- 1Division of Molecular Psychoneuroimmunology, Institute for Genetic Medicine, Graduate School of Medicine, Hokkaido University, Sapporo, Japan
- 2Laboratory of Veterinary Internal Medicine, Department of Clinical Sciences, Graduate School of Veterinary Medicine, Hokkaido University, Sapporo, Japan
- 3Division of Molecular Neuroimmunology, Department of Homeostatic Regulation, National Institute for Physiological Sciences, National Institutes of Natural Sciences, Okazaki, Japan
- 4Veterinary Teaching Hospital, Graduate School of Veterinary Medicine, Hokkaido University, Sapporo, Japan
- 5Laboratory of Veterinary Internal Medicine, Department of Small Animal Clinical Sciences, School of Veterinary Medicine, Rakuno Gakuen University, Ebetsu, Japan
- 6North Lab, Sapporo, Japan
- 7Group of Quantum Immunology, Institute for Quantum Life Science, National Institute for Quantum and Radiological Science and Technology (QST), Chiba, Japan
- 8Institute for Vaccine Research and Development (HU-IVReD), Hokkaido University, Sapporo, Japan
Inflammatory colorectal polyp (ICRP) in miniature dachshunds (MDs) is a chronic inflammatory bowel disease (IBD) characterized by granulomatous inflammation that consists of neutrophil infiltration and goblet cell hyperplasia in the colon. Recently, we identified five MD-associated single-nucleotide polymorphisms (SNPs), namely PLG, TCOF1, TG, COL9A2, and COL4A4, by whole-exome sequencing. Here, we investigated whether TG c.4567C>T (p.R1523W) is associated with the ICRP pathology. We found that the frequency of the T/T SNP risk allele was significantly increased in MDs with ICRP. In vitro experiments showed that TG expression in non-immune cells was increased by inducing the IL-6 amplifier with IL-6 and TNF-α. On the other hand, a deficiency of TG suppressed the IL-6 amplifier. Moreover, recombinant TG treatment enhanced the activation of the IL-6 amplifier, suggesting that TG is both a positive regulator and a target of the IL-6 amplifier. We also found that TG expression together with two NF-κB targets, IL6 and CCL2, was increased in colon samples isolated from MDs with the T/T risk allele compared to those with the C/C non-risk allele, but serum TG was not increased. Cumulatively, these results suggest that the T/T SNP is an expression quantitative trait locus (eQTL) of TG mRNA in the colon, and local TG expression triggered by this SNP increases the risk of ICRP in MDs via the IL-6 amplifier. Therefore, TG c.4567C>T is a diagnostic target for ICRP in MDs, and TG-mediated IL-6 amplifier activation in the colon is a possible therapeutic target for ICRP.
1. Introduction
Inflammatory colorectal polyp (ICRP) in miniature dachshunds (MDs) is a chronic inflammatory disease confined to the colorectal region of dogs. It is characterized by multiple polypoid lesions or a single pedunculated granulomatous lesion growing from the colorectal mucosa, which has been reported only in Japan (1). Histopathologically, ICRP has been differentiated from other types of polyps by its traits of granulomatous inflammation with neovascularization, the infiltration of neutrophils, lymphocytes, and/or macrophages, goblet cell hyperplasia, exaggerated mucus production, and crypt dilation. Clinical signs of hematochezia, tenesmus, and mucoid feces have also been reported (1–3). ICRP responds well to immunosuppressive treatments such as leflunomide, prednisolone, and cyclosporine (4, 5). However, recurrences are common despite these treatments (2, 3), and further study of the ICRP pathogenesis is needed to develop a better cure. The inflammatory mechanism of ICRP has been reported to include the dysregulation of innate immunity, the upregulation of toll-like receptors (TLRs), and the overexpression of pro-inflammatory cytokines (6–10). Notably, MDs have a much higher tendency to develop ICRP, with an odds ratio of 24.63, compared to other dog breeds (1), signifying the importance of the genetic basis of this disease. The single-nucleotide polymorphisms (SNPs) of nucleotide-binding oligomerization domain 2 (NOD2) gene were investigated in the pathogenesis of ICRP but no major effect was found (11), leaving the detailed molecular mechanism of the inflammation unknown. Using whole-exome sequencing (WES), we found several genes related to inflammatory responses are MD-associated SNPs in ICRP (12).
Pioneer studies in chronic inflammation have shown that pro-inflammatory cytokine stimulation including IL-6 can enhance the expressions of inflammatory mediators in non-immune cells in the development of inflammatory diseases (13, 14). The simultaneous activation of NF-κB and STAT3 in non-immune cells has been shown to be the key molecular mechanism that enhances the activation of NF-κB. Although IL-6 is one of very few stimulators of STAT3, there are multiple stimulators of NF-κB, including TNF-α, IL-17A, growth factors, noradrenaline, and TLR ligands, during inflammation development (13–25). The combined effects of IL-6 and NF-κB on NF-κB activation were named the “IL-6 amplifier” (13). The IL-6 amplifier's role in the pathogenesis of inflammatory diseases has been well described in both mouse disease models and clinical samples of human patients (15, 17, 19, 26–38).
A genome-wide screening of the IL-6 amplifier identified approximately 500 target genes and 1,200 positive regulators (39). Recently, we used WES and found that some of these positive regulators, PLG, TCOF1, TG, COL9A2, and COL4A4, are MD-associated SNPs in ICRP (12). In this study, we verified that TG (thyroglobulin) is associated with the pathogenesis of ICRP. We found that MDs have a higher frequency of the TG risk allele T/T, which in turn increases TG expression in the colon, resulting in the activation of the IL-6 amplifier and chronic local inflammation. Furthermore, we found that TG deficiency suppressed and recombinant TG treatment enhanced the IL-6 amplifier activation in non-immune cells in vitro. Along with TG, the expression of other NF-κB targets, such as IL6 and CCL2, was concurrently increased in the colons of MDs with the risk allele T/T, strengthening the conclusion that TG is a potential therapeutic target for ICRP. These data uncover a new function of TG related to chronic inflammation and the breed specificity of ICRP in MDs.
2. Materials and methods
2.1. Animals
The genotyping of selected SNPs (Table 1) was performed by Sanger sequencing on 155 MDs diagnosed with ICRP, 90 age-controlled MDs without ICRP, 36 juvenile MDs aged < 1 year, and 40 dogs of other breeds. The details of the animals recruited and the samples used in this study are summarized in Supplementary Table 1. A DNA Blood & Tissue Kit (QIAGEN, Hilden, Germany) was used to derive genomic DNA (gDNA) from EDTA-archived blood, fresh colon tissue samples, or formalin-fixed paraffin-embedded colon tissue samples of the recruited dogs. Blood was collected by a veterinarian or a veterinary student supervised by a licensed veterinarian through a jugular venipuncture as part of routine blood workup for screening or diagnosis purposes. A signed consent form was obtained from the owners of all dogs for the tissue sample collection and use in this study.
2.2. Tissue sample collection
Endoscopic examinations were performed under general anesthesia for all dogs to retrieve the colon tissues. Each dog was administrated with midazolam (0.1 mg/kg) and butorphanol tartrate (0.2 mg/kg) intravenously as pre-medication and then with propofol (4 to 6 mg/kg) using the same route. Anesthesia was then maintained through the inhalation of isoflurane with oxygen, where additional butorphanol was administrated when necessary. Pulse oximetry readings, electrocardiograph, capnograph, arterial blood pressure, and rectal temperature were monitored throughout the anesthesia to ensure a smooth endoscopic procedure. Endoscopic procedures were completed within 2 h in all dogs, and all dogs recovered uneventfully. In MDs with ICRP, samples were collected as part of the diagnostic procedure, where polyp lesions deemed inflammatory on gross appearance were collected endoscopically with forceps or through electrosurgical snare polypectomy. Normal colorectal mucosae were collected endoscopically from the colorectal region adjacent to the diseased area based on gross appearance. At least six tissue samples were collected from both the polyp lesion site and normal colorectal mucosal region in MDs with ICRP. All biopsy specimens were assessed by a board-certified veterinary pathologist (YK) according to histopathological standards established by the World Small Animal Veterinary Association Gastrointestinal Standardization Group (40). The severity of ICRP inflammation in each case was staged according to a previous report (3). The information on the medication and histopathology for each group is shown in Supplementary Table 2. Each tissue sample was stored at −80°C for protein analysis or RNALater RNA Stabilization Solution (Ambion Inc., Austin, TX, USA) for 24 h at 4°C to allow penetration and stabilization and then at −80°C for prolonged storage. Five specimens were collected for the qPCR analysis to perform a definitive diagnosis of ICRP.
2.3. Targeted genotyping
Polymerase chain reactions (PCRs) and Sanger sequencing were utilized to validate the genotype variants selected from WES data (12). The WES data have been deposited with links to BioProject accession number PRJDB16014 in the DDBJ BioProject database. Primer pairs used for the variant validation were designed using Primer3Plus software (http://www.bioinformatics.nl/cgi-bin/primer3plus/primer3plus.cgi; Table 1). Amplicons post-PCR were purified using a commercial clean-up reagent, ExoSAP-IT Express (Thermo Fisher Scientific, Waltham, MA, USA), and sequencing was performed using the Sanger method utilizing BigDye Terminator v3.1 (Thermo Fisher Scientific) and ABI PRISM 3100 Genetic Analyzer (Applied Biosystems, Waltham, MA, USA). FinchTV (https://digitalworldbiology.com/FinchTV) was used to interrogate the reads generated, and the allele frequency was compared between groups using Fisher's exact test.
2.4. Cell lines and stimulation conditions
The H4 human cancer cell line was purchased from ATCC (Sumitomo Dainippon Pharma, Osaka, Japan). All cell lines were cultured in DMEM (Thermo Fisher Scientific, Waltham, MA) enriched with 10% fetal bovine serum (Thermo Fisher Scientific) and treated with 1% penicillin and streptomycin at 37°C under 5% CO2. For the cytokine stimulation, cells were seeded into 96-well plates (1 × 104 cells/well) and stimulated with human IL-6 (30 ng/ml; Toray Industries, Tokyo, Japan) plus human soluble IL-6 receptor (30 ng/ml; R&D Systems, Minneapolis, MN) and/or TNF- α (10 ng/ml; PeproTech, Tokyo, Japan) for 3 h after 2 h of serum starvation in Opti-MEM (Thermo Fisher Scientific, Waltham, MA). For the TG stimulation, the cytokine stimulation was modified to include cells stimulated with a 5x dilution of the cytokine with the serial addition of recombinant human TG (1 μg/ml, 5 μg/ml, and 10 μg/mL) as per the manufacturer's recommendation (Cloud-Clone Corp., USA). After stimulation, the cells were lysed, and the total RNA was retrieved for real-time PCR.
2.5. Quantitative real-time PCR in H4 cells
The Bio-Rad CFX96 real-time PCR system (Bio-Rad Laboratories, Hercules, CA, USA) and THUNDERBIRD SYBR quantitative PCR (qPCR) Mix (TOYOBO Co. Ltd., Osaka, Japan) were used to quantify the levels of target mRNA and internal control mRNA (glyceraldehyde-3-phosphate dehydrogenase, GAPDH). Total RNA was prepared from cells using a SuperPrep Cell Lysis Kit for qPCR (TOYOBO). The conditions for real-time qPCR were 40 cycles at 94°C for 15 s followed by 40 cycles at 60°C for 60 s. Relative IL6 mRNA expressions were normalized to the level of GAPDH mRNA expression. Primers and their sequences for the qPCR are described in Table 2. For the in vitro experiments, all the experiments were performed in triplicate but also performed twice to ensure replication.
2.6. Human small-interfering RNAs
Human small-interfering RNAs (siRNA) were transfected into H4 cells using Lipofectamine RNAiMAX (Thermo Fisher Scientific). The sequences for the sense oligonucleotides of the knockdown constructs were human si-TG (1: CCUUAUGAGUUCUCACGGAtt and 2: GCUGCUACAUGGUAUUACUtt; Ambion Silencer Select siRNA, Thermo Fisher Scientific), human si-p65 (Ambion Silencer Select RELA siRNA, Thermo Fisher Scientific), and human siRNA negative control (Ambion Negative Control #1 siRNA, Thermo Fisher Scientific).
2.7. Enzyme-linked immunosorbent assay (ELISA) detection of serum TG levels
Serum samples from 22 MD-ICRP, 22 MD-Control, and 36 juvenile MDs were enrolled in this study. For the 44 adult MDs, blood samples were collected from April 2017 to April 2021 by a licensed veterinarian or a supervised veterinary student by venipuncture during the dogs' first visits to the Hokkaido University Veterinary Teaching Hospital (HUVTH) for routine diagnostics with written informed consent obtained from the owners. For the juvenile MDs, blood samples were collected by a licensed veterinarian (YBT) using a venipuncture. The juvenile MDs were owned by a small and medium enterprise pet store in Hokkaido, Japan; written informed consent was obtained from the enterprise's owner and veterinarian on duty, and sample collection was performed from November 2021 to February 2022. Serum samples separated after centrifugation were stored at −80°C until the TG analysis. Serum TG concentrations were measured as per protocol provided by the manufacturer using the Canine Thyroglobulin ELISA Kit (MyBioSource, San Diego, CA, USA, Catalog No: MBS2608140). The intra-assay coefficient of variability between sample replicates was < 20%. The information on the regional/systemic inflammation and medication (e.g., corticosteroids) is given in Supplementary Table 3.
2.8. Real-time quantitative PCR (qPCR) in canine colonic mucosa samples
For qPCR using canine colonic mucosa samples, seven MD-ICRP (three with T/T allele and four with C/C allele), which visited HUVTH between April 2017 and April 2021, were included; total RNA was extracted using an RNeasy Mini Kit (Qiagen, Valencia, CA, USA); and genomic DNA was removed using an RNase-free DNase Set (Qiagen) following the manufacturer's instructions. cDNA synthesis was then performed using oligo (dT) and M-MLV reverse transcriptase (Promega, Madison, Wisconsin, USA) from 1 μg total RNA as per the manufacturer's recommendation. Real-time TaqMan qPCR was performed using a commercially available set of a pre-designed probe and primers (Applied Biosystems) for TG (product no: Cf02701382_m1) with an endogenous control for colonic samples using SDHA (product no: Cf02664981_m1) confirmed by a previous study (41). Real-time qPCR was performed using TaqMan PCR probe master mix (KAPA Biosystems) and at the following cycle conditions: 40 cycles at 94°C for 3 s and 40 cycles at 60°C for 30 s. The relative TG mRNA expression levels were normalized to the level of SDHA mRNA expression. THUNDERBIRD SYBR qPCR Mix (TOYOBO Co. Ltd., Osaka, Japan) was used to quantify the levels of the target mRNA (IL6, CCL2) and internal control mRNA (succinate dehydrogenase complex, subunit A; SDHA). The condition for real-time qPCR was 40 cycles at 94°C for 15 s followed by 40 cycles at 60°C for 60 s. Relative IL6 and CCL2 mRNA expressions were normalized to the level of SDHA mRNA expression. The primers and their sequences for the qPCR are described in Table 3 and reported previously (42, 43). For the qPCR using these samples, all the experiments were performed in duplicates and performed twice to ensure replication.
2.9. Statistical analysis
Two-tailed Student's t-test and Fisher's exact test were used to analyze differences between the two groups. Values of P < 0.05 were considered significant.
3. Results
3.1. TG c.4567c>t (p.1523r>w) allele is increased in MDs with ICRP
The frequency of TG c.4567C>T (p.1523R>W) allele in MDs (n = 281) was 69.04% (48 homozygous and 246 heterozygous), which was significantly higher than in dogs of other breeds (22.5%; one homozygous and eight heterozygous, n = 40) (P < 0.0001, Figure 1A). Sequence chromatograms of the target variant are shown in Supplementary Figure 1A. Furthermore, the frequency of the T risk allele (84.52%) was significantly higher in MD-ICRP (median age = 10 years old; 34 homozygous, 97 heterozygous, n = 155) than in MD-controls (47.78%; median age = 12 years old; 9 homozygous, 34 heterozygous, n = 90) (P < 0.0001, Figure 1B). Thus, the TG c.4567C>T (p.1523R>W) allele was increased in MDs with ICRP. Finally, we found that one amino acid of the TG gene was conserved in dogs and other mammals (Supplementary Figures 1B, C).
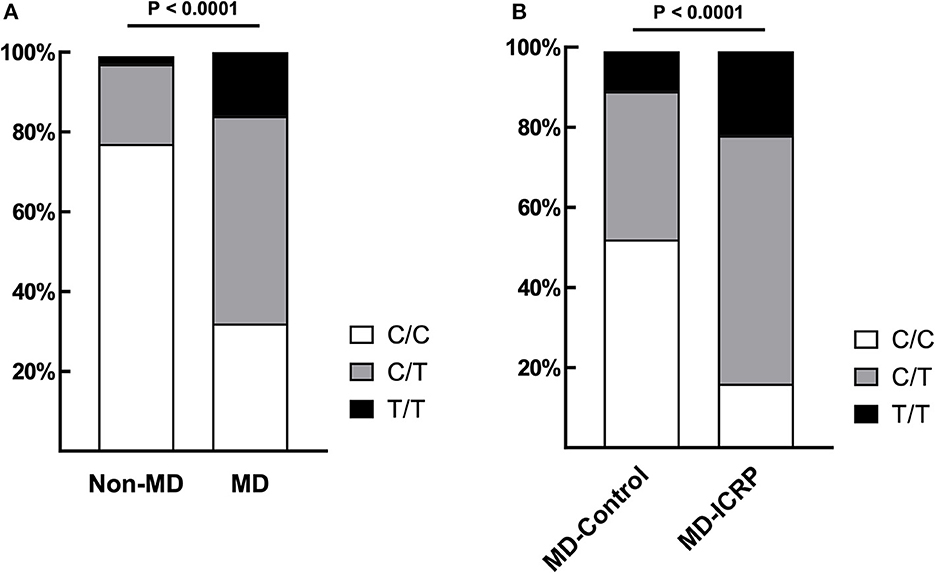
Figure 1. TG SNP is associated with ICRP in MDs. (A) The frequency of variant TG c.4567C>T (p.R1523W) was significantly higher in MDs (n = 281) when compared to other breeds (n = 40). p < 0.0001. (B) Among MDs, age-matched, case–controls showed that the same variant was significantly higher in MD-ICRP (n = 155) than MD-Control (n = 90). p < 0.0001.
3.2. TG is a positive regulator and target of the IL-6 amplifier activation
Because we found that TG is one positive regulator candidate of the IL-6 amplifier (39) and showed that TG c.4567C>T (p.1523R>W) allele is increased in MDs with ICRP, we hypothesized that TG is functionally involved in ICRP development via activation of the IL-6 amplifier. Consistently, we found that TG expression was increased during the activation of the IL-6 amplifier (Figures 2A, B), suggesting that TG is a target gene of the IL-6 amplifier. We then treated H4 cells, a human neuroglioma cell line, with siRNA of TG to investigate cytokine-mediated IL-6 amplifier (39) and found that IL-6 expression and TG expression were reduced after cytokine stimulation (Figures 3A, B). Furthermore, recombinant human TG enhanced IL-6 amplifier activation in H4 cells (Figure 3C). These results suggested that TG is both a positive regulator and a target of the IL-6 amplifier.
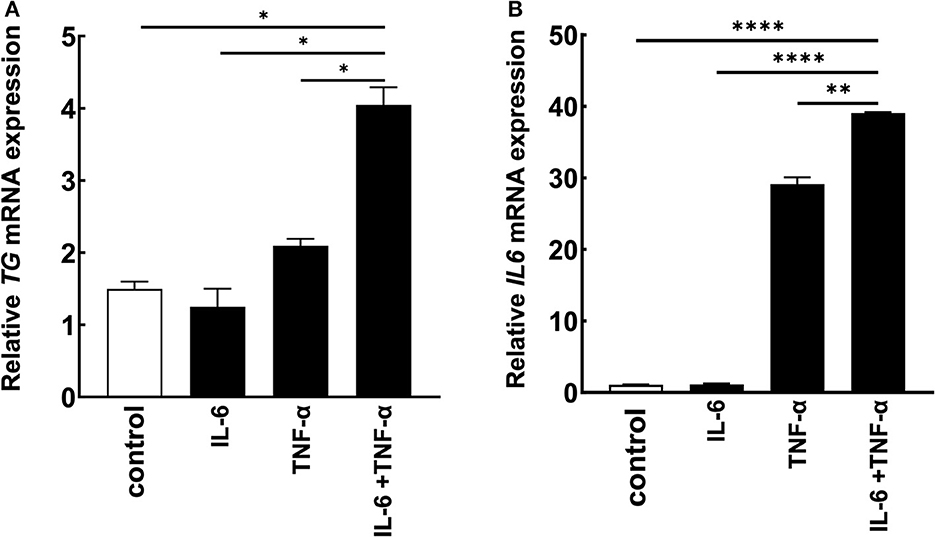
Figure 2. TG is a potential target of the IL-6 amplifier in vitro. (A, B) H4 cells were stimulated with cytokines, and IL6 and TG levels were assessed. Means ± standard deviations are shown. *p < 0.05, **p < 0.01, ****p < 0.0001.
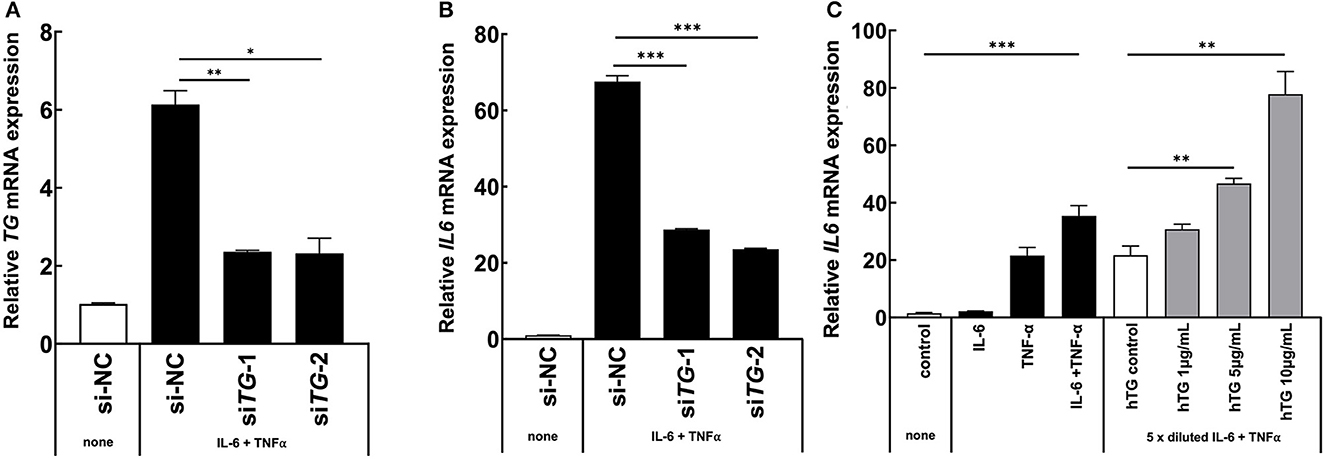
Figure 3. TG is critical for IL-6 amplifier activation in vitro. (A, B) H4 cells were transfected with two different siRNAs for all TG variants or a siRNA negative control. IL6 levels and TG knockdown efficiency were assessed. Means ± standard deviations are shown. *p < 0.05, **p < 0.01, ***p < 0.005. (C) H4 cells were stimulated with cytokines and recombinant human TG. IL6 levels were assessed. Means ± standard deviations are shown. *p < 0.05, **p < 0.01, ***p < 0.005.
3.3. TG is highly expressed and the IL-6 amplifier activation is enhanced in the colon of MD-ICRP with risk allele
We hypothesized that the TG SNP functions to increase TG expression either systematically or locally. To test this hypothesis, we investigated the serum concentrations of TG but found they were unchanged in MDs with or without ICRP and with or without the risk T/T SNP (Figures 4A–C). In addition, we found no difference in serum TG concentrations between dogs receiving systemic anti-inflammatory medication or across genotypes (Supplementary Figures 2A–C). We then investigated the expression levels of TG in the non-inflammatory colonic mucosa of MD-ICRP. We found that TG expression in samples with the risk T/T SNP was significantly higher than those with the non-risk C/C SNP (Figure 5A), suggesting that the TG SNP is an expression quantitative trait locus (eQTL) in the colon of MDs. We next investigated whether TG is induced by the IL-6 amplifier in the colon. Because the IL-6 amplifier reflects the activation of NF-κB in non-immune cells, we analyzed the expression of two NF-κB targets, IL6 and CCL2, in the same colon samples. We found that IL6 and CCL2 were also enhanced in colon samples with the risk T/T SNP (Figures 5B, C). These results indicate that the risk T/T SNP of TG is an eQTL and enhances TG expression. They also indicate that TG is involved in the activation of the IL-6 amplifier in the colon of MD-ICRP. Therefore, the risk T/T SNP of TG may be critical in the pathogenesis of ICRP through the activation of the IL-6 amplifier.
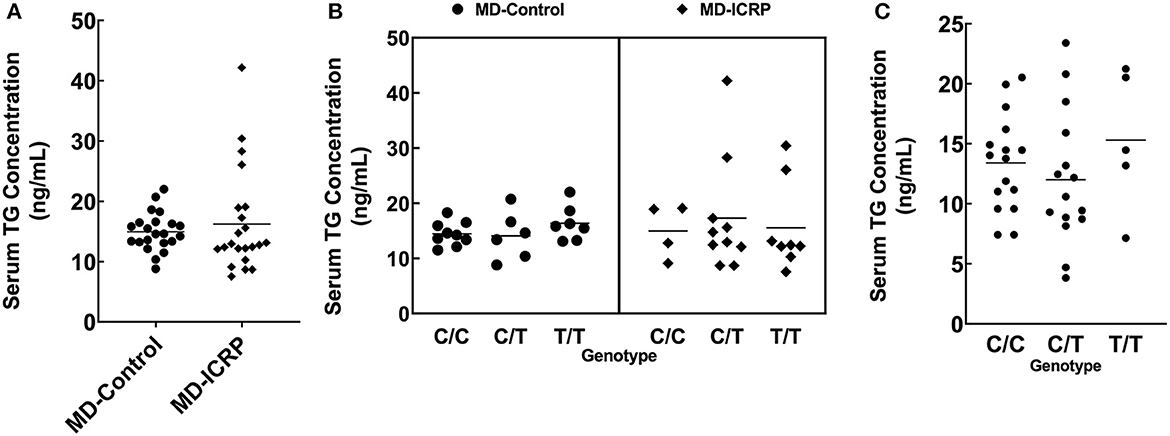
Figure 4. The TG SNP does not affect systemic TG levels. (A) Serum TG concentration between age-controlled MD-Control and MD-ICRP groups. (B) Serum TG concentration between genotypes in MD-Control and MD-ICRP groups. (C) Serum TG concentration between genotypes in MDs <1-year-old.
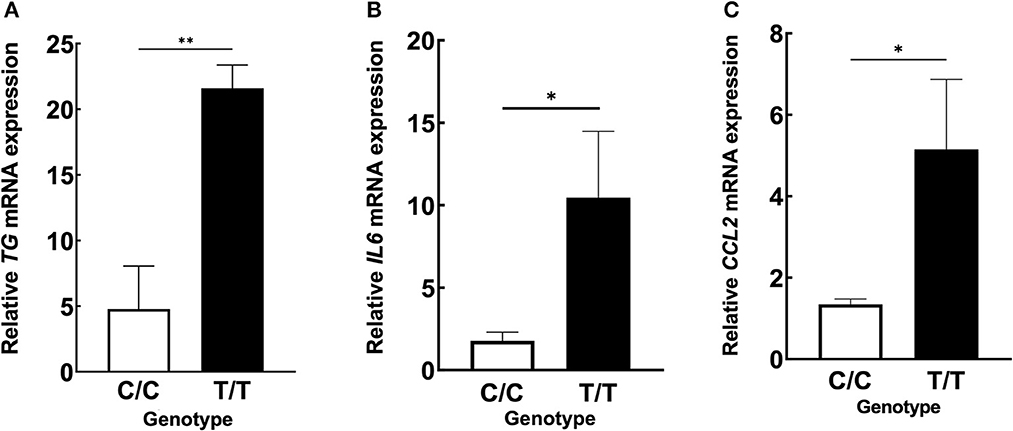
Figure 5. ICRP-MDs with risk alleles have a higher expression of TG and NF-κB-related chemokines in non-inflammatory colonic mucosa. (A) Relative TG, (B) IL6, and (C) CCL2 mRNA expressions in canine non-inflammatory colonic mucosa adjacent to the ICRP lesion site and diagnosed as normal histopathologically in MDs with wild-type (C/C) and risk (T/T) alleles. Means ± standard error of means is shown. *p < 0.05, **p < 0.005.
4. Discussion
ICRP has been reported to be breed-associated (1, 44, 45). We recently identified several disease-associated SNPs, such as PLG, TCOF1, TG, COL9A2, and COL4A4, and found that PLG SNP is associated with IL-6 amplifier activation in the colon (12). In this study, we show that the risk SNP of TG is associated with the pathogenesis of ICRP in MDs and that the increased expression of TG is associated with the risk allele T/T in the non-inflammatory colonic mucosa, showing that TG possesses a biological role in the activation of NF-κB, which is a critical component of the IL-6 amplifier.
TG is commonly known as the precursor protein to thyroid hormones T3 and T4, which regulate multiple metabolic pathways in the mammalian body (46). Recent advances have shown that TG may function both inside and outside of the thyroid, which is no surprise as TG expression was detected in multiple non-thyroidal cells such as human and mouse kidney cells (47). Additionally, TG mRNA is expressed in many organs including the testis, suprarenal gland, appendix, lung, and thymus, as well as the hypophysis, lymphocytes, and leukocytes (48–52). Here, we showed that TG mRNA is expressed in the colon, especially in MDs with the risk allele T/T of TG SNP. Considering that TG expression with the risk alleles in the colon correlated with the development of ICRPs in MDs, TG-mediated activation of the IL-6 amplifier in the intestine may contribute to the high concurrent occurrence of thyroidal disease and inflammatory bowel disease in human patients (53–56).
Because the local expression of TG was higher in the risk allele T/T group compared with the wild-type allele C/C group, we concluded that the function of the SNP is to induce a higher local expression of TG in the colon (Figure 5A) but not in the circulation. The localized increase in TG mRNA levels was accompanied by localized increases in IL6 and CCL2 expression in non-inflammatory colonic mucosa (Figures 5B, C), suggesting that the IL-6 amplifier is activated in the colon of MDs with the risk allele T/T. This result may explain why ICRP only occurs in the colorectal region, although more studies of the expression of TG and other NF-κB targets in other organs, including other regions of the gastrointestinal tract, with or without the risk allele T/T of TG SNP, are needed. Because we found that MDs without the risk allele T/T (C/T or C/C) were affected with ICRP, we hypothesize that one of the reasons is that ICRP in MDs is a polygenic disease and other mechanisms may also be involved in its pathogenesis, although the number of cases for the qPCR analysis in canine colonic mucosa is too small to verify this conclusion. In addition, we are not able to confidently state that there is no blood relationship between the dogs because we do not have the pedigree of each dog used in this study.
In summary, we found a novel SNP variant, TG c.4567C>T (p.R1523W), which is involved in the pathogenesis of ICRP in MDs. We also suggested that TG has a functional role in the development of ICRP in MDs through the IL-6 amplifier in colon cells and that the presence of the risk allele T/T correlates with a higher expression of TG locally compared to allele C/C. Our results also suggest that the T/T SNP is an eQTL of TG mRNA in the colon and the local TG expression triggered by this SNP increases the risk of ICRP in MDs via activation of the IL-6 amplifier. Therefore, TG c.4567C>T is a potential diagnostic target for ICRP in MDs and TG-mediated IL-6 amplifier activation in the colon is a possible therapeutic target for ICRP.
Data availability statement
Publicly available datasets were analyzed in this study. This data can be found at: https://academic.oup.com/intimm/advance-article/doi/10.1093/intimm/dxad006/7080519.
Ethics statement
Ethical review and approval was not required for the animal study because samples retrieved from the animals were part of the diagnostic workup for the disease, including blood test, lower gastrointestinal endoscopy, and biopsy and histopathology submission. Written informed consent was obtained from the owners for the participation of their animals in this study.
Author contributions
MM designed the study and edited the manuscript. J-JJ and YT performed most of the experiments. RH, TY, and NN contributed to the experiments. HO provided the canine samples. KN, NS, and NY advised on the research design. YK confirmed the diagnosis of the canine pathology. YT, MT, and MM wrote the manuscript. MM and MT supervised the study. All authors have read and agreed to the published version of the manuscript.
Funding
This study was supported by JSPS KAKENHI (MT and MM), AMED Grant Numbers JP21zf0127004 and JP22zf0127004 (MM), the Joint Usage/Research Center Institute for Genetic Medicine, Hokkaido University (MM), the Photo-excitonix Project, MEXT Quantum Leap Flagship Program (MEXT Q-LEAP) Grant Number JPMXS0120330644, and the Project of Young Investigator Promotion at Hokkaido University (MM).
Acknowledgments
We thank Dr. P. Karagiannis (CiRA, Kyoto University, Kyoto, Japan) for carefully reading the manuscript, Ms. C. Nakayama and Dr. N. Maekawa for their excellent technical assistance, and Ms. N. Yamamoto, Ms. M. Ohsawa, and Ms. Y. Fujimoto for their excellent secretarial assistance. We would also like to thank all the staff of HUVTH for their kind support in the sample collection for this study. The authors also acknowledge Dr. M. Yuki, Dr. K. Ohmori, Dr. H. Ichihashi, Dr. K. Fujita, and Dr. K. Ueda for providing MD blood samples.
Conflict of interest
The authors declare that the research was conducted in the absence of any commercial or financial relationships that could be construed as a potential conflict of interest.
Publisher's note
All claims expressed in this article are solely those of the authors and do not necessarily represent those of their affiliated organizations, or those of the publisher, the editors and the reviewers. Any product that may be evaluated in this article, or claim that may be made by its manufacturer, is not guaranteed or endorsed by the publisher.
Supplementary material
The Supplementary Material for this article can be found online at: https://www.frontiersin.org/articles/10.3389/fvets.2023.1192888/full#supplementary-material
Supplementary Figure 1. (A) Sequence chromatograms of TG c.4567C>T (p.R1523W) in wild-type, heterozygote, and homozygote variant samples. (B) A schematic diagram of the exon of canine TG gene and thyroglobulin protein. SP, Signal protein; TGT1, Thyroglobulin Repeat Type 1. (C) Alignment of the amino acid sequence in the final thyroglobulin type-1 repeat domain of canine thyroglobulin against selected mammals using the UniProt repository (https://www.uniprot.org/). Asterisks (*): complete conservation. Colon (:): strongly similar. Period (.): weakly similar properties. R1523 in dogs is strongly similar to other mammals but is highly conserved among other mammals.
Supplementary Figure 2. The systemic anti-inflammatory drug does not affect systemic TG levels. (A) Serum TG concentration between MDs that are treated with or without anti-inflammatory drugs. (B) Serum TG concentration between MD-control and MD-ICRP groups that are treated with or without anti-inflammatory drugs. (C) Serum TG concentration between genotypes of MDs that are treated with or without anti-inflammatory drugs.
Supplementary Table 1. Details of MDs and other breeds included in this study.
Supplementary Table 2. Case details of MDs recruited for qPCR analysis.
Supplementary Table 3. Case details of MDs recruited for serum TG evaluation.
References
1. Ohmi A, Tsukamoto A, Ohno K, Uchida K, Nishimura R, Fukushima K, et al. retrospective study of inflammatory colorectal polyps in miniature dachshunds. J Vet Med Sci. (2012) 74:59–64. doi: 10.1292/jvms.11-0352
2. Uchida E, Chambers JK, Nakashima K, Saito T, Ohno K, Tsujimoto H, et al. Pathologic features of colorectal inflammatory polyps in miniature dachshunds. Vet Pathol. (2016) 53:833–9. doi: 10.1177/0300985815618436
3. Saito T, Chambers JK, Nakashima K, Uchida E, Ohno K, Tsujimoto H, et al. Histopathologic features of colorectal adenoma and adenocarcinoma developing within inflammatory polyps in miniature dachshunds. Vet Pathol. (2018) 55:654–62. doi: 10.1177/0300985818777798
4. Murakami A, Shibahashi A, Iwasaki R, Kawabe M, Murakami M, Sakai H, et al. Combination chlorambucil/firocoxib or chlorambucil/prednisolone treatment for inflammatory colorectal polyps in miniature dachshunds. J Am Anim Hosp Assoc. (2018) 54:161–6. doi: 10.5326/JAAHA-MS-6663
5. Fukushima K, Eguchi N, Ohno K, Kanemoto H, Takahashi M, Igarashi H, et al. Efficacy of leflunomide for treatment of refractory inflammatory colorectal polyps in 15 miniature dachshunds. J Vet Med Sci. (2016) 78:265–9. doi: 10.1292/jvms.15-0129
6. Igarashi H, Ohno K, Fujiwara-Igarashi A, Kanemoto H, Fukushima K, Uchida K, et al. Inflammatory colorectal polyps in miniature Dachshunds frequently develop ventrally in the colorectal mucosa. Vet J. (2015) 203:256–8. doi: 10.1016/j.tvjl.2014.12.005
7. Ohta H, Takada K, Torisu S, Yuki M, Tamura Y, Yokoyama N, et al. Expression of CD4+ T cell cytokine genes in the colorectal mucosa of inflammatory colorectal polyps in miniature dachshunds. Vet Immunol Immunopathol. (2013) 155:259–63. doi: 10.1016/j.vetimm.2013.07.006
8. Tamura Y, Ohta H, Torisu S, Yuki M, Yokoyama N, Murakami M, et al. Markedly increased expression of interleukin-8 in the colorectal mucosa of inflammatory colorectal polyps in miniature dachshunds. Vet Immunol Immunopathol. (2013) 156:32–42. doi: 10.1016/j.vetimm.2013.09.017
9. Yokoyama N, Ohta H, Yamazaki J, Kagawa Y, Ichii O, Khoirun N, et al. Localization of toll-like receptor (TLR) 2 and TLR4 mRNA in the colorectal mucosa of miniature dachshunds with inflammatory colorectal polyps. J Comp Pathol. (2017) 156:183–90. doi: 10.1016/j.jcpa.2016.10.010
10. Konishi K, Igarashi H, Maeda S, Uchida E, Hanazono K, Tamamoto T, et al. Distribution of regulatory T cells in inflammatory colorectal polyps of miniature dachshunds. Vet Immunol Immunopathol. (2019) 218:109938. doi: 10.1016/j.vetimm.2019.109938
11. Igarashi H, Ohno K, Uchida E, Fujiwara-Igarashi A, Kanemoto H, Fukushima K, et al. Polymorphisms of nucleotide-binding oligomerization domain 2 (NOD2) gene in miniature dachshunds with inflammatory colorectal polyps. Vet Immunol Immunopathol. (2015) 164:160–9. doi: 10.1016/j.vetimm.2015.02.005
12. Yamasaki T, Nagata N, Atsumi T, Hasebe R, Tanaka Y, Ohki I, et al. Zoobiquity experiments show the importance of the local MMP9-plasminogen axis in inflammatory bowel diseases in both dogs and patients. Int Immunol. (2023) doi: 10.1093/INTIMM/DXAD006
13. Murakami M, Kamimura D, Hirano T. Pleiotropy and specificity: insights from the interleukin 6 family of cytokines. Immunity. (2019) 50:812–31. doi: 10.1016/j.immuni.2019.03.027
14. Ogura H, Murakami M, Okuyama Y, Tsuruoka M, Kitabayashi C, Kanamoto M, et al. Interleukin-17 promotes autoimmunity by triggering a positive-feedback loop via interleukin-6 induction. Immunity. (2008) 29:628–36. doi: 10.1016/J.IMMUNI.2008.07.018
15. Stofkova A, Kamimura D, Ohki T, Ota M, Arima Y, Murakami M. Photopic light-mediated down-regulation of local α 1A -adrenergic signaling protects blood-retina barrier in experimental autoimmune uveoretinitis. Sci Rep. (2019) 9:1–15. doi: 10.1038/s41598-019-38895-y
16. Murakami K, Kamimura D, Hasebe R, Uchida M, Abe N, Yamamoto R, et al. Rhodobacter azotoformans LPS (RAP99-LPS) is a TLR4 agonist that inhibits lung metastasis and enhances TLR3-mediated chemokine expression. Front Immunol. (2021) 12:1638. doi: 10.3389/FIMMU.2021.675909/BIBTEX
17. Tanaka H, Arima Y, Kamimura D, Tanaka Y, Takahashi N, Uehata T, et al. Phosphorylation-dependent Regnase-1 release from endoplasmic reticulum is critical in IL-17 response. J Exp Med. (2019) 216:1431–49. doi: 10.1084/JEM.20181078
18. Atsumi T, Suzuki H, Jiang J-JJ, Okuyama Y, Nakagawa I, Ota M, et al. Rbm10 regulates inflammation development via alternative splicing of Dnmt3b. Int Immunol. (2017) 29:581–91. doi: 10.1093/intimm/dxx067
19. Murakami M, Okuyama Y, Ogura H, Asano S, Arima Y, Tsuruoka M, et al. Local microbleeding facilitates IL-6-and IL-17-dependent arthritis in the absence of tissue antigen recognition by activated T cells. J Exp Med. (2011) 208:103–14. doi: 10.1084/jem.20100900
20. Murakami M, Hirano T, A. four-step model for the IL-6 amplifier, a regulator of chronic inflammations in tissue-specific MHC class II-associated autoimmune diseases. Front Immunol. (2011) 2:22. doi: 10.3389/FIMMU.2011.00022/BIBTEX
21. Arima Y, Harada M, Kamimura D, Park J-HH, Kawano F, Yull FEE, et al. Regional neural activation defines a gateway for autoreactive t cells to cross the blood-brain barrier. Cell. (2012) 148:447–57. doi: 10.1016/j.cell.2012.01.022
22. Okuyama Y, Tanaka Y, Jiang J-J, Kamimura D, Nakamura A, Ota M, et al. Bmi1 regulates IκBα degradation via association with the SCF complex. J Immunol. (2018) 201:2264–72. doi: 10.4049/jimmunol.1701223
23. Ohtani T, Ishihara K, Atsumi T, Nishida K, Kaneko Y, Miyata T, et al. Dissection of signaling cascades through gp130 in vivo: Reciprocal roles for STAT3- and SHP2-mediated signals in immune responses. Immunity. (2000) 12:95–105. doi: 10.1016/S1074-7613(00)80162-4
24. Sawa SI, Kamimura D, Jin GH, Morikawa H, Kamon H, Nishihara M, et al. Autoimmune arthritis associated with mutated interleukin (IL)-6 receptor gp130 is driven by STAT3/IL-7–dependent homeostatic proliferation of CD4+ T cells. J Exp Med. (2006) 203:1459. doi: 10.1084/JEM.20052187
25. Hirano T. IL-6 in inflammation, autoimmunity and cancer. Int Immunol. (2021) 33:127–48. doi: 10.1093/INTIMM/DXAA078
26. Higuchi H, Kamimura D, Jiang J-J, Atsumi T, Iwami D, Hotta K, et al. Orosomucoid 1 is involved in the development of chronic allograft rejection after kidney transplantation. Int Immunol. (2020) 32:335–46. doi: 10.1093/intimm/dxaa003
27. Fujita M, Yamamoto Y, Jiang JJ, Atsumi T, Tanaka Y, Ohki T, et al. NEDD4 is involved in inflammation development during keloid formation. J Investigat Dermatol. (2019) 139:333–41. doi: 10.1016/j.jid.2018.07.044
28. Hasebe R, Murakami K, Harada M, Halaka N, Nakagawa H, Kawano F, et al. ATP spreads inflammation to other limbs through crosstalk between sensory neurons and interneurons. J Exp Med. (2022) 219:e20212019. doi: 10.1084/JEM.20212019
29. Arima Y, Kamimura D, Atsumi T, Harada M, Kawamoto T, Nishikawa N, et al. A pain-mediated neural signal induces relapse in murine autoimmune encephalomyelitis, a multiple sclerosis model. Elife. (2015) 4:8733. doi: 10.7554/ELIFE.08733
30. Takada Y, Kamimura D, Jiang J-J, Higuchi H, Iwami D, Hotta K, et al. Increased urinary exosomal SYT17 levels in chronic active antibody-mediated rejection after kidney transplantation via the IL-6 amplifier. Int Immunol. (2020) 32:653–62. doi: 10.1093/intimm/dxaa032
31. Kida H, Jiang J-J, Matsui Y, Takahashi I, Hasebe R, Kawamura D, et al. Dupuytren's contracture-associated SNPs increase SFRP4 expression in nonimmune cells including fibroblasts to enhance inflammation development. Int Immunol. (2023). doi: 10.1093/INTIMM/DXAD004
32. Meng J, Jiang J-J, Atsumi T, Bando H, Okuyama Y, Sabharwal L, et al. Breakpoint cluster region–mediated inflammation is dependent on casein kinase II. J Immunol. (2016) 197:3111–9. doi: 10.4049/jimmunol.1601082
33. Lee J, Nakagiri T, Oto T, Harada M, Morii E, Shintani Y, et al. IL-6 Amplifier, NF-κB–Triggered Positive Feedback for IL-6 Signaling, in Grafts Is Involved in Allogeneic Rejection Responses. J Immunol. (2012) 189:1928–36. doi: 10.4049/jimmunol.1103613
34. Shimoyama S, Nakagawa I, Jiang JJ, Matsumoto I, Chiorini JA, Hasegawa Y, et al. Sjögren's syndrome-associated SNPs increase GTF2I expression in salivary gland cells to enhance inflammation development. Int Immunol. (2021) 33:423–34. doi: 10.1093/intimm/dxab025
35. Lee J, Nakagiri T, Kamimura D, Harada M, Oto T, Susaki Y, et al. IL-6 amplifier activation in epithelial regions of bronchi after allogeneic lung transplantation. Int Immunol. (2013) 25:319–32. doi: 10.1093/INTIMM/DXS158
36. Tanaka Y, Sabharwal L, Ota M, Nakagawa I, Jiang J-J, Arima Y, et al. Presenilin 1 regulates NF-κB activation via association with breakpoint cluster region and casein kinase II. J Immunol. (2018) 201:2256–63. doi: 10.4049/jimmunol.1701446
37. Arima Y, Ohki T, Nishikawa N, Higuchi K, Ota M, Tanaka Y, et al. Brain micro-inflammation at specific vessels dysregulates organ-homeostasis via the activation of a new neural circuit. Elife. (2017) 6: 5517. doi: 10.7554/ELIFE.25517
38. Ota M, Tanaka Y, Nakagawa I, Jiang JJ, Arima Y, Kamimura D, et al. Role of chondrocytes in the development of rheumatoid arthritis via transmembrane protein 147–mediated NF-κB activation. Arthritis Rheumatology. (2020) 72:931–42. doi: 10.1002/ART.41182
39. Murakami M, Harada M, Kamimura D, Ogura H, Okuyama Y, Kumai N, et al. Disease-association analysis of an inflammation-related feedback loop. Cell Rep. (2013) 3:946–59. doi: 10.1016/j.celrep.2013.01.028
40. Day MJ, Bilzer T, Mansell J, Wilcock B, Hall EJ, Jergens A, et al. Histopathological standards for the diagnosis of gastrointestinal inflammation in endoscopic biopsy samples from the dog and cat: a report from the world small animal veterinary association gastrointestinal standardization group. J Comp Pathol. (2008) 138:1–43. doi: 10.1016/j.jcpa.2008.01.001
41. Tamura Y, Ohta H, Yokoyama N, Lim SY, Osuga T, Morishita K, et al. Evaluation of selected cytokine gene expression in colonic mucosa from dogs with idiopathic lymphocytic-plasmacytic colitis. J Vet Med Sci. (2014) 76:1407–10. doi: 10.1292/jvms.13-0635
42. Ohta H, Takada K, Sunden Y, Tamura Y, Osuga T, Lim SY, et al. CD4+T cell cytokine gene and protein expression in duodenal mucosa of dogs with inflammatory bowel disease. J Vet Med Sci. (2014) 76:409–14. doi: 10.1292/jvms.13-0008
43. Tani A, Tomiyasu H, Asada H, Lin CS, Goto-Koshino Y, Ohno K, et al. Changes in gene expression profiles and cytokine secretions in peripheral monocytes by treatment with small extracellular vesicles derived from a canine lymphoma cell line. J Vet Med Sci. (2022) 84:712–9. doi: 10.1292/JVMS.21-0506
44. Igarashi H, Ohno K, Maeda S, Kanemoto H, Fukushima K, Uchida K, et al. Expression profiling of pattern recognition receptors and selected cytokines in miniature dachshunds with inflammatory colorectal polyps. Vet Immunol Immunopathol. (2014) 159:1–10. doi: 10.1016/j.vetimm.2014.03.003
45. Ohta H, Tamura Y, Yokoyama N, Nagata N, Osuga T, Sasaki N, et al. Gene expression of leucine-rich alpha-2 glycoprotein in the polypoid lesion of inflammatory colorectal polyps in miniature dachshunds. J Vet Med Sci. (2020) 82:1445–9. doi: 10.1292/jvms.20-0242
46. Di Jeso B, Arvan P. Thyroglobulin from molecular and cellular biology to clinical endocrinology. Endocr Rev. (2016) 37:2–36. doi: 10.1210/er.2015-1090
47. Wu H, Suzuki S, Sellitti DF, Doi SQ, Tanigawa K, Aizawa S, et al. Expression of a thyroglobulin (Tg) variant in mouse kidney glomerulus. Biochem Biophys Res Commun. (2009) 389:269–73. doi: 10.1016/j.bbrc.2009.08.129
48. Bojunga J, Röddiger S, Stanisch M, Kusterer K, Kurek R, Renneberg H, et al. Molecular detection of thyroglobulin mRNA transcripts in peripheral blood of patients with thyroid disease by RT-PCR. Br J Cancer. (2000) 82:1650–5. doi: 10.1054/bjoc.1999.1209
49. Bugalho MJ, Domingues RS, Pinto AC, Garrão A, Catarino AL, Ferreira T, et al. Detection of thyroglobulin mRNA transcripts in peripheral blood of individuals with and without thyroid glands: evidence for thyroglobulin expression by blood cells. Eur J Endocrinol. (2001) 145:409–13. doi: 10.1530/eje.0.1450409
50. Bojunga J, Roddiger S, Stanisch M, Kusterer K, Kurek R, Rennenberg H, et al. Molecular detection of thyroglobulin mRNA transcripts in peripheral blood of patients with thyroid disease by RT-PCR. Br J Cancer. (2000) 82:1650–5. doi: 10.1054/BJOC.1999.1209
51. Amakawa M, Kato R, Kameko F, Maruyama M, Tajiri J. Thyroglobulin mRNA expression in peripheral blood lymphocytes of healthy subjects and patients with thyroid disease. Clinica Chimica Acta. (2008) 390:97–103. doi: 10.1016/j.cca.2008.01.007
52. Bilal MY, Dambaeva S, Kwak-Kim J, Gilman-Sachs A, Beaman KD, A. role for iodide and thyroglobulin in modulating the function of human immune cells. Front Immunol. (2017) 8:1–13. doi: 10.3389/fimmu.2017.01573
53. Bianchi GP, Marchesini G, Gueli C, Zoli M. Thyroid involvement in patients with active inflammatory bowel diseases. Ital J Gastroenterol. (1995) 27:291–5.
54. Cesarini M, Angelucci E, Rivera M, Pica R, Paoluzi P, Vernia P, et al. Thyroid disorders and inflammatory bowel diseases: Retrospective evaluation of 909 patients from an Italian Referral Center. Inflamm Bowel Dis. (2010) 16:186–7. doi: 10.1002/ibd.20964
55. Shizuma T. Concomitant thyroid disorders and inflammatory bowel disease: a literature review. Biomed Res Int. (2016) 2016:5187061. doi: 10.1155/2016/5187061
Keywords: miniature dachshund, canine, inflammatory colorectal polyps, SNP, thyroglobulin, inflammation, IL-6 amplifier
Citation: Teoh YB, Jiang J-J, Yamasaki T, Nagata N, Sugawara T, Hasebe R, Ohta H, Sasaki N, Yokoyama N, Nakamura K, Kagawa Y, Takiguchi M and Murakami M (2023) An inflammatory bowel disease-associated SNP increases local thyroglobulin expression to develop inflammation in miniature dachshunds. Front. Vet. Sci. 10:1192888. doi: 10.3389/fvets.2023.1192888
Received: 24 March 2023; Accepted: 21 June 2023;
Published: 14 July 2023.
Edited by:
Isa Ozaydin, Kafkas University, TürkiyeReviewed by:
Hirotaka Igarashi, Azabu University, JapanMichelle Wai Cheng Fong, Putra Malaysia University, Malaysia
Copyright © 2023 Teoh, Jiang, Yamasaki, Nagata, Sugawara, Hasebe, Ohta, Sasaki, Yokoyama, Nakamura, Kagawa, Takiguchi and Murakami. This is an open-access article distributed under the terms of the Creative Commons Attribution License (CC BY). The use, distribution or reproduction in other forums is permitted, provided the original author(s) and the copyright owner(s) are credited and that the original publication in this journal is cited, in accordance with accepted academic practice. No use, distribution or reproduction is permitted which does not comply with these terms.
*Correspondence: Masaaki Murakami, bXVyYWthbWlAaWdtLmhva3VkYWkuYWMuanA=; Mitsuyoshi Takiguchi, bXRha2lAdmV0bWVkLmhva3VkYWkuYWMuanA=
†These authors have contributed equally to this work