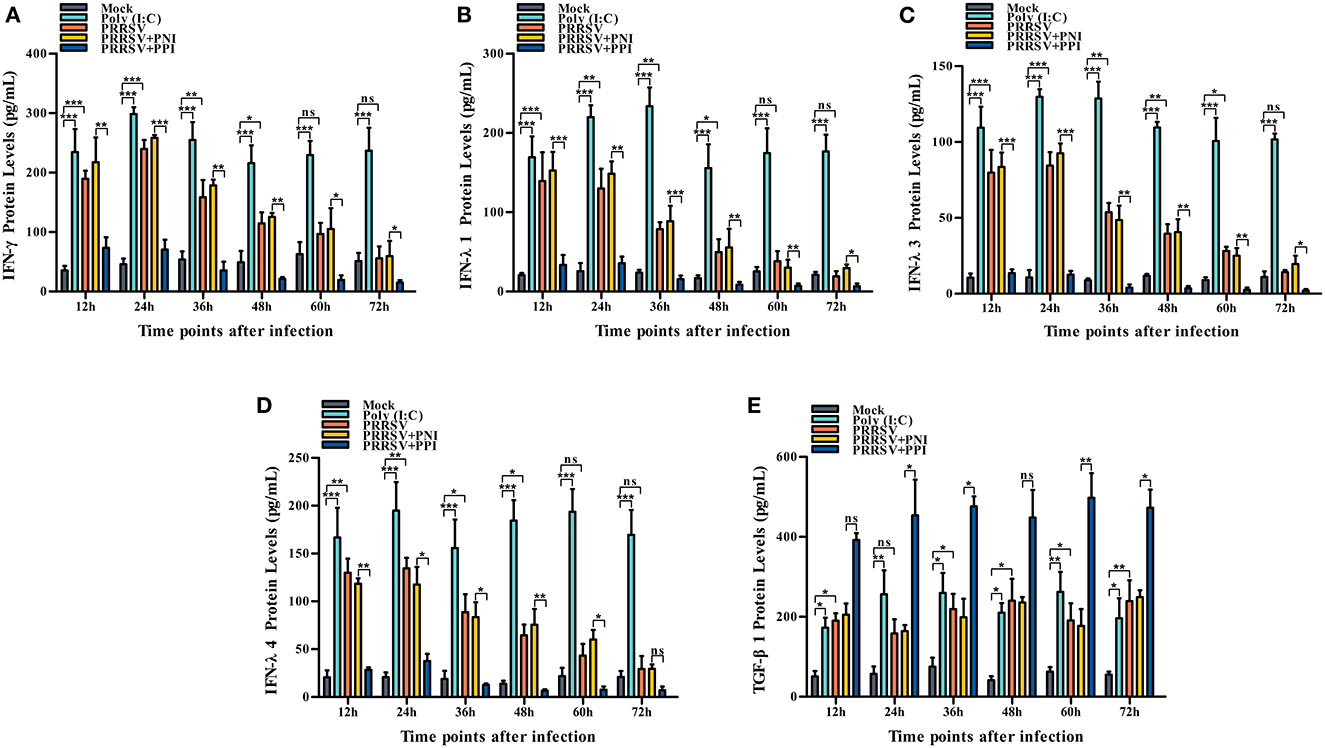
Figure 3. The effect of PRRSV or PRRSV-ADE on proteins of the innate immune cytokines in PAMs. The proteins of the innate immune cytokines in the culture supernatants of the PAM cells treated with the indicated methods were quantified using commercial ELISA Kits. (A) IFN-γ protein, (B) IFN-λ1 protein, (C) IFN-λ3 protein, (D) IFN-λ4 protein, and (E) TGF-β1 protein. The bars indicate the protein concentrations of the innate immune cytokines. The error bars indicate the SEM from three independent experiments. ***p < 0.001, **p < 0.01, *p < 0.05, and ns, no significance.
PRRSV-ADE represses IFN-γ/λs antiviral responses in PAMs
We demonstrated that PRRSV could activate the IFN-γ/λs antiviral responses of host cells. We further examined whether the ADE of PRRSV infection also affected the innate antiviral response by comparing the mRNA or protein levels of IFN-γ, IFN-λ1, IFN-λ3, IFN-λ4, TGF-β1, ISG15, ISG56, and OAS2 in PAMs cells treated with PRRSV+PNI or PRRSV+PPI for the indicated time points. The results obtained from the relative quantitative RT-PCR and ELISA assay show that the expression profiles of IFN-γ, IFN-λ1, IFN-λ3, IFN-λ4, TGF-β1, ISG15, ISG56, and OAS2 in PRRSV+PNI- and PRRSV+PPI-treated macrophages are similar (Figures 2, 3). However, there is a significant downregulation of the transcriptional levels and protein production of IFN-γ, IFN-λ1, IFN-λ3, and IFN-λ4 in PRRSV+PPI-infected macrophages at 12–72 h postinfection compared with PRRSV+PNI cultures (Figures 2A–D, 3A–D). By contrast, the TGF-β1 mRNA and its protein levels were significantly upregulated in the macrophages following the PRRSV+PPI infection (Figures 2E, 3E). Moreover, as summarized in Figures 2F–H, the quantitative analysis shows that the amounts of ISG15, ISG56, and OAS2 mRNA are reduced significantly in the PAM cells infected with PRRSV+PPI for any time point compared with the PRRSV+PNI-infected PAM cells. These results suggest that PRRSV-ADE infection represses IFN-γ/λs antiviral responses in PAMs.
Discussion
IFNs have become essential to the natural immune system against viruses by evoking potent early antiviral responses and regulating subsequent adaptive immunity (30). Three types of IFNs have been described in the IFN family, namely types I–III. Type II IFN only contains a single IFN-γ. Conversely, both type I and III IFNs are composed of multiple subtypes. For example, the groups of type I IFNs include IFN-α, IFN-β, IFN-ω, IFN-ε, and IFN-κ. The recently discovered type III IFNs, also called IFN-λs, consist of three members in pigs (IFN-λ1, IFN-λ3, and IFN-λ4), two in mice (IFN-λ2 and IFN-λ3), and four in humans (IFN-λ1, IFN-λ2, IFN-λ3, and IFN-λ4) (31, 32). The binding of IFNs to their corresponding receptors, with ensuing downstream signal transduction, triggers the expression of a series of ISGs, such as ISG15, ISG56, and OAS2. These ISGs mediate the antiviral effects of IFNs (33). There is conflicting evidence regarding the ability of PRRSV to induce IFN responses both in vivo and in vitro (27, 34, 35). Further research has shown that different PRRSV strains differ in their capability to induce IFNs (36, 37). We found that after infection with the PRRSV HeN-3 strain, PAMs secreted appreciable levels of IFN-γ, IFN-λ1, IFN-λ3, and IFN-λ4 in the early stage of infection. Furthermore, a significant increase was observed in the mRNA of antiviral genes ISG15, ISG56, and OAS2 in the PRRSV-infected PAMs compared with the mock-treated PAMs. However, due to the lack of available antibodies, we could not quantify the protein levels of these antiviral genes. Our current results collectively demonstrate that PRRSV can induce IFN-γ/λs antiviral responses in its host cells. IFN regulatory factor-3 (IRF-3), IRF-7, and nuclear factor kappa-B (NF-κB) are critical transcription factors of IFN production (32, 38). The results in Supplementary Figures 1A, B show that the mRNA levels of IRF-3, IRF-7, and NF-κB in PAMs are considerably upregulated by PRRSV at 12–24 h postinfection. PRRSV may activate IFN-γ/λs production by upregulating the levels of these transcription factors. IFNs are not the only cytokines affected by PRRSV. TGF-β1 is a negative immune regulator that may influence virus replication (39). We found that PRRSV enhanced the transcription and protein levels of TGF-β1 in PAMs, consistent with previous reports (40, 41).
Halstead and O'Rourke first suggested the FcγR-mediated ADE mechanism of virus infection in 1977 (42). Macrophages are immune cells and important host cells of various respiratory pathogens. ADE may promote the viral entry into macrophages through Fc receptor-mediated endocytosis and alter the endocellular signaling pathways, leading to their switching from an antiviral mode to a viral susceptibility mode (25, 43, 44). Data from several early studies show that there was a downregulation of type I (IFN-α/β) and II (IFN-γ) IFNs during ADE infection of the dengue virus or Ross river virus (45–47). A strong suppression of type I IFNs, including IFN-α and –β, was also reported in the ADE of PRRSV infection (29, 48). Whether PRRSV-ADE infection affects the expression of type II and III IFNs is still unknown. The results of the current study show a suppressive effect of IFN-γ, IFN-λ1, IFN-λ3, and IFN-λ4 by PRRSV-ADE infection in PAMs. By contrast, the production of TGF-β1 in PAMs increased after PRRSV infection via the ADE pathway. Moreover, compared with PRRSV+PNI-infected PAMs, a notable transcription decrease in ISG15, ISG56, and OAS2 is seen in PRRSV+PPI-infected PAMs. These results suggest the inhibition of IFN-γ/λs antiviral responses of host cells through the ADE of PRRSV infection. Early studies demonstrated that type I-III IFNs show powerful anti-PRRSV effects (49, 50). Thus, it is not surprising that PRRSV may take advantage of the ADE mechanism to antagonize the activation of these IFNs in target cells for survival. We also observed that PRRSV-ADE infection visibly cut down the transcripts of IRF-3, IRF-7, and NF-κB in PAMs in the early stage of infection (after 12–24 h infection) (Supplementary Figures 1A, B), implying that disruption of these three transcription factors may play a crucial part in PRRSV-ADE infection. In summary, our findings indicate that the ADE pathway of PRRSV infection represses innate antiviral immunity by downregulating the levels of type II and III IFNs, thereby enhancing viral replication. The detailed signaling pathway through which ADE inhibits the production of IFNs remains to be further elucidated in future studies, which will be critical for an in-depth understanding of the molecular mechanisms of ADE-mediated innate antiviral immunosuppression.
Conclusions
PRRSV alone could induce an antiviral response by upregulating the secretion of type II and III IFNs in PAMs in early infection. Moreover, via the ADE pathway, PRRSV suppressed antiviral immunity by downregulating the synthesis of type II and III IFNs in PAMs at any time point postinfection, thereby enhancing viral replication. The ADE mechanism described in this study facilitated our understanding of the pathogenesis of PRRSV-persistent infection.
Data availability statement
The original contributions presented in the study are included in the article/Supplementary material, further inquiries can be directed to the corresponding authors.
Author contributions
LZ, XF, and HW are the primary investigators of this study and carried out the data analysis. SH provided suggestions. HF and DL supervised this study. LZ wrote the manuscript. All authors have read and approved the submitted manuscript.
Funding
This research was funded by the National Natural Science Foundation of China (grant no. 32202817), the Key Natural Science Research Project of Anhui Provincial Higher Education Institution (grant no. KJ2021A0870), and the Talent Introduction Project of Anhui Science and Technology University (grant no. DKYJ202103).
Conflict of interest
The authors declare that the research was conducted in the absence of any commercial or financial relationships that could be construed as a potential conflict of interest.
Publisher's note
All claims expressed in this article are solely those of the authors and do not necessarily represent those of their affiliated organizations, or those of the publisher, the editors and the reviewers. Any product that may be evaluated in this article, or claim that may be made by its manufacturer, is not guaranteed or endorsed by the publisher.
Supplementary material
The Supplementary Material for this article can be found online at: https://www.frontiersin.org/articles/10.3389/fvets.2023.1150430/full#supplementary-material
References
2. Kappes MA, Faaberg KS. PRRSV structure, replication and recombination: origin of phenotype and genotype diversity. Virology. (2015) 479–480:475–86. doi: 10.1016/j.virol.2015.02.012
3. Xu Y, Ye M, Sun S, Cao Q, Luo J, Wang Y, et al. CD163-expressing porcine macrophages support NADC30-like and NADC34-like PRRSV infections. Viruses. (2022) 14:2056. doi: 10.3390/v14092056
4. Snijder EJ, Kikkert M, Fang Y. Arterivirus molecular biology and pathogenesis. J Gen Virol. (2013) 94:2141–63. doi: 10.1099/vir.0.056341-0
5. An TQ Li JN, Su CM, Yoo D. Molecular and cellular mechanisms for PRRSV pathogenesis and host response to infection. Virus Res. (2020) 286:197980. doi: 10.1016/j.virusres.2020.197980
6. Chand RJ, Trible BR, Rowland RR. Pathogenesis of porcine reproductive and respiratory syndrome virus. Curr Opin Virol. (2012) 2:256–63. doi: 10.1016/j.coviro.2012.02.002
7. Lunney JK, Fang Y, Ladinig A, Chen N, Li Y, Rowland B, et al. Porcine reproductive and respiratory syndrome virus (PRRSV): pathogenesis and interaction with the immune system. Annu Rev Anim Biosci. (2016) 4:129–54. doi: 10.1146/annurev-animal-022114-111025
8. Hernández J, Li Y, Mateu E. Swine dendritic cell response to porcine reproductive and respiratory syndrome virus: an update. Front Immunol. (2021) 12:712109. doi: 10.3389/fimmu.2021.712109
9. Kim HS, Kwang J, Yoon IJ, Joo HS, Frey ML. Enhanced replication of porcine reproductive and respiratory syndrome (PRRS) virus in a homogeneous subpopulation of MA-104 cell line. Arch Virol. (1993) 133:477–83. doi: 10.1007/BF01313785
10. Tirado SM, Yoon KJ. Antibody-dependent enhancement of virus infection and disease. Viral Immunol. (2003) 16:69–86. doi: 10.1089/088282403763635465
11. Christianson WT, Choi CS, Collins JE, Molitor TW, Morrison RB, Joo HS. Pathogenesis of porcine reproductive and respiratory syndrome virus infection in mid-gestation sows and fetuses. Can J Vet Res. (1993) 57:262–8.
12. Yoon KJ, Wu LL, Zimmerman JJ, Hill HT, Platt KB. Antibody-dependent enhancement (ADE) of porcine reproductive and respiratory syndrome virus (PRRSV) infection in pigs. Viral Immunol. (1996) 9:51–63. doi: 10.1089/vim.1996.9.51
13. Shibata I, Mori M, Uruno K. Experimental infection of maternally immune pigs with porcine reproductive and respiratory syndrome (PRRS) virus. J Vet Med Sci. (1998) 60:1285–91. doi: 10.1292/jvms.60.1285
14. Zhang L, Wang H, Li W, Feng X, Han F, Zhang Y, et al. Activating Fc gamma receptors and viral receptors are required for antibody-dependent enhancement of porcine reproductive and respiratory syndrome virus infection. Vet Sci. (2022) 9:470. doi: 10.3390/vetsci9090470
15. Cancel-Tirado SM, Evans RB, Yoon KJ. Monoclonal antibody analysis of porcine reproductive and respiratory syndrome virus epitopes associated with antibody-dependent enhancement and neutralization of virus infection. Vet Immunol Immunopathol. (2004) 102:249–62. doi: 10.1016/j.vetimm.2004.09.017
16. Hu J, Zhang C. Porcine reproductive and respiratory syndrome virus vaccines: current status and strategies to a universal vaccine. Transbound Emerg Dis. (2014) 61:109–20. doi: 10.1111/tbed.12016
17. Renukaradhya GJ, Meng XJ, Calvert JG, Roof M, Lager KM. Inactivated and subunit vaccines against porcine reproductive and respiratory syndrome: Current status and future direction. Vaccine. (2015) 33:3065–72. doi: 10.1016/j.vaccine.2015.04.102
18. Wei C, Dai A, Fan J, Li Y, Chen A, Zhou X, et al. Efficacy of Type 2 PRRSV vaccine against challenge with the Chinese lineage 1 (NADC30-like) PRRSVs in pigs. Sci Rep. (2019) 9:10781. doi: 10.1038/s41598-019-47239-9
19. Nan Y, Wu C, Gu G, Sun W, Zhang YJ, Zhou EM. Improved vaccine against PRRSV: current progress and future perspective. Front Microbiol. (2017) 8:1635. doi: 10.3389/fmicb.2017.01635
20. Xu L, Ma Z, Li Y, Pang Z, Xiao S. Antibody dependent enhancement: unavoidable problems in vaccine development. Adv Immunol. (2021) 151:99–133. doi: 10.1016/bs.ai.2021.08.003
21. Huisman W, Martina BE, Rimmelzwaan GF, Gruters RA, Osterhaus AD. Vaccine-induced enhancement of viral infections. Vaccine. (2009) 27:505–12. doi: 10.1016/j.vaccine.2008.10.087
22. Halstead SB. Vaccine-associated enhanced viral disease: implications for viral vaccine development. BioDrugs. (2021) 35:505–15. doi: 10.1007/s40259-021-00495-6
23. Suhrbier A, La Linn M. Suppression of antiviral responses by antibody-dependent enhancement of macrophage infection. Trends Immunol. (2003) 24:165–8. doi: 10.1016/s1471-4906(03)00065-6
24. Halstead SB, Mahalingam S, Marovich MA, Ubol S, Mosser DM. Intrinsic antibody-dependent enhancement of microbial infection in macrophages: disease regulation by immune complexes. Lancet Infect Dis. (2010) 10:712–22. doi: 10.1016/S1473-3099(10)70166-3
25. Ubol S, Halstead SB. How innate immune mechanisms contribute to antibody-enhanced viral infections. Clin Vaccine Immunol. (2010) 17:1829–35. doi: 10.1128/CVI.00316-10
26. Huang C, Zhang Q, Feng WH. Regulation and evasion of antiviral immune responses by porcine reproductive and respiratory syndrome virus. Virus Res. (2015) 202:101–11. doi: 10.1016/j.virusres.2014.12.014
27. Loving CL, Osorio FA, Murtaugh MP, Zuckermann FA. Innate and adaptive immunity against porcine reproductive and respiratory syndrome virus. Vet Immunol Immunopathol. (2015) 167:1–14. doi: 10.1016/j.vetimm.2015.07.003
28. Zhang Y, Zhou Y, Yang Q, Mu C, Duan E, Chen J, et al. Ligation of Fc gamma receptor IIB enhances levels of antiviral cytokine in response to PRRSV infection in vitro. Vet Microbiol. (2012) 160:473–80. doi: 10.1016/j.vetmic.2012.06.021
29. Zhang L, Li W, Sun Y, Kong L, Xu P, Xia P, et al. Antibody-mediated porcine reproductive and respiratory syndrome virus infection downregulates the production of interferon-α and tumor necrosis factor-α in porcine alveolar macrophages via Fc gamma receptor I and III. Viruses. (2020) 12:187. doi: 10.3390/v12020187
30. Mesev EV, LeDesma RA, Ploss A. Decoding type I and III interferon signalling during viral infection. Nat Microbiol. (2019) 4:914–24. doi: 10.1038/s41564-019-0421-x
31. Liu S, Fang P, Ke W, Wang J, Wang X, Xiao S, et al. Porcine deltacoronavirus (PDCoV) infection antagonizes interferon-λ1 production. Vet Microbiol. (2020) 247:108785. doi: 10.1016/j.vetmic.2020.108785
32. Negishi H, Taniguchi T, Yanai H. The interferon (IFN) class of cytokines and the IFN regulatory factor (IRF) transcription factor family. Cold Spring Harb Perspect Biol. (2018) 10:a028423. doi: 10.1101/cshperspect.a028423
33. Schoggins JW. Interferon-stimulated genes: what do they all do? Annu Rev Virol. (2019) 6:567–84. doi: 10.1146/annurev-virology-092818-015756
34. Baumann A, Mateu E, Murtaugh MP, Summerfield A. Impact of genotype 1 and 2 of porcine reproductive and respiratory syndrome viruses on interferon-α responses by plasmacytoid dendritic cells. Vet Res. (2013) 44:33. doi: 10.1186/1297-9716-44-33
35. Calzada-Nova G, Schnitzlein WM, Husmann RJ, Zuckermann FA. North American porcine reproductive and respiratory syndrome viruses inhibit type I interferon production by plasmacytoid dendritic cells. J Virol. (2011) 85:2703–13. doi: 10.1128/JVI.01616-10
36. Gimeno M, Darwich L, Diaz I, de la Torre E, Pujols J, Martín M, et al. Cytokine profiles and phenotype regulation of antigen presenting cells by genotype-I porcine reproductive and respiratory syndrome virus isolates. Vet Res. (2011) 42:9. doi: 10.1186/1297-9716-42-9
37. García-Nicolás O, Rosales RS, Pallarés FJ, Risco D, Quereda JJ, Graham SP, et al. Comparative analysis of cytokine transcript profiles within mediastinal lymph node compartments of pigs after infection with porcine reproductive and respiratory syndrome genotype 1 strains differing in pathogenicity. Vet Res. (2015) 46:34. doi: 10.1186/s13567-015-0161-8
38. Marsili G, Perrotti E, Remoli AL, Acchioni C, Sgarbanti M, Battistini A, et al. regulatory factors and antiviral innate immunity: how viruses can get better. J Interferon Cytokine Res. (2016) 36:414–32. doi: 10.1089/jir.2016.0002
39. Li MO, Wan YY, Sanjabi S, Robertson AK, Flavell RA. Transforming growth factor-beta regulation of immune responses. Annu Rev Immunol. (2006) 24:99–146. doi: 10.1146/annurev.immunol.24.021605.090737
40. Silva-Campa E, Flores-Mendoza L, Reséndiz M, Pinelli-Saavedra A, Mata-Haro V, Mwangi W, et al. Induction of T helper 3 regulatory cells by dendritic cells infected with porcine reproductive and respiratory syndrome virus. Virology. (2009) 387:373–9. doi: 10.1016/j.virol.2009.02.033
41. Zhang L, Xia Y, Li W, Sun Y, Kong L, Xu P, et al. Activation of Fc gamma receptor IIb up-regulates the production of interferon-alpha and interferon-gamma in porcine alveolar macrophages during PRRSV infection. Dev Comp Immunol. (2020) 109:103696. doi: 10.1016/j.dci.2020.103696
42. Halstead SB, O'Rourke EJ. Antibody-enhanced dengue virus infection in primate leukocytes. Nature. (1977) 265:739–41. doi: 10.1038/265739a0
43. Bournazos S, Gupta A, Ravetch JV. The role of IgG Fc receptors in antibody-dependent enhancement. Nat Rev Immunol. (2020) 20:633–43. doi: 10.1038/s41577-020-00410-0
44. Ubol S, Phuklia W, Kalayanarooj S, Modhiran N. Mechanisms of immune evasion induced by a complex of dengue virus and preexisting enhancing antibodies. J Infect Dis. (2010) 201:923–35. doi: 10.1086/651018
45. Chareonsirisuthigul T, Kalayanarooj S, Ubol S. Dengue virus (DENV) antibody-dependent enhancement of infection upregulates the production of anti-inflammatory cytokines, but suppresses anti-DENV free radical and pro-inflammatory cytokine production, in THP-1 cells. J Gen Virol. (2007) 88:365–75. doi: 10.1099/vir.0.82537-0
46. Halstead SB. Dengue antibody-dependent enhancement: knowns and unknowns. Microbiol Spectr. (2014) 2. doi: 10.1128/microbiolspec.AID-0022-2014
47. Modhiran N, Kalayanarooj S, Ubol S. Subversion of innate defenses by the interplay between DENV and pre-existing enhancing antibodies: TLRs signaling collapse. PLoS Negl Trop Dis. (2010) 4:e924. doi: 10.1371/journal.pntd.0000924
48. Wan B, Chen X, Li Y, Pang M, Chen H, Nie X, et al. Porcine FcγRIIb mediated PRRSV ADE infection through inhibiting IFN-β by cytoplasmic inhibitory signal transduction. Int J Biol Macromol. (2019) 138:198–206. doi: 10.1016/j.ijbiomac.2019.07.005
49. Luo R, Fang L, Jin H, Jiang Y, Wang D, Chen H, et al. Antiviral activity of type I and type III interferons against porcine reproductive and respiratory syndrome virus (PRRSV). Antiviral Res. (2011) 91:99–101. doi: 10.1016/j.antiviral.2011.04.017
Keywords: PRRSV-ADE, IFN-γ, IFN-λs, TGF-β1, PAMs
Citation: Zhang L, Feng X, Wang H, He S, Fan H and Liu D (2023) Antibody-dependent enhancement of porcine reproductive and respiratory syndrome virus infection downregulates the levels of interferon-gamma/lambdas in porcine alveolar macrophages in vitro. Front. Vet. Sci. 10:1150430. doi: 10.3389/fvets.2023.1150430
Received: 24 January 2023; Accepted: 24 February 2023;
Published: 15 March 2023.
Edited by:
Hua-Ji Qiu, Harbin Veterinary Research Institute (CAAS), ChinaReviewed by:
Scott B. Halstead, Uniformed Services University of the Health Sciences, United StatesNicolas Bertho, INRA Biologie, Épidémiologie et Analyse de Risque en santé animale (BIOEPAR), France
Copyright © 2023 Zhang, Feng, Wang, He, Fan and Liu. This is an open-access article distributed under the terms of the Creative Commons Attribution License (CC BY). The use, distribution or reproduction in other forums is permitted, provided the original author(s) and the copyright owner(s) are credited and that the original publication in this journal is cited, in accordance with accepted academic practice. No use, distribution or reproduction is permitted which does not comply with these terms.
*Correspondence: Hongjie Fan, ZmFuaGpAYWhzdHUuZWR1LmNu; Deyi Liu, bGl1ZHlAYWhzdHUuZWR1LmNu