- 1Department of Animal Health, Istituto Zooprofilattico Sperimentale della Sardegna, Sassari, Italy
- 2Council for Agricultural Research and Economics (CREA)- Research Centre for Animal Production and Aquaculture, Monterotondo (RM), Italy
- 3National Reference Centre for Hygiene and Technologies of Mediterranean Buffalo Farming and Productions, Istituto Zooprofilattico Sperimentale del Mezzogiorno, Salerno, Italy
Brucellosis is a zoonotic disease, with an important economic impact on the livestock industry and public health worldwide. Both Brucella abortus and Brucella melitensis can infect Mediterranean Buffalo (Bubalus bubalis), leading to infertility and abortion. In ruminants, the standard diagnostic approach involves two serological tests, the Rose Bengal Test and the Complement Fixation Test, applied in parallel, though their specificity requires improvement. Cytokines play a crucial role in coordinating immune responses through complex networks and can serve as biomarkers for various diseases. This study explored the potential use of cytokines as immunological biomarkers for Brucella infection in Mediterranean Buffalo. For this purpose, we included 18 healthy and 20 Brucella-infected buffaloes in our analysis. Heparinized blood samples were stimulated with the Brucella antigen, with PBS as nil control and PWM as lymphocyte viability control. After 16–24 h, plasma levels of IL-1α, IL-1β, IL-4, IL-6, IL-10, IL-17, IL-36Ra, MIP-1α, MIP-1β, MCP-1, CXCL8, IP-10, IFN-γ, TNF, and VEGF-A were measured using multiplex ELISA. Our results showed that infected animals released significantly higher levels of IFN-γ, IP-10, MCP-1 in response to Brucella antigen compared to healthy controls. Conversely, healthy animals released instead higher levels of IL-1α, IL-1β, IL-6 and IL-10 following antigen stimulation compared to infected animals. Finally, sequential canonical discriminant analyses were performed to generate predictive cytokine profiles for each group. The findings indicated that a combination of five cytokines (IFN-γ, IP-10, IL-1α, IL-1β, IL-6) can effectively distinguished infected from healthy buffaloes. Overall, this study suggests that incorporating these key immune cytokines could improve the diagnostic accuracy of brucellosis in Mediterranean Buffalo.
1 Introduction
Brucellosis is a zoonotic disease caused by bacteria belonging to the genus Brucella (1). This disease has significant economic impact for the livestock industry and pose a serious public health concern.
Brucella are Gram-negative coccobacilli and intracellular pathogens capable of surviving and replicating within macrophages (1). Both Brucella abortus (B. abortus) and Brucella melitensis (B. melitensis) can infect buffalo species (Bubalus bubalis) (2). In Italy, B. abortus is the most prevalent Brucella species affecting cattle and buffaloes, with several outbreaks reported in recent years, particularly in the province of Caserta (3). In Mediterranean Buffaloes, the infection leads to infertility and abortion, resulting in considerable economic losses (1). In humans, B. abortus infection causes a severe, chronic and debilitating disease (4), primarily transmitted through contaminated milk and raw dairy products (5) or direct contact with infected animals (6).
Italy is currently running a compulsory eradication and surveillance program for brucellosis in cattle and Mediterranean Buffaloes. In regions not yet declared free of the disease, surveillance is conducted through serological testing of all animals over 1 year old (7). To enhance specificity, at least two diagnostic tests should be used in parallel (8).
A widely accepted diagnostic approach combines the Rose Bengal Test (RBT) with the Complement Fixation Test (CFT) (8). Nevertheless, these tests present some limitations, particularly in cattle and buffaloes, due to their low specificity. False-positive serological reactions (FPSR) might result from exposure to cross reacting microorganisms (9). Both RBT and CFT can detect antibodies produced to the S-LPS and false positive results might occur in animals exposed to Gram-negative bacteria with LPS O-chains similar to those of brucellae. These bacteria include Escherichia coli O:157, Salmonella group N (O:30), and Yersinia enterocolitica O:9. Notably, Y. enterocolitica O:9 is a major cause of FPSR in diagnosis of brucellosis in bovine and buffaloes (9, 10).
The lack of Brucella specific antigen highlights the need to develop new diagnostic methods, in order to improve the efficacy of eradication strategies and to avoid un-necessary animal sacrifices. In addition, in Italy Mediterranean buffaloes are regarded as a national livestock heritage (7, 11). Accurate diagnosis is essential for an effective surveillance in brucellosis-free areas and for achieving the final stages of eradication (11). Advancing our understanding of host immune responses to Brucella is crucial for developing improved diagnostic strategies and ultimately eliminating the disease from affected infected regions.
In our previous studies, we demonstrated the effectiveness of the interferon-gamma (IFN-γ) assay test for diagnosing Mycobacterium bovis (M. bovis) infection in Mediterranean Buffaloes (12, 13). More recently, we identified additional cytokines that could enhance M. bovis diagnostic accuracy in this species (14). Building on this knowledge, we investigated the potential of key immune cytokines as diagnostic biomarkers for Brucella infection in Mediterranean Buffaloes.
2 Materials and methods
2.1 Ethical statements
Mediterranean Buffaloes used in this study were analyzed within the context of National and Regional buffalo brucellosis-surveillance program, provided by the EU Delegates Regulations 2020/689 and Campania Region Regional Regulation (DGRC 104/2022) (15, 16).
No animal was harmed or killed for the specific purpose of this study and the experimental procedure was carried out in compliance with the European Directive 210/63/UE and the Italian regulation D Lgs n26/2014.
2.2 Animals and study design
Thirty-eight Mediterranean Buffaloes were enrolled in the study and were divided in two groups: healthy (N = 18) and infected (N = 20).
Infected animals were selected from herds with confirmed brucellosis outbreaks. Infection status was determined according to current legislation using serological tests RBT and CFT, following the guidelines outlined in the WOAH Manual of Diagnostic Tests and Vaccines for Terrestrial Animals (8). RBT is used as a screening test (carried out every 6 months in all herds of Campania region), while CFT is used to confirm RBT positive cases. If an animal tests positive on CFT, the herd is classified as a brucellosis outbreak, then it is subsequently monitored every 21 days using both RBT and CFT (8). Seropositive animals were culled in compliance with national and regional regulations. Brucella presence in selected organs was assessed through PCR and culture isolation (see Section 2.3). Animals included in the infected group were seropositive, with Brucella DNA detected in target organs (Supplementary Table S1). Brucella was isolated from at least one animal per outbreak.
Healthy animals were selected from Officially Brucellosis-Free (OTF) herds in the Campania region (Southern Italy). These animals tested negative during the annual serological screening tests performed in the last 6 years (Supplementary Table S1).
2.3 PCR and culture isolation
Lymph nodes (retropharyngeal, supra-mammary, iliac, and mandibular lymph nodes) and other organs (spleen, uterus, mammary gland) were collected and sent to the IZSME (Portici, Italy) for Brucella detection though PCR and culture isolation.
PCR analysis was performed to detect Brucella DNA in the collected tissues. Genomic DNA was extracted using the QIAamp DNA MINI KIT (QIAGEN) following the manufacturer’s instructions, as previously described (17). Then, the identification of the Brucella spp. was performed though a real-time PCR assay using TaqMan probes and targeting IS711 (18). The presence of viable Brucella bacteria was assessed through culture isolation, according to the WOAH Manual of Diagnostic Tests and Vaccines for Terrestrial Animals (8). Bacterial isolates were further identified using the VITEK 2 system (3).
2.4 Whole blood stimulation and evaluation of cytokines release
Blood samples were collected from the jugular vein and heparin was used as anticoagulant and processed within 8 h of collection.
Whole blood from each animal was dispensed in aliquots of 1 mL, using a 24 well-plate and stimulated with Phosphate-buffered saline (PBS, nil Control Antigen) or Brucella antigen (Brucellergene® OCB) (100 μL per well, corresponding to 1,500–2,500 UI) or Pokeweed Mitogen (PWM, final concentration 1 μg/mL, used as a lymphocyte viability control). After incubation for 16–24 h at 37°C in a humidified atmosphere, plasma samples were collected. Then, levels of 15 key immune cytokines (IFN-γ, IL-1α, IL-1β, IL-4, IL-6, IL-10, IL17, IL-36Ra, MIP-1α, MIP-1β, MCP-1, IP-10, CXCL8, TNF, VEGF-A) were measured using Bovine Cytokine/Chemokine Magnetic Bead Panel Multiplex assay (Merck Millipore, Darmstadt, Germany) and a Bioplex MAGPIX Multiplex Reader (Bio-Rad, Hercules, CA, United States), as previously described (14). All samples were tested in duplicate (two technical replicates).
Antigen-specific cytokine in responses were calculated by subtracting baseline cytokines concentrations (PBS, nil control) from those of the antigen condition (Brucellergene® OCB).
2.5 Statistical analysis
Levels of the 15 tested cytokines were analyzed using the general linear model (GLM) to estimate the mean of each trait per stimulus (PBS, BRC, and PWM) within groups (healthy and infected):
where Yjk is the trait measured for each animal, μ is the overall mean, Gj is the fixed effect of the stimuli (j = 3 levels: PBS, BRC, and PWM), and ejk is the random residual effect of each observation.
The statistical significance of all traits and least-square means were assessed by Dunnet’s multiple test in the GLM procedure.
Additionally, the difference (∆_cytokine) between the level of each specific cytokine measured in the Brucella antigen condition (BRC) and its baseline concentration (PBS) was analyzed and displayed by GraphPad Prism 10.01 (GraphPad Software Inc., La Jolla, CA, United States).
A multivariate approach was conducted using canonical discriminant analysis (CDA) on 15, 7 and finally 5 ∆_cytokines by the CANDISC Procedure.
The significance level for both statistical analyses was set at a p-value < 0.05.
The CDAs were conducted by categorizing animals prior to healthy and infected. The CANDISC method was utilized to estimate linear functions of all quantitative variables that best discriminated against the groups while minimizing the variation within each group.
All statistical analyses were performed with SAS software version 9.4.
3 Results
Whole blood samples from healthy (N = 18) and infected (N = 20) Mediterranean Buffaloes were stimulated with a specific Brucella antigen (Brucellergene® OCB), alongside controls, and then the release of key immune cytokines was determined.
In both infected and healthy animal groups, PWM triggered the release of IFN-γ, IL-17, IL-10, and TNF indicating that T cells viability and functionality were not altered by treatments that could have affected the analyses (e.g., corticosteroid administration) (Table 1). PWM triggered also the release of chemokines IP-10, MIP-1α, and MIP-1β (Table 1), suggesting that the viability of other immune cells (e.g., monocytes) was not altered as well.
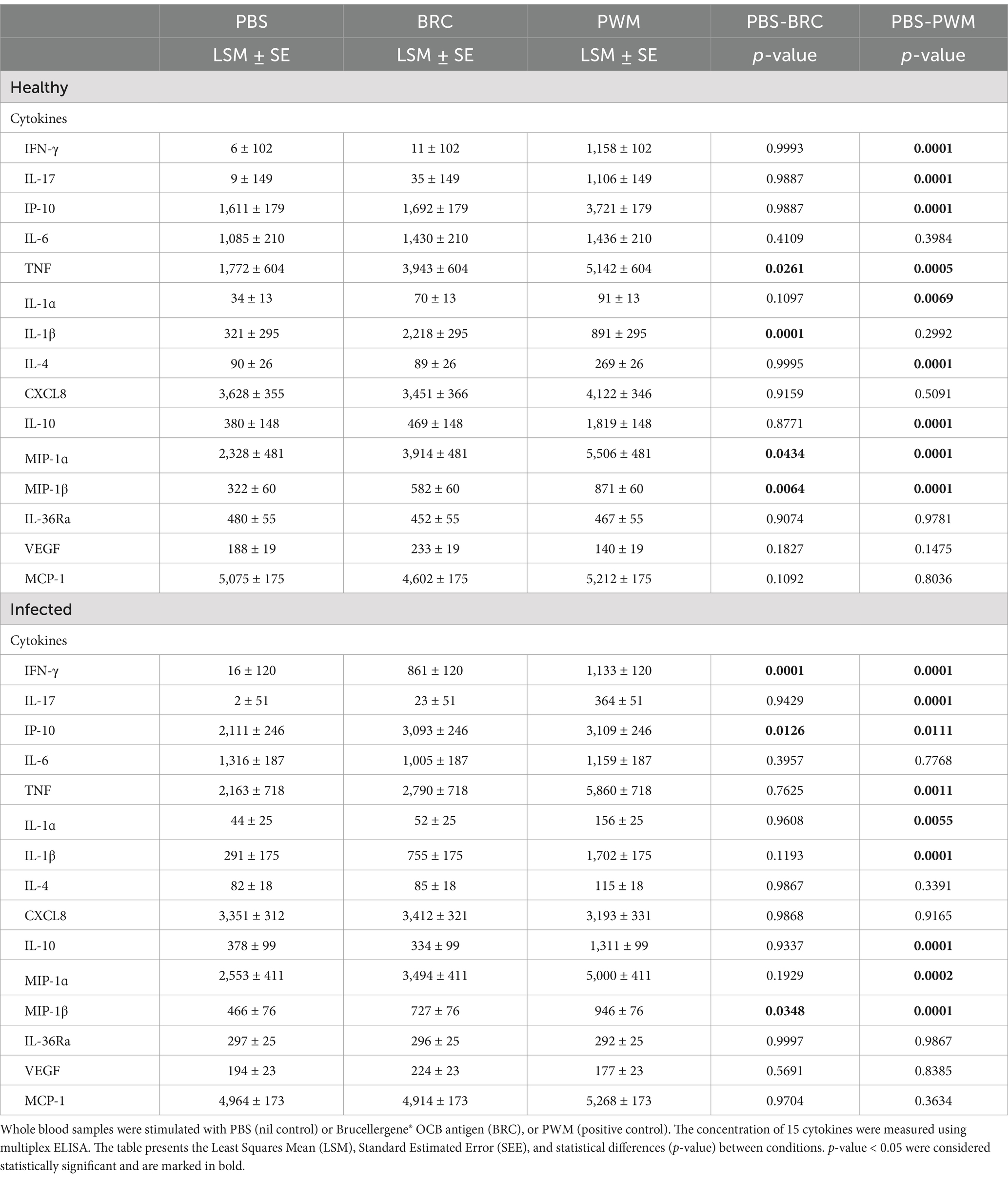
Table 1. Cytokine production in whole blood from healthy and Brucella-infected Mediterranean Buffaloes.
In the infected group, but not in the healthy group, stimulation with Brucella antigen (BRC) led to a significantly higher release of IFN-γ and IP-10 compared to the nil control (Table 1). Both groups exhibited an increase in MIP-1β levels in response to Brucella antigen. In the healthy group, additional pro-inflammatory cytokines (TNF, IL-1β, MIP-1α) were also released upon antigen stimulation, suggesting an innate immune response to Brucella components in naïve animals.
Then, differences between the two groups (healthy and infected) in terms of antigen-specific cytokine releases were assessed. Baseline cytokines levels (PBS, nil control) were subtracted from those in the antigen condition (Brucellergene® OCB) and then differences between groups were analyzed.
In Figure 1, the results of four key T cell cytokines are presented: IFN-γ (Th1 response marker), IL-4 (Th2 response marker), IL-17 (mainly released by Th17), IL-10 (Treg-associated immunosuppressive cytokine) (19). Infected animals released higher levels of antigen-specific IFN-γ compared to healthy animals (p < 0.0001), whereas no significant differences were observed for IL-4 and IL-17. Conversely, healthy animals produced higher antigen-specific IL-10 levels compared to -infected subjects (p = 0.0184) (Figure 1).
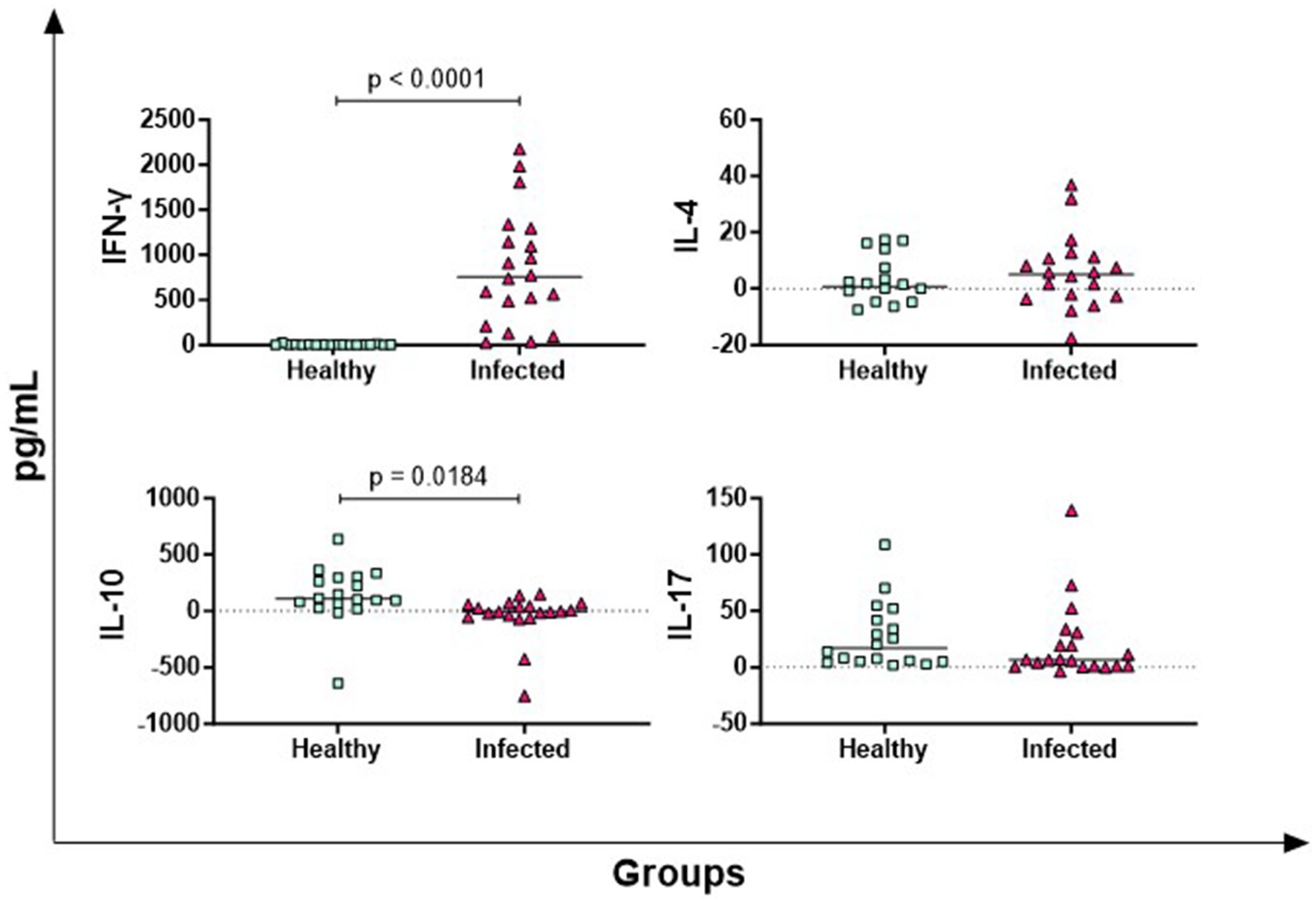
Figure 1. Antigen-specific release of T-cell cytokines in Brucella-Infected buffaloes. Blood samples from Brucella-infected (n = 20) and healthy (n = 18) Mediterranean Buffaloes were collected using heparin as anticoagulant. Whole blood was stimulated with PBS (nil control) or Brucellergene® OCB antigen (BRC). After 16–24 h of incubation, plasma was collected, and levels of T-cell cytokines (IFN-γ, IL-17, IL-4, IL-10) were determined using multiplex ELISA. Brucella-specific cytokines values were calculated by subtracting baseline cytokines levels (PBS) from those in antigen condition. Differences between groups are shown, with statistical significance set at p < 0.05.
In Figure 2, the results of four key pro-inflammatory cytokines are shown: IL-1α, IL-1β, IL-6, TNF (19). Infected animals released lower levels of antigen-specific IL-1α, IL-1β, IL-6 compared to healthy subjects, whereas TNF levels did not differ significantly between groups (Figure 2).
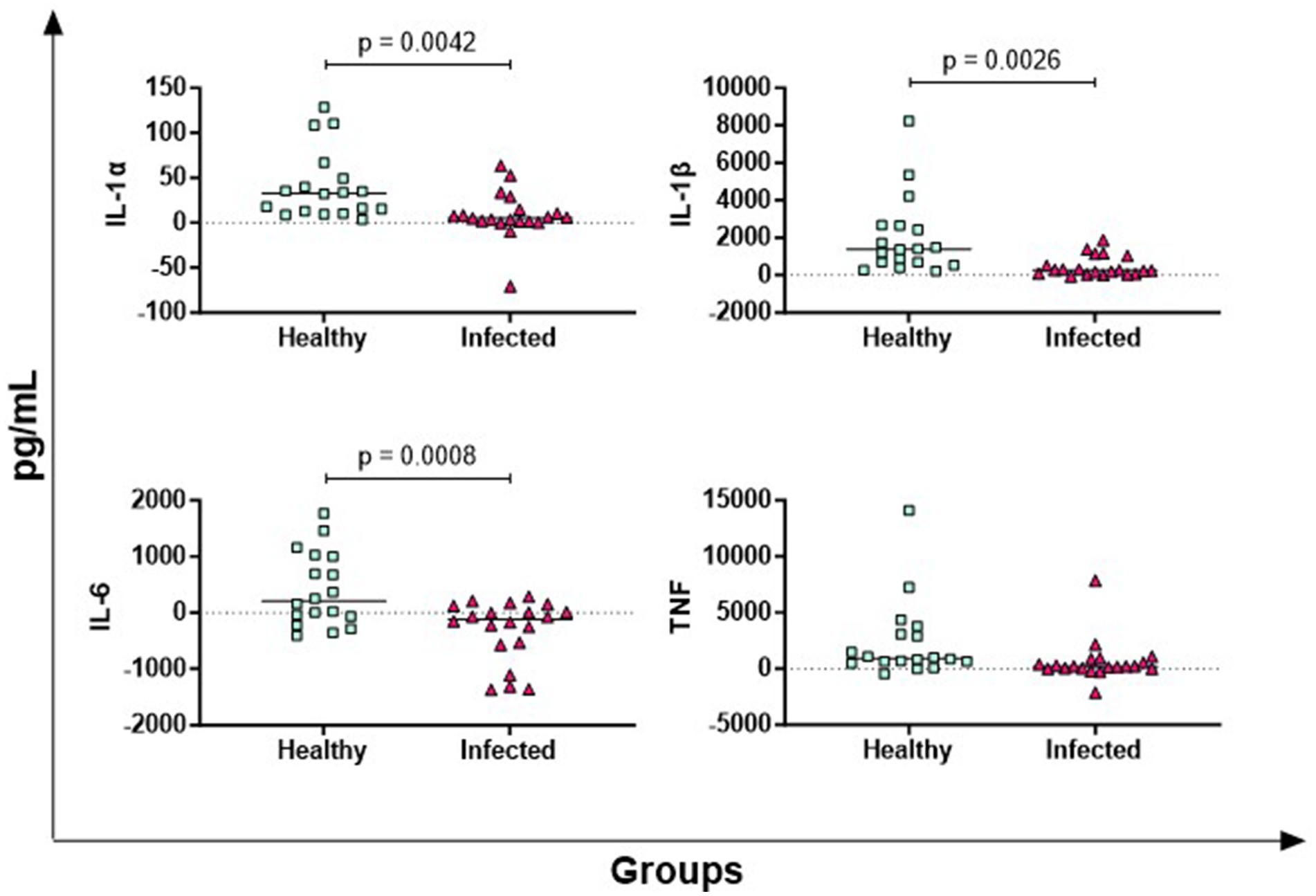
Figure 2. Antigen-specific release of pro-inflammatory cytokines in Brucella-infected buffaloes. Blood samples from Brucella-infected (n = 20) and healthy (n = 18) Mediterranean Buffaloes were collected using heparin as anticoagulant. Whole blood was stimulated with PBS (nil control) or Brucellergene® OCB antigen (BRC). After 16–24 h of incubation, plasma was collected, and levels of pro-inflammatory cytokines (IL-1α, IL-1β, IL-6, TNF) were measured using multiplex ELISA. Brucella-specific cytokines values were determined by subtracting baseline cytokines levels (PBS) from those in the antigen condition. Differences between the groups are shown, with statistical significance set at p < 0.05.
The results of five key chemokines are presented in Figure 3. No differences between groups were observed for MIP-1α and MIP-1β, whereas infected animals released higher levels of antigen-specific IP-10 (p < 0.0001) and MCP-1 (p = 0.0277) compared to healthy subjects. Regarding CXCL8, 5 out of 38 buffaloes presented cytokine levels higher than the detection limit of the kit, so they were excluded from the analysis. In the other animals (17 infected vs. 16 healthy), no differences were observed between groups (Figure 3).
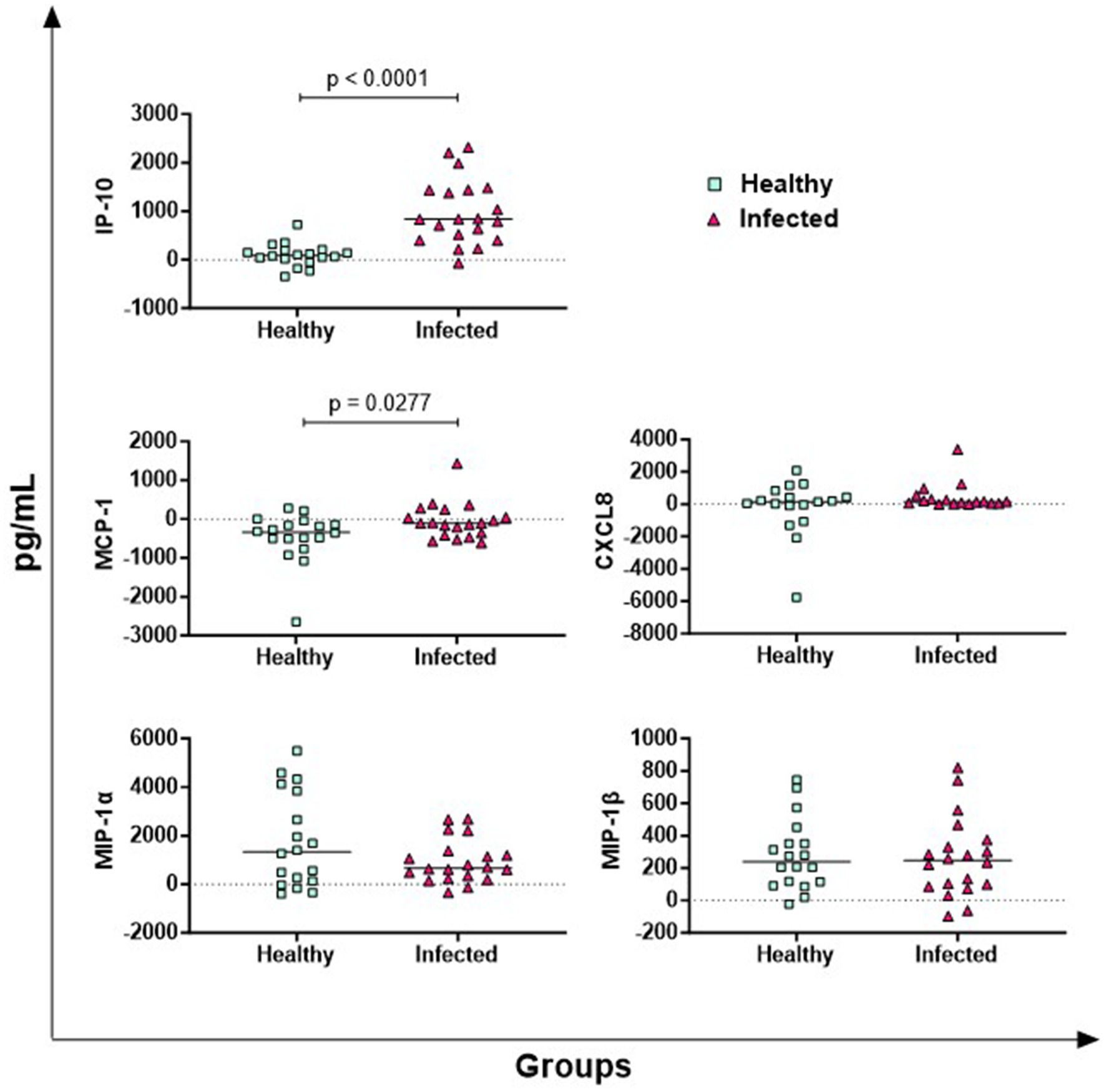
Figure 3. Antigen-specific release of chemokines in Brucella-Infected buffaloes. Blood samples from Brucella-infected (n = 20) and healthy (n = 18) Mediterranean Buffaloes were collected using heparin as anticoagulant. Whole blood was stimulated with PBS (nil control) or antigen Brucellergene® OCB antigen (BRC). After 16–24 h of incubation, plasma was collected, and levels of key chemokines (IP-10, MIP-1α, MIP-1β, MCP-1, CXCL8) were measured using multiplex ELISA. Brucella-specific cytokines values were calculated by subtracting baseline cytokines levels (PBS) from those in antigen condition. Differences between the groups are shown, with statistical significance set at p < 0.05.
The antigen-specific release of IL-36Ra (receptor antagonist) and VEGF-A (growth factor) was also investigated, but no significant differences between groups were observed (Figure 4).
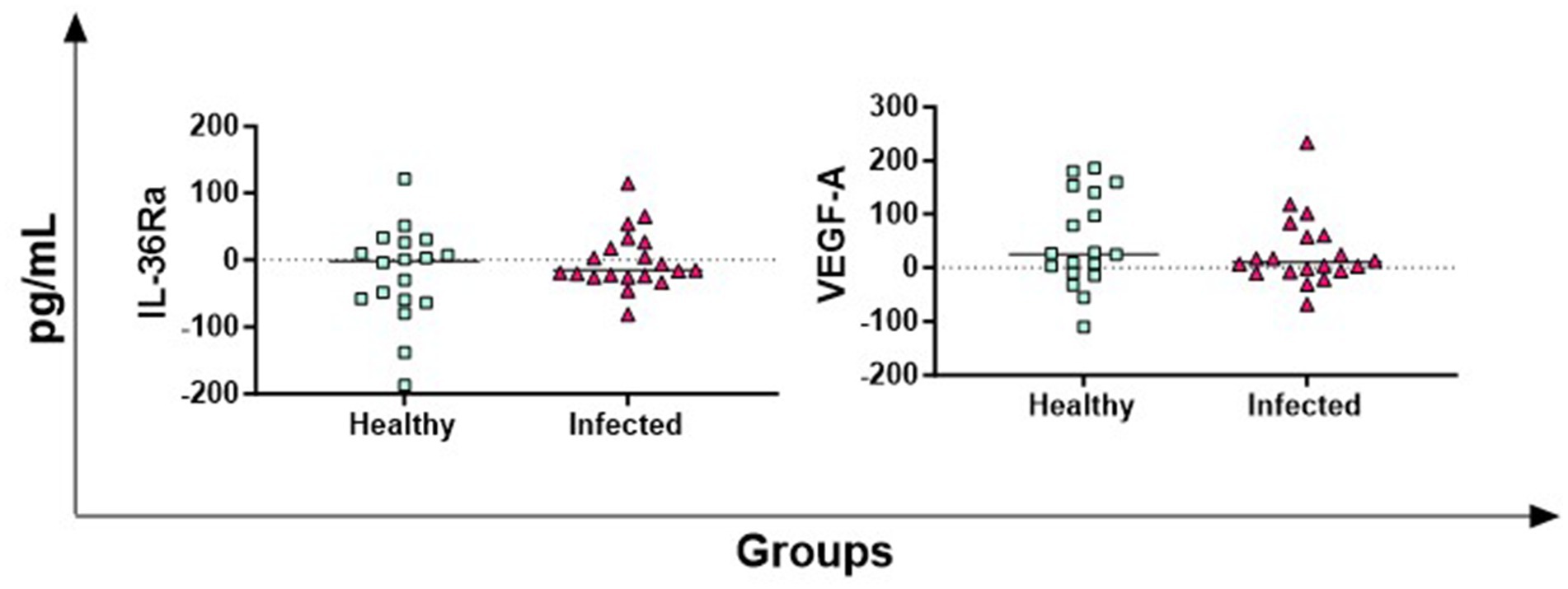
Figure 4. Antigen-specific release of IL-36Ra and VEGF-A in Brucella-Infected buffaloes. Blood samples from Brucella-infected (n = 20) and healthy (n = 18) Mediterranean Buffaloes were collected using heparin as anticoagulant. Whole blood was stimulated with PBS (nil control) or Brucellergene® OCB antigen (BRC). After 16–24 h of incubation, plasma was collected, and levels of IL-36Ra and VEGF-A were determined through multiplex ELISA. Brucella-specific cytokines values were quantified by subtracting baseline cytokines levels (PBS) from those in the antigen condition. Differences between the groups are shown, with statistical significance set at p < 0.05.
Canonical discriminant analysis was then used to generate predictive cytokine profiles distinguishing healthy and infected animals as potential diagnostic biomarkers. First, a CDA was performed with the 15 cytokines analyzed in the study. As presented in Figure 5, these 15 cytokines can clearly differentiate between the two groups under evaluation. Table 2A reports the factor loading (FL) for each cytokine in the canonical variable (Can 1), showing a positive and high correlation with ∆_IFN-γ, ∆_IP-10 (FL ≥ 0.70), characterizing the infected group, and a negative correlation with ∆_IL-6, ∆_IL-10, ∆_IL1-β, and ∆_IL1-α (FL ≥ 0.50), characterizing the healthy one.
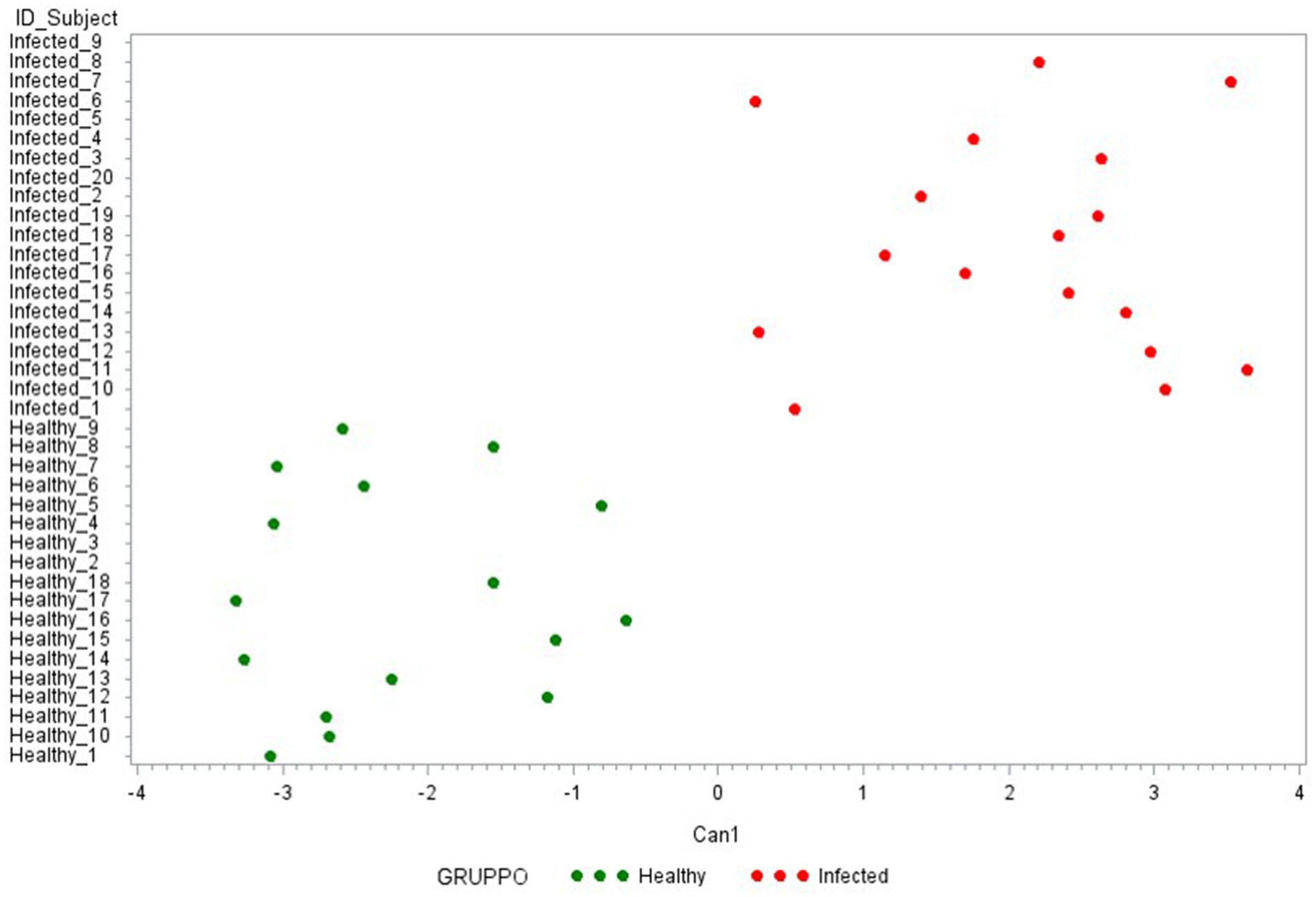
Figure 5. Plot from canonical discriminant analysis. Canonical discriminant analysis on 15 Δ_cytokines by the CANDISC Procedure. Animals belonging to the two groups are displayed based on the canonical function Can1.
Then, to identify the most discriminating combination of cytokines, two other CDAs were conducted. The first one was performed with the 7 ∆_cytokine levels that significantly differentiate the two groups (IFN-γ, IP-10, MCP-1, IL-6, IL1-β, IL-1α, IL-10). As presented in Figure 6, this model clearly discriminated against the two groups, except of one healthy animal (Healthy_2). Table 2B reports the cytokine FL in this new canonical function, revealing a high correlation with ∆_IFN-γ, ∆_IP-10 (0.80–0.79, respectively) and a medium one with IL-6, ∆_IL1-β, and ∆_IL1-α (FL ≥ 0.50).
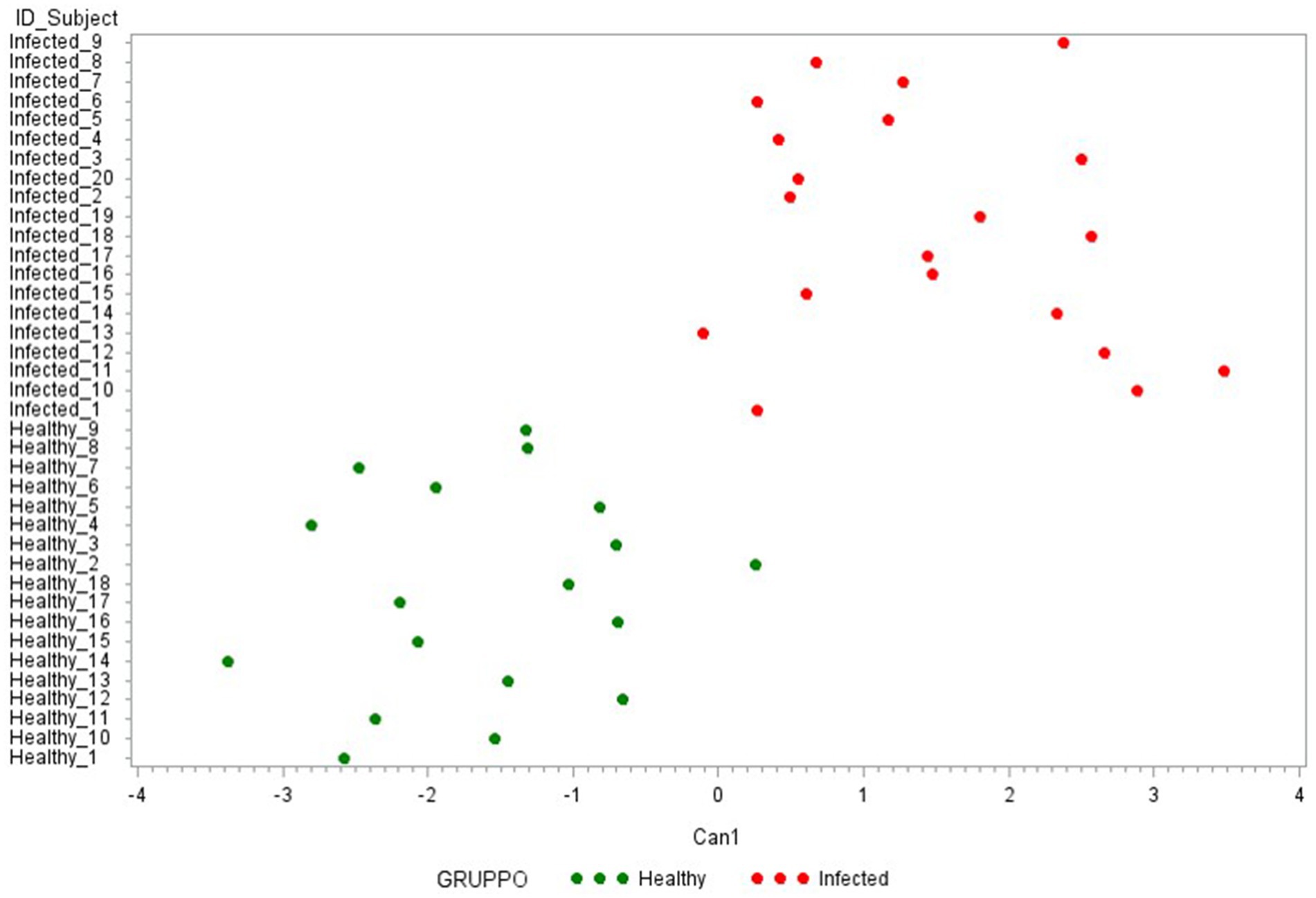
Figure 6. Plot from canonical discriminant analysis. Canonical discriminant analysis on 7 Δ_cytokines by the CANDISC Procedure. Animals belonging to the two groups are displayed based on the canonical function Can1.
Finally, the third CDA (Table 2C) was conducted using only the 5 cytokines with an FL ≥ 0.50 in the previous Can 1: IFN-γ, IP-10, IL-6, IL1-β, and IL1-α. As presented in Figure 7, the use of these 5 cytokines can clearly differentiate the two groups under evaluation (healthy-infected).
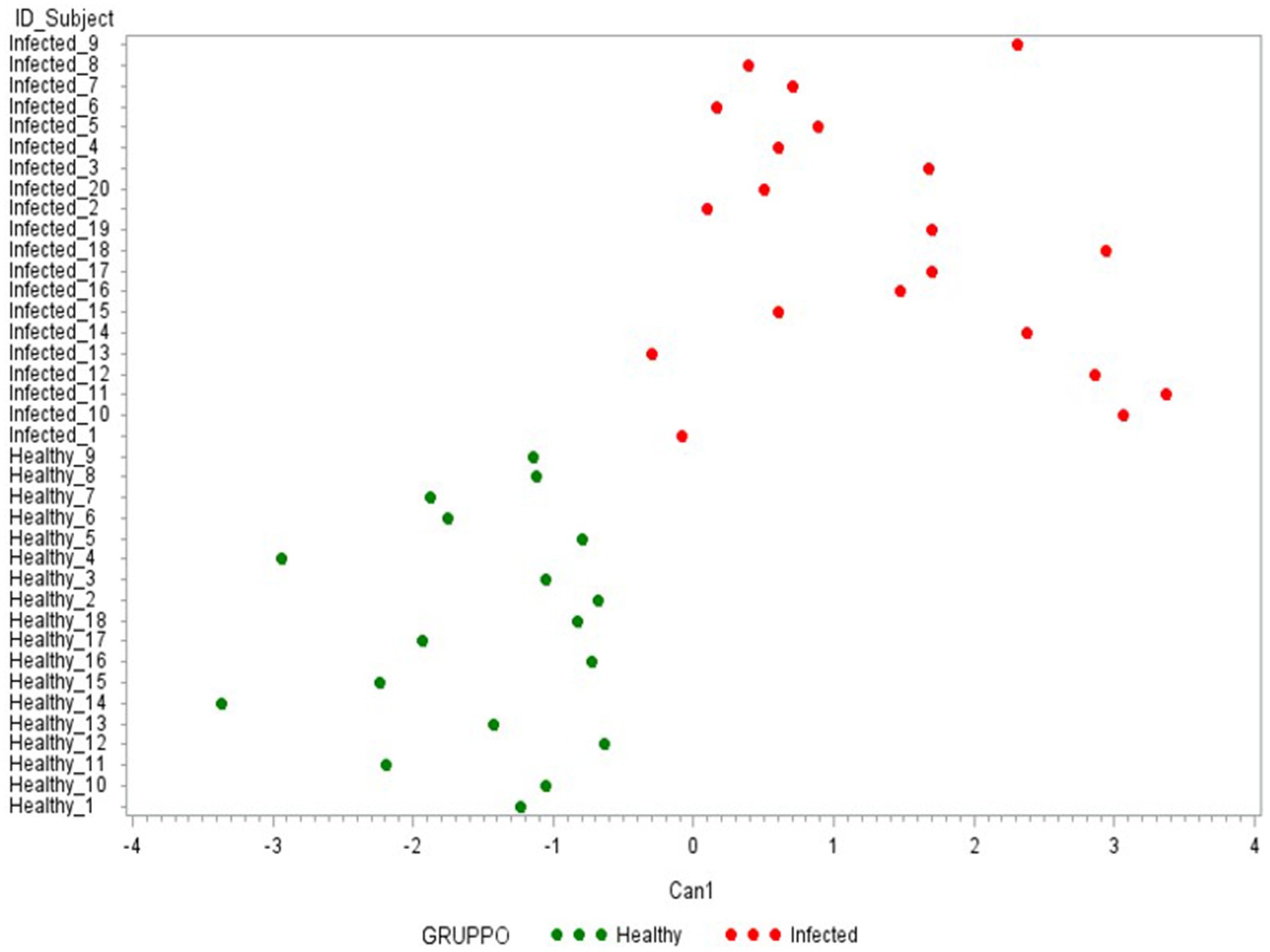
Figure 7. Plot from canonical discriminant analysis. Canonical discriminant analysis on 5 Δ_cytokines by the CANDISC Procedure. Animals belonging to the two groups are displayed based on the canonical function Can1.
4 Discussion
Brucella is a globally distributed zoonotic pathogen that affects various domestic and wild animals species, including Mediterranean Buffalo (3, 20). Early and accurate detection of Brucella infection in this specie is crucial for effective disease control.
A widely accepted diagnostic approach for Brucella in cattle and buffaloes combines two serological assays (the RBT with the CFT), although these test present some disadvantages, such as low specificity. False-positive serological reactions (FPSR) might result from exposure to cross reacting microorganisms, especially Gram-negative bacteria with LPS O-chains similar to those of Brucella (8, 9). There is a need to develop new diagnostic methods, in order to improve the efficacy of eradication strategies and to avoid unnecessary animal sacrifices. Brucella is an intracellular pathogen, which primarily targets macrophages and dendritic cells, employing sophisticated mechanism to both innate and adaptive immune response, enabling its survival and persistence within host cells (20). Resistance to intracellular bacterial pathogens relies on cell-mediated immunity, and it was reported that an adequate Th1 immune response is critical for the clearance of Brucella infection. In humans, IFN-γ is the key cytokine involved in the immune response against Brucella (20, 21). Cytokines are crucial mediators of immune responses and their quantification provides insights into physiological and pathological processes, aiding diagnosis and treatment, thus they have been widely studied as biomarkers for many diseases. Cytokine testing has the potential to support diagnosis also due to its lack of invasiveness and relative low cost (22).
Biomarker development is a multistep process that starts with its discovery in a pathophysiological context and progresses through various validation phases. This study provides preliminary insights into the role of key immune cytokines as biomarkers for Brucella infection in Mediterranean Buffaloes, with the aim to implement the diagnosis of Brucella in this specie. Understating cytokine responses to antigen-stimulation could offer also a more comprehensive picture of host immunity against this pathogen.
IFN-γ is an antiviral cytokine released mainly by NK and activated T cells; it is regarded as a hallmark of a Th1 response, which is associated with resistance to intracellular pathogens (23, 24). Our finding indicates that Brucella-infected buffaloes release higher levels of antigen-specific IFN-γ compared to uninfected controls. Similar observations have been reported in cattle infected with B. abortus, where infected animals exhibited elevated IFN-γ levels upon antigen stimulation (25, 26). Likewise, in B. melitensis infected sheep, increased IFN-γ secretion was noted following antigen exposure (27, 28). Overall, our data suggest that antigen-specific IFN-γ release could serve as a potential diagnostic marker for Brucella infection in Mediterranean Buffaloes.
We recently observed that Mediterranean Buffaloes infected with B. abortus presented lower levels of circulating T and B cells compared to healthy controls (29) and this might result in a different T-cell cytokine pattern in response to antigen stimulation. IL-17 and IL-4 releases were therefore investigated. IL-17 and IL-14 are regarded as hallmark of the Th17 and Th2 response, respectively (19, 30). IL-17 promotes inflammation and a granulocyte-dependent response to pathogens; it triggers the release of pro-inflammatory cytokines and enhances the recruitment of neutrophils (30). IL-4 promotes instead tissue regeneration and the differentiation of Th2 cells (19). Despite the important role of these cytokines in the immune response to several pathogens, little is known about their function during Brucella infection. In humans, the IL-17 serum levels were significantly higher in subjects with brucellosis compared to healthy controls and the levels of this cytokine decreased in patients after specific treatment (31). However, in our study, no differences in antigen-specific IL-17 or IL-4 secretion were observed between infected and healthy buffaloes, suggesting that these cytokines may not be reliable diagnostic markers for brucellosis in this specie.
IL-10 is a cytokine with strong anti-inflammatory action, with the ability to reduce or terminate inflammation (32). It promotes the expansion and persistence of T reg cells, which prevent autoimmunity and limit chronic inflammatory diseases (33). Our data revealed that infected buffaloes release lower levels of antigen-specific IL-10 compared to healthy subjects. Similarly, we observed that Mediterranean Buffaloes with brucellosis release lower levels of three pro-inflammatory cytokines than healthy controls: IL-1α, IL-1β, IL-6. These cytokines are typically released early during infection to initiate inflammation, with IL-10 acting to balance their effects (19, 32). Comparable findings have been reported in human patients with brucellosis, where monocytes exhibited diminished IL-1β, IL-6, and IL-10 secretion in response to LPS stimulation (34). Brucella employs immune evasion strategies, including inflammasome downregulation and inhibition of macrophage polarization toward a pro-inflammatory phenotype (M1) (34, 35). Our results suggest that Brucella infection impairs monocyte ability to release pro-inflammatory cytokines in response to pathogen associated molecular patterns, such as those contained in the antigen Brucellergene® OCB (protein extract of B. melitensis strain BB15).
TNF is another pro-inflammatory cytokine, which triggers the release of pro-inflammatory chemokines, effectively recruiting leukocytes to the inflammatory site (36). Studies in humans reported that monocytes and dendritic cells from patients with brucellosis released lower levels of TNF in response to external stimuli compared to healthy controls (34, 37). However, in our study, no differences in antigen-specific TNF secretion were observed between infected and healthy buffaloes. This discrepancy could be attributed to the involvement of other immune cells, such as CD4+ and CD8+ T cells, in TNF production (38), equalizing the difference between groups in terms of total TNF-release in response to antigen-stimulation. Our previous research suggested that TNF might be a useful biomarker for identifying buffaloes infected with M. bovis (14), but the current data indicate that TNF is not a suitable biomarker for Brucella infection.
We also examined the antigen-specific secretion of key chemokines: IP-10, MCP-1, MIP-1α, MIP-1β, CXCL8. Chemokines play a crucial role in immune cell recruitment to infected tissues (39). IP-10 is secreted by monocytes, macrophages, endothelial cells and fibroblasts and its release is sharply enhanced by IFN-γ (40). This chemokine triggers the recruitment of monocytes, macrophages, NK cells, and activated T cells to the inflammatory site (40). It has been identified as a promising biomarker for M. bovis infection in cattle and buffaloes (14, 41–44). In this study, we observed that Brucella-infected animals release high levels of antigen-specific IP-10 compared to uninfected controls. These data are in agreement with what observed in a mouse models of brucellosis: immune cells of mice immunized with a B. melitensis attenuated strains (WR201) release high levels of antigen-specific IP-10 compared to healthy controls (45). Overall, our preliminary data suggest that this chemokine could be a promising biomarker of brucellosis in buffaloes. MCP-1 is a strong chemoattractant for monocytes and it is released mainly by monocytes and macrophages (46). In a mouse model of brucellosis, immune cells of Brucella-infected mice release high levels of antigen-specific MCP-1 compared to healthy controls (45). In agreement, in our study we observed that Brucella-infected animals release high levels of antigen-specific MCP-1 compared to uninfected controls, suggesting that this chemokine could be a promising biomarker of brucellosis in buffaloes. MIP-1α and MIP-1β are pro-inflammatory chemokines mainly produced by monocytes/macrophages (47). They promote recruitment of diverse cell types (chemotaxis of monocytes, dendritic cells, T cells, NK cells, and granulocytes) to the inflammatory sites (47). Previous studies suggest that these chemokines were involved in the immune response to other intracellular bacteria infecting Mediterranean Buffaloes, such as M. bovis (14). Our data revealed that infected and healthy buffaloes release similar levels of antigen-specific MIP-1α and MIP-1β, suggesting that these two chemokines could not be useful biomarkers of brucellosis in buffaloes. CXCL8, also known as IL-8, is a strong neutrophil chemoattractant, which triggers the recruitment of neutrophils and other granulocytes to the site of infection, promotes neutrophil degranulation and enhancements in their phagocytic functions (39, 48). This chemokine is released by neutrophils and macrophages in response to Brucella infection (49, 50), but infection results also in a lower ability of macrophages to present antigen to T cells and to release pro-inflammatory cytokines in response to external stimuli (34, 51). Our data revealed that there were no differences between groups, suggesting that the evaluation of CXCL8 will not improve the diagnosis of brucellosis in Mediterranean Buffaloes.
Subsequently, we investigated the antigen-specific release of IL-36Ra and VEGF. IL-36Ra is the receptor antagonist of the pro-inflammatory interleukin IL-36 and it is secreted to prevent the development of an exacerbated inflammatory response to stressors (52). VEGF is a growth factor, and it possesses pro-angiogenic activity, promoting endothelial cell survival, cell migration and increasing vascular permeability (53). We observed no differences between infected and healthy animals, suggesting that they are unlikely to serve as diagnostic biomarkers for Brucella infection in buffaloes.
Finally, we aimed to identify the cytokine set which better discriminate Brucella-infected and healthy Mediterranean Buffaloes. Three canonical analyses were performed sequentially to reduce the number of cytokines while ensuring effective discrimination between the two groups of animals. From the initial 15, we narrowed it down to five: our analysis showed that the quantitative determination of IFN-γ, in parallel with the chemokine IP-10 and three pro-inflammatory cytokines (IL-6, IL-1α and IL-1β) could be useful in the diagnosis of brucellosis in Mediterranean Buffaloes. The infected group is characterized by high values of IFN-γ and IP-10, unlike the other three pro-inflammatory markers, which have higher values in healthy subjects.
Overall, our data suggest that IFN-γ, IP-10, IL-1a, IL-1ß, IL-6 could enhance brucellosis diagnosis in Mediterranean Buffaloes. These preliminary observation should be validated on a larger set of samples, in order to properly establish the sensitivity and specificity of these ELISAs. In particular, samples from buffaloes infected with other Gram-negative bacteria should be included in the analysis, to evaluate the specificity of these potential biomarkers and to implement the diagnosis of Brucella in this specie, in order to avoid un-necessary animal sacrifices. In conclusion, these preliminary findings provide a foundation for developing cytokine-based diagnostic tools for Brucella infection in Mediterranean Buffaloes.
Data availability statement
The raw data supporting the conclusions of this article will be made available by the authors, without undue reservation.
Ethics statement
The animal study was approved by the Institutional Ethics Committee of the Istituto Zooprofilattico Sperimentale del Mezzogiorno. Mediterranean Buffaloes used in this study were analyzed within the context of official eradication program, therefore were not considered experimental animals. The study was conducted in accordance with the local legislation and institutional requirements.
Author contributions
GF: Conceptualization, Data curation, Formal analysis, Investigation, Writing – original draft. FS: Conceptualization, Data curation, Formal analysis, Investigation, Writing – review & editing. AD: Investigation, Methodology, Writing – review & editing. LS: Investigation, Methodology, Writing – review & editing. MN: Investigation, Methodology, Writing – review & editing. GM: Data curation, Investigation, Writing – review & editing. FG: Data curation, Investigation, Writing – review & editing. SZ: Investigation, Methodology, Writing – review & editing. VB: Investigation, Methodology, Writing – review & editing. SD: Formal analysis, Investigation, Methodology, Writing – review & editing. EC: Investigation, Resources, Writing – review & editing. GG: Investigation, Resources, Writing – review & editing. FN: Conceptualization, Data curation, Formal analysis, Investigation, Writing – review & editing. AM: Conceptualization, Funding acquisition, Investigation, Project administration, Resources, Writing – review & editing.
Funding
The author(s) declare that financial support was received for the research and/or publication of this article. This research was funded by the Italian Ministry for Health (grant number Research Project RC IZSME 11/21 RC).
Acknowledgments
The authors would like to acknowledge the Veterinary Services for their help in sample collection.
Conflict of interest
The authors declare that the research was conducted in the absence of any commercial or financial relationships that could be construed as a potential conflict of interest.
The author(s) declared that they were an editorial board member of Frontiers, at the time of submission. This had no impact on the peer review process and the final decision.
Generative AI statement
The author(s) declare that no Gen AI was used in the creation of this manuscript.
Publisher’s note
All claims expressed in this article are solely those of the authors and do not necessarily represent those of their affiliated organizations, or those of the publisher, the editors and the reviewers. Any product that may be evaluated in this article, or claim that may be made by its manufacturer, is not guaranteed or endorsed by the publisher.
Supplementary material
The Supplementary material for this article can be found online at: https://www.frontiersin.org/articles/10.3389/fvets.2025.1583858/full#supplementary-material
References
1. Franc, KA, Krecek, RC, Häsler, BN, and Arenas-Gamboa, AM. Brucellosis remains a neglected disease in the developing world: a call for interdisciplinary action. BMC Public Health. (2018) 18:125. doi: 10.1186/s12889-017-5016-y
2. Jun-Feng, S, Qing-Long, G, Bo, Z, Ma, BY, Chen, ZY, Yang, Y, et al. Seroprevalence of brucellosis in buffalo worldwide and associated risk factors: a systematic review and meta-analysis. Front Vet Sci. (2021) 8:553. doi: 10.3389/fvets.2021.649252
3. Orrù, L, Lamontanara, A, Mascolo, C, Borriello, G, Paradiso, R, Cerrone, A, et al. Genetic diversity of Brucella abortus strains from cattle and water buffalo in the Italian province of Caserta. Vet Microbiol. (2024) 299:110314. doi: 10.1016/j.vetmic.2024.110314
4. Franco, MP, Mulder, M, and Gilman, RH. Human brucellosis. Lancet Infect Dis. (2007) 7:775–86. doi: 10.1016/S1473-3099(07)70286-4
5. Dadar, M, Shahali, Y, and Whatmore, AM. Human brucellosis caused by raw dairy products: a review on the occurrence, major risk factors and prevention. Int J Food Microbiol. (2019) 292:39–47. doi: 10.1016/j.ijfoodmicro.2018.12.009
6. Govindasamy, K, Etter, EMC, Harris, BN, Rossouw, J, Abernethy, DA, and Thompson, PN. Knowledge of brucellosis, health-seeking behaviour, and risk factors for brucella infection amongst workers on cattle farms in Gauteng, South Africa. Pathogens. (2021) 10:1484. doi: 10.3390/pathogens10111484
7. Mazzeo, A, Tremonte, P, Rossi, N, Ferrara, C, Mascolo, C, Lombardi, SJ, et al. Modulation of the one health approach to tackle brucellosis in buffaloes and cattle in two Italian territories with different characteristics. J Buffalo Sci. (2023) 12:55–69. doi: 10.6000/1927-520X.2023.12.07
8. World Organization for Animal Health. Infection with Brucella abortus, B. melitensis and B. suis. Terr. Anim. Heal. Code 1–48 (2022). Avaialble at: https://www.woah.org/fileadmin/Home/fr/Health_standards/tahm/3.01.04_BRUCELLOSIS.pdf
9. Nielsen, K. Diagnosis of brucellosis by serology. Vet Microbiol. (2002) 90:447–59. doi: 10.1016/s0378-1135(02)00229-8
10. Muñoz, PM, Marín, CM, Monreal, D, González, D, Garin-Bastuji, B, Díaz, R, et al. Efficacy of several serological tests and antigens for diagnosis of bovine brucellosis in the presence of false-positive serological results due to Yersinia enterocolitica O:9. Clin Diagn Lab Immunol. (2005) 12:141–51. doi: 10.1128/CDLI.12.1.141-151.2005
11. Mainar-Jaime, RC, Munoz, PM, de Miguel, MJ, Grilló, MJ, Marín, CM, Moriyón, I, et al. Specificity dependence between serological tests for diagnosing brucellosis in Brucella-free farms showing false positive serological reactions due to Yersinia enterocolitica O:9. Can Vet J. (2005) 2005:913–6.
12. Martucciello, A, Vitale, N, Mazzone, P, Dondo, A, Archetti, I, Chiavacci, L, et al. Field evaluation of the interferon gamma assay for diagnosis of tuberculosis in Mediterranean Buffalo (Bubalus bubalis) comparing four interpretative criteria. Front Vet Sci. (2020) 7:563792. doi: 10.3389/fvets.2020.563792
13. Martucciello, A, Ottaiano, M, Mazzone, P, Vitale, N, Donniacuo, A, Brunetti, R, et al. A decade of tuberculosis eradication programs in the Mediterranean water buffalo (Bubalusbubalis) in South Italy: are we heading toward eradication? Front Vet Sci. (2024) 11:1405416. doi: 10.3389/fvets.2024.1405416
14. Franzoni, G, Signorelli, F, Mazzone, P, Donniacuo, A, De Matteis, G, Grandoni, F, et al. Cytokines as potential biomarkers for the diagnosis of Mycobacterium bovis infection in Mediterranean buffaloes (Bubalus bubalis). Front Vet Sci. (2024) 11:1512571. doi: 10.3389/fvets.2024.1512571
15. Commission Delegated Regulation (EU) 2020/689 of 17 December2019 supplementing regulation (EU) 2016/429 of the European parliamentand of the council as regards rules for surveillance, eradicationprogrammes, and disease-free status for certain listed and emergingdiseases. (2020), p. 211–340. Available online at: http://data.europa.eu/eli/reg_del/2020/689/oj (Accessed January 17, 2025).
16. DGRC “Compulsory program of eradication from infectious diseases of bovine and buffalo species in Campania Region” (2022)
17. Fusco, G, Cardillo, L, Valvini, O, Pucciarelli, A, Picazio, G, Cerrone, A, et al. Detection and quantification of Brucella abortus DNA in water buffaloes (bubalus bubalis) using droplet digital polymerase chain reaction. Vet Q. (2024) 44:1–8. doi: 10.1080/01652176.2024.2390944
18. Bounaadja, L, Albert, D, Chénais, B, Hénault, S, Zygmunt, MS, Poliak, S, et al. Real-time PCR for identification of Brucella spp.: a comparative study of IS711, bcsp31 and per target genes. Vet Microbiol. (2009) 137:156–64. doi: 10.1016/j.vetmic.2008.12.023
19. Dinarello, AC. Historical insights into cytokines. Eur J Immunol. (2007) 37:S34–45. doi: 10.1002/eji.200737772
20. Byndloss, MX, and Tsolis, RM. Brucella spp. Virulence factors and immunity. Annu Rev Anim Biosci. (2016) 4:111–27. doi: 10.1146/annurev-animal-021815-111326
21. Skendros, P, and Boura, P. Immunity to brucellosis. Rev Sci Tech. (2013) 32:137–47. doi: 10.20506/rst.32.1.2190
22. Liu, C, Chu, D, Kalantar-Zadeh, K, George, J, Young, HA, and Liu, G. Cytokines: from clinical significance to quantification. Adv Sci. (2021) 8:e2004433. doi: 10.1002/advs.202004433
23. Righi, C, Franzoni, G, Feliziani, F, Jones, C, and Petrini, S. The cell-mediated immune response against bovine alphaherpesvirus 1 (BoHV-1) infection and vaccination. Vaccine. (2023) 11:785. doi: 10.3390/vaccines11040785
24. Schroder, K, Hertzog, PJ, Ravasi, T, and Hume, DA. Interferon-γ: an overview of signals, mechanisms and functions. J Leukoc Biol. (2004) 75:163–89. doi: 10.1189/jlb.0603252
25. Priyanka Shringi, BN, Choudhary, OP, and Kashyap, SK. Expression profiling of cytokine-related genes in Brucella abortus infected cattle. Biol Rhythm Res. (2019) 52:654–65. doi: 10.1080/09291016.2019.1600263
26. Agnone, A, La Manna, MP, Vesco, G, Gargano, V, Macaluso, G, Dieli, F, et al. Analysis of interferon-gamma producing cells during infections by Yersinia enterocolitica O:9 and Brucella abortus in cattle. Vet Ital. (2019) 55:149–55. doi: 10.12834/VetIt.1374.7538.2
27. Pérez-Sancho, M, Durán-Ferrer, M, García-Seco, T, Macías, P, García, N, Martínez, I, et al. Interferon-gamma responses in sheep exposed to virulent and attenuated Brucella melitensis strains. Vet Immunol Immunopathol. (2014) 160:123–8. doi: 10.1016/j.vetimm.2014.03.014
28. Suraud, V, Jacques, I, Olivier, M, and Guilloteau, LA. Acute infection by conjunctival route with Brucella melitensis induces IgG+ cells and IFN-gamma producing cells in peripheral and mucosal lymph nodes in sheep. Microbes Infect. (2008) 10:1370–8. doi: 10.1016/j.micinf.2008.08.003
29. Grandoni, F, Signorelli, F, Martucciello, A, Napolitano, F, De Donato, I, Donniacuo, A, et al. In-depth immunophenotyping reveals significant alteration of lymphocytes in buffalo with brucellosis. Cytometry A. (2023) 103:528–36. doi: 10.1002/cyto.a.24710
30. Iwakura, Y, and Ishigame, H. The IL-23/IL-17 axis in inflammation. J Clin Invest. (2006) 116:1218–22. doi: 10.1172/JCI28508
31. Sofian, M, Ramezani, A, Mousavi, A, Banifazl, M, Cherei, S, Ali, C, et al. Interlukine-17 and TGF-ß levels in patients with acute brucellosisbefore and after treatment. Turk J Med Sci. (2016) 46:1348–52. doi: 10.3906/sag-1506-59
32. Mosser, DM, and Zhang, X. Interleukin-10: new perspectives on an old cytokine. Immunol Rev. (2008) 226:205–18. doi: 10.1111/j.1600-065X.2008.00706.x
33. Vignali, D, Collison, L, and Workman, C. How regulatory T cells work. Nat Rev Immunol. (2008) 8:523–32. doi: 10.1038/nri2343
34. Wang, Y, Li, Y, Li, H, Song, H, Zhai, N, Lou, L, et al. Brucella dysregulates monocytes and inhibits macrophage polarization through LC3-dependent autophagy. Front Immunol. (2017) 8:691. doi: 10.3389/fimmu.2017.00691
35. Weiss, G, and Schaible, UE. Macrophage defense mechanisms against intracellular bacteria. Immunol Rev. (2015) 264:182–203. doi: 10.1111/imr.12266
36. Kalliolias, GD, and Ivashkiv, LB. TNF biology, pathogenic mechanisms and emerging therapeutic strategies. Nat Rev Rheumatol. (2016) 12:49–62. doi: 10.1038/nrrheum.2015.169
37. Billard, E, Dornand, J, and Gross, A. Brucella suis prevents human dendritic cell maturation and antigen presentation through regulation of tumor necrosis factor alpha secretion. Infect Immun. (2007) 75:4980–9. doi: 10.1128/IAI.00637-07
38. Yang, J, Wang, Y, Hou, Y, Sun, M, Xia, T, and Wu, X. Evasion of host defense by Brucella. Cell Insight. (2024) 3:100143. doi: 10.1016/j.cellin.2023.100143
39. Comerford, I, and McColl, SR. Mini-review series: focus on chemokines. Immunol Cell Biol. (2011) 89:183–4. doi: 10.1038/icb.2010.164
40. Antonelli, A, Ferrari, SM, Giuggioli, D, Ferrannini, E, Ferri, C, and Fallahi, P. Chemokine (C-X-C motif) ligand (CXCL)10 in autoimmune diseases. Autoimmun Rev. (2014) 13:272–80. doi: 10.1016/j.autrev.2013.10.010
41. Bernitz, N, Kerr, TJ, Goosen, WJ, Clarke, C, Higgitt, R, Roos, EO, et al. Parallel measurement of IFN-γ and IP-10 in QuantiFERON®-TB gold (QFT) plasma improves the detection of Mycobacterium bovis infection in African buffaloes (Syncerus caffer). Prev Vet Med. (2019) 169:104700. doi: 10.1016/j.prevetmed.2019.104700
42. Goosen, WJ, Cooper, D, Miller, MA, van Helden, PD, and Parsons, SDC. IP-10 is a sensitive biomarker of antigen recognition in whole-blood stimulation assays used for the diagnosis of Mycobacterium bovis infection in African buffaloes (Syncerus caffer). Clin Vaccine Immunol. (2015) 22:974–8. doi: 10.1128/CVI.00324-15
43. Smith, K, Kleynhans, L, Warren, RM, Goosen, WJ, and Miller, MA. Cell-mediated immunological biomarkers and their diagnostic application in livestock and wildlife infected with Mycobacterium bovis. Front Immunol. (2021) 12:639605. doi: 10.3389/fimmu.2021.639605
44. Coad, M, Doyle, M, Steinbach, S, Gormley, E, Vordermeier, M, and Jones, G. Simultaneous measurement of antigen-induced CXCL10 and IFN-γ enhances test sensitivity for bovine TB detection in cattle. Vet Microbiol. (2019) 230:1–6. doi: 10.1016/j.vetmic.2019.01.007
45. Paranavitana, C, Zelazowska, E, Izadjoo, M, and Hoover, D. Interferon-g associated cytokines and chemokines produced by spleen cells from Brucella-immune mice. Cytokine. (2005) 30:86–92. doi: 10.1016/j.cyto.2004.12.009
46. Deshmane, SL, Kremlev, S, Amini, S, and Sawaya, BE. Monocyte chemoattractant protein-1 (MCP-1): an overview. J Interf Cytokine Res. (2009) 29:313–26. doi: 10.1089/jir.2008.0027
47. Menten, P, Wuyts, A, and Van Damme, J. Macrophage inflammatory protein-1. Cytokine Growth Factor Rev. (2002) 13:455–81. doi: 10.1016/s1359-6101(02)00045-x
48. Beste, MT, Lomakina, EB, Hammer, DA, and Waugh, RE. Immobilized IL-8 triggers phagocytosis and dynamic changes in membrane microtopology in human neutrophils. Ann Biomed Eng. (2015) 43:2207–19. doi: 10.1007/s10439-014-1242-y
49. Moreno, E, and Barquero-Calvo, E. The role of neutrophils in brucellosis. Microbiol Mol Biol Rev. (2020) 84:e00048–20. doi: 10.1128/MMBR.00048-20
50. Dornand, J, Gross, A, Lafont, V, Liautard, J, Oliaro, J, and Liautard, JP. The innate immune response against Brucella in humans. Vet Microbiol. (2002) 90:383–94. doi: 10.1016/s0378-1135(02)00223-7
51. Wang, Y, Yang, S, Han, B, Du, X, Sun, H, Du, Y, et al. Single-cell landscape revealed immune characteristics associated with disease phases in brucellosis patients. iMeta. (2024) 3:e226. doi: 10.1002/imt2.226
52. Garlanda, C, Dinarello, CA, and Mantovani, A. The interleukin-1 family: back to the future. Immunity. (2013) 39:1003–18. doi: 10.1016/j.immuni.2013.11.010
Keywords: Brucella, biomarkers, IFN-γ, cytokines, Mediterranean Buffaloes
Citation: Franzoni G, Signorelli F, Donniacuo A, Schiavo L, Napoletano M, De Matteis G, Grandoni F, Zinellu S, Bove V, Dei Giudici S, De Carlo E, Galiero G, Napolitano F and Martucciello A (2025) Exploring potential cytokine profiles as diagnostic biomarkers for brucellosis in Mediterranean Buffaloes. Front. Vet. Sci. 12:1583858. doi: 10.3389/fvets.2025.1583858
Edited by:
Camila Hamond, University of Connecticut, United StatesReviewed by:
Mirinda Van Kleef, Agricultural Research Council of South Africa (ARC-SA), South AfricaAman Ullah Khan, University of Veterinary and Animal Sciences, Pakistan
Copyright © 2025 Franzoni, Signorelli, Donniacuo, Schiavo, Napoletano, De Matteis, Grandoni, Zinellu, Bove, Dei Giudici, De Carlo, Galiero, Napolitano and Martucciello. This is an open-access article distributed under the terms of the Creative Commons Attribution License (CC BY). The use, distribution or reproduction in other forums is permitted, provided the original author(s) and the copyright owner(s) are credited and that the original publication in this journal is cited, in accordance with accepted academic practice. No use, distribution or reproduction is permitted which does not comply with these terms.
*Correspondence: Giulia Franzoni, Z2l1bGlhLmZyYW56b25pQGl6cy1zYXJkZWduYS5pdA==