- 1Hebei Key Laboratory of Preventive Veterinary Medicine, Hebei Normal University of Science and Technology, Qinhuangdao, China
- 2College of Veterinary Medicine, Hebei Agricultural University, Baoding, China
Bacterial pneumonia is a leading cause of mortality in fur-bearing animals, posing significant threat to fur production. To clarify the pathogenic agent of bacterial pneumonia in farmed foxes from eastern Hebei province, China, we performed bacterial isolation and identification from samples between 2020 and 2023. A total of 142 bacterial strains were isolated, of which 101 were identified as Escherichia coli (E. coli), indicating that E. coli is the major cause responsible for bacterial pneumonia in farmed foxes. Serotyping identification showed that a total of 8 serotypes were prevalent in the E. coli isolates, with O1, O8, O78 and O12 being the dominant ones. Five E. coli isolates were randomly picked for pathogenicity testing, and all of them were able to cause pneumonia symptoms in 6-week-old Kunming mice, accompanied by organ damage in lung. Eleven virulence genes were demonstrated present among the E. coli isolates. Antibiotic susceptibility tests showed that 78 of 101 E. coli strains exhibited multi-drug resistance (MDR), with the highest resistance rates against tetracyclines, and some strains showed resistance to carbapenems. Notably, no single antibiotic was effective against all strains. Twenty antibiotic resistance genes (ARGs) were detected among the isolates. Multilocus sequence typing (MLST) revealed 11 sequence types (STs) among 19 E. coli isolates, with ST-101 predominating (4/19). These findings enhance our understanding of the epidemiology, resistance traits, and pathogenicity of fox-derived pathogenic E. coli in Hebei.
1 Introduction
Bacterial pneumonia is a prevalent and recurrent disease in fur-bearing animal farming, serving as the leading cause of mortality during the growth phase (1–3). This disease primarily affects young animals aged 4 to 6 months and is particularly endemic from August to October, resulting in significant mortality rates (4, 5). In minks, Pseudomonas aeruginosa (P. aeruginosa) has been reported as the primary pathogen responsible for hemorrhagic pneumonia (1, 4, 6, 7); as for other fur-bearing animals, the etiology is complex, with involvement of multiple pathogens, such as Escherichia coli (E. coli), Klebsiella pneumoniae (KP), and P. aeruginosa (7, 8).
E. coli can cause a variety of infections in humans and animals, mainly manifesting as gastrointestinal diseases (9–13). While in farmed foxes, raccoon dogs, and minks, E. coli is associated with diverse severe health issues, including acute hemorrhagic pneumonia and systemic infections (3, 7, 8). Currently, there are commercial vaccines available for P. aeruginosa-induced pneumonia in minks, but due to the complex serotype diversity of E. coli, no commercial vaccine is available (14). Consequently, antibiotics remain the primary method for controlling pulmonary infections; however, the increasing prevalence of antibiotic resistance among bacterial strains presents significant challenges for disease management (15–17).
The eastern region of Hebei province is one of the three main breeding areas for fur animals in China, with fox and raccoon being the major breeding species. And the fur-bearing animals here, especially foxes, have been suffering from the severe threat of bacterial pneumonia in recent years. To elucidate the pathogenic characteristics of bacterial pneumonia in foxes within Hebei Province, this study focused on isolating and identifying pathogens from foxes with pneumonia between 2020 and 2023, and further investigating the antibiotic resistance and pathogenicity of the isolates to provide clinical guidance for the prevention and control of this disease.
2 Materials and methods
2.1 Sample collection and identification of bacteria
In this study, we collected 142 samples of fox pneumonia cases from 98 farms in eastern Hebei Province, China, between 2020 and 2023. The collected samples, including lungs and blood were transported to the laboratory within 24 h in ice packs for bacterial isolation and culture. First, the samples were inoculated onto a 5% defibrinated sheep blood agar medium (Qingdao Haibo Biotechnology Co., Ltd., Qingdao, China), followed by incubation at 37°C for 12 h in a constant temperature incubator. After purification, the isolates were subjected to Gram staining (Beijing Solarbio Science & Technology Co., Ltd., Beijing, China) and 16S rDNA sequence analysis.
2.2 Serotype identification
To expose the O antigen for detection, the bacterial suspension was autoclaved for 2 h to remove the K antigen on the bacterial surface. Then, sera specific to various E. coli O antigens (Beijing Zhonghai Biotech Co., Ltd., Beijing, China) were performed slide agglutination tests for the serotyping of isolated bacteria. A clear agglutination reaction (marked as ++) within 30 s was considered the standard for a positive result. The procedure was performed according to the manufacturer’s instructions.
2.3 Pathogenicity testing of Escherichia coli isolates in mice
Five E. coli isolates of different serotypes (The serotypes of the five strains were O1, O8, O78, O12, and O9, respectively) were tested for pathogenicity in 6-week-old Kunming mice, purchased from SPF (Beijing, China) Biotechnology Co., Ltd. Pathogenicity testing followed Zhang et al. (8). Sixty mice were randomly divided into 6 groups with 10 mice per group. The isolates were cultured to logarithmic growth phase and then diluted to a concentration of 1 × 107 CFU/mL with phosphate buffered saline (PBS). The Kunming mice were intraperitoneally challenged with 0.2 mL bacterial suspension or PBS as control, and the infected mice were continuously observed for 7 d. The clinical symptoms, pathologic variation, and mortality were recorded, and E. coli was reisolated and identified from the lungs of the infected mice. During the experiment, mice were euthanized to observe the pathological changes at 5-days postinfection (dpi), and one healthy mouse was randomly selected from the control group, followed by pathologic alterations being observed and compared. And the lung tissues of mice were taken and fixed in 3.5% neutral buffered formalin solution and further made into pathological sections for histopathological analysis.
2.4 Detection of virulence genes
DNA was extracted from the isolated strains by bacterial genome extraction kit (Beijing Solarbio Science & Technology Co., Ltd., Beijing, China). In total, 11 virulence genes previously reported were further identified by PCR, including vat, iutA, Iss, hlyF, iucD, tsh, cvaA/B, cvaC, trat, ECs3737, and ECs3703. The primer sequences and amplified fragment sizes are shown in Supplementary Table 1, and the primers were synthesized by Sangon Bioengineering (Shanghai, China) Co., Ltd.
2.5 Antibiotic sensitivity test
The antibiotic sensitivity of the E. coli isolates to 25 antibiotics was assayed by the Kirby-Bauer disk diffusion method according to the Clinical and Laboratory Standards Institute (CLSI) standards. The bacterial culture was diluted to 0.5 McFarland units with normal saline and spread on the Mueller-Hinton agar medium. Then the antibiotic disks (Hangzhou Microbial Reagent Co., Ltd., Hangzhou, China) were placed on the surface of the Mueller-Hinton agar medium and cultured in a constant temperature incubator at 37°C for 16–18 h. The diameter of the inhibition zone of each strain was measured, and based on the CLSI standards, the drug susceptibility test results were judged in three forms: sensitivity (S), intermediate (I), and resistance (R). E. coli ATCC 25922 was used as the quality control strain. Multi-drug resistance (MDR) strains were defined as those resistance to three or more antimicrobial classes (18).
2.6 Detection of antibiotic resistance genes
In this study, carriage of 25 antibiotic resistance genes (ARGs) in the E. coli isolates was further determined by PCR, including aminoglycoside resistance genes (strA, aadA2, aac2, aac4), fluoroquinolones resistance genes (gyrA, QnrS, qnrD, qnrC, qepA, qnrA, qnrB, gyrB), β-lactam resistance genes (CMY-2, CTX-M, SHV), amphenicol resistance gene (floR), macrolide resistance gene (mph(A)), tetracycline resistance genes (tet(A), tet(B), tet(E)), fosfomycin resistance gene (fosA), sulfonamides resistance genes (sul, sul2), and the Class 1 and Class 2 integrons (int1, int2). The primer sequences and amplified fragment sizes are shown in Supplementary Table 2, and some primers were designed according the literature previously reported (14) and synthesized by Sangon Bioengineering (Shanghai, China) Co., Ltd.
2.7 Multilocus sequence typing
To investigate the genetic diversity and epidemiological links of the isolates, 19 E. coli strains were randomly selected for multilocus sequence typing (MLST). The primer sequences for the seven housekeeping genes of E. coli (adk, fumC, icd, purA, gyrB, recA, and mdh) were obtained from http://enterobase.warwick.ac.uk/species/ecoli/allele_st_search (14, 19). The primer sequences, fragment sizes, and annealing temperatures are shown in Supplementary Table 3. And the sequencing work of the experiment was completed by Shanghai Hongxu Biotechnology (Shanghai, China) Co., Ltd. After sequencing was completed, cluster analysis was performed using PHYLOViZ software, and a Venn diagram was constructed following the methodology described in Peng et al. (20) to illustrate the shared and unique sequence types (STs) between human commensal E. coli and fox-derived E. coli.
3 Results
3.1 Strain source and identification
From 2020 to 2023, respiratory diseases occurred frequently in foxes at fur-bearing animal farms in the eastern region of Hebei Province, leading to mortality and economic losses. To identify the pathogens causing pneumonia, samples were collected from the affected foxes, and isolation and identification of the pathogens were conducted. A total of 142 dominant bacterial strains were isolated, and further identified by 16S rDNA sequencing. Of these strains, 101 were identified as E. coli, 15 as Pasteurella, 13 as KP, 8 as P. aeruginosa, 3 as Streptococcus, and 2 as Staphylococcus, suggesting that E. coli is the major, but not the only, etiologic agent responsible for bacterial pneumonia in farmed foxes. Figure 1A illustrates the geographical distribution of samples and E. coli isolates. Clinically, symptoms such as depression, lethargy, dyspnea, and diarrhea are exhibited by foxes infected with E. coli. Necropsy findings often include acute hemorrhagic pneumonia and systemic infection, with particularly evident lung lesions (Figures 1B–D).
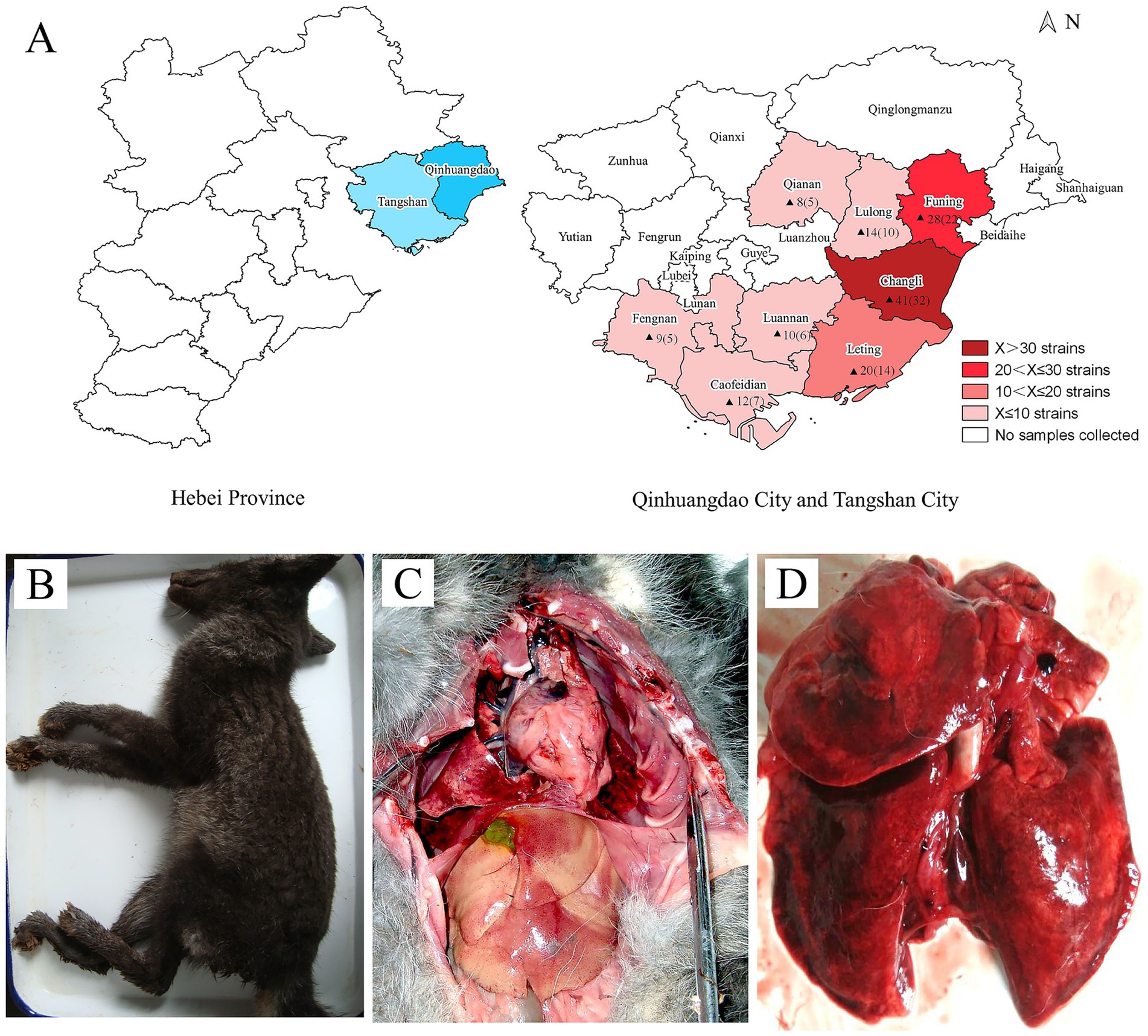
Figure 1. Geographical distribution of E. coli isolates and pathological observations of E. coli-infected fox. (A) Geographical distribution of samples and E. coli isolates. The sample-sourced region is marked in red, and the number of samples and E. coli isolates is labeled in the corresponding region, with the latter indicated in parentheses. (B) E. coli-infected fox. (C) Multi-organ pathological observations of fox. (D) Pathological observation of lungs in fox.
3.2 Serotype identification
To identify the serotypes of pathogenic E. coli, slide agglutination tests were performed on 101 isolated strains. The data showed that, a total of 98 isolates were successfully serotyped with 3 isolates untyped, 8 distinct serotypes were determined present in the E. coli isolates, of which O1 (26/101), O8 (19/101), O78 (17/101), and O12 (17/101), were predominant serotypes (Table 1).
3.3 The Escherichia coli isolates showed high pathogenicity to mice
To assess the pathogenicity of E. coli isolates, five strains were randomly selected for pathogenicity assessment. The data showed that, the mice received E. coli infection at a dose of 2 × 106 CFU exhibited apparent clinical symptoms such as ruffled fur, hunched posture, and anorexia. The mice began to suffer deaths at 3 dpi, with a cumulative mortality rate of 100% by day 7. The pathogenicity of the five strains showed no significant differences. In contrast, no abnormalities were observed in the control group. The isolate-like strains were re-isolated from the deceased mice.
These findings suggest that the 5 E. coli isolates are highly pathogenic to mice. Pathological observations revealed congestion and hemorrhage in the lungs of deceased mice, indicating significant pathological changes (Figures 2A,B).
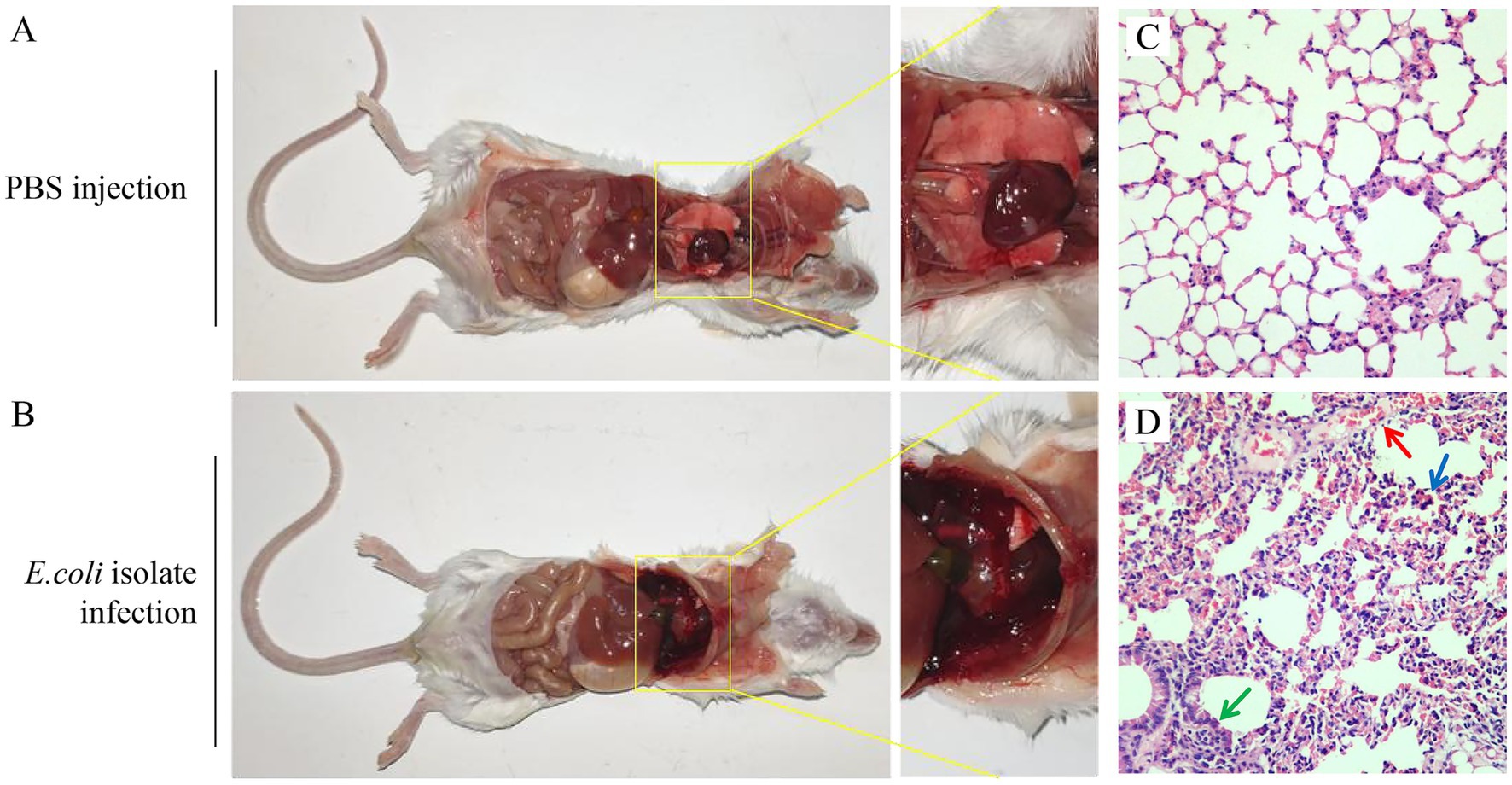
Figure 2. Pathological observations and histopathological analysis of infected mice. (A) Mouse in the control group. (B) Mouse in the experimental group. (C) Lung sample was collected from healthy mouse for pathological sectioning and hematoxylin–eosin staining. The histopathological changes were observed under a microscope (200×). (D) Lung sample was collected from E. coli-infected mouse for pathological sectioning and hematoxylin–eosin staining. The histopathological changes were observed under a microscope (200×).
Further histopathological analysis showed that, compared with the control group, the experimental group of mice revealed significant thickening of alveolar walls (green arrow) and diffuse erythrocyte infiltration in interstitial tissues (red arrow), with extensive inflammatory cell infiltration (blue arrow) (Figures 2C,D). Figure 2 shows the pathological results of mouse infection caused by the strain with serotype O1.
3.4 The Escherichia coli isolates carried multiple virulence genes
Considering the high pathogenicity of E. coli isolates, we detected the prevalence of 11 virulence genes using PCR. All targeted genes were found among the isolates, with the highest carrying rate of ECs3737 and ECs3703 genes at 63.4 and 58.4%, respectively, followed by the virulence genes trat, iutA, and iucD, with rates of 38.6, 28.7, and 27.7%, respectively (Table 2).
3.5 The Escherichia coli isolates displayed severe resistances to multiple antibiotics
To elucidate the antibiotic resistance patterns of fox-derived E. coli, antibiotic susceptibility testing was conducted on 101 E. coli isolates against 25 antibiotics. The results revealed a highly complex antibiotic resistance profile with significant variation among strains; 13 isolates showed the most severe drug resistance by resistance to 16 antibiotics, accounting for 12.9% (13/101). An additional table shows this in more detail (see Supplementary Table 4). The highest resistance rate was observed for tetracycline (98.0%), followed by sulfisoxazole (78.3%), ampicillin (72.3%), and sulfamethoxazole (72.3%). Notably, no single antibiotic was effective against all strains (Figure 3A). Statistical analysis revealed that 78 strains were resistant to three or more classes of antibiotics, exhibiting varying degrees of MDR, with an MDR rate of 77.3% (Figure 3B).
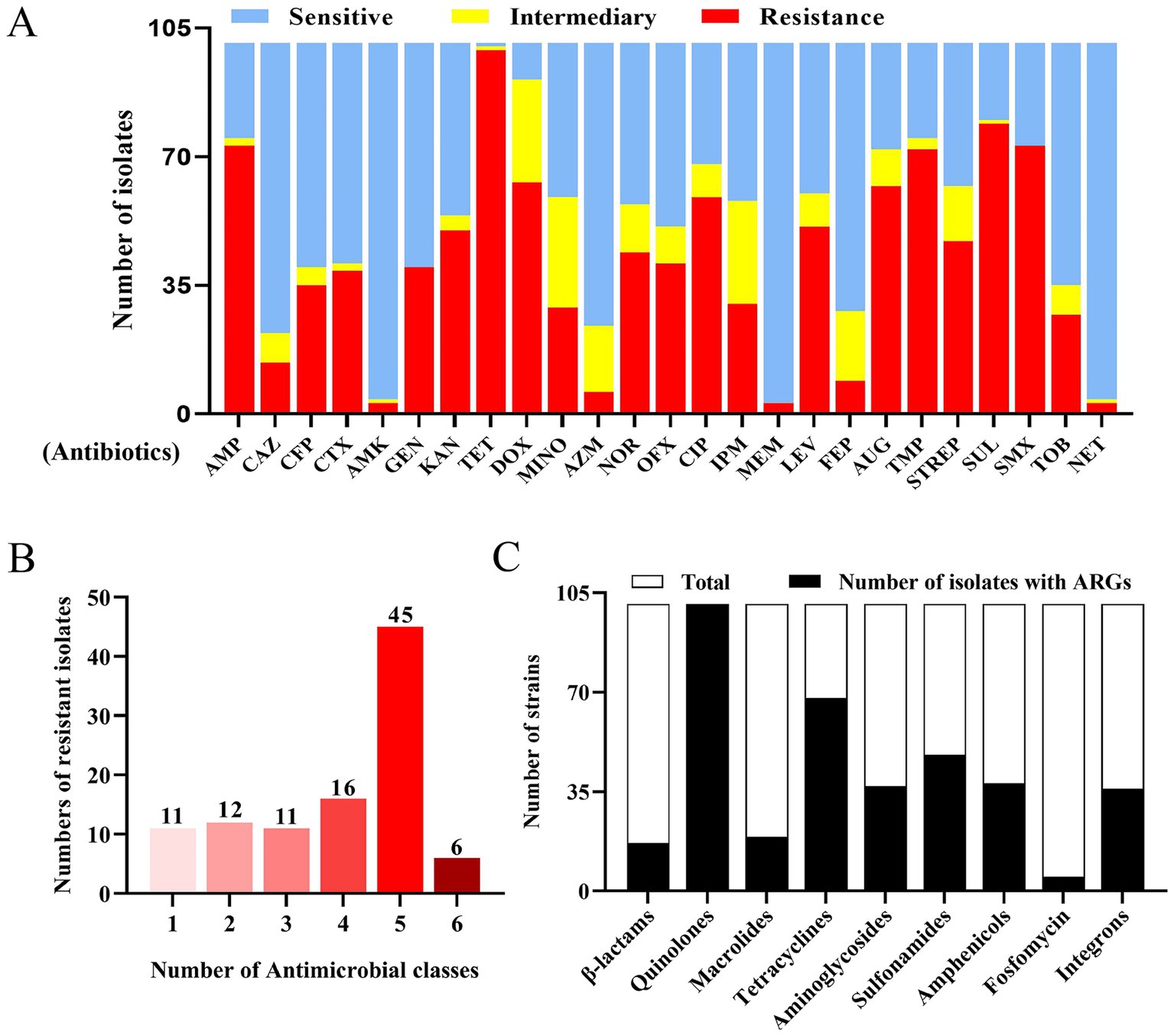
Figure 3. Drug resistance characteristics of 101 E. coli isolates. (A) Sensitivity, moderate sensitivity, and resistance ratios of E. coli isolates to 25 antibiotics. The ratios were calculated by dividing the number of isolates showing sensitivity, intermediate, and resistance to a certain antibiotic by the total number of 101. ampicillin (AMP); Ceftazidime (CAZ); Cefoperazone (CFP); Cefotaxime (CTX); Amikacin (AMK); Gentamicin (GEN); Kanamycin (KAN); Tetracycline (TET); Doxycycline (DOX); Minocycline (MINO); Azithromycin (AZM); Norfloxacin (NOR); Ofloxacin (OFX); Ciprofloxacin (CIP); Imipenem (IPM); Meropenem (MEM); Levofloxacin (LEV); Cefepime (FEP); Augmentin (AUG); Trimethoprim (TMP); Streptomycin (STREP); Sulfisoxazole (SUL); Sulfamethoxazole (SMX); Tobramycin (TOB); Netilmicin (NET). (B) Numbers of E. coli isolates resistant to different antibiotic classes tested. The antibiotic classes include β-Lactams, Aminoglycosides, Tetracyclines, Macrolides, Quinolones, and Sulfonamides. (C) Detection of ARGs in E. coli.
3.6 The Escherichia coli isolates carried multiple ARGs
The carriage and horizontal transfer of ARGs are the main reasons for bacteria developing antibiotic resistance. Here, 25 ARGs were determined by PCR, and a total of 20 ARGs were detected in 101 E. coli isolates (Table 3). It is noteworthy that all types of resistance genes were detected among the 101 E. coli strains. Specifically, 16.8% (17/101) harbored β-lactam resistance genes, while 100% (101/101) possessed fluoroquinolone resistance genes. Macrolide resistance genes were identified in 18.8% (19/101), tetracycline resistance genes in 67.3% (68/101), aminoglycoside resistance genes in 36.6% (37/101), amphenicol resistance genes in 37.6% (38/101), sulfonamide resistance genes in 47.5% (48/101), fosfomycin resistance genes in 5% (5/101), and integron genes in 35.6% (36/101) of the strains (Figure 3C).
3.7 The fox-derived Escherichia coli isolates displayed partial same MLSTs with that of human originated isolates
Following MLST analysis of 19 E. coli strains and subsequent cluster analysis using PHYLOViZ software, 11 distinct STs were identified. ST101 was the most prevalent, representing 21.1% of the strains. ST410, ST127, ST38, ST224, and ST7203 each accounted for 10.5%, while ST744, ST3285, ST7584, ST23, and ST48 were less common, each detected in 5.3% of the strains (Figure 4A). Details of E. coli strains that underwent MLST are presented in Supplementary Table 5. To determine the genetic propensity of drug-resistant isolates to spread into the human sector, a Venn diagram was constructed to compare shared and unique STs between human commensal E. coli and fox-derived E. coli. The analysis identified 6 STs that were common to both sources: ST101, ST410, ST224, ST23, ST48, and ST744 (Figure 4B).
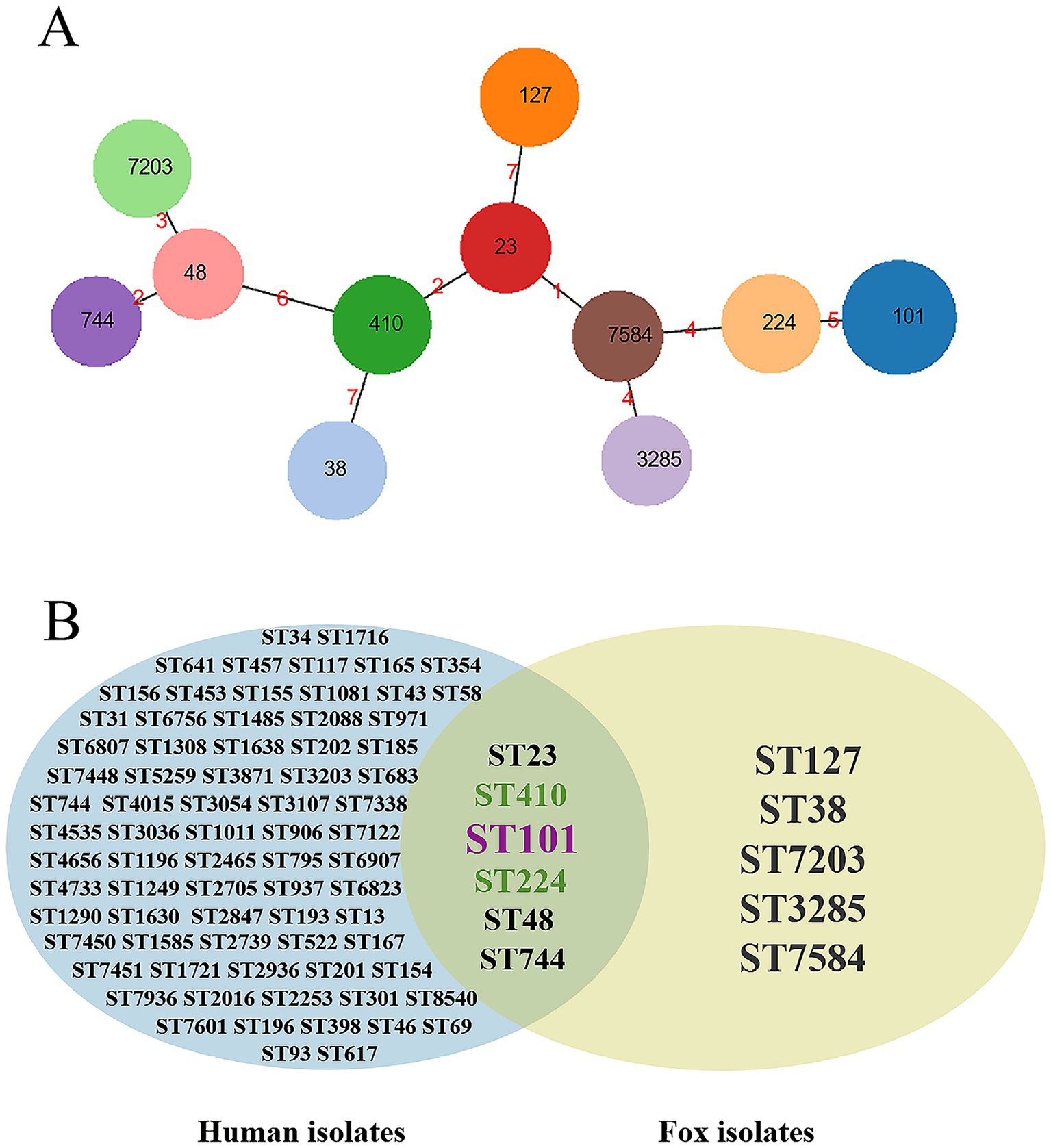
Figure 4. Cluster analysis and Venn diagram. (A) Cluster analysis was performed by PHYLOViZ software v1.1.5. The black number represents ST types; the red number represents the difference in allele numbers between different ST types. (B) Venn diagram showing shared and unique STs between human commensal E. coli and fox-derived E. coli.
4 Discussion
Hemorrhagic pneumonia is one of the leading causes of mortality in fur-bearing animals, commonly occurring in the autumn months and presenting with rapid onset and swift mortality, making it a significant challenge for fur-bearing animal production (21). Although P. aeruginosa has been determined as the primary pathogen responsible for hemorrhagic pneumonia (22), recent studies have reported the close relationship between E. coli and pneumonia outbreaks in farmed fur-bearing animals (3).
As a major province for fur-bearing animal breeding, the farming area is mainly focused at Qinhuangdao and Tangshan City, with fox and raccoon dogs as the most important species. From 2020 to 2023, outbreaks of hemorrhagic pneumonia were frequently reported in farmed foxes in the eastern region of Hebei Province. In the present study, we performed bacterial isolation and identification from pneumonia-affected foxes, and recovered a total of 142 bacterial strains. Among these isolates, 101 were identified as E. coli, while the remaining 41 strains were identified as Pasteurella, KP, P. aeruginosa, Streptococcus, and Staphylococcus. It is noteworthy that P. aeruginosa, the primary pathogen responsible for hemorrhagic pneumonia in mink, has also been detected in foxes, demonstrating its capacity to cause hemorrhagic pneumonia in this species. However, the low detection rate observed in this study suggests that P. aeruginosa is not the predominant pathogen underlying bacterial pneumonia in foxes. Pathogenicity tests demonstrated that the isolates could replicate pneumonia-like symptoms in a mouse model, indicating that E. coli is a predominant pathogen responsible for pneumonia in foxes in this region. E. coli is known to cause various types of infections, and there are numerous reports regarding its ability to induce severe pneumonia in humans as well as in animals such as horses, dogs, and cats (23–26). However, the literature about E. coli-induced infections in fur-bearing animals is limited. Similar to infections caused by P. aeruginosa, E. coli infections in foxes frequently lead to hemorrhagic pneumonia. In this study, the data of pathogenicity testing revealed that the isolates displayed high pathogenicity to mice, with severe hemorrhage and damage in the lungs.
The virulence factors contribute to the pathogenicity of E. coli infections (27, 28), and we examined the virulence factors of clinical isolates of E. coli and found that ECs3737 (63.4%), ECs3703 (58.4%), and trat (38.6%) had the highest detection rates. ECs3703 and ECs3737 genes are the marker genes for the virulence island of E. coli type III secretion system 2 (ETT2), suggesting that ETT2 plays a key role in E. coli causing bacterial pneumonia in foxes (29–31). The trat gene from the tra manipulator could confer bacteria the ability to escape from serum-killing (32), which is critical to bacterial septic infection, and the high detection rate of this gene may be an important explanation for E. coli’s susceptibility to cause septic infections in fur-bearing animals.
The development of a vaccine against E. coli remains under experimental stage due to complex serotypes. Here we serotyped E. coli isolates from Hebei, and identified four dominant serotypes. These serotypes partially overlapped with those reported in other regions and animal species (33–35), while maintaining distinct regional features. This finding provides guidance for vaccine development. Antibiotics remain the primary strategy for dealing with bacterial pneumonia in fur-bearing animals (3), but the increasing bacterial antibiotic resistance posed a considerable challenge to both the treatment of this disease and to human health (36). We examined the antibiotic susceptibility of the 101 E. coli isolates, and found that the isolates exhibited severe MDR, with the most severe resistance to tetracycline with a 98% resistance rate, and a high resistance rate to ampicillin and sulfonamides, which was consistent with a previous report (7). Notably, none of the 25 antibiotics tested proved to be sensitive to all the isolates, indicating the severe resistance of pathogenic E. coli in the Hebei region.
The bacterial antibiotic resistance in livestock production has become an increasing concern (37–39), and the isolates carrying plasmids targeting third-generation cephalosporins and carbapenem resistance genes from farm animals have been reported recently (40). In this study, some isolates were determined to exhibit resistance to the carbapenem antibiotics imipenem and meropenem, with a resistance rate of 29.7 and 3%, respectively, whereas both antibiotics are not approved to be used in farmed animals. The emergence of carbapenem-resistant E. coli from farmed animals would pose a significant threat to human health (41). The carriage and transmission of ARGs are the basis for the bacterial resistance (42, 43), and we examined the carriage of ARGs in 101 E. coli isolates and found that all the ARGs, including β-lactams, aminoglycosides, quinolones, amphenicol, macrolides, tetracyclines, sulfonamides, and fosfomycin resistance genes, as well as integrons, were determined prevalent. Among which, the detection rate of quinolone resistance genes was 100%, followed by tetracyclines (67.3%) and sulfonamides resistance genes (47.5%), which was consistent with the results of resistance phenotype detection. Integrons are considered to be a significant cause for the transmission and development of bacterial drug resistance (44–46), and in this study, a 35.6% carrier rate of class 1 integrons was determined among the E. coli isolates.
MLST analysis is a bacterial typing method based on housekeeping genes (47), and here we randomly picked 19 fox-derived E. coli isolates for MLST analysis, and identified a total of 11 STs. Among these, 6 STs were also the types found in human commensal E. coli, suggesting that fox-derived E. coli may pose a threat to human health.
In sum, we isolated and identified the causative pathogens from foxes suffering from pneumonia in the eastern region of Hebei Province from 2020 to 2023, and demonstrated that E. coli is the major etiological agent responsible for hemorrhagic pneumonia in foxes in this region. These E. coli isolates exhibited high pathogenicity and severe MDR, posing a potential threat to fox farming and human health.
Data availability statement
The original contributions presented in the study are included in the article/Supplementary material, further inquiries can be directed to the corresponding author.
Ethics statement
The animal study was approved by the study protocol was approved by the Animal Ethics Committee of Hebei Normal University of Science and Technology (Document number of approval: 20220101). The study was conducted in accordance with the local legislation and institutional requirements.
Author contributions
CZ: Data curation, Investigation, Writing – original draft. HL: Data curation, Investigation, Writing – review & editing. QZ: Data curation, Investigation, Writing – review & editing. LW: Data curation, Investigation, Writing – review & editing. GH: Data curation, Investigation, Writing – review & editing. QS: Supervision, Writing – review & editing. TW: Supervision, Writing – review & editing. GG: Supervision, Writing – review & editing. ZZ: Conceptualization, Methodology, Supervision, Writing – original draft, Writing – review & editing.
Funding
The author(s) declare that financial support was received for the research and/or publication of this article. This study was supported by the Natural Science Foundation of Hebei Province, grant no. C2024407005; Hebei High-Level Talent Funding Program, grant no. C20231014; Basic scientific research fund from Hebei Normal University of Science and Technology, grant number 2023JK14; National Key Research and Development Program, grant no. 2023YFD1800701-01.
Acknowledgments
We express our gratitude to all the members of the key laboratory of preventive veterinary medicine for their valuable suggestions and exceptional technical support.
Conflict of interest
The authors declare that the research was conducted in the absence of any commercial or financial relationships that could be construed as a potential conflict of interest.
Publisher’s note
All claims expressed in this article are solely those of the authors and do not necessarily represent those of their affiliated organizations, or those of the publisher, the editors and the reviewers. Any product that may be evaluated in this article, or claim that may be made by its manufacturer, is not guaranteed or endorsed by the publisher.
Supplementary material
The Supplementary material for this article can be found online at: https://www.frontiersin.org/articles/10.3389/fvets.2025.1567009/full#supplementary-material
References
1. Gu, J, Li, X, Yang, M, Du, C, Cui, Z, Gong, P, et al. Therapeutic effect of Pseudomonas aeruginosa phage YH30 on mink hemorrhagic pneumonia. Vet Microbiol. (2016) 190:5–11. doi: 10.1016/j.vetmic.2016.03.016
2. Sun, N, Yang, Y, Wang, G, Guo, L, Liu, L, San, Z, et al. Whole-genome sequencing of multidrug-resistant Klebsiella pneumoniae with capsular serotype K2 isolates from mink in China. BMC Vet Res. (2024) 20:356. doi: 10.1186/s12917-024-04222-5
3. Yu, Y, Hu, B, Fan, H, Zhang, H, Lian, S, Li, H, et al. Molecular epidemiology of Extraintestinal pathogenic Escherichia coli causing hemorrhagic pneumonia in mink in northern China. Front Cell Infect Microbiol. (2021) 11:781068. doi: 10.3389/fcimb.2021.781068
4. Cui, J, Shi, X, Wang, X, Sun, H, Yan, Y, Zhao, F, et al. Characterization of a lytic Pseudomonas aeruginosa phage vB_PaeP_ASP23 and functional analysis of its lysin LysASP and holin HolASP. Front Microbiol. (2023) 14:1093668. doi: 10.3389/fmicb.2023.1093668
5. Zhao, Y, Guo, L, Li, J, Fang, B, and Huang, X. Molecular epidemiology, antimicrobial susceptibility, and pulsed-field gel electrophoresis genotyping of Pseudomonas aeruginosa isolates from mink. Can J Vet Res. (2018) 82:256–63.
6. Bai, J, Wang, X, Zhang, Z, Lian, P, and Qiao, J. Overview of mink immunity and resistance to Pseudomonas aeruginosa. Vet Med Int. (2023) 2023:6158844. doi: 10.1155/2023/6158844
7. Nikolaisen, NK, Lassen, DCK, Chriél, M, Larsen, G, Jensen, VF, and Pedersen, K. Antimicrobial resistance among pathogenic bacteria from mink (Neovison vison) in Denmark. Acta Vet Scand. (2017) 59:60. doi: 10.1186/s13028-017-0328-6
8. Zhang, Z, Wu, T, Li, Y, Bai, X, Yan, X, Gao, Y, et al. Contribution of the serine protease HtrA in Escherichia coli to infection in foxes. Microb Pathog. (2019) 135:103570. doi: 10.1016/j.micpath.2019.103570
9. Geurtsen, J, de Been, M, Weerdenburg, E, Zomer, A, McNally, A, and Poolman, J. Genomics and pathotypes of the many faces of Escherichia coli. FEMS Microbiol Rev. (2022) 46:fuac031. doi: 10.1093/femsre/fuac031
10. He, L, Wang, C, Simujide, H, Aricha, H, Zhang, J, Liu, B, et al. Effect of early pathogenic Escherichia coli infection on the intestinal barrier and immune function in newborn calves. Front Cell Infect Microbiol. (2022) 12:818276. doi: 10.3389/fcimb.2022.818276
11. Marder, EP, Cui, Z, Bruce, BB, Richardson, LC, Boyle, MM, Cieslak, PR, et al. Risk factors for non-O157 Shiga toxin-producing Escherichia coli infections, United States. Emerg Infect Dis. (2023) 29:1183–90. doi: 10.3201/eid2906.221521
12. Perna, A, Hay, E, Contieri, M, De Luca, A, Guerra, G, and Lucariello, A. Adherent-invasive Escherichia coli (AIEC): cause or consequence of inflammation, dysbiosis, and rupture of cellular joints in patients with IBD? J Cell Physiol. (2020) 235:5041–9. doi: 10.1002/jcp.29430
13. Tontanahal, A, Sperandio, V, Kovbasnjuk, O, Loos, S, Kristoffersson, A-C, Karpman, D, et al. IgG binds Escherichia coli serine protease EspP and protects mice from E. coli O157:H7 infection. Front Immunol. (2022) 13:807959. doi: 10.3389/fimmu.2022.807959
14. Liang, C, Cui, H, Chen, L, Zhang, H, Zhang, C, and Liu, J. Identification, typing, and drug resistance analysis of Escherichia coli in two different types of broiler farms in Hebei Province. Animals (Basel). (2023) 13:3194. doi: 10.3390/ani13203194
15. Pulingam, T, Parumasivam, T, Gazzali, AM, Sulaiman, AM, Chee, JY, Lakshmanan, M, et al. Antimicrobial resistance: prevalence, economic burden, mechanisms of resistance and strategies to overcome. Eur J Pharm Sci. (2022) 170:106103. doi: 10.1016/j.ejps.2021.106103
16. Tang, B, Wang, J, Zheng, X, Chang, J, Ma, J, Wang, J, et al. Antimicrobial resistance surveillance of Escherichia coli from chickens in the Qinghai plateau of China. Front Microbiol. (2022) 13:885132. doi: 10.3389/fmicb.2022.885132
17. Zhou, W, Lin, R, Zhou, Z, Ma, J, Lin, H, Zheng, X, et al. Antimicrobial resistance and genomic characterization of Escherichia coli from pigs and chickens in Zhejiang, China. Front Microbiol. (2022) 13:1018682. doi: 10.3389/fmicb.2022.1018682
18. Zhou, L, Ye, Q, Zhou, Q, Wang, J, Li, G, Xiang, J, et al. Antimicrobial resistance and genomic investigation of Salmonella isolated from retail foods in Guizhou, China. Front Microbiol. (2024) 15:1345045. doi: 10.3389/fmicb.2024.1345045
19. Zhou, Z, Alikhan, N-F, Mohamed, K, Fan, Y, and Agama Study GroupAchtman, M. The EnteroBase user’s guide, with case studies on Salmonella transmissions, Yersinia pestis phylogeny, and Escherichia core genomic diversity. Genome Res. (2020) 30:138–52. doi: 10.1101/gr.251678.119
20. Peng, Z, Hu, Z, Li, Z, Zhang, X, Jia, C, Li, T, et al. Antimicrobial resistance and population genomics of multidrug-resistant Escherichia coli in pig farms in mainland China. Nat Commun. (2022) 13:1116. doi: 10.1038/s41467-022-28750-6
21. Qi, J, Li, L, Du, Y, Wang, S, Wang, J, Luo, Y, et al. The identification, typing, and antimicrobial susceptibility of Pseudomonas aeruginosa isolated from mink with hemorrhagic pneumonia. Vet Microbiol. (2014) 170:456–61. doi: 10.1016/j.vetmic.2014.02.025
22. Salomonsen, CM, Chriél, M, Jensen, TH, Rangstrup-Christensen, L, Høiby, N, and Hammer, AS. Effect of infectious dose and season on development of hemorrhagic pneumonia in mink caused by Pseudomonas aeruginosa. Can J Vet Res. (2013) 77:221–5.
23. Almes, KM, Janardhan, KS, Anderson, J, Hesse, RA, and Patton, KM. Fatal canine adenoviral pneumonia in two litters of bulldogs. J Vet Diagn Invest. (2010) 22:780–4. doi: 10.1177/104063871002200524
24. Brooks, JW, Roberts, EL, Kocher, K, Kariyawasam, S, and DebRoy, C. Fatal pneumonia caused by Extraintestinal pathogenic Escherichia coli (ExPEC) in a juvenile cat recovered from an animal hoarding incident. Vet Microbiol. (2013) 167:704–7. doi: 10.1016/j.vetmic.2013.08.015
25. DebRoy, C, Roberts, E, Jayarao, BM, and Brooks, JW. Bronchopneumonia associated with extraintestinal pathogenic Escherichia coli in a horse. J Vet Diagn Invest. (2008) 20:661–4. doi: 10.1177/104063870802000524
26. Kong, L-C, Guo, X, Wang, Z, Gao, Y-H, Jia, B-Y, Liu, S-M, et al. Whole genome sequencing of an ExPEC that caused fatal pneumonia at a pig farm in Changchun, China. BMC Vet Res. (2017) 13:169. doi: 10.1186/s12917-017-1093-5
27. Gao, J, Duan, X, Li, X, Cao, H, Wang, Y, and Zheng, SJ. Emerging of a highly pathogenic and multi-drug resistant strain of Escherichia coli causing an outbreak of colibacillosis in chickens. Infect Genet Evol J. (2018) 65:392–8. doi: 10.1016/j.meegid.2018.08.026
28. Gao, Q, Wang, X, Xu, H, Xu, Y, Ling, J, Zhang, D, et al. Roles of iron acquisition systems in virulence of extraintestinal pathogenic Escherichia coli: salmochelin and aerobactin contribute more to virulence than heme in a chicken infection model. BMC Microbiol. (2012) 12:143. doi: 10.1186/1471-2180-12-143
29. Cheng, D, Zhu, S, Su, Z, Zuo, W, and Lu, H. Prevalence and isoforms of the pathogenicity island ETT2 among Escherichia coli isolates from colibacillosis in pigs and mastitis in cows. Curr Microbiol. (2012) 64:43–9. doi: 10.1007/s00284-011-0032-0
30. Sheikh, J, Dudley, EG, Sui, B, Tamboura, B, Suleman, A, and Nataro, JP. EilA, a HilA-like regulator in enteroaggregative Escherichia coli. Mol Microbiol. (2006) 61:338–50. doi: 10.1111/j.1365-2958.2006.05234.x
31. Wang, S, Liu, X, Xu, X, Zhao, Y, Yang, D, Han, X, et al. Escherichia coli type III secretion system 2 (ETT2) is widely distributed in avian pathogenic Escherichia coli isolates from eastern China. Epidemiol Infect. (2016) 144:2824–30. doi: 10.1017/S0950268816000820
32. Pramoonjago, P, Kaneko, M, Kinoshita, T, Ohtsubo, E, Takeda, J, Hong, KS, et al. Role of TraT protein, an anticomplementary protein produced in Escherichia coli by R100 factor, in serum resistance. J Immunol. (1992) 148:827–36. doi: 10.4049/jimmunol.148.3.827
33. Jamali, H, Akrami, F, Bouakkaz, S, and Dozois, CM. Prevalence of specific serogroups, antibiotic resistance and virulence factors of avian pathogenic Escherichia coli (APEC) isolated from clinical cases: a systematic review and meta-analysis. Microb Pathog. (2024) 194:106843. doi: 10.1016/j.micpath.2024.106843
34. Runcharoon, K, Favro, ME, and Logue, CM. Longitudinal analysis of avian pathogenic Escherichia coli (APEC) serogroups and pathotypes from avian colibacillosis in Georgia: a continued investigation - year 2 analysis. Poult Sci. (2025) 104:104722. doi: 10.1016/j.psj.2024.104722
35. Torres, AG, Amaral, MM, Bentancor, L, Galli, L, Goldstein, J, Krüger, A, et al. Recent advances in Shiga toxin-producing Escherichia coli research in Latin America. Microorganisms. (2018) 6:100. doi: 10.3390/microorganisms6040100
36. Breijyeh, Z, Jubeh, B, and Karaman, R. Resistance of gram-negative Bacteria to current antibacterial agents and approaches to resolve it. Mol Basel Switz. (2020) 25:1340. doi: 10.3390/molecules25061340
37. Halawa, EM, Fadel, M, Al-Rabia, MW, Behairy, A, Nouh, NA, Abdo, M, et al. Antibiotic action and resistance: updated review of mechanisms, spread, influencing factors, and alternative approaches for combating resistance. Front Pharmacol. (2023) 14:1305294. doi: 10.3389/fphar.2023.1305294
38. Xu, C, Kong, L, Gao, H, Cheng, X, and Wang, X. A review of current bacterial resistance to antibiotics in food animals. Front Microbiol. (2022) 13:822689. doi: 10.3389/fmicb.2022.822689
39. Zhang, T, Nickerson, R, Zhang, W, Peng, X, Shang, Y, Zhou, Y, et al. The impacts of animal agriculture on one health-bacterial zoonosis, antimicrobial resistance, and beyond. One Health. (2024) 18:100748. doi: 10.1016/j.onehlt.2024.100748
40. Falgenhauer, L, Ghosh, H, Guerra, B, Yao, Y, Fritzenwanker, M, Fischer, J, et al. Comparative genome analysis of IncHI2 VIM-1 carbapenemase-encoding plasmids of Escherichia coli and Salmonella enterica isolated from a livestock farm in Germany. Vet Microbiol. (2017) 200:114–7. doi: 10.1016/j.vetmic.2015.09.001
41. Gregova, G, and Kmet, V. Antibiotic resistance and virulence of Escherichia coli strains isolated from animal rendering plant. Sci Rep. (2020) 10:17108. doi: 10.1038/s41598-020-72851-5
42. Destoumieux-Garzón, D, Mavingui, P, Boetsch, G, Boissier, J, Darriet, F, Duboz, P, et al. The one health concept: 10 years old and a long road ahead. Front Vet Sci. (2018) 5:14. doi: 10.3389/fvets.2018.00014
43. Ejikeugwu, C, Nworie, O, Saki, M, Al-Dahmoshi, HOM, Al-Khafaji, NSK, Ezeador, C, et al. Metallo-β-lactamase and AmpC genes in Escherichia coli, Klebsiella pneumoniae, and Pseudomonas aeruginosa isolates from abattoir and poultry origin in Nigeria. BMC Microbiol. (2021) 21:124. doi: 10.1186/s12866-021-02179-1
44. Chen, Q, Gong, X, Zheng, F, Ji, G, Li, S, Stipkovits, L, et al. Interplay between the phenotype and genotype, and efflux pumps in drug-resistant strains of Riemerella anatipestifer. Front Microbiol. (2018) 9:2136. doi: 10.3389/fmicb.2018.02136
45. Hall, RM, Collis, CM, Kim, MJ, Partridge, SR, Recchia, GD, and Stokes, HW. Mobile gene cassettes and integrons in evolution. Ann N Y Acad Sci. (1999) 870:68–80. doi: 10.1111/j.1749-6632.1999.tb08866.x
46. Partridge, SR, Kwong, SM, Firth, N, and Jensen, SO. Mobile genetic elements associated with antimicrobial resistance. Clin Microbiol Rev. (2018) 31:e00088–17. doi: 10.1128/CMR.00088-17
Keywords: fox, pneumonia, Escherichia coli , multi-drug resistance, multilocus sequence typing
Citation: Zhang C, Li H, Zhao Q, Wang L, Hou G, Shi Q, Wu T, Gao G and Zhang Z (2025) Drug resistance and pathogenicity characteristics of Escherichia coli causing pneumonia in farmed foxes. Front. Vet. Sci. 12:1567009. doi: 10.3389/fvets.2025.1567009
Edited by:
Guoqiang ZHU, Yangzhou University, ChinaReviewed by:
Yang Yang, Agricultural Research Service (USDA), United StatesNa Jiang, Beijing Academy of Agricultural and Forestry Sciences, China
Copyright © 2025 Zhang, Li, Zhao, Wang, Hou, Shi, Wu, Gao and Zhang. This is an open-access article distributed under the terms of the Creative Commons Attribution License (CC BY). The use, distribution or reproduction in other forums is permitted, provided the original author(s) and the copyright owner(s) are credited and that the original publication in this journal is cited, in accordance with accepted academic practice. No use, distribution or reproduction is permitted which does not comply with these terms.
*Correspondence: Zhiqiang Zhang, emhhbmd6aGlxaWFuZzg3QGhldnR0Yy5lZHUuY24=