- 1Department of Cosmosciences, Graduate School of Science, Hokkaido University, Sapporo, Japan
- 2Department of Economics, Management and Information Science, Onomichi City University, Onomichi, Japan
- 3Kavli Institute for the Physics and Mathematics of the Universe, Todai Institutes for Advanced Study, University of Tokyo, Kashiwa, Japan
- 4Max-Planck-Institut fur Astrophysik, Garching, Germany
- 5Institute of Management and Information Technologies, Chiba University, Chiba, Japan
- 6Research Center for Space and Cosmic Evolution, Ehime University, Matsuyama, Japan
- 7Faculty of Education, Bunkyo University, Koshigaya, Japan
- 8Faculty of Business Administration, Tokyo Keizai University, Kokubunji, Japan
- 9Faculty of Natural Sciences, National Institute of Technology, Kure College, Kure, Japan
We use a semi-analytic model of galaxy formation and investigate how the mass of a seed black hole affect the scaling relation between black hole mass and bulge mass at z ~ 0. When the mass of the seed is set at 105M⊙, we find that the model results become inconsistent with recent observational results of the scaling relation for dwarf galaxies. On the other hand, when we set seed black hole mass as 103M⊙ or as randomly chosen value within a range, we find the results are consistent with observational results including the dispersion. We also find that black hole mass—bulge mass relations for less massive bulges at z ~ 0 put stronger constraints on the seed BH mass than the relations at higher redshifts.
1. Introduction
Many observations (e.g., Kormendy and Richstone, 1995; Magorrian et al., 1998; Häring and Rix, 2004; McConnell and Ma, 2013) have suggested that the mass of supermassive black holes (MBH) correlates with the properties of their host galaxies such as stellar mass of bulges (Mbulge) at z ~ 0. This MBH – Mbulge relation might suggest that supermassive black holes (SMBHs) would have co-evolved with their host galaxies.
SMBHs grow to the current mass () from their initial mass. The initial mass and its distribution have been debating. Although, there are many theoretical suggestions of formation mechanism and mass of seed BHs (e.g., Begelman et al., 2006), we cannot obtain what is the dominant mechanism by comparing theoretical models with observations since seed BHs are not observable directly.
Here, we focus on the MBH – Mbulge relation for galaxies with bulge mass is less than to get the constraints on mass of seed BHs. This paper is a summary of Shirakata et al. (2016) in which we investigate the effect of the seed BHs' mass on model predictions of MBH – Mbulge relation at by using an semi-analytic model of galaxy formation (hereafter SA model). In section 2 we briefly review the SA model we used. Section 3 includes the main results. Finally, in section 4, we summarize this review and briefly mention future prospects.
2. Models
We use a revised version of an SA model, “New Numerical Galaxy Catalogue” (ν2GC ; Makiya et al., 2016, hereafter M16), where the models related to the SMBH and AGNs are described in Enoki et al. (2003), Enoki et al. (2014), and Shirakata et al. (2015). We consider star formation in galactic disk and bulge, mergers of galaxies, atomic gas cooling, gas heating by UV feedback and feedbacks via supernovae and AGNs, and the growth of SMBHs by coalescence and gas accretion from their host galaxies.
Merging histories of dark matter halos are calculated from state-of-the-art cosmological N-body simulations (Ishiyama et al., 2015). The cosmological simulations have a high mass resolution and large volume compared to previous simulations (e.g., mass resolution is roughly four times better than those of Millennium simulations, Springel et al., 2005). Here we employ a simulation with L = 70.0 [h−1 Mpc] of box size and 5123 particles, which corresponds to of minimum halo mass.
We assume a ΛCDM universe which have the following parameters: Ω0 = 0.31, λ0 = 0.69, Ωb = 0.048, σ8 = 0.83, ns = 0.96, and a Hubble constant of Mpc−1, where h = 0.68 (Planck Collaboration et al., 2014).
2.1. Setting of Seed Black Holes
We place a seed BH soon after the time of a galaxy formation. We present results with (hereafter “light seed model”) where MBH, seed is the seed BH mass, and (“massive seed model”). In addition, we employ the model in which MBH, seed takes uniformly random values in the logarithmic scale in the range of 3 ≤ log(MBH, seed/M⊙) ≤ 5 (hereafter “random seed model”).
2.2. Summary of Bulge and SMBH Growth Model
We assume that the bulge grows via starbursts and the migration of disk stars. Starbursts are triggered by mergers of galaxies (major and minor) or disk instability. The model of merger driven bulge formation in ν2GC is based on Hopkins et al. (2009). We consider that mergers of galaxies occur both by dynamical friction (central-satellite merger) and random collision (satellite-sattelite merger). We also introduce the spheroid formation by disk instability following Mo et al. (1998) and Cole et al. (2000). In both cases, the gas supplyed from galactic disk to the bulge is completely exhausted by a starburst and fueling onto their central SMBHs.
SMBHs in ν2GC are mainly grown by gas accretion from their host galaxy. When a starburst occurs in a bulge, a part of cold gas gets accreted by the SMBH :
where Macc is the cold gas mass accreted onto the SMBH, which is assumed to be proportional to the stellar mass formed by a current starburst, ΔM*, burst. Here we set fBH = 0.01. SMBHs also grow via coalescence of BHs which occurs with mergers of host galaxies. For simplicity, we assume BHs merge instantaneously when their host galaxies merge.
3. Results
Figure 1 shows the main result which depicts the MBH – Mbulge relation at z ~ 0 obtained from the model and observations. Each panels correspond to the results of massive seed model (top), random seed model (middle), and light seed model (bottom), respectively. Red solid lines represents the model result, blue and green points represents the observational data.
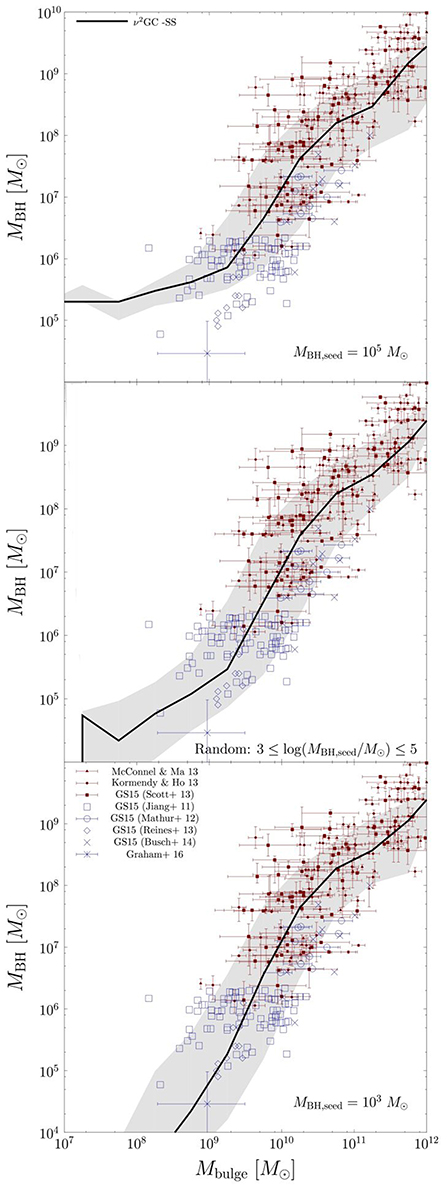
Figure 1. MBH – Mbulge relations at z ~ 0 for different MBH, seed; the massive (top), random (middle), and light (bottom) seed models. Black solid lines track the median, and shaded regions indicate 10–90 percentile of the models of the model result. Red filled symbols indicate observational results obtained from McConnell and Ma (2013), Kormendy and Ho (2013), and GS153(triangles, diamonds, and squares, respectively). Blue open symbols are AGN sample obtained from GS15, (see the text for more details). Blue asterisks correspond LEDA 87300 (Baldassare et al., 2015; Graham et al., 2016).
We find all of the models reproduce the relation at , while the massive seed model has an inconsistency in the observational results for less massive galaxies (). Random and light seed models, on the other hand, provide the consistent results in the range of , with observational estimates. We thus conclude that to explain recent observational data of the MBH – Mbulge relation at z ~ 0, seed BH mass should dominate with .
We note that since the number of samples of galaxies with (corresponds to ) are not sufficient. Observational data with the mass range are thus necessary to investigate the detailed mass distribution of the seed BHs. It is however difficult to estimate BH and bulge mass of less massive galaxies. We thus investigate whether the MBH – Mbulge relation at higher redshifts could be useful for getting further constraints on the mass of seed BHs. Figure 2 displays the ratio of the average BH masses in the light seed model (≡ 〈MBH〉3) and those in the massive seed model (≡ 〈MBH〉5), as a function of bulge masses. The difference in the seed mass significantly appears in galaxies with bulge mass below at z ~ 0, 1, and 2. We also find that the difference becomes smaller at higher redshift for a given Mbulge. Observations of less massive bulges at z ~ 0 would thus be more important than at higher redshifts for investigating the mass distribution of seed BHs.
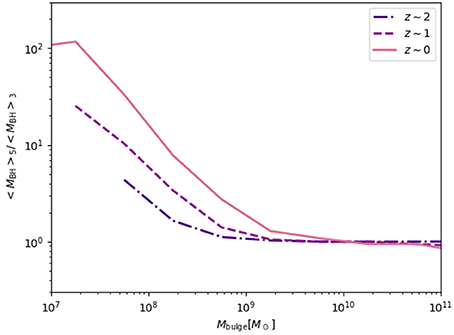
Figure 2. The difference of averaged SMBH mass due to the seed BH mass at z ~ 2 (dash-doted line in blue), z ~ 1 (dashed line in purple), and z ~ 0 (solid line in pink) as a function of their bulge stellar mass with the ν2GC -H2 simulation. The difference becomes smaller at higher redshift.
4. Summary and Future Prospects
We investigate how the mass of the seed BHs affects model predictions of the local MBH – Mbulge relation by using an SA model. The results suggest that seed BHs with as massive as should not be dominant for reproducing the observed MBH – Mbulge relation at z ~ 0 over a wide range of bulge masses down to . Obtaining stronger constraints of the detailed mass distribution of seed BHs observations of would be required.
We have shown results of the local MBH – Mbulge relations varying the mass of seed BHs. According to Shankar et al. (2016), Mbulge obtained from observations could be biased in favor of larger stellar masses. If so, we might have to use MBH – velocity dispersion relation instead of the local MBH – Mbulge. We leave it for future studies.
The spheroids formed through disk instability might be classified as so-called “pseudo bulges”. There are some debates whether pseudo bulges and classical bulges follow the same MBH – Mbulge relation (e.g., Kormendy and Ho, 2013). We might need the model of the properties of pseudo bulges in the near future.
Author Contributions
HS, RM, MN, ME, and MK have developed ν2GC. In addition, HS analyze the output data obtained from ν2GC. TK, TakO, and TaiO gave comments for the analysis. TI provides merger trees obtained from cosmological N-body simulations for ν2GC. YM gave fruitful comments from a standpoint of AGN observations.
Funding
TK was supported in part by an University Research Support Grant from the NAOJ and JSPS KAKENHI (17K05389). TakO was supported by JSPS Grant-in-Aid for Young Scientists (16H01085). RM was supported in part by MEXT KAKENHI (15H05896). TI was supported by MEXT HPCI STRATEGIC PROGRAM and MEXT/JSPS KAKENHI (15K12031) and by Yamada Science Foundation. MN was supported by the Grant-in-Aid (25287041 and 17H02867) from the MEXT of Japan.
Conflict of Interest Statement
The authors declare that the research was conducted in the absence of any commercial or financial relationships that could be construed as a potential conflict of interest.
Footnotes
3. ^Originally obtained from Scott et al. (2013).
References
Baldassare, V. F., Reines, A. E., Gallo, E., and Greene, J. E. (2015). A ~ 50,000 M⊙ solar mass black hole in the nucleus of RGG 118. Astrophys. J. Lett. 809:L14. doi: 10.1088/2041-8205/809/1/L14
Begelman, M. C., Volonteri, M., and Rees, M. J. (2006). Formation of supermassive black holes by direct collapse in pre-galactic haloes. Mon. Not. R. Astron. Soc. 370, 289–298. doi: 10.1111/j.1365-2966.2006.10467.x
Cole, S., Lacey, C. G., Baugh, C. M., and Frenk, C. S. (2000). Hierarchical galaxy formation. Mon. Not. R. Astron. Soc. 319, 168–204. doi: 10.1046/j.1365-8711.2000.03879.x
Enoki, M., Ishiyama, T., Kobayashi, M. A. R., and Nagashima, M. (2014). Anti-hierarchical evolution of the active galactic nucleus space density in a hierarchical universe. Astrophys. J. 794:69. doi: 10.1088/0004-637X/794/1/69
Enoki, M., Nagashima, M., and Gouda, N. (2003). Relations between galaxy formation and the environments of quasars. Publ. Astron. Soc. Jpn. 55, 133–142. doi: 10.1093/pasj/55.1.133
Graham, A. W., Ciambur, B. C., and Soria, R. (2016). Does the intermediate-mass black hole in LEDA 87300 (RGG 118) follow the near-quadratic MBH–MSpheroid relation? Astrophys. J. 818:172. doi: 10.3847/0004-637X/818/2/172
Graham, A. W., and Scott, N. (2015). The (black hole)-bulge mass scaling relation at low masses. Astrophys. J. 798:54. doi: 10.1088/0004-637X/798/1/54
Häring, N., and Rix, H.-W. (2004). On the black hole mass-bulge mass relation. Astrophys. J. Lett. 604, L89–L92. doi: 10.1086/383567
Hopkins, P. F., Cox, T. J., Younger, J. D., and Hernquist, L. (2009). How do disks survive mergers? Astrophys. J. 691, 1168–1201. doi: 10.1088/0004-637X/691/2/1168
Ishiyama, T., Enoki, M., Kobayashi, M. A. R., Makiya, R., Nagashima, M., and Oogi, T. (2015). The ν2GC simulations: quantifying the dark side of the universe in the Planck cosmology. Publ. Astron. Soc. Jpn. 67:61. doi: 10.1093/pasj/psv021
Kormendy, J., and Ho, L. C. (2013). Coevolution (or not) of supermassive black holes and host galaxies. Annu. Rev. Astron. Astrophys. 51, 511–653. doi: 10.1146/annurev-astro-082708-101811
Kormendy, J., and Richstone, D. (1995). Inward bound—the search for supermassive black holes in galactic nuclei. Annu. Rev. Astron. Astrophys. 33:581.
Magorrian, J., Tremaine, S., Richstone, D., Bender, R., Bower, G., Dressler, A., et al. (1998). The demography of massive dark objects in galaxy centers. Astron. J. 115, 2285–2305.
Makiya, R., Enoki, M., Ishiyama, T., Kobayashi, M. A. R., Nagashima, M., Okamoto, T., et al. (2016). The new numerical galaxy catalog (ν2GC): an updated semi-analytic model of galaxy and active galactic nucleus formation with large cosmological N-body simulations. Publ. Astron. Soc. Jpn. 68:25. doi: 10.1093/pasj/psw005
McConnell, N. J., and Ma, C.-P. (2013). Revisiting the scaling relations of black hole masses and host galaxy properties. Astrophys. J. 764:184. doi: 10.1088/0004-637X/764/2/184
Mo, H. J., Mao, S., and White, S. D. M. (1998). The formation of galactic discs. Mon. Not. R. Astron. Soc. 295, 319–336. doi: 10.1046/j.1365-8711.1998.01227.x
Ade, P. A. R., Aghanim, N., Alves, M. I. R., Armitage-Caplan, C., Arnaud, M., et al. (2014). Planck 2013 results. I. Overview of products and scientific results. Astron. Astrophys. 571:A1. doi: 10.1051/0004-6361/201321529
Scott, N., Graham, A. W., and Schombert, J. (2013). The supermassive black hole mass-spheroid stellar mass relation for sérsic and core-Sérsic galaxies. Astrophys. J. 768:76. doi: 10.1088/0004-637X/768/1/76
Shankar, F., Bernardi, M., Sheth, R. K., Ferrarese, L., Graham, A. W., Savorgnan, G., et al. (2016). Selection bias in dynamically-measured super-massive black holes: its consequences and the quest for the most fundamental relation. Mon. Not. R. Astron. Soc. arXiv:1603.01276.
Shirakata, H., Kawaguchi, T., Okamoto, T., Makiya, R., Ishiyama, T., Matsuoka, Y., et al. (2016). Theoretical re-evaluations of the black hole mass-bulge mass relation - I. Effect of seed black hole mass. Mon. Not. R. Astron. Soc. 461, 4389–4394. doi: 10.1093/mnras/stw1798
Shirakata, H., Okamoto, T., Enoki, M., Nagashima, M., Kobayashi, M. A. R., Ishiyama, T., et al. (2015). The impact of dust in host galaxies on quasar luminosity functions. Mon. Not. R. Astron. Soc. 450, L6–L10. doi: 10.1093/mnrasl/slv035
Keywords: galaxies, active galactic nuclei, bulge, galaxy formation, statistics
Citation: Shirakata H, Kawaguchi T, Okamoto T, Makiya R, Ishiyama T, Matsuoka Y, Nagashima M, Enoki M, Oogi T and Kobayashi MAR (2017) Theoretical Re-evaluations of Scaling Relations between SMBHs and Their Host Galaxies—1. Effect of Seed BH Mass. Front. Astron. Space Sci. 4:15. doi: 10.3389/fspas.2017.00015
Received: 10 July 2017; Accepted: 08 September 2017;
Published: 21 September 2017.
Edited by:
Mauro D'Onofrio, University of Padua, ItalyReviewed by:
Jaime Perea, Instituto de Astrofísica de Andalucía (CSIC), SpainVyacheslav Ivanovich Dokuchaev, Institute for Nuclear Research (RAS), Russia
Copyright © 2017 Shirakata, Kawaguchi, Okamoto, Makiya, Ishiyama, Matsuoka, Nagashima, Enoki, Oogi and Kobayashi. This is an open-access article distributed under the terms of the Creative Commons Attribution License (CC BY). The use, distribution or reproduction in other forums is permitted, provided the original author(s) or licensor are credited and that the original publication in this journal is cited, in accordance with accepted academic practice. No use, distribution or reproduction is permitted which does not comply with these terms.
*Correspondence: Hikari Shirakata, c2hpcmFrYXRhQGFzdHJvMS5zY2kuaG9rdWRhaS5hYy5qcA==