- 1Key Laboratory of Energy Conservation and Waste Management in Agricultural Structures, Institute of Environment and Sustainable Development in Agriculture, Chinese Academy of Agricultural Sciences, Beijing, China
- 2Institute of Urban Agriculture, Chinese Academy of Agricultural Sciences, Chengdu, China
- 3Department of Animal Science, The University of Tennessee, Knoxville, Knoxville, TN, United States
- 4Henan Zhumei Swine Breeding Co., Ltd., Zhumadian, China
- 5Zhengyang Pig Farm in Henan Province, Zhumadian, China
Objective: The environment influences the sow's health and physiology during gestation. This study was conducted to evaluate indoor environmental parameters and physiological responses of early-gestation sows and investigate the possible methods for assessing the thermal environment in commercial houses.
Methods: A total of 20 early-gestation sows (commercial purebred Yorkshire) with an average body weight of 193.20 ± 3.62 kg were used for this study in winter, spring, summer, and autumn. The indoor environment parameters comprising dry-bulb temperature (Tdb), relative humidity (RH), and carbon dioxide (CO2) were recorded in 30-min intervals. Physiological parameters including heart rate (HR) and respiration rate (RR) of sows were also measured every 30 min. Wet-bulb temperature (Twb) was calculated using Tdb, RH and atmospheric pressure was recorded at a nearby weather station.
Results: The average indoor Tdb and RH were 12.98 ± 2.03°C and 80.4 ± 6.4% in winter, 18.98 ± 2.68°C and 74.4 ± 9.0% in spring, 27.49 ± 2.05°C and 90.6 ± 6.4% in summer, and 17.10 ± 2.72°C and 64.5 ± 10.9% in autumn. A higher average concentration of CO2 was observed in winter (1,493 ± 578 mg/m3) than in spring (1,299 ± 489 mg/m3), autumn (1,269 ± 229 mg/m3), and summer (702 ± 128 mg/m3). Compared with the HR and RR in the optimum environment, high RH in the house led to a significant decrease in both HR and RR (P < 0.05). In addition, a significant decline in HR was also obtained at high temperatures (P < 0.05). A temperature humidity index (THI), THI = 0.82 × Tdb + 0.18 × Twb, was determined for early-gestation sows, and the THI thresholds were 25.6 for HR. The variation in THI in summer showed that heat stress still occurred under the pad-fan cooling system.
Conclusion: This study demonstrated the critical significance of considering physiological responses of early-gestation sows in commercial houses and THI thresholds. We recommend that much more cooling measures should be taken for early-gestation sows in summer.
1. Introduction
It is well-known that China is the largest pig producer and pork consumer in the world. Even under the influence of African Swine Fever in 2019, annual pork production reached 42.55 million tons, with the average profit value of a commercial pig being 21 times more than that of 2018 (1). However, the prolonged and adverse effects of indoor air quality and thermal environment are well known to have significant implications for intensive pig production in commercial houses (2–4), resulting in impaired health and welfare and even death (5–8). Particularly, heat stress (HS) caused by elevated temperature (32°C) has been widely recognized as one of the greatest challenges in the pig industry, which affects feed efficiency, weight gain, reproduction, and welfare (9–11). As a result, it is imperative to alleviate HS and maintain optimal environment surroundings for the animals for sustainable and intensive pig production (12).
Gestation sows are critical to confined animal feeding operations since the quality and development of fetal growth were dependent on the health and strength of sows. The sows are sensitive to HS due to the limited number of sweat glands and the presence of back fat. However, sows are sensitive to HS (5, 6, 13), which could lead to decreased feed intake, anestrus, and farrowing rates, increased embryonic mortality of late-gestation sows, and declined growth and viability of piglets (6, 12, 14–17). The impaired reproduction performance of late-gestation sows might be attributed to the physiological responses under acute and cyclic high-temperature (28–32°C) conditions (5, 18). Only a few studies regarding the impacts of thermal environments on early-gestation sows have been published, which mainly focused on behavior, gut microbiota, placental efficiency, and fetal development (19). In addition, as an important indicator for assessing indoor air quality, carbon dioxide (CO2) is another gas contaminant produced in confined pig houses, which is also a useful tool to determine the ventilation rate for pig production (20, 21). CO2 also limits weight gain, behavior, vocalizations, and muscular excitation (22). Within this context, the impact of the indoor environment represents a critical issue for consideration in ensuring the health and welfare of pigs in confined buildings.
In pig production, several cooling methods have been widely used to improve animal comfort during hot weather. In particular, an increasing body of studies has implicated that the heat was removed effectively via different cooling technologies combining water evaporation and high forced air velocity, together with floor cooling (23). Previous studies also claimed that the cooling measures led to a reduction in respiratory rate and skin temperature and a higher level of feed intake (23–25). Taking into consideration the intensive pig production systems in China, the swine houses are typically made of brick with sidewall windows; the windows are open for ventilation under mild climates, which could reduce the energy consumption of ventilation, and pad-fan cooling systems are preferred in the summer. In recent years, researchers have investigated the cooling efficiency and environmental characteristics of swine barns with pad-fan cooling systems. Meanwhile, the effect of pad-fan cooling systems on sows' physiological responses was also explored under hot conditions (26, 27). However, there is a lack of research concerning HS alleviation of sows under hot conditions in commercial pig productions. As a cooling method, the effects of pad-fan systems on sows' HS need further investigation. We hypothesized that the utilization of pad-fan systems in commercial houses in China could not be effective in reducing the HS of sows. Therefore, in our study, we evaluated the cooling effect of pad-fan cooling systems in a swine house in the summer, delineated the physiological responses of early-gestation sows under commercial conditions in different seasons, and explored the HS assessment method for sows under commercial pig production conditions.
2. Materials and methods
2.1. Animals and housing
All of the animal handling involving sows was approved by the Animal Welfare Committee of the Institute of Environment and Sustainable Development in Agriculture, Chinese Academy of Agriculture Sciences (IEDACAAS, 20201201).
This study was conducted on a pig-breeding farm (Henan, China). There were five parallel pig pens on the north and south sides of the building. Each pen was comprised with nine individual crates, with fully slatted concrete floors, which had a capacity of housing 45 sows (Figure 1). Separate feeders and drinkers were equipped in each crate. Feces, urine, and wastewater were stored in a manure gutter (16.2 m × 0.3 m × 0.3 m, length × width × deepth) beneath the pen and were removed once a week. Air flowed into the barn through ventilation windows in spring and autumn, and the windows were closed in winter and summer. A mechanical ventilation system with three exhaust fans and a cooling pad system (wet curtain) was used in the summer. The fan had two operating options (off or 100% speed), and it was running at 100% speed during summer treatment.
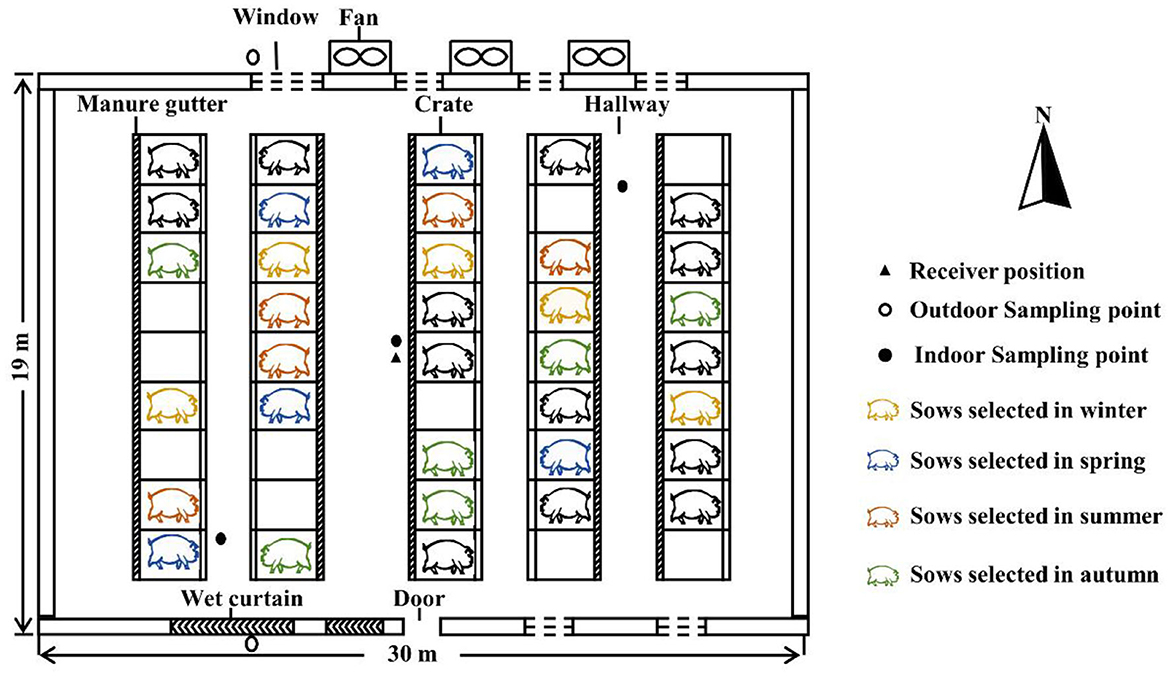
Figure 1. Schematic drawing of the sow barn (top view) and sampling locations. Twenty sows (out of 35) were selected for testing in winter, spring, summer, and autumn (five sows in each season). Signals of physiological parameters were recorded by a receiver positioned in the middle of the hallway.
The experiments were performed in winter (21 January to 26 February), spring (6 April to 13 May), summer (5 July to 3 August), and autumn (12 October to 15 November), 2021. Taking into consideration the commercial conditions in the commercial swine barn, a total of five multiparous and healthy sows (commercial purebred Yorkshire) were randomly selected in each season. The sows were artificially inseminated and confirmed pregnant, and then, the trials began and lasted for 30–35 days. The five sows were draped in a vest tailored to their size. Before the experiment, the selected sows were allowed to acclimatize to the tailored vest for 5 days after mating. Throughout the experiment, all the sows in the barn were limit-fed (2 kg/day) a diet, to meet or exceed nutrient requirements. Meanwhile, sows had ad libitum access to water, and the daily diet was divided into two meals at ~09:00 and 15:00. The body weight and parity of sows in the experiment are shown in Supplementary Table S1.
2.2. Environmental condition
There were three indoor sampling locations in this study. Indoor dry-bulb temperature (Tin) and relative humidity (RHin) were recorded with three logger devices (Hobo; accuracy ±0.10°C and 3% for Tdb and relative humidity, respectively; Onset UX100-003; Bourne, MA, USA). These three data loggers were situated at a height of 0.8 m above the floor at sows standing level, away from water sources. Meanwhile, two different data loggers (TS-WD; data logger temperature/RH; accuracy ±0.21°C and 3.5% for temperature and relative humidity, respectively; Zhengzhou TOLES Technology Co., Ltd, Henan, China) were installed at a level of 1.8 m outside the north and south windows to avoid the influence of sunlight on the outdoor dry-bulb temperature (Tout) and relative humidity (RHout).
The indoor CO2 concentration was monitored using a gas detector (accuracy ±2% F.S, respectively; MIC-600, Shenzhen Eranntex electronics CO., LTD., Shenzhen, China), and the data were recorded at an interval of 30 min. The calibration procedure was performed every 2 weeks with standard gases (2,650 ppm for CO2; National Institute of Metrology, Beijing, China).
2.3. Physiological measurements
After a 5-day acclimation, the physiological responses (heart rate and respiration rate) of sows were recorded at an interval of 30 min using the Jacketed External Telemetry system (accuracy ±0.01V, Data Sciences International, St. Paul, MN, USA). The sampling sites are presented in Supplementary Figure S1.
To avoid the variation of physiological responses caused by feed intake, the differences in physiological parameters were compared at 0 h (feeding), 0.5 h (0.5 h after feeding), 1 h (1 h after feeding), 1.5 h (1.5 h after feeding), and 2 h (2 h after feeding). The variations in physiological responses after feeding are shown in Supplementary Table S2. According to the results of the comparison, the data of 0 h (feeding), 0.5 h (0.5 h after feeding), and 1 h (1 h after feeding) were excluded when evaluating the influence of the environment on sows.
2.4. Statistics
All the statistical analyses were performed using SPSS (version 21, IBM, Armonk, NY, USA). In this study, the data were first tested for the normal distribution with the Shapiro–Wilk test and the log10 transformation occurred for those that did not pass the test. Based on the season, the environmental parameters and physiological responses of sows were analyzed using repeated analysis of variance (ANOVA) to determine the differences among the subjects at all observation times (30 min). Statistical comparisons were made using Tukey–Kramer testing, and the significance was set at P ≤ 0.05. Tukey–Kramer multiple comparison tests were performed to compare the mean values, and all of the environmental and physiological parameters were represented as mean ± standard deviation (SD).
2.4.1. Correlation between environmental factors and physiological responses
A canonical correlation analysis (CCA) was applied to quantify the relationships between environmental parameters and physiological responses, and the detailed calculation of CCA was described by Xie et al. (28).
2.4.2. Quantitative relationship between the thermal environment and physiological indices
The thermoneutral zone (TNZ) of gestation sows was 15–20°C and 60%−70% of relative humidity (29). The HR means of sows under the TNZ were averaged as the baseline. The theoretical thermodynamic wet-bulb temperature (Twb) was calculated using recommended equations described previously (30).
The HR and RR of sows at Tin more than 20°C were sorted out and compared with the corresponding baseline to obtain the variation of physiological indices, i.e., ΔHR and ΔRR. The weighting factors of dry-bulb temperature (Tdb) varied from 0 to 1, and correlation coefficients between assumed THI and physiological parameters (HR and RR) were calculated. A method of multiple regression analyses was conducted to determine the temperature–humidity index, incorporating temperature and humidity, which could describe the quantitative relationships between the thermal environment and physiological responses. Hence, the weighting factor (a) of Tdb was determined when the quadratic regression model was solved.
The relationship between THI and the physiological parameters was performed to identify the THI thresholds at which HR and RR begin to increase or decrease. The THI thresholds were determined using a 2-phase segmented regression with the piecewise procedure using OriginPro 2022b (OriginLab Corp., Northampton, MA, United States).
3. Results
3.1. Thermal and gaseous environment condition
The profiles of daily averaged Tin and RHin during experimental periods are shown in Figures 2A, B. A larger variation in indoor Tin was observed in autumn (from 11.90 to 22.17°C) than that in winter (from 9.19 to 17.81°C), spring (from 15.52 to 24.29°C), and summer (from 23.55 to 30.23°C). The indoor RHin had a larger range and fluctuation in spring (from 57.2 to 87.3%) and autumn (from 49.6 to 79.8%) than the RHin in winter (from 67.0 to 87.9%) and summer (from 82.9 to 97.4%). The variations in CO2 concentration are shown in Figure 2C. Compared with the indoor CO2 concentration in winter, spring, and autumn, the smallest fluctuations were found in summer, which ranged from 603 to 998 mg/m3.
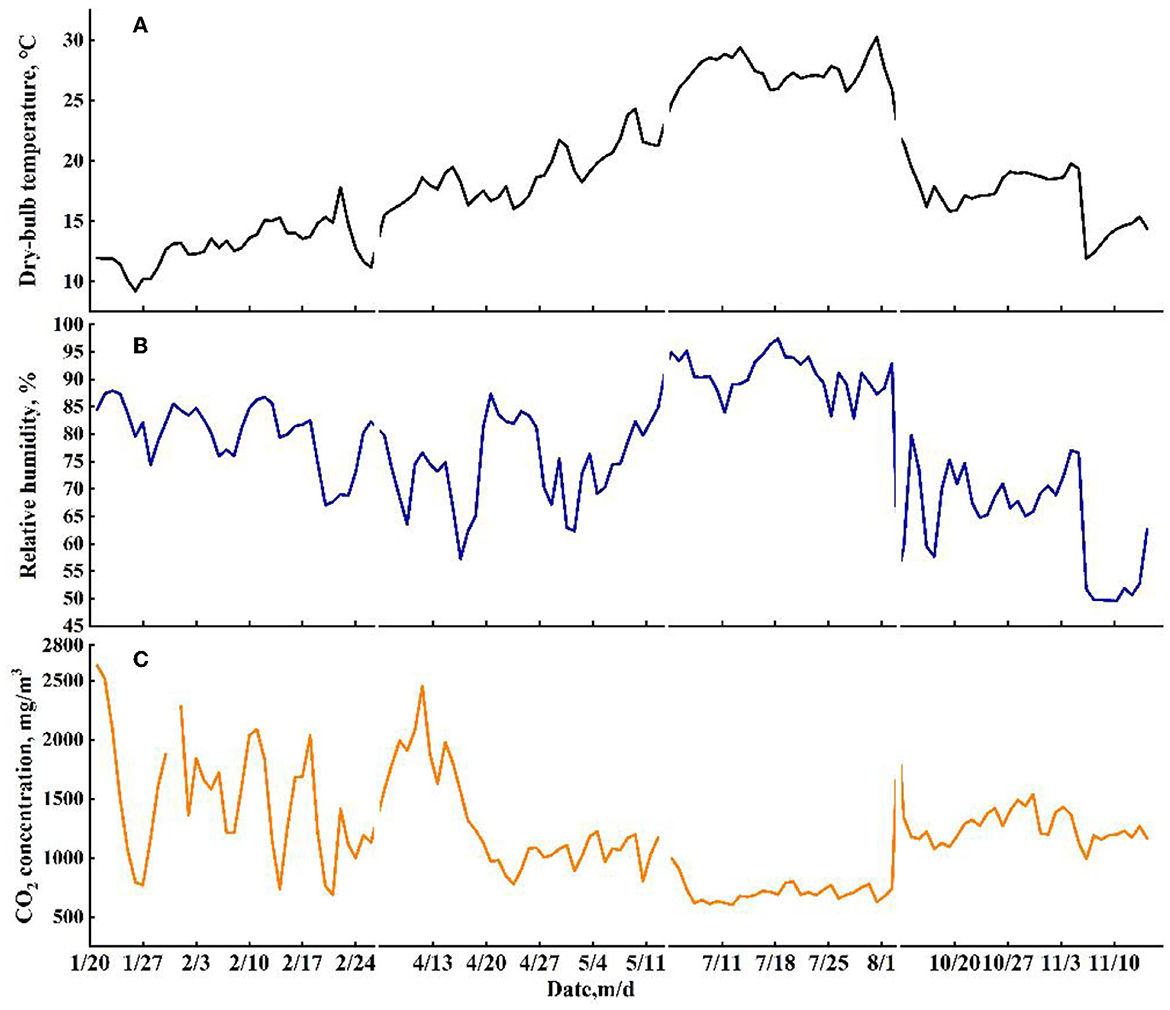
Figure 2. The profiles of daily averaged dry-bulb temperature (A), relative humidity (B), and CO2 concentration (C) during the experimental period.
The seasonal average environmental parameters (indoor and outdoor) are shown in Figure 3. There is a significant difference in temperature both inside and outside the house, and the seasonal average Tin was significantly lower than Tout (P < 0.01). In spring, no difference was found between RHin and RHout. In each season, the CO2 concentration inside the barn was higher than the outdoor CO2 concentration (P < 0.01).
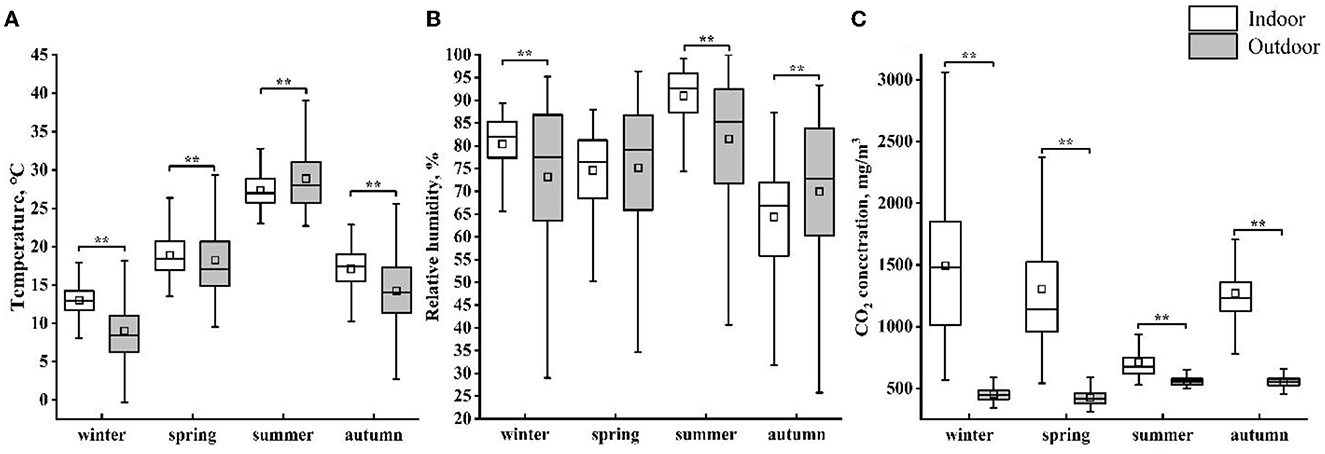
Figure 3. The indoor and outdoor averages of temperature (A), relative humidity (B), and CO2 concentration (C) for each season. The plot shows mean (square box), median (line within box), 25th and 75th percentiles (box), and 5th and 95th percentiles (whiskers). ** indicates a significant difference (P < 0.01) between indoor and outdoor environmental parameters in each season.
3.2. Physiological responses under commercial environmental conditions
The HR means were 85.44 ± 9.54 beats per min (bpm), 80.88 ± 8.34 bpm, 71.01 ± 9.74 bpm, and 86.02 ± 12.85 bpm in winter, spring, summer, and autumn, respectively. The peak HR of 123.06 bpm appeared in autumn, and the valley value of 50.00 bpm was observed in summer. The sows' RR means were 13.80 ± 6.79 breaths per min (brpm), 13.03 ± 5.66 brpm, 16.39 ± 10.48 brpm, and 14.81 ± 8.83 brpm in spring, summer, and autumn, respectively. The averaged values of HR and RR within and beyond the TNZ are shown in Table 1. The HR of sows under the TNZ was significantly higher than that under the environment beyond the TNZ (P < 0.001). A significant difference (P < 0.05) in RR was also observed between humidity within and beyond the TNZ.

Table 1. Heart rate and respiration rate of early-gestation sows exposed to a different environment.
3.3. Correlation of environmental factors with physiological responses
The result of CCA between independent variables (indoor environmental parameters) and dependent variables (physiological responses) is shown in Table 2, and two pairs of canonical variables were identified (P < 0.001). According to the percentage of variation in two pairs of canonical variables, the first pair of canonical variables could represent well the relationship between independent and dependent variables. Canonical loadings and cross-loadings of the first pair of canonical variables indicated that the Tin and RHin were strongly correlated with their first canonical variate (Table 3). The first pair of canonical variates was also characterized by a strong canonical loading on HR. Tin, RHin, and HR had the highest correlations in the first canonical variables, which were considered the major reference for the evaluation of the indoor environment.
3.4. The relationship between thermal environment and physiological indices
The THI based on HR for early-gestation sows was fitted as the following equation:
The relationships between THI and HR were plotted (Figure 4A). The THI varied from 18 to 32 during the treatment. The results of the two-phase segment regression indicated a breakpoint (THI threshold) at 25.6 THI (95% CI: 24.7–26.5, Figure 4A). There was an overall decline in HR as the THI increased, and the HR dropped slightly when THI was >25.6. The THI for each season is presented in Figure 4B, the THI threshold indicated that HS occurred in the summer under the pad-fan cooling system. The time proportion of the THI raised above the threshold in summer was 70.95%.
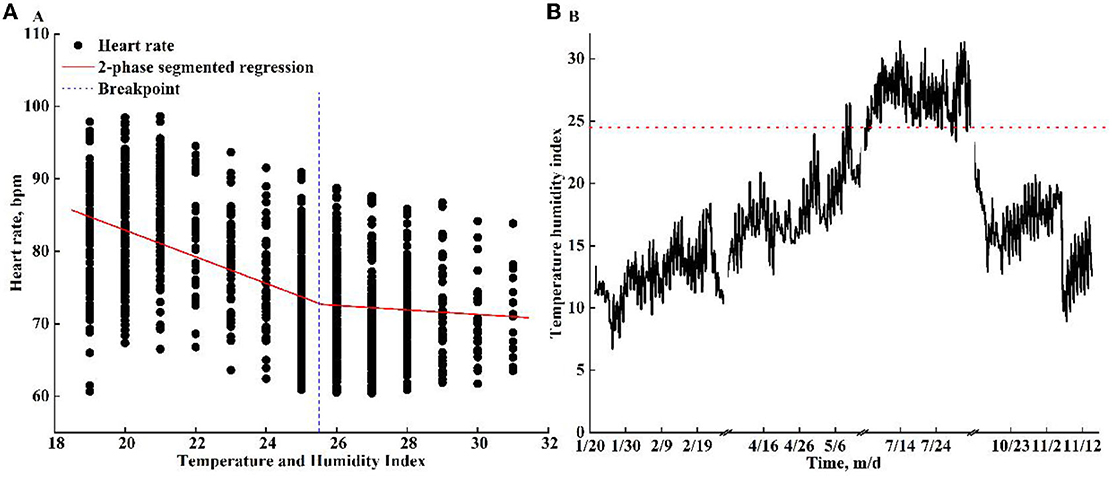
Figure 4. The variation of heart rate (beats per min, bpm) when the temperature–humidity index (THI) ranged from 18 to 32 (A) and THI (B) in each season. A breakpoint (blue vertical dot line) was detected at 25.6 THI for heart rate. The red horizontal dotted line indicates the THI threshold.
4. Discussion
The indoor Tin, RHin, and CO2 concentrations varied greatly in four seasons, which were affected by the management and structure of buildings, ventilation control, stock density, season, and outside weather conditions (3). The larger fluctuations of Tin, RHin, and CO2 concentration in spring and autumn than that in winter and summer could be attributed to the varied weather outside the house (31, 32). The smallest variations in Tin and RHin were observed in summer in comparison with those values in spring and autumn due to the pan-fan cooling systems in summer. Several studies indicated that variations in ambient temperature, as well as the activity, weight of pigs, and ventilation control, would all have a significant impact on CO2 concentrations in pig houses (33–35). The relatively low CO2 readings in summer might be attributed to the mechanical ventilation coupled with the wet-pad system.
The HR of gestation sows under the TNZ was found in this study to be slightly higher than the values of 70–80 bpm (36), which might be attributed to the larger weight and litter sizes of modern pigs (37). A declining trend was observed in HR with increasing Tin and RHin. Our result did not agree with the study by Parois et al. (38), who reported an increased HR tendency of late lactation sows exposed to acute HS. Some studies proved that the HR of pigs was raised to support the increase in blood flow to the skin, which was beneficial to maintaining euthermia in hot environments (39). On the contrary, another research reported a decrease in HR when pigs were exposed to hot environments (40). Previous literature proved that HR and its rhythm were mainly regulated by the sympathetic nervous system and the parasympathetic nervous system (41). The method of heart rate variability (HRV), including time domain analysis and frequency domain analysis, was developed to explore the effect of HS on HR. The results of HRV indicate that the HRV decreased due to the increase in sympathetic excitability in hot environments, which accounted for the decline in HR (42). This may explain the overall decrease in HR when gestation sows are exposed to the hot environment in the present study.
As a result of increased fetal growth and development, gestation sows would produce an increase in metabolic heat production (13), which may lead to an increased heat load (43). Hence, greater heat loss efforts through skin vasodilation and increased RR would be required for sows under gestation to maintain euthermia. The average RR of gestation sows increased by 10 brpm per °C when the ambient temperature was higher than 20°C (15). In this present study, higher RR was observed in sows under hot temperatures, which was consistent with previous studies (16, 44, 45). Animals have evolved coping strategies to mitigate the effects of external stresses when exposed to a continued stressor (46), and the increase in RR is likely to be related to the duration of heat treatment (19). Liu et al. (47) reported an average RR of 21.54 brpm for gestation sows exposed to chronic HS, which was a bit higher than that in our research.
Temperature and humidity play essential roles in total heat exchange between animals and their environments. The combination of Tin and RHin is effective at assessing the thermal environment (48), and the THI was proven to be an effective index that reflects the animal's response to the thermal environment (49). The THI was expressed by the equation THI = 0.65 × Tdb + 0.35 × Twb for young pigs (10–15 kg) and THI = 0.75 × Tdb + 0.25 × Twb for fattening or finishing pigs weighing from 70 to 120 kg (50, 51). In our study, the weight of 0.82 for Tdb was obtained for early-gestation sows. A comparison of the findings with other studies confirmed that the thresholds were determined, and body temperature increased rapidly when THI>28 for pigs at a weight of 12 kg (52). Some other THIs combining temperature and relative humidity were also proposed to assess the impact of the thermal environment on pigs. Lucas et al. (53) reported that HS occurred when the index value reached 75. In addition, based on the coefficient of determination for different heat load functions, the THI thresholds in the crossbreed data were 67 in Missouri, 72 in North Carolina, and 70 in North Carolina for pure breeds (54). Regarding the relationship between pregnancy rates, the results indicated that the THI threshold for sows was 78 (55). However, unlike studies related to cattle, there are few studies to determine THI thresholds in sows based on the correlation between THI and physiological responses. The finding of the THI thresholds determined in the current study was lower than in previous research. A possible explanation for a lower threshold in our research is that the sows selected in our experiment had a greater live weight (193.20 ± 3.62 kg) than pigs in previous research. It has been reported that pigs with higher live weight were more sensitive to HS, which compromised their feed intake, growth performance, and reproductive performance (56, 57). Moreover, sows under gestation were more vulnerable to HS (6, 58–60). In addition, the percentage above the THI threshold was as high as 70.95, implying that the pad-fan cooling system could not tackle the HS of sows incurred in summer weather, the cooling efficiency of the pad-fan cooling system should be improved, or other efficient measures should be sought in summer.
5. Conclusion
In this study, we evaluated the indoor thermal and gas environment in a commercial pig house for four seasons and assessed the physiological responses of early-gestation sows. There were large variations in temperature, relative humidity, and CO2 concentration in both spring and autumn. Higher RR and lower HR of early-gestation sows were exhibited in summer. Furthermore, the THI thresholds of early-gestation sows were 25.6 for HR. These THI thresholds imply that the HS of early-gestation sows occurred under pad-fan cooling systems, and much more efficient cooling measures should be taken in commercial houses. These thresholds can be served as a basis to develop HS mitigation techniques for early-gestation sows.
Data availability statement
The original contributions presented in the study are included in the article/Supplementary material, further inquiries can be directed to the corresponding author.
Ethics statement
The animal study was reviewed and approved by Ethics Committee, Institute of Urban Agriculture, Chinese Academy of Agricultural Sciences.
Author contributions
YL: conceptualization, methodology, writing—original draft, and visualization. TL: methodology, validation, and resources. BS: methodology, software, and validation. YZ: writing—reviewing and editing. XT: methodology, validation, writing—reviewing and editing, and supervision. FP and SZ: resources. XZ: resources and investigation. All authors contributed to the article and approved the submitted version.
Funding
The co-authors appreciate the support of staff from the pig-breeding farm during the experiment. This research was supported by the Shanghai Agriculture Applied Technology Development Program, G20190301 and the Agricultural Science and Technology Innovation Program, CAAS-XTCX2016011-01.
Conflict of interest
FP and XZ were employed by Henan Zhumei Swine Breeding Co., Ltd.
The remaining authors declare that the research was conducted in the absence of any commercial or financial relationships that could be construed as a potential conflict of interest.
Publisher's note
All claims expressed in this article are solely those of the authors and do not necessarily represent those of their affiliated organizations, or those of the publisher, the editors and the reviewers. Any product that may be evaluated in this article, or claim that may be made by its manufacturer, is not guaranteed or endorsed by the publisher.
Supplementary material
The Supplementary Material for this article can be found online at: https://www.frontiersin.org/articles/10.3389/fvets.2023.1178970/full#supplementary-material
References
1. National Statistical Bureau of China (NSBC). China Statistical Yearbook. Beijing: China Statistics Press (2020).
2. DeShazer J. A livestock energetics and thermal environmental management. In: DeShazer, JA, LeRoy Hahn G, Xin H, editors. Principles of the Thermal Environment and Livestock Energetics. St Joseph, MI: American Society of Agricultural and Biological Engineers (2009). p. 1–22. doi: 10.13031/2013.28294
3. Ni JQ, Heber AJ, Lim TT. Ammonia and hydrogen sulphide in swine production. In: Air Quality and Livestock Farming/ Florida, FA, USA: CRC Press (2018). p. 69–88. doi: 10.1201/9781315738338-3
4. Mihina S, Sauter M, Palkovicova Z, Karanduovská I, Broucek J. Concentration of harmful gases in poultry and pig houses. Anim Sci Papers Rep. (2012) 30:395–406.
5. Williams AM, Safranski TJ, Spiers DE, Eichen PA, Coate EA, Lucy MC. Effects of a controlled heat stress during late gestation, lactation, and after weaning on thermoregulation, metabolism, and reproduction of primiparous sows. J Anim Sci. (2013) 91:2700–14. doi: 10.2527/jas.2012-6055
6. Ross JW, Hale BJ, Gabler NK, Rhoads RP, Keating AF, Baumgard LH. Physiological consequences of heat stress in pigs. Anim Reprod Sci. (2015) 55:1381–90. doi: 10.1071/AN15267
7. Cobanovic N, Jamnikar-Ciglenecki U, Kirbis A, Krizman M, Stukelj M, Karabasil N. Impact of various housing conditions on the occurrence of pathological lesions in slaughtered pigs. Vet Glas. (2019) 73:17–29. doi: 10.2298/VETGL190318010C
8. Cobanovic N, Stankovic SD, Dimitrijevic M, Suvajdzic B, Grkovic N, Vasilev D, et al. Identifying Physiological stress biomarkers for prediction of pork quality variation. Animals. (2020) 10:614. doi: 10.3390/ani10040614
9. Johnson JS, Abuajamieh M, Sanz Fernandez MV, Seibert JT, Stoakes SK, Nteeba J, et al. Thermal stress alters postabsorptive metabolism during pre- and postnatal development. In: Sejian V, Gaughan J, Baumgard L, editors. Climate Change Impact on Livestock: Adaptation and Mitigation. New Delhi: Springer (2015). p. 61–80. doi: 10.1007/978-81-322-2265-1_5
10. Johnson JS. Heat stress: impact on livestock well-being and productivity and mitigation strategies to alleviate the negative effects. Anim Prod Sci. (2018) 58:1404–13. doi: 10.1071/AN17725
11. Brito LF, Oliveira HR, McConn BR, Schinckel AP, Arrazola A, Marchant-Forde JN, et al. Large-Scale phenotyping of livestock welfare in commercial production systems: a new frontier in animal breeding. Front Genet. (2020) 11:793. doi: 10.3389/fgene.2020.00793
12. Renaudeau D, Collin A, de Basilio Yahav S, Gourdine JL, Collier RJ. Adaptation to hot climate and strategies to alleviate heat stress in livestock production. Animal. (2012) 6:707–28. doi: 10.1017/S1751731111002448
13. He J, Zheng W, Lu M, Yang X, Xue Y, Yao W. A controlled heat stress during late gestation affects thermoregulation, productive performance, and metabolite profiles of primiparous sow. J Therm Boil. (2019) 81:33–40. doi: 10.1016/j.jtherbio.2019.01.011
14. Nardone A, Ronchi B, Lacetera N, Bernabucci U. Climatic effects on productive traits in livestock. Vet Res Commun. (2006) 30:75–81. doi: 10.1007/s11259-006-0016-x
15. Quiniou N, Noblet J. Influence of high ambient temperatures on performance of multiparous lactating sows. J Anim Sci. (1999) 77:2124–34. doi: 10.2527/1999.7782124x
16. Lucy MC, Safranski TJ. Heat stress in pregnant sows: thermal responses and subsequent performance of sows and their offspring. Mol Reprod Dev. (2017) 84:946–56. doi: 10.1002/mrd.22844
17. Prunier A, de Braganca MM, Le Dividich J. Influence of high ambient temperature on performance of reproductive sows. Livest Prod Sci. (1997) 52:123–33. doi: 10.1016/S0301-6226(97)00137-1
18. McConn BR, Gaskill BN, Schinckel AP, Green-Miller AR, Lay DC, Johnson JS. Thermoregulatory and physiological responses of nonpregnant, mid-gestation, and late-gestation sows exposed to incrementally increasing dry bulb temperature. J Anim Sci. (2021) 99:1–8. doi: 10.1093/jas/skab181
19. Zhao W, Liu F, Bell AW, Le HH, Cottrell JJ, Leury BJ, et al. Controlled elevated temperatures during early-mid gestation cause placental insufficiency and implications for fetal growth in pregnant pigs. Sci Rep. (2020) 10:20677. doi: 10.1038/s41598-020-77647-1
20. Estellés F, Rodríguez-Latorre AR, Calvet S, Villagrá A, Torres AG. Daily carbon dioxide emission and activity of rabbits during the fattening period. Biosyst Eng. (2010) 106:338–43. doi: 10.1016/j.biosystemseng.2010.02.011
21. Heederik D, Sigsgaard T, Thorne PS, Kline JN, Avery R, Bonlokke JH, et al. Health effects of airborne exposures from concentrated animal feeding operations. Environ Health Perspect. (2007) 115:298–302. doi: 10.1289/ehp.8835
22. Llonch P, Rodríguez P, Jospin M, Dalmau A, Manteca X, Velarde A. Assessment of unconsciousness in pigs during exposure to nitrogen and carbon dioxide mixtures. Animal. (2013) 7:492–8. doi: 10.1017/S1751731112001966
23. Godyn, ' D, Herbut P, Angrecka S, Corrêa Vieira FM. Use of different cooling methods in pig facilities to alleviate the effects of heat stress—a review. Animals. (2020) 10:1459. doi: 10.3390/ani10091459
24. Huynh TTT, Aarnink AJA, Truong CT, Kemp B, Verstegen MWA. Effects of tropical climate and water cooling methods on growing pigs' responses. Livest Sci. (2006) 104:278–91. doi: 10.1016/j.livsci.2006.04.029
25. Perin J, Gaggini TS, Manica S, Magnabosco D, Bernardi ML, Wentz I, et al. Evaporative snout cooling system on the performance of lactating sows and their litters in a subtropical region. Ciência Rural. (2016) 46:342–7. doi: 10.1590/0103-8478cr20141693
26. Chen J, Guo J, Guan W-t., Song J-J, Deng Z-X, Cheng L, et al. Effect of pad-fan cooling and dietary organic acid supplementation during late gestation and lactation on reproductive performance and antioxidant status of multiparous sows in hot weather. Trop Anim Health Prod. (2018) 50:973–82. doi: 10.1007/s11250-018-1520-z
27. Johnson JS, Jansen TL, Galvin M, Field TC, Graham JR, Stwalley RM, et al. Electronically controlled cooling pads can improve litter growth performance and indirect measures of milk production in heat-stressed lactating sows. J Anim Sci. (2022) 100:1–10. doi: 10.1093/jas/skab371
28. Xie Q, Ni J-Q, Bao J, Su Z. Correlations, variations, and modelling of indoor environment in a mechanically-ventilated pig building. J Cleaner Prod. (2021) 2:124441. doi: 10.1016/j.jclepro.2020.124441
29. Wathes C, Whittemore C. Environmental management of pigs. in: Kyriazakis I, Whittemore CT, editors. Whittemore's Science and Practice of Pig Production. Oxford, UK: Blackwell (2006). p. 533–92 doi: 10.1002/9780470995624.ch17
30. Stull R. Wet-Bulb Temperature from Relative Humidity and Air Temperature. J Appl Meteorol Climatol. (2011) 50:2267–9. doi: 10.1175/JAMC-D-11-0143.1
31. Shen D, Wu S, Li Z, Tang Q, Dai P, Li Y, et al. Distribution and physicochemical properties of particulate matter in swine confinement barns. Environ Pollut. (2019) 250:746–53. doi: 10.1016/j.envpol.2019.04.086
32. Wegner K, Lambertz C, Das G, Reiner G, Gauly M. Climatic effects on sow fertility and piglet survival under influence of a moderate climate. Animal. (2014) 8:1526–33. doi: 10.1017/S1751731114001219
33. Blunden J, Aneja VP, Westerman PW. Measurement and analysis of ammonia and hydrogen sulfide emissions from a mechanically ventilated swine confinement building in North Carolina. Atmos Environ. (2008) 42:3315–31. doi: 10.1016/j.atmosenv.2007.06.040
34. Wang K, Wei B, Zhu S, Ye Z. Ammonia and odor emitted from deep litter and fully slatted floor systems for growing–finishing pigs. Biosyst Eng. (2011) 109:203–10. doi: 10.1016/j.biosystemseng.2011.04.001
35. Philippe FX, Nicks B. Review on greenhouse gas emissions from pig houses: production of carbon dioxide, methane and nitrous oxide by animals and manure. Agric Ecosyst Environ. (2014) 199:10–25. doi: 10.1016/j.agee.2014.08.015
36. Straw BE, Zimmerman JJ, D'Allaire S, Taylorm DJ. Diseases of Swine. 9th ed. Hoboken, NJ, USA: Wiley-Blackwell (2006).
37. Sipos W, Wiener S, Entenfellner F, Sipos S. Physiological changes of rectal temperature, pulse rate and respiratory rate of pigs at different ages including the critical periportal period. Vet Med. Austria. (2013) 100:93–8
38. Parois SP, Cabezón FA, Schinckel AP, Johnson JS, Stwalley RM, Marchant-Forde JN. Effect of floor cooling on behavior and heart rate of late lactation sows under acute heat stress. Front Vet Sci. (2018) 5:223. doi: 10.3389/fvets.2018.00223
39. Moran DS, Shitzer A, Pandolf KB. A physiological strain index to evaluate heat stress. Am J Physiol. (1998) 275:R129–34. doi: 10.1152/ajpregu.1998.275.1.R129
40. Pérez Laspiur J, Trottier NL. Effect of dietary arginine supplementation and environmental temperature on sow lactation performance. Livestock Prod Sci. (2001) 70:159–65. doi: 10.1016/S0301-6226(01)00209-3
41. Iwanaga M, Kobayashi A, Kawasaki C. Heart rate variability with repetitive exposure to music. Biol Psychol. (2005) 70:61–6. doi: 10.1016/j.biopsycho.2004.11.015
42. Bruce-Low SS, Cotterrell D, Jones GE. Heart rate variability during high ambient heat exposure. Aviat Space Environ Med. (2006) 77:915–20.
43. Zumbach B, Misztal I, Tsuruta S, Sanchez JP, Azain M, Herring W, et al. Genetic components of heat stress in finishing pigs: development of a heat load function. J Anim Sci. (2008) 86:2082–8. doi: 10.2527/jas.2007-0523
44. Bjerg B, Brandt P, Pedersen P, Zhang G. Sows' responses to increased heat load—a review. J Therm Biol. (2020) 94:102758. doi: 10.1016/j.jtherbio.2020.102758
45. Malmkvist J, Pedersen LJ, Kammersgaard TS, Jorgensen E. Influence of thermal environment on sows around farrowing and during the lactation period. J Anim Sci. (2012) 90:3186–99. doi: 10.2527/jas.2011-4342
46. Collier RJ, Baumgard LH, Zimbelman RB, Xiao Y. Heat stress: physiology of acclimation and adaptation. Anim Front. (2018) 9:12–9. doi: 10.1093/af/vfy031
47. Liu F, Ford EM, Morrison RS, Brewster CJ, Henman DJ, Smits RJ, et al. The greater proportion of born-light progeny from sows mated in summer contributes to increased carcass fatness observed in spring. Animals. (2020) 10:2080. doi: 10.3390/ani10112080
48. Mader TL, Davis MS. Effect of management strategies on reducing heat stress of feedlot cattle: feed and water intake. J Anim Sci. (2004) 82:3077–87. doi: 10.2527/2004.82103077x
49. Vitt R, Weber L, Zollitsch W, Hörtenhuber SJ, Baumgartner J, Niebuhr K, et al. Modelled performance of energy saving air treatment devices to mitigate heat stress for confined livestock buildings in Central Europe. Biosyst Eng. (2017) 164:85–97. doi: 10.1016/j.biosystemseng.2017.09.013
51. Ingram DL. The effect of humidity on temperature regulation and cutaneous water loss in the young pig. Res Vet Sci. (1965) 6:9–17. doi: 10.1016/S0034-5288(18)34762-3
52. Wang K, Miao X, Cui S, Hogenboom CM, Geers R. Effects of ambient temperature and relative humidity on physiological parameters and performance of growing pigs. Trans CSAE. (2002) 18:99–102.
53. Lucas EM, Randall JM, Meneses JF. Potential for evaporative cooling during heat stress periods in pig production in Portugal (Alentejo). J Agric Eng Res. (2000) 76:363–71. doi: 10.1006/jaer.2000.0550
54. Fragomeni BO, Lourenco DAL, Tsuruta S, Andonov S, Gray K, Huang Y, et al. Modeling response to heat stress in pigs from nucleus and commercial farms in different locations in the United States. J Anim Sci. (2016) 94:4789–98. doi: 10.2527/jas.2016-0536
55. Mellado M, Gaytan L, Macias-Cruz U, Avendano L, Meza-Herrera C, Lozano EA, et al. Effect of climate and insemination technique on reproductive performance of gilts and sows in a subtropical zone of Mexico. Austral J Vet Sci. (2018) 50:27–34. doi: 10.4067/S0719-81322018000100106
56. Mayorga EJ, Renaudeau D, Ramirez BC, Ross JW, Baumgard LH. Heat stress adaptations in pigs. Anim Front. (2018) 9:54–61. doi: 10.1093/af/vfy035
57. Rauw WM, de Mercado de la Peña E, Gomez-Raya L, García Cortés LA, Ciruelos JJ, Gómez Izquierdo E. Impact of environmental temperature on production traits in pigs. Sci Rep. (2020) 10:2106. doi: 10.1038/s41598-020-58981-w
58. Tummaruk P, Tantasuparuk W, Techakumphuk M, Kunavongkrit A. Effect of season and outdoor climate on litter size at birth in purebred Landrace and Yorkshire sows in Thailand. J Vet Res. (2004) 66:477–82. doi: 10.1292/jvms.66.477
59. Heitman HJr, Hughes EH, Kelly CF. Effects of elevated ambient temperature on pregnant sows. J Anim Sci. (1951) 10:907–15. doi: 10.2527/jas1951.104907x
Keywords: physiology, sow, temperature humidity index, threshold, index
Citation: Li Y, Li T, Shang B, Zhao Y, Tao X, Peng F, Zou X and Zhang S (2023) Evaluation of the effect of the indoor environment on the physiological responses of early-gestation sows in a commercial house in China. Front. Vet. Sci. 10:1178970. doi: 10.3389/fvets.2023.1178970
Received: 03 March 2023; Accepted: 11 May 2023;
Published: 01 June 2023.
Edited by:
Daniel Mota-Rojas, Metropolitan Autonomous University, MexicoReviewed by:
Adriana Domínguez-Oliva, Metropolitan Autonomous University, MexicoAdriana Olmos-Hernández, National Institute of Rehabilitation Luis Guillermo Ibarra Ibarra, Mexico
Chunbo Wei, Heilongjiang Bayi Agricultural University, China
Copyright © 2023 Li, Li, Shang, Zhao, Tao, Peng, Zou and Zhang. This is an open-access article distributed under the terms of the Creative Commons Attribution License (CC BY). The use, distribution or reproduction in other forums is permitted, provided the original author(s) and the copyright owner(s) are credited and that the original publication in this journal is cited, in accordance with accepted academic practice. No use, distribution or reproduction is permitted which does not comply with these terms.
*Correspondence: Xiuping Tao, dGFveGl1cGluZ0BjYWFzLmNu