- 1School of Animal and Veterinary Sciences, The University of Adelaide, Roseworthy, SA, Australia
- 2Bioproperties Pty Ltd, Ringwood, VIC, Australia
- 3School of Science, RMIT University, Bundoora, VIC, Australia
- 4U. S. National Poultry Research Center, USDA Agricultural Research Service, Athens, GA, United States
Among the Salmonella reduction strategies in poultry production, one option is to use a Salmonella vaccine. The aim of vaccinating layer flocks is to reduce the shedding of wild-type Salmonella in the poultry environment, thereby reducing the contamination of poultry products (eggs and meat). Nutritive diluent and a higher dose of vaccine may enhance its colonization potential in the gut of chickens. In this study, a commercially available live attenuated vaccine (Vaxsafe® ST) was reconstituted in different media and delivered orally to day-old chicks at three different doses (107, 108, and 109 CFU/chick). Gut colonization of the vaccine strain and the effects of vaccination on gut microbiota were assessed in commercial-layer chickens. The vaccine diluent and dosage minimally affected microbiota alpha diversity. Microbiota beta diversity was significantly different (P < 0.05) based on the vaccine diluent and dose, which indicated that the vaccinated and unvaccinated chickens had different gut microbial communities. Differences were noted in the abundance of several genera, including Blautia, Colidextribacter, Dickeya, Enterococcus, Lactobacillus, Pediococcus, and Sellimonas. The abundance of Colidextribacter was significantly lower in chickens that received vaccine reconstituted in Marek's and water diluents, while Lactobacillus abundance was significantly lower in the water group. The highest vaccine dose (109 CFU/chick) did not significantly alter (P > 0.05) the abundance of microbial genera. Chicken age affected the microbiota composition more significantly than the vaccine dose and diluent. The abundance of Lactobacillus, Blautia, Caproiciproducens, Pediococcus, and Colidextribacter was significantly higher on day 14 compared with day 7 post-vaccination. The Salmonella Typhimurium vaccine load in the caeca was not significantly affected by diluent and vaccine dose; however, it was significantly lower (P < 0.0001) on day 14 compared with day 7 post-vaccination. Overall, the S. Typhimurium vaccine minimally affected the gut microbiota structure of layer chicks, whereas changes in microbiota were more significant with chicken age.
Introduction
Pathogenic serotypes of Salmonella associated with food-producing animals cause salmonellosis in humans if contaminated products are consumed. According to the World Health Organization (WHO), Salmonella is one of four key causes of diarrheal diseases globally. In European Union member countries, a total of 50,817 human salmonellosis cases were reported in 2021 (1). In Australia, in 2021, the National Notifiable Disease Surveillance System documented a total of 10,828 reported cases of human salmonellosis. Chicken meat and eggs are a significant source of human salmonellosis (2). Therefore, strategies to reduce Salmonella contamination at the farm level are one way to reduce human salmonellosis.
Among the Salmonella reduction strategies at the farm level, vaccination of flocks plays a pivotal role. Vaccinated flocks shed lower levels of Salmonella, and this results in reduced contamination of eggs and meat during egg grading or meat processing (3). In Australia, many commercial layer breeders, broiler breeders, and commercial layers are vaccinated with a live, attenuated Salmonella Typhimurium vaccine, Vaxsafe® ST (Bioproperties Pty Ltd). Vaxsafe ST is an aroA mutant, freeze-dried vaccine that is normally reconstituted in sterile water for spray and oral administration. The aroA mutation disrupts the shikimate biochemical pathway, thus disrupting the production of essential aromatic amino acids, which prevents growth in the host as these compounds are not freely available. Previous studies of Vaxsafe ST in chickens showed that it was partially effective in protecting chickens from wild-type Salmonella infection (4, 5). The other forms of Salmonella vaccines, autogenous or killed vaccines, are not commonly used in the Australian poultry industry. A study comparing the effects of live, attenuated versus multivalently killed vaccines showed that the killed vaccine provided short-term protection, whereas the injected live, attenuated vaccine produced longer-lasting protection (4). The limitation of autogenous vaccines is that they must be administered multiple times for priming and the generation of long-term immune responses. Multiple administrations of an injected vaccine make it impractical, particularly considering chicken flock size and the associated labor cost. The live, attenuated vaccine registered in Australia, Vaxsafe ST, is administered on day 1 via coarse spray, at weeks 2 to 4 via drinking water, and at week 12 of chicken age through intramuscular injection, a vaccination protocol that is economically feasible and widely adopted. For intramuscular administration at 12 weeks of chicken age, this vaccine is either reconstituted in water or Marek's diluent.
In recent years, many studies have been undertaken to understand and define the beneficial role of gut microbiota in the competitive exclusion of pathogens and how this is influenced by variations in composition, host environment, and treatments. In chickens, differences in the gut microbiota of broilers and layers (6) indicate the role of genotype and diet. In layer chickens, gut microbiota varies with age and gut segment, where there are more taxonomical complex microbiota present in the caecum compared with the ileum (7). Days 1 to 3 of chicken age are critical for microbiota development, whereas at around day 7, most of the taxa found in mature birds are already present; however, fluctuations in the abundance of microbial community members continue for several weeks (8).
Gut microbiota can be influenced positively or negatively, depending upon the triggering factor and its associated level. In chickens, among different segments of the gut, caeca contain the highest diversity and most dense populations of microbiota (9). Generally, stress conditions, infectious agents, and the use of antibiotics can alter the gut microbiota. For example, the use of dietary antibiotics reduced alpha diversity (richness), beta diversity, and abundance of the caecal microbiota in broilers (10). In laying hens, heat stress has been shown to reduce Firmicutes and increase Bacteroidetes in the feces, leading to the perturbation of the gut microbiota and its functions (11). Infectious agents can have profound negative effects on chicken gut microbiota, as seen in Marek's disease (12), infectious bronchitis (13), campylobacteriosis (14), salmonellosis (15), necrotic enteritis (16), and avian influenza (17).
Unlike infectious pathogens, the vaccines used to protect against them have not been extensively tested for microbiota interactions for many poultry vaccines. A study in broiler chickens suggested that coccidiosis vaccination positively influenced the population of Lactobacillaceae, Enterobacteriaceae, Clostridiaceae, and Streptococcaceae in caeca at 21 days of age (18). An oral administration of a recombinant S. Typhimurium vaccine in 4-day-old White Leghorn chickens significantly altered the alpha diversity (richness) and beta diversity of the caecal microbiota analyzed at week 5 of chicken age (19).
Since Vaxsafe ST is prepared by partial disruption of the aroA gene, the vaccine is unable to synthesize some aromatic compounds. Bacteria grown in nutritive broth may increase the expression of genes from Salmonella pathogenicity island-1 that facilitate attachment and invasion into intestinal epithelial cells. Multiple Salmonella serovars grown statically on agar and suspended in saline exhibit markedly lower invasion capacities than the same strains grown in nutrient-rich broth (20, 21). Therefore, we hypothesized that reconstituting Vaxsafe ST in nutritive diluent would enhance its colonization ability in the gut of chickens. Marek's diluent was selected as it is used in the poultry industry for Vaxsafe ST reconstitution for intramuscular injection at week 12 and is also used for reconstituting Marek's disease vaccine. Buffered peptone water (BPW) diluent was also used, as its composition is very similar to Marek's diluent. To compare the nutritive diluents, sterile water was used as a control. Additionally, multiple doses of Vaxsafe ST were used to evaluate whether a higher dose would lead to a significant increase in vaccine colonization in the gut. The main objectives of the current study were to understand the effects of vaccine diluent and vaccine (Vaxsafe ST) dosage on vaccine colonization in the caeca and the host caecal microbiota in commercial layer chickens vaccinated at day old, a time when the gut microbiota is rapidly changing.
Materials and methods
Ethics statement
The Animal Ethics Committee at the University of Adelaide approved all work (approval number S-2017-080) in accordance with the guidelines specified in “Australian code for the care and use of animals for scientific purposes, 8th edition (2013)”. The study followed the ARRIVE guidelines required for in vivo experiments (22).
Hatching and rearing of layer chickens
Mixed-sex Isa-Brown layer chickens were hatched and reared (n = 192) at the School of Animal and Veterinary Sciences, Roseworthy Campus, The University of Adelaide, as per standard procedures detailed in the Isa-Brown Management Guide. Different treatment groups were reared in floor pens (55 cm wide × 125 cm long) in separate rooms in a small animal research facility. Birds were divided into 12 treatment groups, and at each sampling timepoint, a minimum of 6 chickens were humanely euthanized by cervical dislocation. The mesh in each pen was covered with chick paper (205 GSM). Salmonella-free status of the hatched chickens was confirmed through the culturing of meconium samples (n = 4) from the incubator (Maru 190 Deluxe Cabinet), as previously described (15). The details of the treatment groups are provided in Table 1.
Vaccine reconstitution and chicken vaccination
Vials of Vaxsafe ST (Bioproperties) were either reconstituted in 1 mL of Marek's diluent, buffered peptone water, or water. For Marek's diluent, the reconstituted vaccine vial (containing 1010 CFU/mL) was 10-fold serially diluted in the same diluent to achieve 109 and 108 CFU per ml. The process was repeated to prepare vaccine dilutions in BPW and water diluents. The vaccine manufacturer's recommended dose is 107 CFU per chicken. The higher vaccine doses (109 and 108 CFU/chick) were included in the study to understand if they enhanced vaccine colonization ability in the gut. On day 1 post-hatch, individual chicken in the respective treatment groups received 100 μl doses of the respective reconstituted vaccine orally. Each bird was orally vaccinated to ensure uniform administration of the vaccine. Unvaccinated chickens received 100 μl of the sterile diluents. Chickens were euthanized on days 7 and 14 for caecal microbiota analysis and vaccine load quantification in the caecal contents. Caecal contents were collected and frozen at−80°C until used for DNA extraction.
Total DNA extraction from caecal contents
Total DNA was extracted from 192 luminal caecal content samples using a modified protocol for the QIAamp FAST DNA Stool Mini Kit (Qiagen). Caecal contents were collected from birds at each sampling point. Briefly, approximately 200 mg of caecal contents per sample was weighed into a 1.5-ml safe-lock tube and into each sample 700 μl of InhibitEx Buffer was added. For maximum lysis of microbial cells, a mixture of glass beads (acid-washed ≤ 106 μm and 425–600 μm; Sigma Aldrich) was added to the samples and homogenized in a bullet blender (Next Advances) for 5 min at speed 10. The samples were processed for DNA extraction as previously described (23). The quantity (average 88 ng/μl) and purity (average 260/280 value of 1.90 and 260/230 value of 1.70) of each DNA sample were tested to ensure that they were suitable for sequencing and qPCR.
16s rRNA metagenome sequencing and data analysis
All the samples were PCR-amplified for 16S rRNA gene analysis. The V3-V4 regions were amplified with Q5 high-fidelity polymerase (New England Biolabs) using the 338F (5′-ACTCCTACGGGAGGCAGCAG-3′) and 806R (5′-GGACTACHVGGGTWTCTAAT-3′) primer pairs as previously described (24) and sequenced on an Illumina MiSeq instrument using a 2 × 300 bp paired-end kit. The sequence data were demultiplexed with the onboard Illumina software, and the analysis was performed in Quantitative Insights into Microbial Ecology 2 (QIIME2) (25). Quality filtering, denoising, and chimera removal were performed using Dada2 (26) as a QIIME2 plugin with all recommended parameters, and the sequences were grouped into amplicon sequence variants (ASVs). Taxonomy was assigned using the SILVA v138.1 database (27). The ASV frequency table was loaded into the online MicrobiomeAnalyst analysis system for data normalization using the cumulative sum scaling (CSS) option, and the data were analyzed using alpha diversity, beta diversity, linear discriminant analysis effect size (LEfSe), and non-parametric tests, as per the recommendations of the online software (28).
Vaccine load determination in cecal contents
Salmonella Typhimurium vaccine-specific qPCR
Vaxsafe ST-specific qPCR was optimized with the primer pair (F: 5-GGTGTAATTGATCCCCAACG-3 and R: 5-GGTGTAATTGATCCCCAACG-3) designed by the vaccine manufacturing company (Bioproperties, Pty Ltd, Ringwood, Victoria, Australia) that targeted the aroA gene and produced a 204-bp product. The PCR was tested for specificity and amplification efficiency using 10-fold serially diluted Vaxsafe ST DNA. The primer pair was also tested against wild-type S. Typhimurium PT9 and Escherichia coli (chicken isolate) DNA to further test its specificity. The qPCR was performed using the SensiFAST SYBR Hi-ROX Kit (Bioline) in a 20-μl final reaction volume. The reaction volume contained 10 μl SensiFAST buffer, 1 μl each of the forward and reverse primers (10 μM), 2 μl of DNA template, and 6 μl of water. The cycling conditions in a QuantStudio 6 instrument were initial denature at 95°C for 3 min; 40 cycles of annealing at 60°C for 30 s; extension at 72°C for 30 s; a hold stage at 72°C for 5 min; and melting from 60°C to 95°C. The specificity of the primer pair was confirmed by the presence of a single peak in the melt curve analysis and electrophoretic analysis in a 2% agarose gel. The amplification efficiency (%) was calculated using E = -1+10(−1/slope).
Vaxsafe ST DNA fragment cloning and generation of a standard curve
A freshly generated qPCR product (204-bp amplicon length) of Vaxsafe ST DNA was cloned into a plasmid (pCR4-TOPO) that was inserted into DH5α-T1R chemically competent E. coli cells as per the manufacturer's protocol for One Shot Chemical Transformation, TOPO TA Cloning Kit for Sequencing (Invitrogen). The recombinant plasmid was extracted using a PureLink Quick Plasmid Miniprep Kit as per the manufacturer's protocol (Invitrogen). The insertion of the Vaxsafe ST fragment into the plasmid was confirmed by qPCR, melt curve analysis, and running the amplicon on a 2% agarose gel. Vaxsafe ST DNA was used as a positive control. The recombinant plasmid was serially diluted to construct a standard curve for the quantification of vaccine load from caecal contents. The DNA copy number for the recombinant plasmid was calculated from the plasmid DNA concentration and the molecular weight of the plasmid with a Vaxsafe ST fragment insert.
Vaxsafe ST load in caeca
To quantify the vaccine load from caecal contents, qPCR was performed, in duplicate, on the caecal content DNA of all the samples (n = 192). The optimized qPCR was highly sensitive for the quantification of Vaxsafe ST from chicken gut. A 10-fold dilution series of the plasmid was used to construct a standard curve. Vaxsafe ST DNA was included as a positive control, and no-template controls were also used. Samples falling out of the range of the standard curve (Cq > 35) were excluded from the Vaxsafe ST copy number determination.
Statistical analysis
The S. Typhimurium vaccine load (log10 DNA copy number) per gram of caecal contents data were analyzed in GraphPad Prism using non-parametric (Mann-Whitney) analysis. The level of significance was determined by PLSD at P < 0.05.
Results
Vaccine load in the caeca of chickens
The vaccine load from the caecal contents was quantified using qPCR. The optimized qPCR was highly target-specific, with a primer amplification efficiency of 97%. All unvaccinated groups were negative for the vaccine strain. Within each diluent treatment group, there was no significant effect of the vaccine dose on the load of the vaccine in the caecal contents (Figure 1A). The average vaccine load in the caecal contents on day 7 post-vaccination was Log10 8.94, while on day 14, it was Log10 8.37 (Figure 1B).
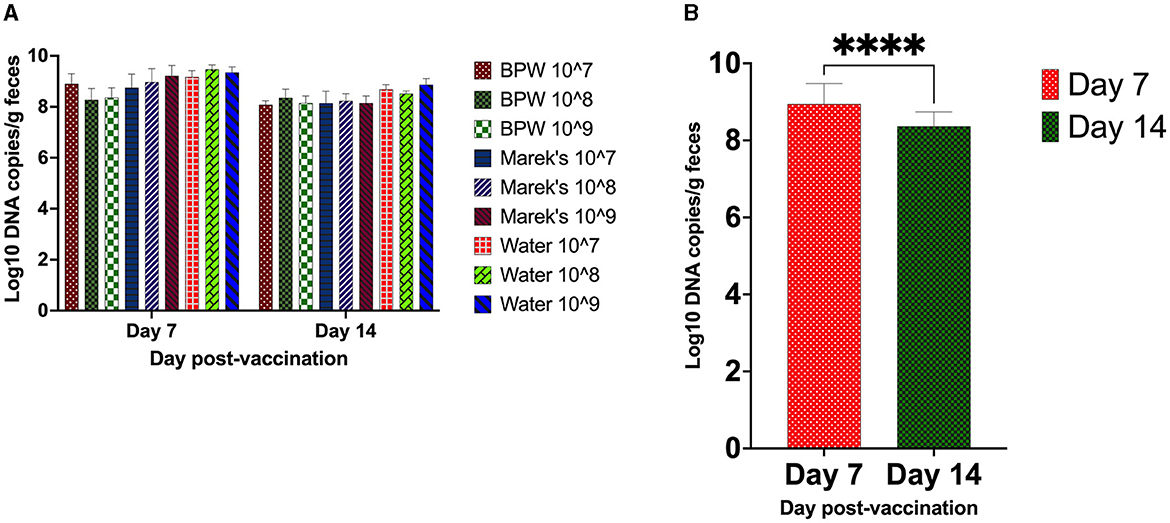
Figure 1. S. Typhimurium vaccine (Vaxsafe ST) load in caecal contents of vaccinated chickens. Day-old Isa-Brown layer chickens were vaccinated by reconstituting vaccine in BPW, Marek's diluent, or water and administered at 107, 108, or 109 CFU/chicken. The vaccine load through qPCR was determined on days 7 and 14 post-vaccination. (A) Vaccine load is affected by dose and diluent. (B) Vaccine load compared on day 7 and day 14 post-vaccination (P < 0.0001). DNA extracted from the caecal contents of the unvaccinated groups did not yield any PCR product; therefore, they were excluded from the statistical data analysis. DNA copy number data are log10 expressed. In (B), all treatment groups were combined to understand the chicken age's effect on vaccine load. Six chickens from each treatment group were processed on day 7, while up to 11 chickens on day 14 were processed for sample collection. Asterisks (****) in (B) show a P < 0.0001.
The effects of vaccine dosage and diluent on caecal microbiota alpha and beta diversities
The 16S rRNA gene amplicon sequencing produced 3,646,251 sequences after quality trimming and chimera removal, with an average of 23,224 reads per sample from 157 samples. Alpha diversity measures the community structure of individual samples in terms of how many taxa are present (richness) and the distribution of taxa abundances within a sample (evenness). Prior to administration at three different doses, Vaxsafe ST was reconstituted in buffered peptone water (BPW), Marek's diluent, and water to understand if the diluent and dose had any effects on gut microbiota (Figures 2A–D). There were no significant differences in the alpha diversity of the caecal microbiota of 14-day-old layer chickens vaccinated using different diluents (Figure 2A). However, the chickens vaccinated with 108 and 109 CFU/chicken showed significantly higher alpha diversity compared with the control unvaccinated groups (Figure 2B), and more detailed analysis revealed that this was completely driven by the unusually low alpha diversity of the water control group (Figure 2D). Compared to their respective controls, the three different vaccine doses (107, 108, and 109 CFU/chicken) prepared in BPW and water significantly affected (P < 0.05) the alpha diversity of the caecal microbiota (Supplementary Figures 1A, B). However, for Marek's diluent, the vaccine doses did not significantly affect the alpha diversity (Supplementary Figure 1C). Irrespective of the three diluents, the alpha diversity was significantly higher on day 14 compared with day 7 post-vaccination (Figure 2C). The overall significant effect of vaccine dose and diluent on the alpha diversity when the data were analyzed based on vaccine diluent and dosage was mainly due to the lower diversity in the water control group (Figure 2D).
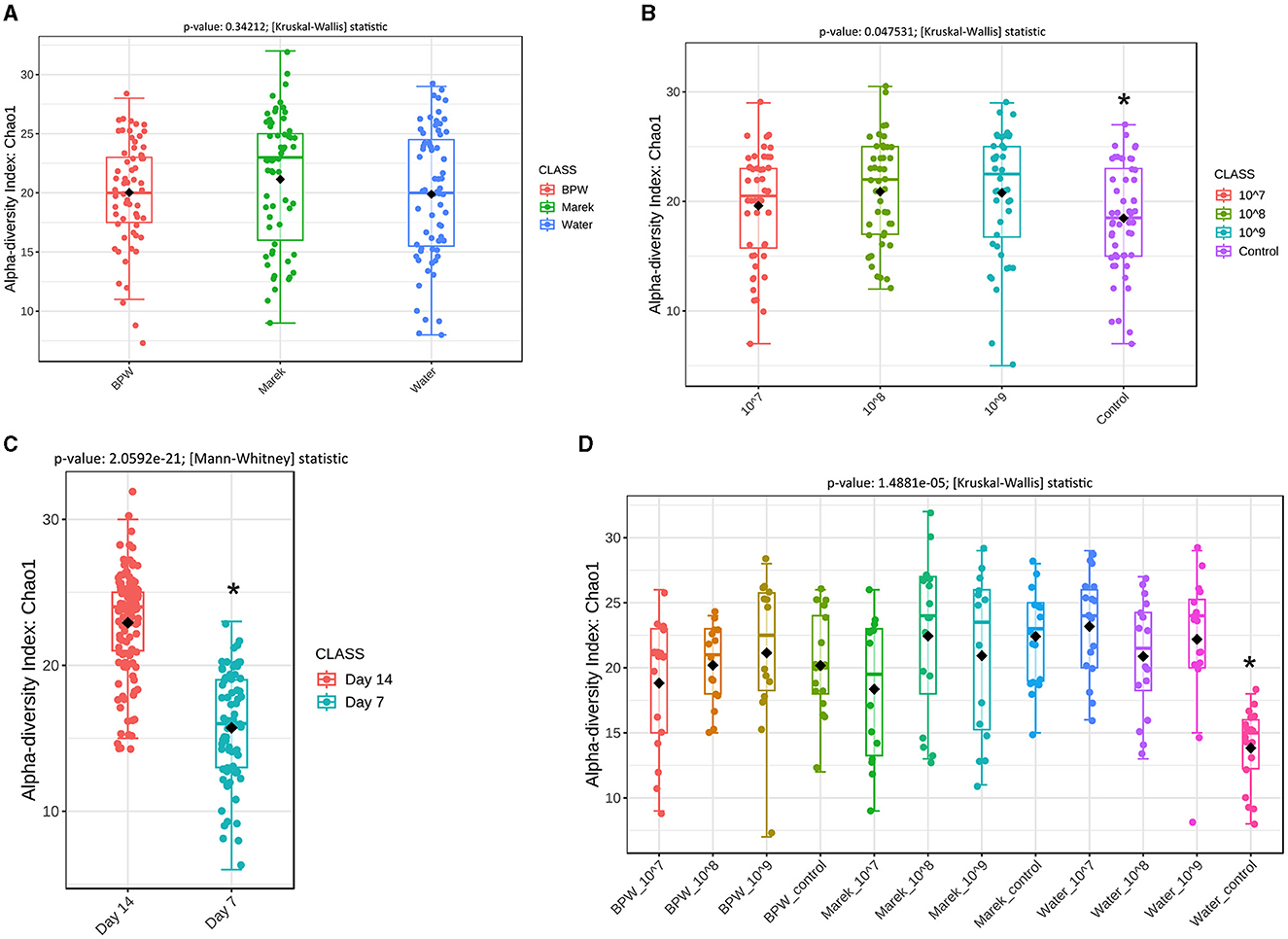
Figure 2. Overall alpha diversity of the caecal microbiota of layer chicks affected by diluent and Salmonella Typhimurium vaccine doses. (A) Alpha diversity is affected by vaccine diluent (P = 0.34212). (B) Alpha diversity is affected by vaccine dose (P = 0.047531). Data are presented by pooling relevant dose treatment groups together, and the control group represents controls of BPW, Marek's, and water diluents. (C) Alpha diversity is affected by age (P = 2.0592e-21). All the treatment groups were pooled together based on age. (D) Alpha diversity is affected by vaccine dose and diluent in individual treatment groups (P = 1.4881e-05). Alpha diversity was by Chao1 using the Mann-Whitney test, while beta diversity was measured by the distance method Bray-Curtis index and ANOSIM statistical method. Diversity was measured at the genus level. Chao1 is a nonparametric method that measures species richness, which refers to the total number of species in a sample. Within each panel graph, an asterisk (*) shows a significant difference. Each treatment group had a minimum of six chickens at each sampling timepoint.
Beta diversity measures the distance or dissimilarity of community structure between samples. In the assessment of the effect of individual diluents and the vaccine doses, despite significant dissimilarities between the treatment groups, the beta diversity of different treatment groups overlapped with each other (Figures 3A–D). Beta diversity of the caecal microbiota was also assessed in relation to three different vaccine doses prepared in three diluents, namely, BPW, Marek's, and water. Beta diversity of the control group (unvaccinated) clustered separately (P < 0.001), although overlapped, compared with the groups that received 107, 108, and 109 CFU/chick of vaccine (Figure 3B). Beta diversity was significantly affected by chicken age. Overall, beta diversity at day 7 post-vaccination was significantly different from chickens sampled at day 14 (Figure 3C). Within individual diluents (BPW, Marek's, and water), beta diversity was significantly dissimilar (P < 0.001), although heavily overlapped (Figure 3D). Unlike the significantly lower alpha diversity in the water control group, the beta diversity of the water control group was not significantly dissimilar from the water-vaccinated groups.
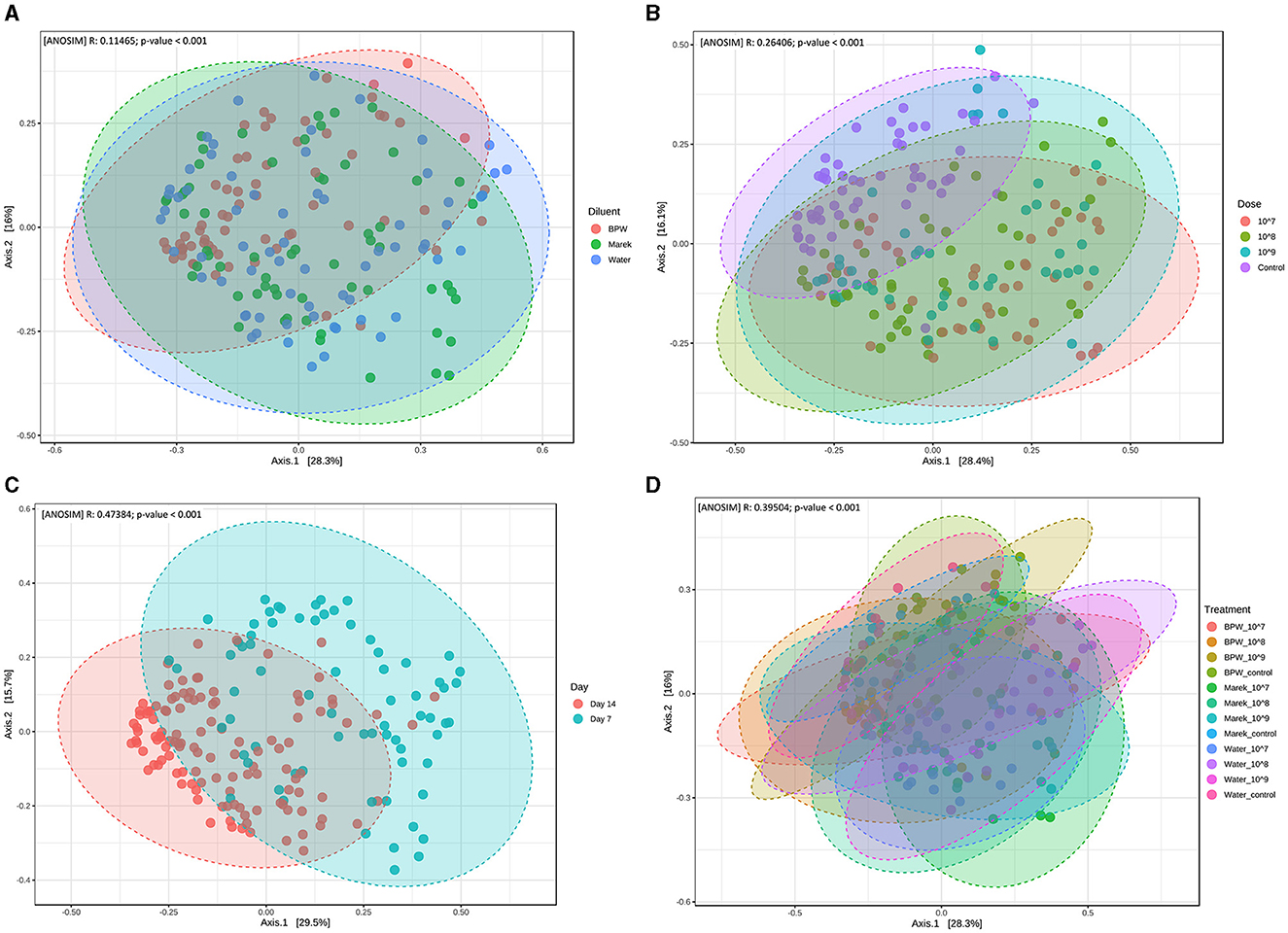
Figure 3. Beta diversity of the caecal microbiota of layer chickens influenced by vaccination. (A) Beta diversity is affected by vaccine diluent (P < 0.001). (B) Beta diversity is affected by vaccine dose (P < 0.001). Data are presented by pooling relevant dose treatment groups together, and the control group represents controls of BPW, Marek's, and water diluents. (C) Beta diversity is affected by age (P < 0.001). All the treatment groups were pooled together based on age. (D) Beta diversity is affected by vaccine dose and diluent in individual treatment groups (P < 0.001). Beta diversity was measured by the distance method with the Bray-Curtis index and the ANOSIM statistical method at the genus level. The abscissa (Axis 1) represents the first principal component, and the percentage represents the contribution of the first principal component to the sample difference; the ordinate (Axis 2) represents the second principal component, and the percentage represents the contribution of the second principal component to the sample difference. Each point in the Principal Coordinate Analysis (PCoA) plot represents a sample, and the distance between the points represents the dissimilarity of the caecal microbiota. Each treatment group had a minimum of six chickens at each sampling timepoint.
Vaccine dose and diluent had minimal, consistent effects on the caecal microbiota
The 16S rRNA sequencing data showed that Anaerostignum, Blautia, Caproiciproducens, Dickeya, Erysipelatoclostridium, Lactobacillus, Pediucoccus, and Sellimonas were significantly different in abundance across some vaccine doses and diluents (Figures 4A–H). The chickens inoculated with vaccine reconstituted in Marek's diluent did not display any significant alterations in the abundance of the most common genera. Only four low-abundance genera, including Incertae_Sedis, Flavonifractor, Christensenellaceae_R_7_group, and Dickeya, were significantly higher (FDR < 0.05) in abundance in the Marek's diluent control compared with the Marek's diluent-vaccinated groups. Interestingly, the Blautia abundance level was not consistent with treatments but was significantly lower in the water treatment groups (Figure 4B).
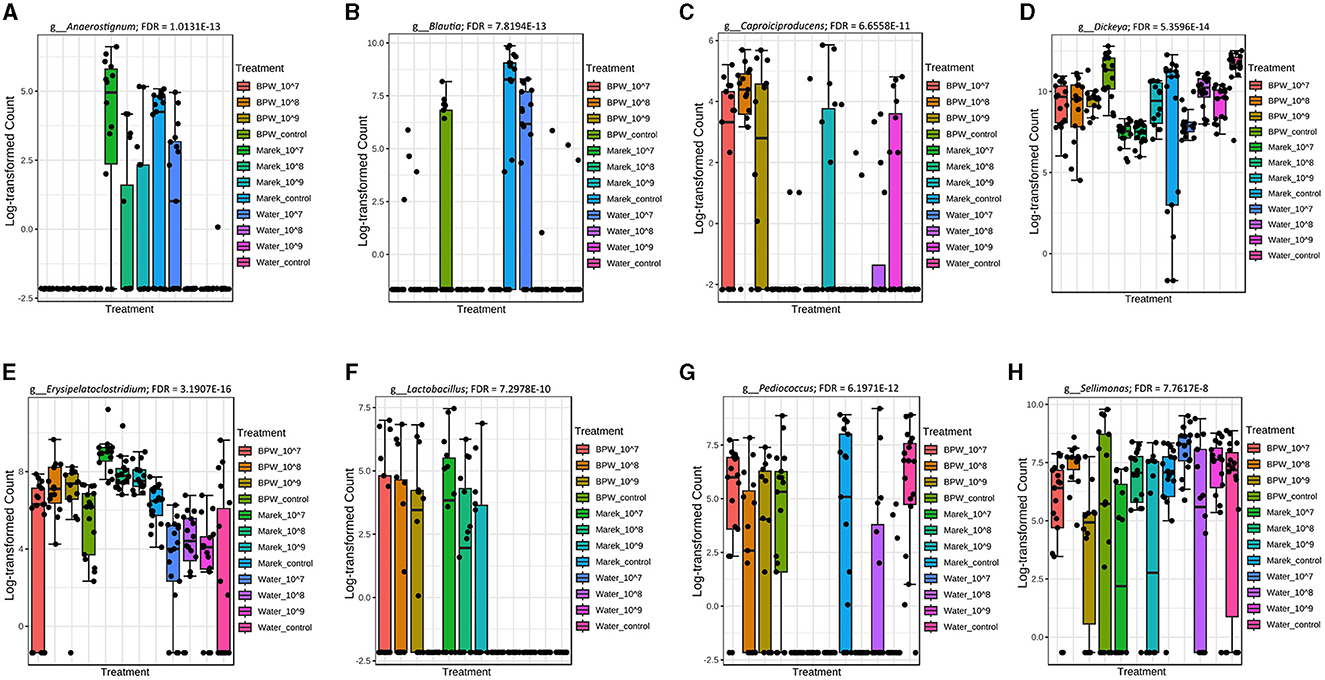
Figure 4. Genera that were significantly different in abundance in the various vaccine dose and diluent groups. Abundance levels of genera (A) Anaerostignum (FDR = 1.0131E-13); (B) Blautia (FDR = 7.8194E-13); (C) Capriociproducens (FDR = 6.6558E-11); (D) Dickeya (FDR = 5.3596E-14); (E) Erysipelatoclostridium (FDR = 3.1907E-16); (F) Lactobacillus (FDR = 7.2978E-10); (G) Pediococcus (FDR = 6.1971E-12); and (H) Sellimonas (FDR = 7.7617E-8). Abundance levels were compared using a non-parametric test in MicrobiomeAnalyst. Each treatment group had a minimum of six chickens at each sampling timepoint.
Chicken age had more profound effects on taxa abundance in the caeca than vaccine diluent or dose
Overall, there were 22 genera significantly different (FDR < 0.05) in abundance at day 7 compared with day 14 of chicken age. Among the 22 genera, the abundance levels of Anaerostignum, Blautia, Caproiciproducens, Sellimonas, Dickeya, Colidextribacter, Flavonifractor, Incertae_sedis, Lactobacillus, and Pediococcus were significantly higher at day 14 (Figures 5A–J), while Enterococcus and Paenibacillus were significantly higher at day 7 post-vaccination (Figures 5K, L).
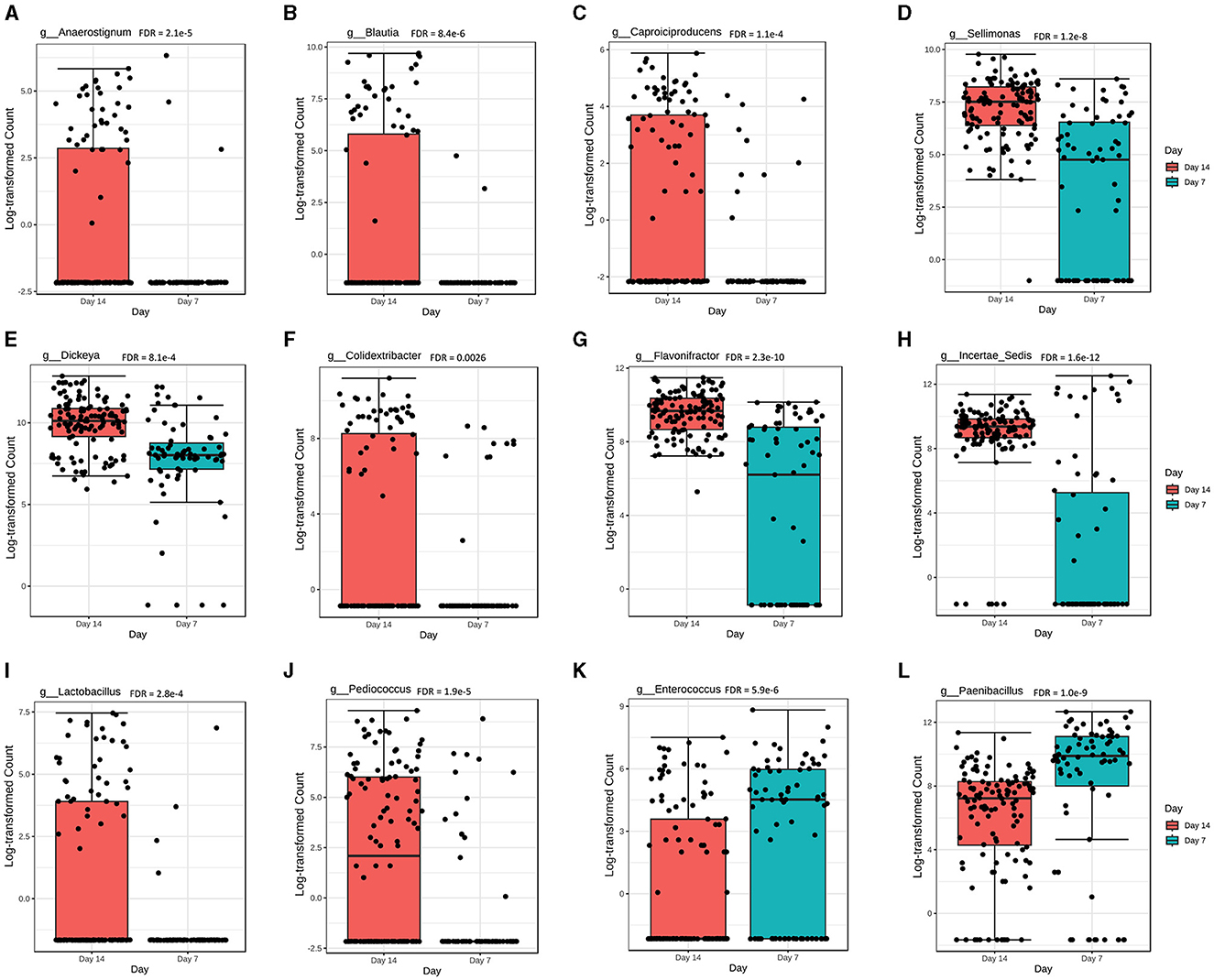
Figure 5. The overall abundance of caecal microbial communities is significantly affected by chicken age. Abundance levels for genera (A) Anaerostignum (FDR = 2.1e-5); (B) Blautia (FDR = 8.4e-6); (C) Caproiciproducens (FDR = 1.1e-4); (D) Sellimonas (FDR = 1.2e-8); (E) Dickeya (FDR = 8.1e-4); (F) Colidextribacter (FDR = 0.0026); (G) Flavonifractor (FDR = 2.3e-10); (H) Incertae_sedis (FDR = 1.6e-12); (I) Lactobacillus (FDR = 2.8e-4); (J) Pediococcus (FDR = 1.9e-5); (K) Enterococcus (FDR = 5.9e-6); and (L) Paenibacillus (FDR = 1.0e-9). Abundance level differences were assessed using a non-parametric test in MicrobiomeAnalyst. Each treatment group had a minimum of six chickens at each sampling timepoint.
To further understand the effects of vaccine dose and diluent, a linear discriminant analysis (LDA) effect size (LEfSe) analysis was performed on the ASV abundance data for the visualization of the top 10 most abundant genera within the caecal microbiota. LEfSe analysis was used to predict biomarkers associated with treatment groups. Erysipelatoclostridium was the only genera associated with Marek's diluent, while Dickeya, Flavonifractor, Colidextribacter, and Clostridium_sensu_stricto_1 were associated with BPW. Sellimonas, Ruminococcus_torques_group, Propionispora, and Christensenellaceae_R_7_group were associated with the water treatment group (Supplementary Figure 2A). Flavonifractor and UCG_005 were associated with treatment groups (all diluents combined) that received 109 CFU/chick, while Eubacterium_hallii_group was associated with 108 CFU/chick vaccine (Supplementary Figure 2B). As expected, chicken age had more profound effects, with six microbial genera associated with day 14 and four genera associated with day 7. The genera associated with day 14 samples included Dickeya, Flavonifractor, Christensenellaceae_R_7_group, Incertae_Sedis, Sellimonas, and Colidextribacter (Supplementary Figure 2C). There were not many microbial genera associated with individual vaccine doses prepared in specific diluent treatment groups (Supplementary Figure 2D).
Discussion
In this study, the S. Typhimurium vaccine (Vaxsafe ST) was studied to determine if it had any effects on caecal microbiota composition. The minimum dose of Vaxsafe ST recommended by the manufacturer is 107 CFU/chicken. Three different doses (107, 108, and 109 CFUs/chicken) were included to determine whether a higher dose would affect the colonization of the vaccine in the caeca. The S. Typhimurium strain in Vaxsafe ST is attenuated by disruption of the aroA gene, whose expression in a normal Salmonella cell is required in the shikimate pathway for the biosynthesis of aromatic amino acids. As an auxotroph, Vaxsafe ST has reduced competitiveness compared to wild-type ST.
The average vaccine load in the caecal contents quantified through qPCR showed that the vaccine was present until day 14 post-vaccination. This shows the successful colonization ability of Vaxsafe ST for stimulation of the gut immune system in layer chickens. The qPCR data also showed that the load of vaccine significantly decreased on day 14 compared with day 7 post-vaccination. A non-significant difference in the vaccine load between the treatment groups that received the three different doses of vaccine prepared in three different diluents shows that 107 CFU/chick of vaccine dose was sufficient to colonize the chicken caeca. The vaccine load data also showed that a vaccine reconstituted in water is as efficient as a vaccine reconstituted in nutritive diluents (e.g., BPW, Marek's) in gut colonization. A recent study involving AviPro Salmonella DUO's vaccine (a live attenuated vaccine consisting of S. Typhimurium and enteritidis strains) in commercial layer day-old chicks showed that the vaccine was not detected in cloacal swabs collected on day 2 post-vaccination (29). However, in a separate study, the vaccine strains of AviPro® Salmonella VacE and AviPro® Salmonella Duo were detected by culture of cloacal swabs collected 2 days post-vaccination of a day-old layer chicks (30). Vaxsafe ST could be quantified from the caeca of layer chicks at day 7 post-vaccination and could be detected in the spleen, liver, and jejunum following a culturing method (31). Perhaps differences in the detection of vaccines in various samples could be due to multiple factors, including the sensitivity of the tests applied. Overall, the qPCR data of Vaxsafe ST quantification from caecal contents show that the vaccine colonized the gut effectively and was quantifiable for at least 14 days post-vaccination, which was the experimental period of the current study.
The objective of reconstituting Vaxsafe ST in nutritive diluents (BPW and Marek's) was to determine whether these diluents could improve vaccine colonization in the chicken caeca. Water was used as a control, as it is the diluent used for administering Vaxsafe ST to chicks using a coarse spray. Additionally, it was aimed at establishing whether the dose and diluent could cause a significant shift in the structure of the caecal microbiota of layer chicks. The dosage of the vaccine (107, 108, and 109 CFU/chick) did not significantly change the composition of the gut microbiota. Statistically significant differences in caecal microbiota beta diversity were found between vaccinated and unvaccinated chickens, but the changes driving that difference were mainly among low-abundance taxa and were not shared across different diluent groups. The dose and diluent did not significantly change the colonization of the vaccine in the gut, suggesting that the recommended 107 CFUs/chick dose during vaccination is appropriate. However, in contrast, a recent study using a dose of 109 CFU/chicken of a different recombinant attenuated Salmonella vaccine (S. Typhimurium strain UK-1) has shown a major shift in the diversity of the caecal microbiota of the vaccinated chickens (19). A previously published study has shown that the AviPro Salmonella Duo (which contains S. enteritidis and S. Typhimurium serovars) vaccine in specific pathogen-free layer chickens (< 16 days old) did not significantly affect the alpha diversity of the caecal microbiota (32).
The analysis showed that overall the vaccine reconstituted in three different diluents did not change the alpha diversity of the caecal microbiota. Marek's vaccine diluent contains peptone and sucrose as the main ingredients, in addition to salts. Overall, a higher alpha diversity at day 14 compared with day 7 of the chicken's age showed that the population of caecal microbial communities in individual chickens became more diverse as the chickens aged. A higher alpha diversity of caecal microbiota at day 12 compared with days 5, 3, and 0 of layer chicken age has been previously reported (9, 33). Overall, the data showed that Vaxsafe ST administration minimally affected the alpha diversity of the caecal microbiota of layer chicks.
In the current study, a significantly dissimilar beta diversity at day 14 compared with day 7 post-vaccination showed that the caecal microbiota changed with chicken age. A shift in the beta diversity of the gut microbiota with chicken age has been previously reported (7). In the current study, an overlap in the beta diversity of different treatment groups showed that the Salmonella vaccination only minimally changed the community structure of the caecal microbiota. A previous study in 5-week-old layer chickens showed that a different Salmonella vaccine did change the composition of the gut microbiota in terms of beta diversity (19).
It is important to evaluate the impact of Vaxsafe ST on gut microbiota composition and understand that it is quite different from the effect of infection with wild-type Salmonella on chicken gut microbiota. Studies have shown that wild-type Salmonella infections negatively impact alpha and beta diversities of the gut microbiota, leading to a population surge of Enterobacteriaceae. For example, S. Typhimurium infection in 1-week-old layer chickens significantly changed the alpha and beta diversities of the caecal microbiota; decreased the abundance of Coprococcus, Ruminococcus, Lactococcus, and Lactobacillus; and increased unclassified Enterobacteriaceae (34). Salmonella enteritidis infection in a week-old layer of chickens significantly reduced overall diversity by increasing the abundance level of Enterobacteriaceae (35). A higher abundance of Lactobacillus in the BPW and Marek's diluents administered to vaccine groups shows the usefulness of nutrient-rich diluents used for vaccine reconstitution. The chicken age effect was obvious in the abundance of various microbial communities. Overall, Anaerostignum, Blautia, Caproiciproducens, Dickeya, Lactobacillus, Pediococcus, Sellimonas, and Colidextribacter significantly increased in abundance on day 14 compared with day 7 of chicken age. Among these communities, Lactobacillus has been shown, in a previous study, to be low on day 1 compared with days 7, 21, and 35 of broiler age (36). Blautia is involved in the production of short-chain fatty acids, primarily acetate (37), and plays a positive role in the modulation of gut functions (38). Lactobacillus has been widely implicated in the gut health of chickens (39, 40). In the future, vaccine manufacturers might use probiotics during vaccination to further improve the gut microbial environment as part of their gut health strategy. Studies in layer chickens have shown that both the Salmonella vaccine and probiotics are useful in improving gut health (19, 41).
Data obtained in this study demonstrate that Vaxsafe ST shows good levels of caecal colonization with a dose of 107 CFU/chick without disruption of the caecal microbiota. The vaccine can be used with confidence, knowing that there is less risk that it can cause dysbiosis.
Data availability statement
The original contributions presented in the study are publicly available. This data can be found here: https://www.ncbi.nlm.nih.gov/bioproject/; PRJNA1003953.
Ethics statement
The animal study was approved by the University of Adelaide Animal Ethics Committee. The study was conducted in accordance with the local legislation and institutional requirements.
Author contributions
SK: Data curation, Formal analysis, Investigation, Methodology, Validation, Visualization, Writing – original draft. AM: Conceptualization, Investigation, Methodology, Project administration, Resources, Writing – review & editing. DA: Conceptualization, Funding acquisition, Project administration, Writing – review & editing. GU: Conceptualization, Funding acquisition, Project administration, Supervision, Writing – review & editing. RM: Investigation, Project administration, Resources, Supervision, Writing – review & editing. TV: Investigation, Methodology, Resources, Writing – review & editing. RG: Investigation, Project administration, Supervision, Visualization, Writing – review & editing. KC: Conceptualization, Funding acquisition, Investigation, Methodology, Project administration, Resources, Supervision, Writing – review & editing.
Funding
The author(s) declare that financial support was received for the research, authorship, and/or publication of this article. This study was financially supported through the ARC-Linkage (grant number LP190100864), a co-funded project by the Australian Research Council and Bioproperties Pty Ltd, Australia. The Australian Research Council (ARC) had no role in the study design, data collection and analysis, decision to publish, or preparation of the manuscript.
Acknowledgments
The support received from Dr. Nicky-Lee Willson, Mr, Siyuan Jia, and Dr, Nitish Joat in sample collection is greatly acknowledged.
Conflict of interest
The authors declare that this study received funding from Bioproperties Pty Ltd. Bioproperties Pty Ltd. was involved in study design, interpretation of data and reviewing this article, and supplied the vaccine and Marek's diluent used in this research project. GU and DA are employees of Bioproperties Pty Ltd, a commercial veterinary vaccine business that manufactures and sells the Vaxsafe ST vaccine.
The remaining authors declare that the research was conducted in the absence of any commercial or financial relationships that could be construed as a potential conflict of interest.
The author(s) declared that they were an editorial board member of Frontiers, at the time of submission. This had no impact on the peer review process and the final decision.
Publisher's note
All claims expressed in this article are solely those of the authors and do not necessarily represent those of their affiliated organizations, or those of the publisher, the editors and the reviewers. Any product that may be evaluated in this article, or claim that may be made by its manufacturer, is not guaranteed or endorsed by the publisher.
Supplementary material
The Supplementary Material for this article can be found online at: https://www.frontiersin.org/articles/10.3389/fvets.2024.1364731/full#supplementary-material
References
1. European Food Safety Authority and European Centre for Disease Prevention and Control. The european union one health 2021 zoonoses report. EFSA J. (2022) 20:e07666. doi: 10.2903/j.efsa.2022.7666
2. Ford L, Moffatt CR, Fearnley E, Miller M, Gregory J, Sloan-Gardner TS, et al. The epidemiology of Salmonella enterica outbreaks in Australia, 2001–2016. Front Sustain Food Syst. (2018) 2:86. doi: 10.3389/fsufs.2018.00086
3. Crouch CF, Nell T, Reijnders M, Donkers T, Pugh C, Patel A, et al. Safety and efficacy of a novel inactivated trivalent Salmonella enterica vaccine in chickens. Vaccine. (2020) 38:6741–50. doi: 10.1016/j.vaccine.2020.08.033
4. Groves PJ, Sharpe SM, Muir WI, Pavic A, Cox JM. Live and inactivated vaccine regimens against caecal Salmonella Typhimurium colonisation in laying hens. Aust Vet J. (2016) 94:387–93. doi: 10.1111/avj.12490
5. Mcwhorter AR, Chousalkar KK. A long-term efficacy trial of a live, attenuated Salmonella Typhimurium vaccine in layer hens. Front Microbiol. (2018) 9:1380. doi: 10.3389/fmicb.2018.01380
6. Ocejo M, Oporto B, Hurtado A. 16S rRNA amplicon sequencing characterization of caecal microbiome composition of broilers and free-range slow-growing chickens throughout their productive lifespan. Sci Rep. (2019) 9:2506. doi: 10.1038/s41598-019-39323-x
7. Ngunjiri JM, Taylor KJ, Abundo MC, Jang H, Elaish M, Kc M, et al. Farm stage, bird age, and body site dominantly affect the quantity, taxonomic composition, and dynamics of respiratory and gut microbiota of commercial layer chickens. Appl Environ Microbiol. (2019) 85:e03137–e03118. doi: 10.1128/AEM.03137-18
8. Ballou AL, Ali RA, Mendoza MA, Ellis JC, Hassan HM, Croom WJ, et al. Development of the chick microbiome: how early exposure influences future microbial diversity. Front Vet Sci. (2016) 3:2. doi: 10.3389/fvets.2016.00002
9. Xiao SS, Mi JD, Mei L, Liang J, Feng KX, Wu YB, et al. Microbial diversity and community variation in the intestines of layer chickens. Animals. (2021) 11:840. doi: 10.3390/ani11030840
10. Kairmi SH, Taha-Abdelaziz K, Yitbarek A, Sargolzaei M, Spahany H, Astill J, et al. Effects of therapeutic levels of dietary antibiotics on the cecal microbiome composition of broiler chickens. Poult Sci. (2022) 101:101864. doi: 10.1016/j.psj.2022.101864
11. Zhu L, Liao R, Wu N, Zhu G, Yang C. Heat stress mediates changes in fecal microbiome and functional pathways of laying hens. Appl Microbiol Biotechnol. (2019) 103:461–72. doi: 10.1007/s00253-018-9465-8
12. Bavananthasivam J, Astill J, Matsuyama-Kato A, Taha-Abdelaziz K, Shojadoost B, Sharif S. Gut microbiota is associated with protection against Marek's disease virus infection in chickens. Virology. (2021) 553:122–30. doi: 10.1016/j.virol.2020.10.011
13. Xu P, Shi Y, Liu P, Yang Y, Zhou C, Li G, et al. 16S rRNA gene sequencing reveals an altered composition of the gut microbiota in chickens infected with a nephropathogenic infectious bronchitis virus. Sci Rep. (2020) 10:1–12. doi: 10.1038/s41598-020-60564-8
14. Hankel J, Jung K, Kuder H, Keller B, Keller C, Galvez E, et al. Caecal microbiota of experimentally Campylobacter jejuni-infected chickens at different ages. Front Microbiol. (2019) 10:2303. doi: 10.3389/fmicb.2019.02303
15. Khan S, Chousalkar KK. Salmonella Typhimurium infection disrupts but continuous feeding of Bacillus based probiotic restores gut microbiota in infected hens. J Anim Sci Biotechnol. (2020) 11:1–16. doi: 10.1186/s40104-020-0433-7
16. Lacey JA, Stanley D, Keyburn AL, Ford M, Chen H, Johanesen P, et al. Clostridium perfringens-mediated necrotic enteritis is not influenced by the pre-existing microbiota but is promoted by large changes in the post-challenge microbiota. Vet Microbiol. (2018) 227:119–26. doi: 10.1016/j.vetmic.2018.10.022
17. Chrzastek K, Leng J, Zakaria MK, Bialy D, La Ragione R, Shelton H. Low pathogenic avian influenza virus infection retards colon microbiota diversification in two different chicken lines. Animal Microbiome. (2021) 3:1–15. doi: 10.1186/s42523-021-00128-x
18. Das Q, Shay J, Gauthier M, Yin X, Hasted T-L, Ross K, et al. Effects of vaccination against coccidiosis on gut microbiota and immunity in broiler fed bacitracin and berry pomace. Front Immunol. (2021) 12:1874. doi: 10.3389/fimmu.2021.621803
19. Redweik GJ, Daniels K, Severin AJ, Lyte M, Mellata M. Oral treatments with probiotics and live Salmonella vaccine induce unique changes in gut neurochemicals and microbiome in chickens. Front Microbiol. (2020) 10:3064. doi: 10.3389/fmicb.2019.03064
20. Mcwhorter A, Chousalkar K. Comparative phenotypic and genotypic virulence of Salmonella strains isolated from Australian layer farms. Front Microbiol. (2015) 6:12. doi: 10.3389/fmicb.2015.00012
21. Mcwhorter AR, Davos D, Chousalkar K. Pathogenicity of Salmonella strains isolated from egg shells and the layer farm environment in Australia. Appl Environ Microbiol. (2015) 81:405–14. doi: 10.1128/AEM.02931-14
22. Percie Du Sert N, Hurst V, Ahluwalia A, Alam S, Avey MT, Baker M, et al. The ARRIVE guidelines 2.0: Updated guidelines for reporting animal research. J Cereb Blood Flow Metabol. (2020) 40:1769–77. doi: 10.1177/0271678X20943823
23. Khan S, Chousalkar KK. Functional enrichment of gut microbiome by early supplementation of Bacillus based probiotic in cage free hens: a field study. Animal Microbiome. (2021) 3:1–18. doi: 10.1186/s42523-021-00112-5
24. Joat N, Van TTH, Stanley D, Moore RJ, Chousalkar K. Temporal dynamics of gut microbiota in caged laying hens: a field observation from hatching to end of lay. Appl Microbiol Biotechnol. (2021) 105:4719–30. doi: 10.1007/s00253-021-11333-8
25. Bolyen E, Rideout JR, Dillon MR, Bokulich NA, Abnet CC, Al-Ghalith GA, et al. Reproducible, interactive, scalable and extensible microbiome data science using QIIME 2. Nat Biotechnol. (2019) 37:852–7. doi: 10.1038/s41587-019-0209-9
26. Callahan BJ, Mcmurdie PJ, Rosen MJ, Han AW, Johnson AJA, Holmes SP. DADA2: High-resolution sample inference from Illumina amplicon data. Nat Methods. (2016) 13:581–3. doi: 10.1038/nmeth.3869
27. Quast C, Pruesse E, Yilmaz P, Gerken J, Schweer T, Yarza P, et al. The SILVA ribosomal RNA gene database project: improved data processing and web-based tools. Nucleic Acids Res. (2012) 41:D590–6. doi: 10.1093/nar/gks1219
28. Chong J, Liu P, Zhou G, Xia J. Using MicrobiomeAnalyst for comprehensive statistical, functional, and meta-analysis of microbiome data. Nat Protoc. (2020) 15:799–821. doi: 10.1038/s41596-019-0264-1
29. Lin C-S, Lu T-L, Chen Y-A, Yu H-Y, Wu C-Y, Yang W-Y. Safety of bivalent live attenuated Salmonella vaccine and its protection against bacterial shedding and tissue invasion in layers challenged with Salmonella. Poult Sci. (2022) 101:101943. doi: 10.1016/j.psj.2022.101943
30. Eeckhaut V, Haesebrouck F, Ducatelle R, Van Immerseel F. Oral vaccination with a live Salmonella Enteritidis/Typhimurium bivalent vaccine in layers induces cross-protection against caecal and internal organ colonization by a Salmonella Infantis strain. Vet Microbiol. (2018) 218:7–12. doi: 10.1016/j.vetmic.2018.03.022
31. Jia S, Mcwhorter AR, Khan S, Andrews DM, Underwood GJ, Chousalkar KK. Investigation of a gel-based delivery method for the administration of a live, attenuated Salmonella Typhimurium vaccine. Vet Microbiol. (2023) 280:109721. doi: 10.1016/j.vetmic.2023.109721
32. Jan T-R, Lin C-S, Wang S-Y, Yang W-Y. Cytokines and cecal microbiome modulations conferred by a dual vaccine in Salmonella-infected layers. Poult Sci. (2023) 102:102373. doi: 10.1016/j.psj.2022.102373
33. Cui Y, Wang Q, Liu S, Sun R, Zhou Y, Li Y. Age-related variations in intestinal microflora of free-range and caged hens. Front Microbiol. (2017) 8:1310. doi: 10.3389/fmicb.2017.01310
34. Khan S, Chousalkar KK. Short-term feeding of probiotics and synbiotics modulates caecal microbiota during Salmonella Typhimurium infection but does not reduce shedding and invasion in chickens. Appl Microbiol Biotechnol. (2020) 104:319–34. doi: 10.1007/s00253-019-10220-7
35. Mon KK, Saelao P, Halstead MM, Chanthavixay G, Chang H-C, Garas L, et al. Salmonella enterica serovars Enteritidis infection alters the indigenous microbiota diversity in young layer chicks. Front Vet Sci. (2015) 2:61. doi: 10.3389/fvets.2015.00061
36. Zhou Q, Lan F, Li X, Yan W, Sun C, Li J, et al. The spatial and temporal characterization of gut microbiota in broilers. Front Vet Sci. (2021) 8:712226. doi: 10.3389/fvets.2021.712226
37. Rey FE, Faith JJ, Bain J, Muehlbauer MJ, Stevens RD, Newgard CB, et al. Dissecting the in vivo metabolic potential of two human gut acetogens. J Biol Chem. (2010) 285:22082–90. doi: 10.1074/jbc.M110.117713
38. Liu X, Guo W, Cui S, Tang X, Zhao J, Zhang H, et al. A comprehensive assessment of the safety of Blautia producta DSM 2950. Microorganisms. (2021) 9:908. doi: 10.3390/microorganisms9050908
39. Meimandipour A, Shuhaimi M, Soleimani A, Azhar K, Hair-Bejo M, Kabeir B, et al. Selected microbial groups and short-chain fatty acids profile in a simulated chicken cecum supplemented with two strains of Lactobacillus. Poult Sci. (2010) 89:470–6. doi: 10.3382/ps.2009-00495
40. Forte C, Manuali E, Abbate Y, Papa P, Vieceli L, Tentellini M, et al. Dietary Lactobacillus acidophilus positively influences growth performance, gut morphology, and gut microbiology in rurally reared chickens. Poult Sci. (2018) 97:930–6. doi: 10.3382/ps/pex396
41. Groves PJ, Williamson SL, Ahaduzzaman M, Diamond M, Ngo M, Han A, et al. Can a combination of vaccination, probiotic and organic acid treatment in layer hens protect against early life exposure to Salmonella Typhimurium and challenge at sexual maturity? Vaccine. (2021) 39:815–24. doi: 10.1016/j.vaccine.2020.12.044
Keywords: Salmonella Typhimurium vaccine, layer chicks, poultry production, salmonellosis, gut microbiota
Citation: Khan S, McWhorter AR, Andrews DM, Underwood GJ, Moore RJ, Van TTH, Gast RK and Chousalkar KK (2024) A live attenuated Salmonella Typhimurium vaccine dose and diluent have minimal effects on the caecal microbiota of layer chickens. Front. Vet. Sci. 11:1364731. doi: 10.3389/fvets.2024.1364731
Received: 03 January 2024; Accepted: 06 March 2024;
Published: 15 April 2024.
Edited by:
Wageha Awad, University of Veterinary Medicine Vienna, AustriaReviewed by:
Daniel Hernandez-Patlan, National Autonomous University of Mexico, MexicoDanielle Mahaffey Graham, University of Arkansas, United States
Copyright © 2024 Khan, McWhorter, Andrews, Underwood, Moore, Van, Gast and Chousalkar. This is an open-access article distributed under the terms of the Creative Commons Attribution License (CC BY). The use, distribution or reproduction in other forums is permitted, provided the original author(s) and the copyright owner(s) are credited and that the original publication in this journal is cited, in accordance with accepted academic practice. No use, distribution or reproduction is permitted which does not comply with these terms.
*Correspondence: Kapil K. Chousalkar, a2FwaWwuY2hvdXNhbGthckBhZGVsYWlkZS5lZHUuYXU=