- 1X-ray Astrophysics Laboratory, NASA Goddard Space Flight Center, Greenbelt, MD, United States
- 2NASA Postdoctoral Program Fellow, Oak Ridge Associated Universities, NASA NPP Program, Oak Ridge, TN, United States
- 3Cahill Center for Astrophysics, California Institute of Technology, Pasadena, CA, United States
- 4Max Planck Institute for Extraterrestrial Physics, Garching, Germany
- 5Jet Propulsion Laboratory, California Institute of Technology, Pasadena, CA, United States
- 6Department of Physics and Astronomy, Clemson University, Clemson, SC, United States
- 7INAF—Osservatorio Astrofisico di Arcetri, Firenze, Italy
- 8Instituto de Estudios Astrofísicos, Facultad de Ingeniería y Ciencias, Universidad Diego Portales, Santiago, Chile
- 9Kavli Institute for Astronomy and Astrophysics, Peking University, Beijing, China
- 10George Mason University, Department of Physics and Astronomy, MS3F3, Fairfax, VA, United States
- 11Dipartimento di Fisica e Astronomia (DIFA) Augusto Righi, Università di Bologna, Firenze, Italy
- 12INAF—Osservatorio di Astrofisico e Scienza dello Spazio (OAS), Bologna, Italy
- 13Center for Relativistic Astrophysics, School of Physics, George Institute of Technology, Atlanta, GA, United States
- 14Department of Physics, University of Miami, Coral Gables, FL, United States
- 15Science and Technology Institute, Universities Space Research Association, Huntsville, AL, United States
- 16Astrophysics Office, NASA Marshall Space Flight Center, Huntsville, AL, United States
- 17UMR5277 Institut de Recherche en Astrophysique et Planétologie (IRAP), CNRS, UPS, CNES, Université de Toulouse, Toulouse, France
- 18Dipartimento di Matematica e Fisica, Università Roma Tre, Rome, Italy
- 19Department of Physics and Astronomy, Dartmouth College, Hanover, NH, United States
- 20CITA National Fellow, University of Manitoba, Winnipeg, MB, Canada
- 21Canadian Institute for Theoretical Astrophysics, University of Toronto, Toronto, ON, Canada
A fundamental goal of modern-day astrophysics is to understand the connection between supermassive black hole (SMBH) growth and galaxy evolution. Merging galaxies offer one of the most dramatic channels for galaxy evolution known, capable of driving inflows of gas into galactic nuclei, potentially fueling both star formation and central SMBH activity. Dual active galactic nuclei (dual AGNs) in late-stage mergers with nuclear pair separations
1 Introduction
It has long been known that galaxy interactions and mergers give rise to gravitational torques that can transform the structure and composition of the constituent galaxies (e.g., Toomre and Toomre, 1972). These torques can funnel vast quantities of gas into the central nuclei of the galaxies (e.g., Barnes and Hernquist, 1991; Barnes and Hernquist, 1996), creating reservoirs that can then fuel star formation as well as the central supermassive black holes (SMBHs), which ignite as active galactic nuclei (AGNs) (Hopkins et al., 2006; Hopkins et al., 2008). A natural product of galaxy mergers are dual AGNs, that is, synchronized SMBH growth in both galaxies (Van Wassenhove et al., 2012). Simulations of galaxy mergers predict that the growth of the central SMBHs is better synchronized in the latest stages of the merger sequence, when the central nuclei are separated by ≲ 10 kpc (Van Wassenhove et al., 2012; Capelo et al., 2015; Blecha et al., 2018), though dual AGNs can be found across the full merger sequence, from separations below 1 kpc (e.g., Komossa et al., 2003; Koss et al., 2023) and up to
Despite this presumed importance, dual AGNs have remained relatively elusive, and a variety of selection strategies have been pursued to uncover them: optical spectroscopic emission line ratios Liu et al. (2011), double-peaked emission lines (e.g., Zhou et al., 2004; Wang et al., 2009; Liu et al., 2010; Smith et al., 2010; Comerford et al., 2011; Comerford et al., 2012; Ge et al., 2012), infrared colors (Imanishi and Saito, 2014; Satyapal et al., 2017; Ellison et al., 2017; Pfeifle et al., 2019b; Pfeifle et al., 2019a; Imanishi et al., 2020; Barrows et al., 2023), hard X-ray selection (Koss et al., 2012), and most recently varstrometry (Hwang et al., 2020; Shen et al., 2021; Schwartzman et al., 2023) and Gaia multi-source strategies (Shen et al., 2019; Mannucci et al., 2022; Ciurlo et al., 2023). Serendipitous (e.g., Komossa et al., 2003; Guainazzi et al., 2005; Piconcelli et al., 2010) and follow-up X-ray observations (Bianchi et al., 2008; Comerford et al., 2011; Koss et al., 2012; Liu et al., 2013; Comerford et al., 2015; Satyapal et al., 2017; Pfeifle et al., 2019b; Pfeifle et al., 2019a; Hou et al., 2019; Gross et al., 2019; Hou et al., 2020; Hou et al., 2023) are a common method by which dual AGNs are confirmed, particularly in cases where dual AGNs cannot be selected through common optical diagnostics (e.g., NGC 6240, Komossa et al., 2003). These investigations have revealed a prevalence of high column densities in the known population of dual AGNs (e.g., Komossa et al., 2003; Bianchi et al., 2008; Piconcelli et al., 2010; Ptak et al., 2015; Nardini, 2017; De Rosa et al., 2018; Pfeifle et al., 2019b; Pfeifle et al., 2019a; De Rosa et al., 2023); see discussion in Pfeifle et al. (2023). Radio imaging is also an effective method of selecting (Fu et al., 2015a) as well as confirming (e.g., Fu et al., 2011; Frey et al., 2012; Fu et al., 2015b; Müller-Sánchez et al., 2015; Rubinur et al., 2019; Rubinur et al., 2021; Gross et al., 2023) or rejecting dual AGN candidates (e.g., Gabányi et al., 2014; Gabányi et al., 2016; Fu et al., 2015b; Müller-Sánchez et al., 2015; Rubinur et al., 2019). Radio emission is insensitive to the line-of-sight obscuration and therefore radio observations are not biased against the detection of heavily obscured dual AGNs, though confirmation and characterization via radio imaging is not always unambiguous (e.g., Müller-Sánchez et al., 2015; Veres et al., 2021) and does require that one or both AGNs be emitting in the radio, which is not always the case (e.g., Gabányi et al., 2016).
While Chandra and XMM-Newton have been used effectively in the past to identify, confirm, and/or characterize dual AGN systems, their soft X-ray bandpasses (0.5–10 keV) bias them against detecting and properly characterizing the X-ray spectral properties and the circumnuclear obscurers in heavily obscured dual AGNs with column densities NH > 5 × 1023–1024 cm−2, as predicted (Hopkins et al., 2008; Capelo et al., 2015; Capelo et al., 2017; Blecha et al., 2018) and observed (e.g., Komossa et al., 2003; Ballo et al., 2004; Bianchi et al., 2008; Mazzarella et al., 2012; Koss et al., 2016; De Rosa et al., 2018; Iwasawa et al., 2018; Pfeifle et al., 2019a; Iwasawa et al., 2020; Guainazzi et al., 2021; De Rosa et al., 2023), particularly in late-stage mergers (e.g., Ricci et al., 2017; Ricci et al., 2021). In fact, this is a particular concern in LIRGs and ULIRGs; though the statistics are small, a higher fraction of dual AGNs were identified among the lower IR luminosity GOALS sample (Torres-Albà et al., 2018) than the brighter IR luminosity sample (Iwasawa et al., 2011), which can possibly be interpreted as the dual AGNs in the high luminosity sample being too obscured for XMM-Newton or Chandra to detect and too close together to be resolved by NuSTAR (Torres-Albà et al., 2018). Hard X-ray bandpasses (
In this paper, we discuss the feasibility of hard X-ray dual AGN science with the High Energy X-ray Probe (HEX-P) mission concept. The current mission design for HEX-P is described in Section 2. In Section 3 we describe our HEX-P imaging and spectroscopic simulations, generated using the soxs (ZuHone et al., 2023) and sixte (Dauser et al., 2019) software packages. We outline the potential for resolving hard and soft X-ray signatures from dual AGNs with HEX-P using the instrument and facility design described in Section 4.1, the feasibility of using nested sampling and Bayesian statistical source detection techniques to probe closely-separated hard X-ray sources in Section 4.1, and we compare these results to what is currently possible with NuSTAR in Section 4.2. In Section 5, we examine simulated hard X-ray imaging and spectra for the dual AGN in Arp 299 as a test case for HEX-P dual AGN science, wherein we use physically-motivated obscuration models and Bayesian fitting techniques to constrain the line-of-sight column densities, photon indices, and other spectroscopic parameters of the AGNs. We discuss the issue of spectral contamination for closely separated sources in Section 6. In Section 7 we briefly outline synergies with upcoming ground-based and space-based facilities. We provide concluding remarks in Section 8.
2 Mission design
The HEX-P (Madsen et al., 2023) is a probe-class mission concept that offers sensitive broad-band spectral coverage (0.2–80 keV) with exceptional spectral, timing, and angular capabilities (see Madsen et al., 2023, for further details). It features two high-energy telescopes (HETs) that focus hard X-rays, and one low-energy telescope (LET) that focuses lower energy X-rays.
The LET consists of a segmented mirror assembly coated with Ir on monocrystalline silicon that achieves a half power diameter (HPD) of 3.5″, and a low-energy DEPFET detector, of the same type as the Wide Field Imager (WFI; Meidinger et al., 2020) onboard Athena (Nandra et al., 2013). It has 512 × 512 pixels that cover a field of view of 11.3′ x 11.3′. It has an effective passband of 0.2–25 keV, and a full frame readout time of 2 ms, which can be operated in a 128 and 64 channel window mode for higher count-rates to mitigate pile-up and faster readout. Pile-up effects remain below an acceptable limit of
The HET consists of two co-aligned telescopes and detector modules. The optics are made of Ni-electroformed full shell mirror substrates, leveraging the heritage of XMM-Newton (Jansen et al., 2001), and coated with Pt/C and W/Si multilayers for an effective passband of 2–80 keV. The high-energy detectors are of the same type as flown on NuSTAR (Harrison et al., 2013), and they consist of 16 CZT sensors per focal plane, tiled 4 × 4, for a total of 128 × 128 pixel spanning a field of view slightly larger than for the LET, 13.4′x13.4′.
The broad X-ray passband and superior sensitivity (minimum detectable 10–20 keV flux F10–20 keV = 1.4 × 10−15 erg cm−2 s−1 in 1 Ms; see Table 2 in Madsen et al., 2023) will provide a unique opportunity to study dual AGNs and galaxy mergers across a wide range of energies, luminosity ratios, pair separations, and dynamical regimes.
All the simulations presented here were produced with a set of response files that represent the observatory performance based on current best estimates as of Spring 2023 (see Madsen et al., 2023). The effective area is derived from ray-tracing calculations for the mirror design including obscuration by all known structures. The detector responses are based on simulations performed by the respective hardware groups, with an optical blocking filter for the LET and a Be window and thermal insulation for the HET. The LET background was derived from a GEANT4 simulation (Eraerds et al., 2021) of the WFI instrument, and the HET backgrounds was derived from a GEANT4 simulation of the NuSTAR instrument. Both simulations adopt the L1 orbit for HEX-P.
3 Simulating dual AGNs using SOXS and SIXTE
To simulate HEX-P observations of dual AGNs, we rely upon two software suites: 1) the Simulated Observations of X-ray Sources (soxs, ZuHone et al., 2023) suite, to create the SIMulated inPUT (SIMPUT) files that store the spatial and spectral models used for the simulations, and 2) the Simulation of X-ray Telescopes (sixte, Dauser et al. (2019)) suite, which was used to generate the actual simulated observations.
Each simulation includes two AGNs represented as point source spatial models (hereafter AGN 1 and AGN 2), and we produced simulations for a variety of separations, ranging from 50″ down to 20″ in increments of 10″ as well as from 20″ down to 0″ in increments of 2″. Here, the 0″ case represents the “null” expectation of what a single source would look like at the combined flux level of the AGNs in our simulations and it is included for visual comparison purposes. For illustrative purposes in this work, we also include simulations of AGNs separated by 15″, 5″, 3″, and 1″. We use the following components to develop the spectral models for the AGNs: 1) an absorbed power law (Γ = 1.8) that accounts for photoelectric absorption and Compton scattering; 2) reprocessed emission from a torus (borus, Baloković et al. (2018), which self consistently accounts for absorption, fluorescence, and Compton scattering for a toroidal distribution of obscuring gas); 3) two soft X-ray thermal components (apec) to model emission due to star formation, motivated by the common presence of soft thermal components in many dual AGNs and candidate systems (see, e.g., Section 5.4 in Pfeifle et al. (2023)); 4) a power law to model Thomson scattering (0.5%). This AGN spectroscopic model is described in xspec as:
Each AGN spectrum is simulated at a redshift of z = 0.05 (DL = 222.3 Mpc). We assume an approximately edge-on torus viewing angle of θview = 70° and a covering factor of C = 85%; the former assumption was a conservative choice that satisfied our fundamental requirement that the sight line be obscured but not grazing the torus, while the latter assumption is motivated by the expectation that mergers should result in heavily obscured AGNs with high covering factors (Ricci et al., 2017). AGNs 1 and 2 have observed (uncorrected for intrinsic absorption) 2–10 keV fluxes normalized to 1.0 × 10−13 and 5.0 × 10−14 erg cm−2 s−1, respectively. For each choice of separation, we run the spectral model of each AGN over a small list of line-of-sight column densities, log (NH/cm2) = 22, 23, 24 (for simplicity we assume the average torus column density and the line-of-sight column density are equal); we therefore end up with nine SIMPUT files for each separation, where each SIMPUT file probes a separate pairing in terms of obscuration; i.e., one Compton-thick pair (NH,1 and 2 = 1024 cm−2), four Compton-thick and Compton-thin pairs (NH,1 or 2 = 1024 cm−2 and NH,1 or 2 = 1023 cm−2, NH,1 or 2 = 1024 cm−2 and NH,1 or 2 = 1022 cm−2), and four Compton-thin-Compton-thin pairs (NH,1 and 2 = 1023 cm−2, NH,1 or 2 = 1022 cm−2 and NH,1 or 2 = 1023 cm−2, NH,1 and 2 = 1022 cm−2). soxs is then used to convolve the spatial and spectral models to produce the SIMPUT files for each AGN pairing.
Each SIMPUT file generated above was fed to sixte in order to generate the HEX-P HET and LET event files. For each AGN pairing, we assumed a 50 ks exposure (which could be the typical exposure time in the HEX-P wide area survey, and is also similar in length to archival NuSTAR observations of dual AGNs, i.e.,
4 Hard X-ray imaging results for dual AGNs
4.1 HEX-P HET and LET imaging of dual AGNs
We show a subset of our dual AGN simulation suite in Figure 1 and Figure 2 for the 0.2–25 keV LET and (co-added two-camera) 2–80 keV HET imaging, respectively, with each panel representing a distinct pair separation, ranging from 50″ down to 0″ in 10″ or 5″ intervals. AGN 1 (centered in the field of view) is clearly seen in the center of each panel, while AGN 2 can be seen moving closer in from the left with each successive panel. These images clearly show the two AGNs are distinguishable by eye down to ∼5″ in both the HET and LET; we include a finer grid of separations in Figure 9 with separations ranging from 5″ down to 1″ in 1″ intervals, which demonstrates that the AGNs can be distinguished by eye even down to
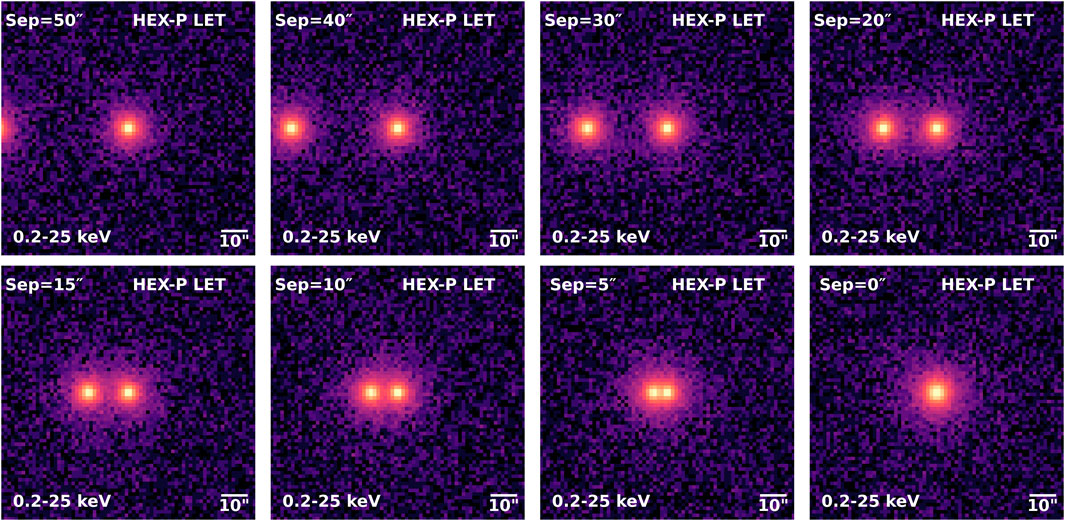
Figure 1. This 8-panel figure shows the LET 0.2–25 keV 50 ks images (1-pixel smoothing). Panels represent angular separations ranging from 50″ down to 0″. The two AGNs are distinguishable by eye down to 5″ and can be identified using Bayesian detection methods (see Section 4.1) down to separations of
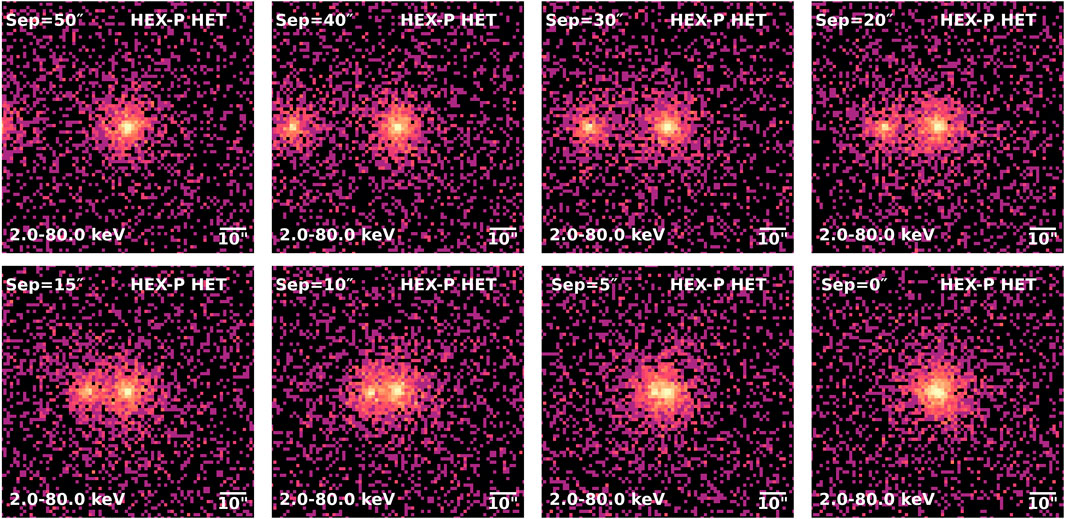
Figure 2. This 8-panel figure shows the effective 2-camera HET 2–80 keV, 50 ks images (1-pixel smoothing). As in Figure 1, panels represent angular separations ranging from 50″ down to 0″. The two AGNs are distinguishable by eye down to 5″ and can be identified using Bayesian detection methods (see Section 4.1 down to separations of
Probing dual AGNs with HEX-P down to separations ≲ 5″ is a particularly important task, as dual AGNs are predicted to experience the peak of their merger-driven luminosities and obscuration in late-stage mergers (Capelo et al., 2015; Blecha et al., 2018) when the nuclei of the galaxies are separated by ≲ 10 kpc (≲ 10.2″ at z = 0.05). HEX-P’s access to X-ray energies beyond 10 keV will be vital to constraining the intrinsic luminosities and distinct column densities along the line-of-sight to each AGN in a merging system, an impossibility with NuSTAR for all but the nearest and brightest (Nardini, 2017) and/or most widely-separated dual AGNs known (e.g., Ptak et al. (2015); Kosec et al. (2017); Oda et al. (2018)). Furthermore, broadband coverage is fundamental not only to detect obscured AGN, but also to accurately characterize the properties of their obscurers, including line-of-sight column densities, covering factors, and obscurer geometries; broadband hard X-ray coverage with NuSTAR, for example, has led to dramatic refinements in AGN column density estimates compared to constraints derived from only soft X-ray or joint soft and ultra-hard X-ray coverage (e.g., Marchesi et al. (2018); Marchesi et al. (2019). Here we discuss the possibility of probing dual AGNs with separations 1″< rp < 5″ in the hard X-rays with HEX-P.
We analyse each simulated event file with a single and double point source model, with wide, uninformative priors on the location and the total counts of each point source, I. The first point source is assumed to be located near the image centre ±10px. Both models also include a background count rate, B, that is constant across the image and left free during the fit. A standard Poisson likelihood is used, with the symmetric PSF model PSF(Δr) extracted from simulations:
For the single point source model,
while for the two point source model there are two centres and intensities.
The Akaike Information Criterion (AIC; Akaike, 1974) of the two models is compared to determine whether the single or double source model is preferred. In order for this comparison to take place, we first calibrated our detection algorithm using simulations of convolved X-ray sources, where we simulated two co-spatial X-ray sources with identical fluxes to those in our AGN simulations described in Section 3. We simulated 100 cases of convolved sources for the LET as well as for the HET, ran the detection algorithm on each case, and recorded the resulting AIC values. This calibration set the baseline for an acceptable AIC threshold to differentiate between a single and dual source case. We are able to detect X-ray point sources in both the LET 0.2–25 keV bandpass and the HET 2–80 keV bandpass down to separations of 2″. This result comes with the caveat that this detection routine is a proof-of-concept and the results are subject to change based on the exposure time and the fluxes of the sources: all else being equal, we cannot necessarily differentiate between multiple sources at 2″ or 3″ separations for lower exposure times; alternatively, we can probe down to closer pair separations
While dual AGNs are predicted to be heavily obscured in late-stage mergers (Blecha et al., 2018), there are cases of heavily obscured dual AGNs in widely-separated merging pairs of galaxies (Koss et al., 2012; De Rosa et al., 2018; De Rosa et al., 2023). Given that dual AGNs are also predicted to become heavily obscured after only the second pericenter passage (Blecha et al., 2018), and that AGNs are expected to flicker on and off across the merger sequence (e.g., the AGN duty cycle ≪ the merger timescale Schawinski et al., 2015; Goulding et al., 2019), HEX-P may reveal multi-modal distributions of heavily obscured AGNs across the merger sequence, rather than only during the latest stages of the merger sequence.
4.2 A substantial improvement over NuSTAR
In an analogous fashion to the HEX-P simulated event files displayed in Figures 1, 2, we have used identical input models, exposure times, and separations to develop NuSTAR dual AGN imaging that incorporates the NuSTAR background derived from the COSMOS field (Civano et al., 2015). Figure 3 displays these simulated NuSTAR images (where the color scale matches that of the HET imaging in Figure 2), in which the positions of the AGNs are denoted with black crosses; AGN 1 can be seen in a few (but not all) of the panels above the background while AGN 2 is virtually imperceptible in almost every panel. This figure clearly illustrates that - at these realistic observed 2–10 keV flux levels of 1 × 10−13 and 5 × 10−14 erg cm−2 s−1—NuSTAR cannot reliably identify dual AGNs in these pairings; it is important to note that dual AGNs separated by
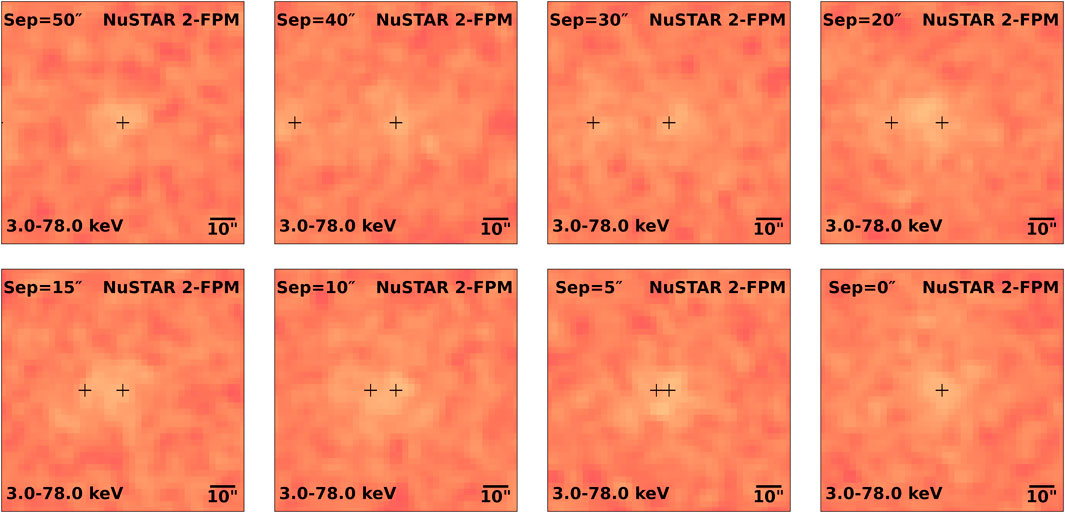
Figure 3. This 8-panel figure shows simulated two-camera 3–78 keV FPMA + B 50 ks imaging with NuSTAR (smoothed by 1-pixel) for the same suite of dual AGNs described in Section 3. The suite of AGNs range in separation from 50″ down to 0″; the scale bar in the bottom right corner of each panel indicates an angular scale of 10″. These panels use the same scaling as in Figure 1 for the HEX-P HET 2–80 keV imaging. The primary AGN can be seen above the background in some panels, but the weaker secondary AGN is essentially imperceptible above the background in all of the panels. Black markers are included to denote the positions of the two AGNs.
4.3 A substantial improvement over soft X-ray missions
In Figure 4 we display a simulated 50 ks Chandra 2–8 keV image alongside the 2–25 keV LET and 2–80 keV HET imaging for the case where the AGNs are separated by 10″. While Chandra can clearly resolve two X-ray cores emitted by the AGNs at
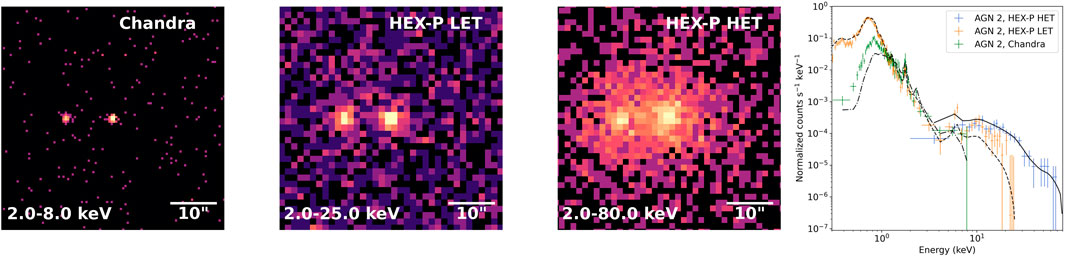
Figure 4. This four-panel figure shows a comparison between simulated Chandra, HEX-P LET, and HEX-P HET imaging and spectroscopy. The three X-ray imaging panels show the Chandra 2–8 keV, HEX-P LET 2–25 keV, and HEX-P HET 2–180 keV energy bands. The fourth panel displays the extracted Chandra (green), HEX-P LET (orange), and HET (blue) spectra for AGN 2 (the weaker of the two AGNs, see Section 3), binned at 3σ. The black solid, dashed, and dash-dotted lines show the result of fitting a simple phenomenological power law model to the HEX-P HET, LET, and Chandra spectra, respectively.
To compare the spectroscopic capabilities of HEX-P to Chandra, we extracted the spectrum of the weaker of the two AGNs (AGN 2) from the Chandra and HEX-P images using a 3″ aperture for the Chandra image, a 3.5″ aperture for LET, and a 3.5″ aperture in HET; these apertures represent the
4.4 Hard X-ray dual AGN science as a function of redshift
Current hard X-ray facilities (e.g., NuSTAR) limit the study of distinct AGNs within dual AGN pairings to only the nearest, brightest, and/or more widely-separated systems. Here we illustrate the imaging capabilities of HEX-P as a function of redshift for different pair separations. Figure 5 shows the distribution of angular separations for optical dual AGN candidates from Liu et al. (2011) as a function of redshift out to z ∼ 0.3. The overlaid black dash-dotted line indicates the angular resolution of NuSTAR (18″ FWHM, 58″ HPD, Harrison et al., 2013), while the red dashed, dash-dotted, and dotted curves indicate nuclear pair separations of 30, 20, and 10 kpc. Overlaid on this figure, we have also plotted the corresponding HEX-P 2–10 keV luminosity limits (vertical dotted, black lines) as a function of redshift, informed by the limiting flux of 3.8 × 10−14 erg cm−2 s−1 for a 50 ks exposure; the vast majority of dual AGNs have 2–10 keV luminosities ≲ 1043 erg s−1. Therefore, z ≈ 0.3 is an approximate redshift limit for dual AGN studies with HEX-P. Even late-stage mergers (≲ 10 kpc) become virtually inaccessible to NuSTAR already by z ≈ 0.025, and more widely separated pairs (
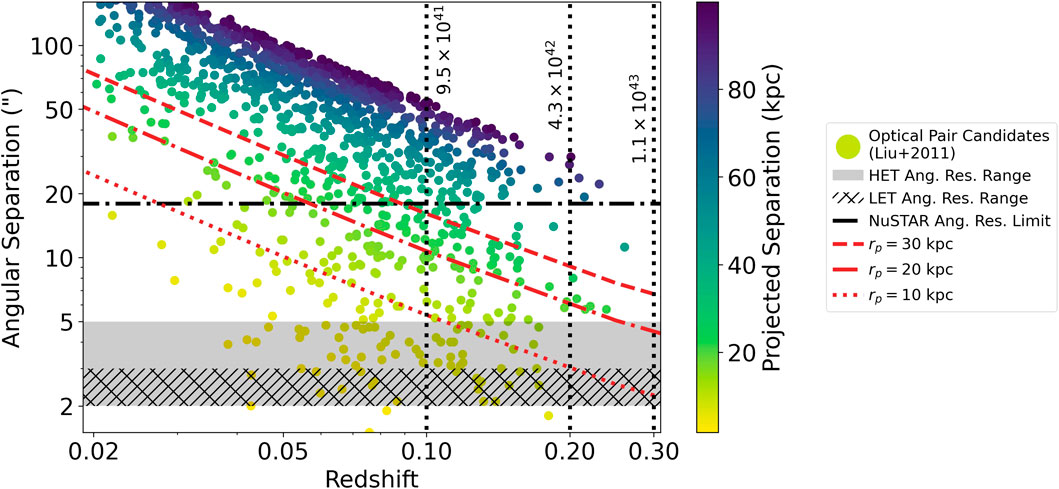
Figure 5. Redshift vs. angular separation. We have plotted the sample of optical dual AGN candidates from Liu et al. (2011) at z <0.3. Markers are color coded according to the projected separation of the pair (given on the auxiliary axis). Red dashed, dash dotted, and dotted lines indicate projected separations of 30, 20, and 10 kpc. The grey, shaded region indicates the HET angular resolution limits when examining sources visually by eye
5 A test case for HEX-P: Arp 299
One illustrative case study for examining the effectiveness of HEX-P over NuSTAR, in terms of spectroscopic and imaging constraints, is Arp 299, a local (44 Mpc, or z ∼ 0.01) dual AGN comprising an X-ray bright and heavily obscured AGN in the southwest nucleus (Arp 299-B, detected by Chandra and NuSTAR, Ballo et al. (2004); Ptak et al. (2015)) and an AGN in the northeast nucleus (Arp 299-A) identified as a flat spectrum radio source (Pérez-Torres et al., 2010), and tentatively identified via mid-IR SEDs (Alonso-Herrero et al., 2013) and ionized Fe Kα emission (Ballo et al., 2004). As reported by Ptak et al. (2015), Arp 299-B is detected by NuSTAR with a hard X-ray flux of F10−30 keV = 3.5 × 10−12 erg cm−2 s−1, while Arp 299-A is dominated by X-ray binaries below 10 keV and contains no hard X-ray emitting AGN above a 10–30 keV limit of
Our input models are informed by the model choices and spectral fitting results in Ptak et al. (2015). Specifically, we adopt the following model to describe the spectrum of each nucleus:
where we have used the borus02 model (Baloković et al., 2018) rather than MYTorus, which was used by Ptak et al. (2015). This model incorporates host galaxy absorption (tbabs), reflected emission (borus02), an absorbed intrinsic power law that accounts for photoelectric absorption and Compton scattering, and a cut-off power law to account for soft X-ray emission from X-ray binaries. For Arp 299-B, we assume Γ = 1.95, NH = 4 × 1024 cm−2 (for simplicity we assume the average torus column density and the line-of-sight column density are equal), covering factor cos (θopen) = 0.85, and we normalize the 10–30 keV emission to 3.5 × 10−12 erg cm−2 s−1. For Arp 299-A, we assume Γ = 1.8, NH = 5 × 1022 cm−2 (since it is likely an unobscured, low luminosity AGN, e.g., Ptak et al. (2015)), and covering factor cos (θopen) = 0.5; while (Ptak et al., 2015) placed an upper limit of
We show the simulated HEX-P LET and HET images in Figure 6 juxtaposed with the tricolor optical ugz imaging from the Dark Energy Camera Legacy Survey (DeCaLs) Legacy Viewer3 and simulated NuSTAR FPM imaging. Each row in Figure 6 represents a distinct energy band: 2–10 keV (top row), 10–25 keV (middle row), and 25–80 keV (bottom row; the NuSTAR imaging corresponds to 25–78 keV here). At 21″ separation, the soft (
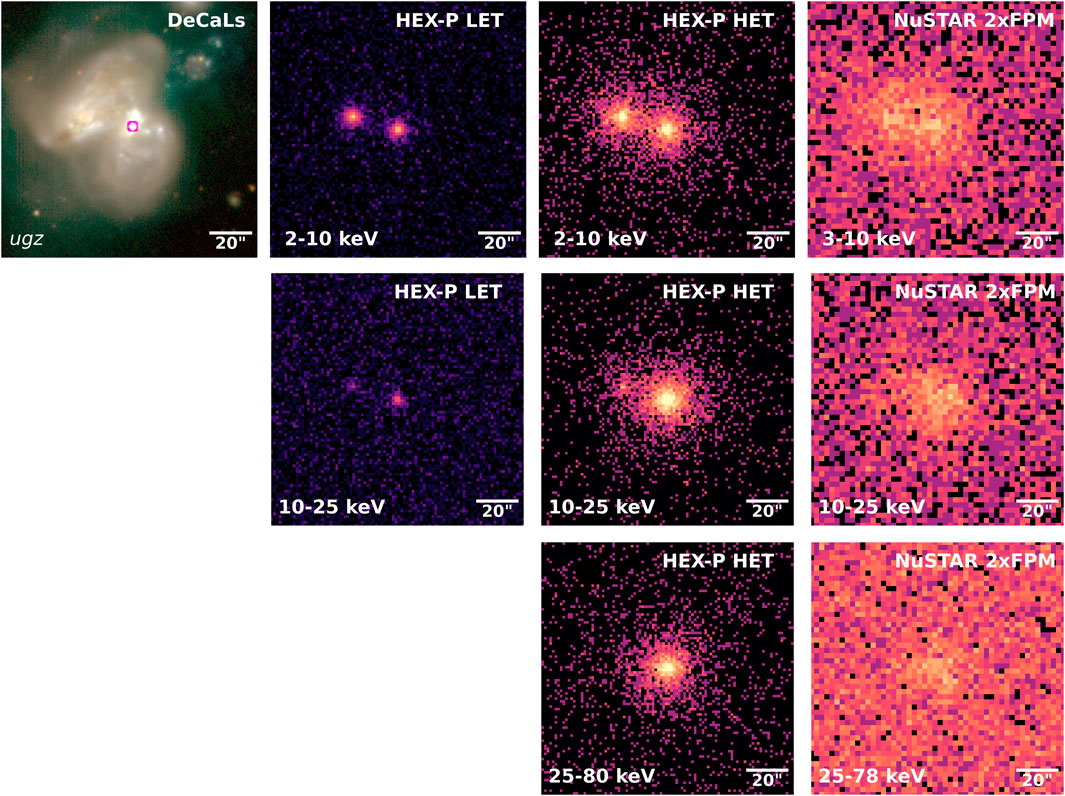
Figure 6. The Arp 299 case study: optical ugz imaging from DeCaLs (left column), simulated LET and HET (2-camera) HEX-P imaging (middle two columns), and simulated 3–78 keV NuSTAR imaging (right column) of Arp 299. The energy band or filter is indicated in the bottom left corner of each panel, while the facility or image source resides in the top right corner of each panel. Each row indicates a distinct energy band: the top, middle, and bottom row corresponds to 2–10 keV, 10–25 keV, and 25–80 keV (or 25–78 keV for NuSTAR), respectively. Scale bars in the bottom right corners indicate 20″. The LET and HET images were simulated following the procedure in Section 5 and include spectral and spatial models for the X-ray binary populations and the two AGNs in the merger system. For simplicity, we assume the X-ray binary populations are limited to the nuclei and model them as point sources coincident with the nuclei rather than as extended sources. While the two AGNs cannot be clearly detected in the NuSTAR imaging (nor was Arp 299-A detected above 10 keV by NuSTAR) (Ptak et al., 2015), the two AGNs can be clearly spatially resolved by both the LET and HET in the 2–10 keV and 10–25 keV bands.
To demonstrate HEX-P’s spectroscopic capabilities, we fit LET and HET spectra extracted with 8″ and 18″ radius apertures, respectively. These aperture choices represent an enclosed energy fraction (EEF) of
We perform the fitting of our simulated Arp 299 HEX-P spectra using v2.9 of the Bayesian X-ray Analysis (BXA; Buchner et al., 2014a; Buchner, 2021a) software package, which connects PyXspec (Arnaud, 1996) to the nested sampling (Skilling, 2004; Buchner, 2023) algorithm MLFriends (Buchner et al., 2014b; Buchner, 2019) implemented in UltraNest (Buchner, 2021b). Our reasoning for using nested sampling here is two-fold. First, the global parameter exploration enables the traversal of high dimensionality parameter spaces in an efficient manner that is minimally affected by local minima. Second, the pre-defined convergence criteria for nested sampling ensures our fitting process is devoid of biases that can be imposed when fitting of simulated data is not performed in a blind manner (see, e.g., Kammoun et al., 2022).
The resolving ability of the LET ensures negligible cross-contamination in the spectral extraction of both sources. However, despite the angular resolution of the HET being a considerable improvement over NuSTAR (see Figure 2), some cross-contamination remains (see also Figure 8). For this reason, we chose to fit the simulated LET and HET spectra for both AGN 1 and AGN 2 simultaneously whilst incorporating nuisance parameters to describe the amount of flux contamination in each source. Our spectral model thus includes the main spectral components used to simulate the AGNs, as well as a multiplicative factor to each AGN component to account for the relative contamination in each AGN HET spectrum. We manually set the cross-contamination to zero for the LET spectra, since any contamination is negligible, and assume that each HET spectrum contains 100% of the AGN emission that the extraction region was centered on and some fraction of the other AGN flux contaminating that extraction region.
We assigned log-uniform priors for all line-of-sight absorption (1022–1025 cm−2), cross-contamination factors (range of 0.001 to 1), high-energy cut-offs and normalizations. We additionally created custom cos-uniform priors (priors uniformly sampled over the angle range in cosine units) for all variable obscurer geometric angles (namely, the torus opening angle [0–84.3 degrees] and line-of-sight inclination [60–87.1 degrees] for both AGNs). Finally, the AGN photon indices were assigned Gaussian priors with mean 1.9 and 0.15 standard deviation and the X-ray binary photon index was assigned a uniform prior.
Figure 7 presents the fit acquired with BXA using a total of 18 free parameters. Owing to the combined broadband, sensitive coverage with the LET and HETs, we constrain the overall intrinsic X-ray luminosities and line-of-sight obscuration (log [NH/cm−2] =
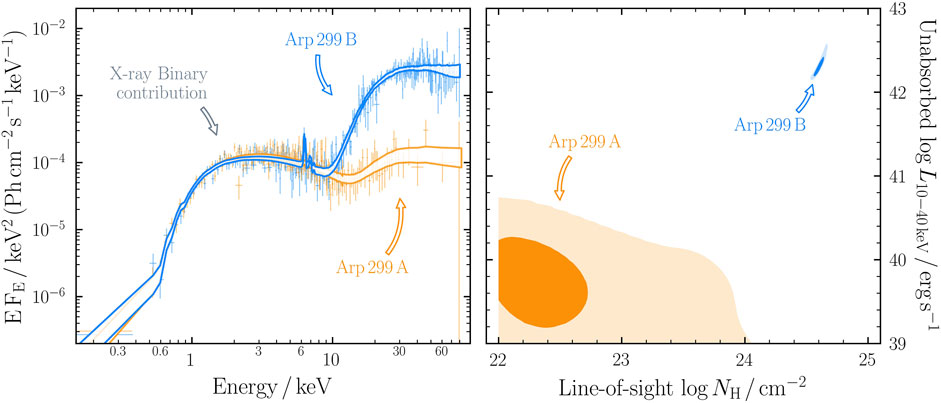
Figure 7. Simulated HEX-P HET (2-camera, co-added) and LET spectra, and the best-fitting models for the simulated Arp 299-A and Arp 299-B sources. The left panel shows the spectra unfolded with the best-fitting models while the right panel shows the contours for the best-fitting line-of-sight column densities and unabsorbed 10–40 keV luminosity in units of erg s−1. Blue and orange data points and model curves correspond to the Arp 299-B and Arp 299-A, respectively. While we find a slightly harder power law slope for the X-ray binary power law component, we recover very similar values for the AGN power law slopes and column densities to those used in the input models.
6 Spectroscopic fitting of dual AGNs
Simultaneous fitting of soft (Chandra and/or XMM-Newton) and hard (NuSTAR) X-ray spectra has been successfully performed in the past for a few closely separated dual AGN (e.g., NGC 6240, Mrk 273, Mrk 266, Nardini, 2017; Iwasawa et al., 2018; Iwasawa et al., 2020). At these pair separations (
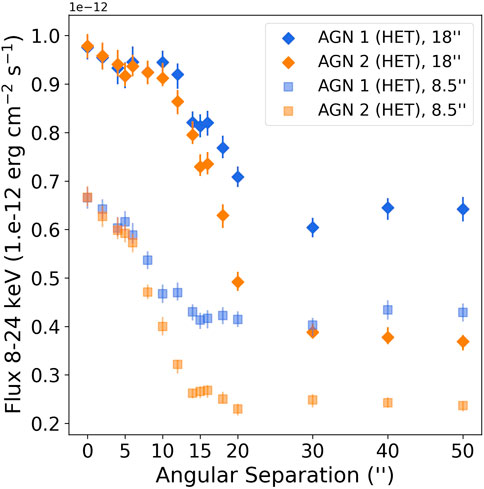
Figure 8. 8–24 keV observed fluxes from AGN 1 and 2 (from our general suite of simulations described in Section 3 and Section 4.1) as a function of pair separation. Diamonds correspond to spectra extracted from 2-camera HET imaging using 18″ radius apertures (
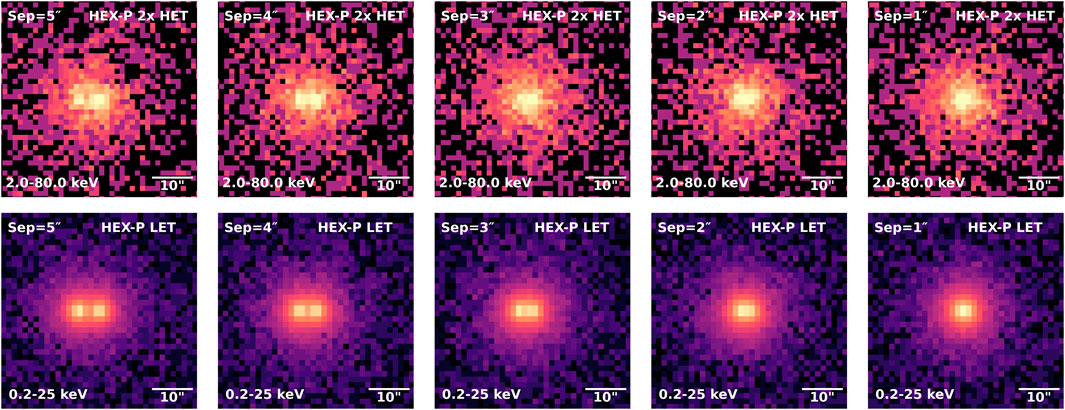
Figure 9. This 8-panel figure highlights the HEX-P HET and LET imaging for pair separations ranging from 5″ down to 1”. With the exception of the choice of pair separations, these panels are identical in design as Figures 1, 2. Here we can identify pairs of sources down to
7 Synergies with upcoming facilities
Given that dual AGNs are predicted to exhibit extremely red infrared colors (Blecha et al., 2018) in concert with high absorbing columns (e.g., Capelo et al. (2015); Blecha et al. (2018)), infrared observations are a natural choice to pair with hard and soft X-ray imaging and spectroscopy from HEX-P. Euclid, the recently launched ESA near-IR and optical imaging and spectroscopy mission (Laureijs et al., 2011), will provide sub-arcsecond near-IR and optical imaging and near-IR spectroscopy across approximately one third of the sky by the time HEX-P launches in the early 2030s. By the very nature of its mission design, Euclid will act as a new dual AGN survey facility, forming an enormous archival sample of interacting and merging galaxies from which promising near-IR dual AGNs can be selected and followed-up with HEX-P. JWST will also offer unprecedented near- and mid-IR imaging and spectroscopic observations of small samples of dual AGNs (and candidates) found both within deep surveys (e.g., Comerford et al. (2009); Civano et al. (2010); Civano et al. (2012)) and through targeted observations, and will provide the opportunity to probe not only heavily obscured AGNs but also heavily obscured star formation in, for example, dual AGNs hosted by LIRGs and ULIRGs (e.g.; Komossa et al. (2003); Iwasawa et al. (2011); Torres-Albà et al. (2018)). On the other hand, Extremely Large Telescopes (ELTs)—expected to come online in the 2030s–will usher in vast improvements in ground-based near-IR and optical spectroscopy and imaging and will experience substantially lower observational overheads and lower subscription rates than JWST. ELTs will therefore be paramount for targeting large samples of dual AGNs and may, like Euclid, represent the optimal dual AGN survey facilities that can work in tandem with HEX-P. In the meantime, high cadence, high resolution (≲ 1″ angular resolution) optical imaging with the upcoming Vera C. Rubin Observatory (Ivezić et al., 2019)—in concert with presently available optical and near-IR data from Euclid–will also aid in the development and preliminary analyses of large samples of dual AGN candidates. The Nancy Grace Roman Telescope (NGR), slated for launch in the 2030s, will operate at infrared wavelengths with a FOV 100× larger than HST with imaging sensitivity and PSF sizes superior to both HST and Euclid. NGR’s combination of FOV and wavelength coverage will make it an optimal facility for serendipitous dual AGN searches and studies and will complement both Euclid and HEX-P. Another important upcoming facility will be the ‘next-generation VLA’ (ngVLA, e.g., Burke-Spolaor et al. (2018)), which will boast 10× the sensitivity of the Jansky VLA and ALMA with 30× longer baselines that will enable 0.01″-0.001″ resolution across the 1.2–116 GHz frequency range. Since radio is also immune to obscuration, the ngVLA will have the sensitivity and resolution to detect dual AGNs to z ∼ 1, if they are sufficiently luminous in the radio. HEX-P and the ngVLA could work as separate follow-up facilities, or HEX-P could work in tandem with the ngVLA to confirm dual AGNs and measure the obscuring columns and try to constrain torus parameters (the latter of which the ngVLA would not be able to do). A joint HEX-P/ngVLA coverage strategy may be able to simultaneously circumvent X-ray and radio selection biases. In a similar vein, HEX-P observations in combination with ALMA 100–200 GHz continuum observations (Ricci et al., 2023) could offer unprecedented views to the most heavily obscured dual AGNs. Due to the reduced opacities in these ALMA bands, one can probe through very high column densities.
8 Conclusion
The HEX-P Probe-class mission concept combines high spatial resolution X-ray imaging (
• Without the aid of specialized detection algorithms, the spatial resolution of HEX-P LET and HET will allow us to resolve dual AGNs down to
• With the aid of Bayesian detection algorithms like that employed in this work, HEX-P will be able to confirm dual AGNs down to separations of
• Given HEX-P’s spatial resolution for both the LET and HET, HEX-P will have the capability to probe dual AGNs in late-stage mergers (
• HEX-P will enable distinct (relatively contamination free) spectral analyses with the LET in the 0.2–25 keV band down to separations of
HEX-P is poised to transform the landscape of dual AGN science, offering one of the cleanest methods of AGN detection that will be largely obscuration independent given the hard X-ray bandpass and enhanced faint source sensitivity of the HET. HEX-P will play a key role in uncovering heretofore unconfirmed dual AGNs in candidate systems, determining the occupation fraction of hard X-ray emitting and obscured dual AGNs in known and currently unknown dual AGN populations, and studying the fueling and obscuration levels of dual AGNs across the merger sequence and as a function of AGN type in the local Universe. Such observations will refine previously proposed correlations between the projected pair separations of the AGNs and their X-ray luminosities (Koss et al., 2012; Hou et al., 2020) and column densities (Guainazzi et al., 2021). Bayesian detection algorithms akin to BAYMAX (Foord et al., 2019; Foord et al., 2020; Foord et al., 2021) will provide statistically powerful improvements to the detectability of closely-separated sources, and such Bayesian methods will provide more accurate constraints on the flux ratios of close angular pairs and thereby improve the statistical priors used during the spectral fitting process. Furthermore, with the high sensitivity and angular resolution of the LET, HEX-P will allow the simultaneous examination of the host stellar populations via the detection of hot star formation-driven components in the soft X-rays, often observed in dual AGNs (see Section 5.4 in Pfeifle et al. (2023)). Thus, HEX-P will also probe connections between the properties of the host stellar populations and dual AGN activation and obscuration.
Data availability statement
The datasets presented in this study can be found in online repositories4. The names of the repository/repositories and accession number(s) can be found below: https://github.com/thatastroguy/HEXP.
Author contributions
RP: Conceptualization, Formal Analysis, Investigation, Methodology, Software, Visualization, Writing–original draft, Writing–review and editing. PB: Conceptualization, Formal Analysis, Software, Visualization, Writing–original draft, Writing–review and editing. KW: Writing–review and editing. JB: Conceptualization, Methodology, Software, Writing–review and editing. FC: Writing–review and editing. KM: Writing–original draft, Writing–review and editing. DS: Conceptualization, Writing–review and editing. NT-A: Writing–review and editing. EN: Writing–review and editing. CR: Writing–review and editing. SM: Writing–review and editing. DB: Writing–review and editing. Dominic Sicilian: Writing–review and editing. C-TC: Software, Writing–review and editing. EK: Writing–review and editing. RH: Conceptualization, Writing–review and editing. JG: Writing–review and editing. LM: Writing–review and editing.
Funding
The author(s) declare that financial support was received for the research, authorship, and/or publication of this article. RP gratefully acknowledges support through an appointment to the NASA Postdoctoral Program at Goddard Space Flight Center, administered by ORAU through a contract with NASA. The work of DS was carried out at the Jet Propulsion Laboratory, California Institute of Technology, under a contract with NASA. CR acknowledges support from Fondecyt Regular grant 1230345 and ANID BASAL project FB210003. EK acknowledges financial support from the Centre National d’Etudes Spatiales (CNES). LM is supported by the CITA National Fellowship.
Acknowledgments
We thank both reviewers for their thoughtful reviews that helped to improve our manuscript. We thank the SIXTE support team (with particular thanks to Ole König) for their assistance in generating spectra from simulated event files and for assistance with the documentation.
Conflict of interest
The authors declare that the research was conducted in the absence of any commercial or financial relationships that could be construed as a potential conflict of interest.
The author(s) declared that they were an editorial board member of Frontiers, at the time of submission. This had no impact on the peer review process and the final decision.
Publisher’s note
All claims expressed in this article are solely those of the authors and do not necessarily represent those of their affiliated organizations, or those of the publisher, the editors and the reviewers. Any product that may be evaluated in this article, or claim that may be made by its manufacturer, is not guaranteed or endorsed by the publisher.
Footnotes
1https://www.nustar.caltech.edu/page/response_files
2Selected presumably in the soft X-rays with Chandra, via high-resolution radio imaging, space-based high-resolution optical or near-IR spectroscopy (e.g., Hubble Space Telescope or JWST), or via ground-based adaptive-optics-assisted optical or near-IR observations. However, some fraction of dual AGNs will be missed at optical wavelengths (e.g., Koss et al., 2012) and require the detection of distinct X-ray AGNs for unambiguous confirmation.
3https://www.legacysurvey.org/viewer
4https://github.com/thatastroguy/HEXP
References
Agazie, G., Anumarlapudi, A., Archibald, A. M., Arzoumanian, Z., Baker, P. T., Bécsy, B., et al. (2023). The NANOGrav 15 yr data set: evidence for a gravitational-wave background. ApJL 951, L8. doi:10.3847/2041-8213/acdac6
Akaike, H. (1974). A new look at the statistical model identification. IEEE Trans. Automatic Control 19, 716–723. doi:10.1109/tac.1974.1100705
Alonso-Herrero, A., Roche, P. F., Esquej, P., González-Martín, O., Pereira-Santaella, M., Ramos Almeida, C., et al. (2013). Uncovering the deeply embedded active galactic nucleus activity in the nuclear regions of the interacting galaxy Arp 299. ApJL 779, L14. doi:10.1088/2041-8205/779/1/L14
Amaro-Seoane, P., Andrews, J., Arca Sedda, M., Askar, A., Baghi, Q., Balasov, R., et al. (2023). Astrophysics with the laser interferometer space antenna. Living Rev. Relativ. 26, 2. doi:10.1007/s41114-022-00041-y
Arnaud, K. A. (1996). “XSPEC: the first ten years,” in Astronomical data analysis software and systems V. Of astronomical Society of the pacific conference series. Editors G. H. Jacoby, and J. Barnes, 101, 17.
Ballo, L., Braito, V., Della Ceca, R., Maraschi, L., Tavecchio, F., and Dadina, M. (2004). Arp 299: a second merging system with two active nuclei? ApJ 600, 634–639. doi:10.1086/379887
Baloković, M., Brightman, M., Harrison, F. A., Comastri, A., Ricci, C., Buchner, J., et al. (2018). New spectral model for constraining torus covering factors from broadband X-ray spectra of active galactic nuclei. ApJ 854, 42. doi:10.3847/1538-4357/aaa7eb
Barnes, J. E., and Hernquist, L. (1996). Transformations of galaxies. II. Gasdynamics in merging disk galaxies. ApJ 471, 115–142. doi:10.1086/177957
Barnes, J. E., and Hernquist, L. E. (1991). Fueling starburst galaxies with gas-rich mergers. ApJL 370, L65. doi:10.1086/185978
Barrows, R. S., Comerford, J. M., Stern, D., and Assef, R. J. (2023). A census of WISE-selected dual and offset AGNs across the sky: new constraints on merger-driven triggering of obscured AGNs. ApJ 951, 92. doi:10.3847/1538-4357/acd2d3
Bianchi, S., Chiaberge, M., Piconcelli, E., Guainazzi, M., and Matt, G. (2008). Chandra unveils a binary active galactic nucleus in Mrk 463. MNRAS 386, 105–110. doi:10.1111/j.1365-2966.2008.13078.x
Blecha, L., Snyder, G. F., Satyapal, S., and Ellison, S. L. (2018). The power of infrared AGN selection in mergers: a theoretical study. MNRAS 478, 3056–3071. doi:10.1093/mnras/sty1274
Boorman, P. G., Torres-Albà, N., Annuar, A., Marchesi, S., Pfeifle, R., Stern, D., et al. (2023). The high energy X-ray probe (HEX-P): the circum-nuclear environment of growing supermassive black holes. arXiv e-prints , arXiv:2311.04949. doi:10.48550/arXiv.2311.04949
Buchner, J. (2019). Collaborative nested sampling: big data versus complex physical models. PASP 131, 108005. doi:10.1088/1538-3873/aae7fc
Buchner, J. (2021a). Bayesian X-ray analysis (BXA) v4.0. J. Open Source Softw. 6, 3045. doi:10.21105/joss.03045
Buchner, J. (2021b). UltraNest - a robust, general purpose Bayesian inference engine. J. Open Source Softw. 6, 3001. doi:10.21105/joss.03001
Buchner, J., Georgakakis, A., Nandra, K., Hsu, L., Rangel, C., Brightman, M., et al. (2014a). X-ray spectral modelling of the AGN obscuring region in the CDFS: Bayesian model selection and catalogue. A&A 564, A125. doi:10.1051/0004-6361/201322971
Buchner, J., Georgakakis, A., Nandra, K., Hsu, L., Rangel, C., Brightman, M., et al. (2014b). X-ray spectral modelling of the AGN obscuring region in the CDFS: Bayesian model selection and catalogue. A&A 564, A125. doi:10.1051/0004-6361/201322971
Burke-Spolaor, S., Blecha, L., Bogdanovic, T., Comerford, J. M., Lazio, T. J. W., Liu, X., et al. (2018). The next-generation very large array: supermassive black hole pairs and binaries. arXiv e-prints , arXiv:1808.04368. doi:10.48550/arXiv.1808.04368
Callegari, S., Kazantzidis, S., Mayer, L., Colpi, M., Bellovary, J. M., Quinn, T., et al. (2011). Growing massive black hole pairs in minor mergers of disk galaxies. ApJ 729, 85. doi:10.1088/0004-637X/729/2/85
Callegari, S., Mayer, L., Kazantzidis, S., Colpi, M., Governato, F., Quinn, T., et al. (2009). Pairing of supermassive black holes in unequal-mass galaxy mergers. ApJL 696, L89–L92. doi:10.1088/0004-637X/696/1/L89
Capelo, P. R., Dotti, M., Volonteri, M., Mayer, L., Bellovary, J. M., and Shen, S. (2017). A survey of dual active galactic nuclei in simulations of galaxy mergers: frequency and properties. MNRAS 469, 4437–4454. doi:10.1093/mnras/stx1067
Capelo, P. R., Volonteri, M., Dotti, M., Bellovary, J. M., Mayer, L., and Governato, F. (2015). Growth and activity of black holes in galaxy mergers with varying mass ratios. MNRAS 447, 2123–2143. doi:10.1093/mnras/stu2500
Ciurlo, A., Mannucci, F., Yeh, S., Amiri, A., Carniani, S., Cicone, C., et al. (2023). New multiple AGN systems with subarcsec separation: confirmation of candidates selected via the novel GMP method. A&A 671, L4. doi:10.1051/0004-6361/202345853
Civano, F., Elvis, M., Lanzuisi, G., Aldcroft, T., Trichas, M., Bongiorno, A., et al. (2012). Chandra high-resolution observations of CID-42, a candidate recoiling supermassive black hole. ApJ 752, 49. doi:10.1088/0004-637X/752/1/49
Civano, F., Elvis, M., Lanzuisi, G., Jahnke, K., Zamorani, G., Blecha, L., et al. (2010). A runaway black hole in COSMOS: gravitational wave or slingshot recoil? ApJ 717, 209–222. doi:10.1088/0004-637X/717/1/209
Civano, F., Hickox, R. C., Puccetti, S., Comastri, A., Mullaney, J. R., Zappacosta, L., et al. (2015). The nustar extragalactic surveys: overview and catalog from the COSMOS field. ApJ 808, 185. doi:10.1088/0004-637X/808/2/185
Comerford, J. M., Gerke, B. F., Stern, D., Cooper, M. C., Weiner, B. J., Newman, J. A., et al. (2012). Kiloparsec-scale spatial offsets in double-peaked narrow-line active galactic nuclei. I. Markers for selection of compelling dual active galactic nucleus candidates. ApJ 753, 42. doi:10.1088/0004-637X/753/1/42
Comerford, J. M., Griffith, R. L., Gerke, B. F., Cooper, M. C., Newman, J. A., Davis, M., et al. (2009). 1.75 h −1 kpc separation dual active galactic nuclei at z = 0.36 in the cosmos field. ApJL 702, L82–L86. –L86. doi:10.1088/0004-637X/702/1/L82
Comerford, J. M., Pooley, D., Barrows, R. S., Greene, J. E., Zakamska, N. L., Madejski, G. M., et al. (2015). Merger-driven fueling of active galactic nuclei: six dual and of agns discovered withchandraandhubble space telescopeobservations. ApJ 806, 219. doi:10.1088/0004-637X/806/2/219
Comerford, J. M., Pooley, D., Gerke, B. F., and Madejski, G. M. (2011). Chandra observations of a 1.9 kpc separation double X-ray source in a candidate dual active galactic nucleus galaxy at z = 0.16. ApJL 737, L19. doi:10.1088/2041-8205/737/1/L19
Dauser, T., Falkner, S., Lorenz, M., Kirsch, C., Peille, P., Cucchetti, E., et al. (2019). SIXTE: a generic X-ray instrument simulation toolkit. A&A 630, A66. doi:10.1051/0004-6361/201935978
De Rosa, A., Vignali, C., Husemann, B., Bianchi, S., Bogdanović, T., Guainazzi, M., et al. (2018). Disclosing the properties of low-redshift dual AGN through XMM-Newton and SDSS spectroscopy. MNRAS 480, 1639–1655. doi:10.1093/mnras/sty1867
De Rosa, A., Vignali, C., Severgnini, P., Bianchi, S., Bogdanović, T., Charisi, M., et al. (2023). The X-ray view of optically selected dual AGN. MNRAS 519, 5149–5160. doi:10.1093/mnras/stac3664
Ellison, S. L., Patton, D. R., Mendel, J. T., and Scudder, J. M. (2011). Galaxy pairs in the Sloan Digital Sky Survey - IV. Interactions trigger active galactic nuclei. MNRAS 418, 2043–2053. doi:10.1111/j.1365-2966.2011.19624.x
Ellison, S. L., Secrest, N. J., Mendel, J. T., Satyapal, S., and Simard, L. (2017). Discovery of a dual active galactic nucleus with ∼8 kpc separation. MNRAS 470, L49–L53. doi:10.1093/mnrasl/slx076
Eraerds, T., Antonelli, V., Davis, C., Hall, D., Hetherington, O., Holland, A., et al. (2021). Enhanced simulations on the athena/wide field imager instrumental background. J. Astronomical Telesc. Instrum. Syst. 7, 034001. doi:10.1117/1.JATIS.7.3.034001
Foord, A., Gültekin, K., Nevin, R., Comerford, J. M., Hodges-Kluck, E., Barrows, R. S., et al. (2020). A second look at 12 candidate dual AGNs using BAYMAX. ApJ 892, 29. doi:10.3847/1538-4357/ab72fa
Foord, A., Gültekin, K., Reynolds, M. T., Hodges-Kluck, E., Cackett, E. M., Comerford, J. M., et al. (2019). A bayesian analysis of SDSS J0914+0853, a low-mass dual AGN candidate. ApJ 877, 17. doi:10.3847/1538-4357/ab18a3
Foord, A., Gültekin, K., Runnoe, J. C., and Koss, M. J. (2021). AGN triality of triple mergers: detection of faint X-ray point sources. ApJ 907, 71. doi:10.3847/1538-4357/abce5d
Frey, S., Paragi, Z., An, T., and Gabányi, K. É. (2012). Two in one? A possible dual radio-emitting nucleus in the quasar SDSS J1425+3231. MNRAS 425, 1185–1191. doi:10.1111/j.1365-2966.2012.21491.x
Fu, H., Myers, A. D., Djorgovski, S. G., Yan, L., Wrobel, J. M., and Stockton, A. (2015a). Radio-selected binary active galactic nuclei from the very large array stripe 82 survey. ApJ 799, 72. doi:10.1088/0004-637X/799/1/72
Fu, H., Wrobel, J. M., Myers, A. D., Djorgovski, S. G., and Yan, L. (2015b). Binary active galactic nuclei in stripe 82: constraints on synchronized black hole accretion in major mergers. ApJL 815, L6. doi:10.1088/2041-8205/815/1/L6
Fu, H., Zhang, Z.-Y., Assef, R. J., Stockton, A., Myers, A. D., Yan, L., et al. (2011). A kiloparsec-scale binary active galactic nucleus confirmed by the expanded very large array. ApJL 740, L44. doi:10.1088/2041-8205/740/2/L44
Gabányi, K. É., An, T., Frey, S., Komossa, S., Paragi, Z., Hong, X. Y., et al. (2016). Four dual AGN candidates observed with the VLBA. ApJ 826, 106. doi:10.3847/0004-637X/826/2/106
Gabányi, K. É., Frey, S., Xiao, T., Paragi, Z., An, T., Kun, E., et al. (2014). A single radio-emitting nucleus in the dual AGN candidate NGC 5515. MNRAS 443, 1509–1514. doi:10.1093/mnras/stu1234
Ge, J.-Q., Hu, C., Wang, J.-M., Bai, J.-M., and Zhang, S. (2012). Double-peaked narrow emission-line galaxies from the sloan digital sky survey. I. Sample and basic properties. ApJS 201, 31. doi:10.1088/0067-0049/201/2/31
Goulding, A. D., Pardo, K., Greene, J. E., Mingarelli, C. M. F., Nyland, K., and Strauss, M. A. (2019). Discovery of a close-separation binary quasar at the heart of a z ∼ 0.2 merging galaxy and its implications for low-frequency gravitational waves. ApJL 879, L21. doi:10.3847/2041-8213/ab2a14
Gross, A. C., Fu, H., Myers, A. D., Djorgovski, S. G., Steffen, J. L., and Wrobel, J. M. (2023). Testing the radio-selection method of dual active galactic nuclei in the stripe 82 field. ApJ 945, 73. doi:10.3847/1538-4357/acb646
Gross, A. C., Fu, H., Myers, A. D., Wrobel, J. M., and Djorgovski, S. G. (2019). X-ray properties of radio-selected dual active galactic nuclei. ApJ 883, 50. doi:10.3847/1538-4357/ab3795
Guainazzi, M., De Rosa, A., Bianchi, S., Husemann, B., Bogdanovic, T., Komossa, S., et al. (2021). An XMM-Newton study of active-inactive galaxy pairs. MNRAS 504, 393–405. doi:10.1093/mnras/stab808
Guainazzi, M., Piconcelli, E., Jiménez-Bailón, E., and Matt, G. (2005). The early stage of a cosmic collision? XMM-Newton unveils two obscured AGN in the galaxy pair ESO509-IG066. A&A 429, L9–L12. doi:10.1051/0004-6361:200400104
Harrison, F. A., Craig, W. W., Christensen, F. E., Hailey, C. J., Zhang, W. W., Boggs, S. E., et al. (2013). The nuclear spectroscopic telescope array (NuSTAR) high-energy X-ray mission. ApJ 770, 103. doi:10.1088/0004-637X/770/2/103
Hopkins, P. F., Hernquist, L., Cox, T. J., Di Matteo, T., Robertson, B., and Springel, V. (2006). A unified, merger-driven model of the origin of starbursts, quasars, the cosmic X-ray background, supermassive black holes, and galaxy spheroids. ApJS 163, 1–49. doi:10.1086/499298
Hopkins, P. F., Hernquist, L., Cox, T. J., and Kereš, D. (2008). A cosmological framework for the Co-evolution of quasars, supermassive black holes, and elliptical galaxies. I. Galaxy mergers and quasar activity. ApJS 175, 356–389. doi:10.1086/524362
Hou, M., Li, Z., and Liu, X. (2020). A Chandra X-ray survey of optically selected AGN pairs. ApJ 900, 79. doi:10.3847/1538-4357/aba4a7
Hou, M., Li, Z., Liu, X., Li, Z., Li, R., Wang, R., et al. (2023). NOEMA detection of circumnuclear molecular gas in X-ray weak dual active galactic nuclei: No evidence for heavy obscuration. ApJ 943, 50. doi:10.3847/1538-4357/acaaf9
Hou, M., Liu, X., Guo, H., Li, Z., Shen, Y., and Green, P. J. (2019). Active galactic nucleus pairs from the sloan digital sky survey. III. Chandra X-ray observations unveil obscured double nuclei. ApJ 882, 41. doi:10.3847/1538-4357/ab3225
Hwang, H.-C., Hamer, J. H., Zakamska, N. L., and Schlaufman, K. C. (2020). Very wide companion fraction from Gaia DR2: a weak or no enhancement for hot Jupiter hosts, and a strong enhancement for contact binaries. MNRAS 497, 2250–2259. doi:10.1093/mnras/staa2124
Imanishi, M., Kawamuro, T., Kikuta, S., Nakano, S., and Saito, Y. (2020). Subaru infrared adaptive optics-assisted high-spatial-resolution imaging search for luminous dual active galactic nuclei in nearby ultraluminous infrared galaxies. ApJ 891, 140. doi:10.3847/1538-4357/ab733e
Imanishi, M., and Saito, Y. (2014). Subaru adaptive-optics high-spatial-resolution infrared K- and L’-band imaging search for deeply buried dual AGNs in merging galaxies. ApJ 780, 106. doi:10.1088/0004-637X/780/1/106
Inaba, K., Ueda, Y., Yamada, S., Ogawa, S., Uematsu, R., Tanimoto, A., et al. (2022). Broadband X-ray spectral analysis of the dual AGN system Mrk 739. ApJ 939, 88. doi:10.3847/1538-4357/ac97ec
Ivezić, Ž., Kahn, S. M., Tyson, J. A., Abel, B., Acosta, E., Allsman, R., et al. (2019). LSST: from science drivers to reference design and anticipated data products. ApJ 873, 111. doi:10.3847/1538-4357/ab042c
Iwasawa, K., Ricci, C., Privon, G. C., Torres-Albà, N., Inami, H., Charmandaris, V., et al. (2020). A Compton-thick nucleus in the dual active galactic nuclei of Mrk 266. A&A 640, A95. doi:10.1051/0004-6361/202038513
Iwasawa, K., Sanders, D. B., Teng, S. H., U, V., Armus, L., Evans, A. S., et al. (2011). C-GOALS: Chandra observations of a complete sample of luminous infrared galaxies from the IRAS Revised Bright Galaxy Survey. A&A 529, A106. doi:10.1051/0004-6361/201015264
Iwasawa, K., U, V., Mazzarella, J. M., Medling, A. M., Sanders, D. B., and Evans, A. S. (2018). Testing a double AGN hypothesis for Mrk 273. A&A 611, A71. doi:10.1051/0004-6361/201731662
Jansen, F., Lumb, D., Altieri, B., Clavel, J., Ehle, M., Erd, C., et al. (2001). XMM-Newton observatory. I. The spacecraft and operations. A&A 365, L1–L6. doi:10.1051/0004-6361:20000036
Kammoun, E. S., Barret, D., Peille, P., Willingale, R., Dauser, T., Wilms, J., et al. (2022). The defocused observations of bright sources with Athena/X-IFU. A&A 664, A29. doi:10.1051/0004-6361/202243606
Komossa, S., Burwitz, V., Hasinger, G., Predehl, P., Kaastra, J. S., and Ikebe, Y. (2003). Discovery of a binary active galactic nucleus in the ultraluminous infrared galaxy NGC 6240 using Chandra. ApJL 582, L15–L19. doi:10.1086/346145
Kosec, P., Brightman, M., Stern, D., Müller-Sánchez, F., Koss, M., Oh, K., et al. (2017). Investigating the evolution of the dual AGN system ESO 509-ig066. ApJ 850, 168. doi:10.3847/1538-4357/aa932e
Koss, M., Mushotzky, R., Treister, E., Veilleux, S., Vasudevan, R., and Trippe, M. (2012). Understanding dual active galactic nucleus activation in the nearby Universe. ApJL 746, L22. doi:10.1088/2041-8205/746/2/L22
Koss, M. J., Glidden, A., Baloković, M., Stern, D., Lamperti, I., Assef, R., et al. (2016). NuSTAR resolves the first dual AGN above 10 keV in SWIFT J2028.5+2543. ApJL 824, L4. doi:10.3847/2041-8205/824/1/L4
Koss, M. J., Treister, E., Kakkad, D., Casey-Clyde, J. A., Kawamuro, T., Williams, J., et al. (2023). UGC 4211: a confirmed dual active galactic nucleus in the local Universe at 230 pc nuclear separation. ApJL 942, L24. doi:10.3847/2041-8213/aca8f0
LaMassa, S. M., Yaqoob, T., Boorman, P. G., Tzanavaris, P., Levenson, N. A., Gandhi, P., et al. (2019). NuSTAR uncovers an extremely local compton-thick AGN in NGC 4968. ApJ 887, 173. doi:10.3847/1538-4357/ab552c
LaMassa, S. M., Yaqoob, T., Levenson, N. A., Boorman, P., Heckman, T. M., Gandhi, P., et al. (2017). Chandra reveals heavy obscuration and circumnuclear star formation in seyfert 2 galaxy NGC 4968. ApJ 835, 91. doi:10.3847/1538-4357/835/1/91
Laureijs, R., Amiaux, J., Arduini, S., Auguères, J. L., Brinchmann, J., Cole, R., et al. (2011). Euclid definition study report. arXiv e-prints , arXiv:1110.3193. doi:10.48550/arXiv.1110.3193
Li, K., Ballantyne, D. R., and Bogdanović, T. (2021). The detectability of kiloparsec-scale dual active galactic nuclei: the impact of galactic structure and black hole orbital properties. ApJ 916, 110. doi:10.3847/1538-4357/ac06a0
Liu, X., Civano, F., Shen, Y., Green, P., Greene, J. E., and Strauss, M. A. (2013). Chandra X-ray and Hubble space telescope imaging of optically selected kiloparsec-scale binary active galactic nuclei. I. Nature of the nuclear ionizing sources. ApJ 762, 110. doi:10.1088/0004-637X/762/2/110
Liu, X., Shen, Y., Strauss, M. A., and Greene, J. E. (2010). Type 2 active galactic nuclei with double-peaked [O III] lines: narrow-line region kinematics or merging supermassive black hole pairs? ApJ 708, 427–434. doi:10.1088/0004-637X/708/1/427
Liu, X., Shen, Y., Strauss, M. A., and Hao, L. (2011). Active galactic nucleus pairs from the sloan digital sky survey. I. The frequency on ˜5-100 kpc scales. ApJ 737, 101. doi:10.1088/0004-637X/737/2/101
Madsen, K. K., García, J. A., Stern, D., Armini, R., Basso, S., Coutinho, D., et al. (2023). The high energy X-ray probe (HEX-P): instrument and mission profile. arXiv e-prints , arXiv:2312.04678. doi:10.48550/arXiv.2312.04678
Mannucci, F., Pancino, E., Belfiore, F., Cicone, C., Ciurlo, A., Cresci, G., et al. (2022). Unveiling the population of dual and lensed active galactic nuclei at sub-arcsec separations. Nat. Astron. 6, 1185–1192. doi:10.1038/s41550-022-01761-5
Marchesi, S., Ajello, M., Marcotulli, L., Comastri, A., Lanzuisi, G., and Vignali, C. (2018). Compton-thick AGNs in the NuSTAR era. ApJ 854, 49. doi:10.3847/1538-4357/aaa410
Marchesi, S., Ajello, M., Zhao, X., Comastri, A., La Parola, V., and Segreto, A. (2019). Compton-thick AGNs in the NuSTAR era. V. Joint NuSTAR and XMM-Newton spectral analysis of three “soft-gamma” candidate CT-AGNs in the swift/BAT 100-month catalog. ApJ 882, 162. doi:10.3847/1538-4357/ab340a
Mazzarella, J. M., Iwasawa, K., Vavilkin, T., Armus, L., Kim, D. C., Bothun, G., et al. (2012). Investigation of dual active nuclei, outflows, shock-heated gas, and young star clusters in markarian 266. AJ 144, 125. doi:10.1088/0004-6256/144/5/125
Meidinger, N., Albrecht, S., Beitler, C., Bonholzer, M., Emberger, V., Frank, J., et al. (2020). “Development status of the wide field imager instrument for Athena,” in Society of Photo-Optical Instrumentation Engineers (SPIE) Conference Series, 114440T. doi:10.1117/12.2560507
Müller-Sánchez, F., Comerford, J. M., Nevin, R., Barrows, R. S., Cooper, M. C., and Greene, J. E. (2015). The origin of double-peaked narrow lines in active galactic nuclei. I. Very large array detections of dual AGNs and AGN outflows. ApJ 813, 103. doi:10.1088/0004-637X/813/2/103
Müller-Sánchez, F., Nevin, R., Comerford, J. M., Davies, R. I., Privon, G. C., and Treister, E. (2018). Two separate outflows in the dual supermassive black hole system NGC 6240. Nature 556, 345–348. doi:10.1038/s41586-018-0033-2
Nandra, K., Barret, D., Barcons, X., Fabian, A., den Herder, J.-W., Piro, L., et al. (2013). The hot and energetic Universe: a white paper presenting the science theme motivating the Athena+ mission. arXiv e-prints , arXiv:1306.2307. doi:10.48550/arXiv.1306.2307
Nardini, E. (2017). Nuclear absorption and emission in the AGN merger NGC 6240: the hard X-ray view. MNRAS 471, 3483–3493. doi:10.1093/mnras/stx1878
Oda, S., Ueda, Y., Tanimoto, A., and Ricci, C. (2018). Hard X-ray view of HCG 16 (Arp 318). ApJ 855, 79. doi:10.3847/1538-4357/aaaccc
Pérez-Torres, M. A., Alberdi, A., Romero-Cañizales, C., and Bondi, M. (2010). Serendipitous discovery of the long-sought active galactic nucleus in Arp 299-A. A&A 519, L5. doi:10.1051/0004-6361/201015462
Pfeifle, R. W., Satyapal, S., Manzano-King, C., Cann, J., Sexton, R. O., Rothberg, B., et al. (2019a). A triple AGN in a mid-infrared selected late-stage galaxy merger. ApJ 883, 167. doi:10.3847/1538-4357/ab3a9b
Pfeifle, R. W., Satyapal, S., Secrest, N. J., Gliozzi, M., Ricci, C., Ellison, S. L., et al. (2019b). Buried black hole growth in IR-selected mergers: new results from Chandra. ApJ 875, 117. doi:10.3847/1538-4357/ab07bc
Pfeifle, R. W., Weaver, K., Satyapal, S., Ricci, C., Secrest, N. J., Gliozzi, M., et al. (2023). NuSTAR observations of four mid-IR selected dual AGN candidates in galaxy mergers. arXiv e-prints , arXiv:2306.16437. doi:10.48550/arXiv.2306.16437
Piconcelli, E., Vignali, C., Bianchi, S., Mathur, S., Fiore, F., Guainazzi, M., et al. (2010). Witnessing the key early phase of quasar evolution: an obscured active galactic nucleus pair in the interacting galaxy IRAS 20210+1121. ApJL 722, L147–L151. doi:10.1088/2041-8205/722/2/L147
Ptak, A., Hornschemeier, A., Zezas, A., Lehmer, B., Yukita, M., Wik, D., et al. (2015). A focused, hard X-ray look at Arp 299 with NuSTAR. ApJ 800, 104. doi:10.1088/0004-637X/800/2/104
Ricci, C., Bauer, F. E., Treister, E., Schawinski, K., Privon, G. C., Blecha, L., et al. (2017). Growing supermassive black holes in the late stages of galaxy mergers are heavily obscured. MNRAS 468, stx173–1299. doi:10.1093/mnras/stx173
Ricci, C., Chang, C.-S., Kawamuro, T., Privon, G. C., Mushotzky, R., Trakhtenbrot, B., et al. (2023). A tight correlation between millimeter and X-ray emission in accreting massive black holes from <100 mas resolution ALMA observations. ApJL 952, L28. doi:10.3847/2041-8213/acda27
Ricci, C., Privon, G. C., Pfeifle, R. W., Armus, L., Iwasawa, K., Torres-Albà, N., et al. (2021). A hard X-ray view of luminous and ultra-luminous infrared galaxies in GOALS - I. AGN obscuration along the merger sequence. MNRAS 506, 5935–5950. doi:10.1093/mnras/stab2052
Rubinur, K., Das, M., and Kharb, P. (2019). Searching for dual AGN in galaxies with double-peaked emission line spectra using radio observations. MNRAS 484, 4933–4950. doi:10.1093/mnras/stz334
Rubinur, K., Kharb, P., Das, M., Rahna, P. T., Honey, M., Paswan, A., et al. (2021). A multiwavelength study of the dual nuclei in Mrk 212. MNRAS 500, 3908–3919. doi:10.1093/mnras/staa3375
Satyapal, S., Ellison, S. L., McAlpine, W., Hickox, R. C., Patton, D. R., and Mendel, J. T. (2014). Galaxy pairs in the sloan digital sky survey - IX. Merger-Induced AGN activity as traced by the wide-field infrared survey explorer. MNRAS 441, 1297–1304. doi:10.1093/mnras/stu650
Satyapal, S., Secrest, N. J., Ricci, C., Ellison, S. L., Rothberg, B., Blecha, L., et al. (2017). Buried AGNs in advanced mergers: mid-infrared color selection as a dual AGN candidate finder. ApJ 848, 126. doi:10.3847/1538-4357/aa88ca
Schawinski, K., Koss, M., Berney, S., and Sartori, L. F. (2015). Active galactic nuclei flicker: an observational estimate of the duration of black hole growth phases of ∼105 yr. MNRAS 451, 2517–2523. doi:10.1093/mnras/stv1136
Schwartzman, E., Clarke, T. E., Nyland, K., Secrest, N. J., Pfeifle, R. W., Schmitt, H. R., et al. (2023). VaDAR: varstrometry for dual AGN using radio interferometry. arXiv e-prints , arXiv:2306.13219. doi:10.48550/arXiv.2306.13219
Shen, Y., Chen, Y.-C., Hwang, H.-C., Liu, X., Zakamska, N., Oguri, M., et al. (2021). A hidden population of high-redshift double quasars unveiled by astrometry. Nat. Astron. 5, 569–574. doi:10.1038/s41550-021-01323-1
Shen, Y., Hwang, H.-C., Zakamska, N., and Liu, X. (2019). Varstrometry for off-nucleus and dual sub-kpc AGN (VODKA): how well centered are low-z AGN? ApJL 885, L4. doi:10.3847/2041-8213/ab4b54
Smith, K. L., Shields, G. A., Bonning, E. W., McMullen, C. C., Rosario, D. J., and Salviander, S. (2010). A search for binary active galactic nuclei: double-peaked [O III] AGNs in the sloan digital sky survey. ApJ 716, 866–877. doi:10.1088/0004-637X/716/1/866
Torres-Albà, N., Iwasawa, K., Díaz-Santos, T., Charmandaris, V., Ricci, C., Chu, J. K., et al. (2018). C-GOALS. II. Chandra observations of the lower luminosity sample of nearby luminous infrared galaxies in GOALS. A&A 620, A140. doi:10.1051/0004-6361/201834105
Van Wassenhove, S., Volonteri, M., Mayer, L., Dotti, M., Bellovary, J., and Callegari, S. (2012). Observability of dual active galactic nuclei in merging galaxies. ApJL 748, L7. doi:10.1088/2041-8205/748/1/L7
Veres, P. M., Gabányi, K. É., Frey, S., Paragi, Z., Kun, E., and An, T. (2021). European vlbi network observations of the proposed dual agn sdss j101022.95+141300.9. ApJ 922, 99. doi:10.3847/1538-4357/ac307d
Wang, J.-M., Chen, Y.-M., Hu, C., Mao, W.-M., Zhang, S., and Bian, W.-H. (2009). Active galactic nuclei with double-peaked narrow lines: are they dual active galactic nuclei? ApJL 705, L76–L80. doi:10.1088/0004-637X/705/1/L76
Weston, M. E., McIntosh, D. H., Brodwin, M., Mann, J., Cooper, A., McConnell, A., et al. (2017). Incidence of WISE -selected obscured AGNs in major mergers and interactions from the SDSS. MNRAS 464, 3882–3906. doi:10.1093/mnras/stw2620
Yamada, S., Ueda, Y., Oda, S., Tanimoto, A., Imanishi, M., Terashima, Y., et al. (2018). Broadband X-ray spectral analysis of the double-nucleus luminous infrared galaxy Mrk 463. ApJ 858, 106. doi:10.3847/1538-4357/aabacb
Zhou, H., Wang, T., Zhang, X., Dong, X., and Li, C. (2004). Obscured binary quasar cores in SDSS J104807.74+005543.5? ApJL 604, L33–L36. doi:10.1086/383310
Keywords: dual AGN, galaxy merger, x-ray astronomy, active galactic nucleus, galaxy interaction
Citation: Pfeifle RW, Boorman PG, Weaver KA, Buchner J, Civano F, Madsen K, Stern D, Torres-Albà N, Nardini E, Ricci C, Marchesi S, Ballantyne DR, Sicilian D, Chen C-T, Kammoun E, Hickox RC, García JA and Mallick L (2024) The high energy X-ray probe (HEX-P): the future of hard X-ray dual AGN science. Front. Astron. Space Sci. 11:1304652. doi: 10.3389/fspas.2024.1304652
Received: 29 September 2023; Accepted: 02 February 2024;
Published: 20 May 2024.
Edited by:
Murray Brightman, California Institute of Technology, United StatesReviewed by:
Roberto Serafinelli, Astronomical Observatory of Rome (INAF), ItalyErika Maria Benitez, National Autonomous University of Mexico, Mexico
Copyright © 2024 Pfeifle, Boorman, Weaver, Buchner, Civano, Madsen, Stern, Torres-Albà, Nardini, Ricci, Marchesi, Ballantyne, Sicilian, Chen, Kammoun, Hickox, García and Mallick. This is an open-access article distributed under the terms of the Creative Commons Attribution License (CC BY). The use, distribution or reproduction in other forums is permitted, provided the original author(s) and the copyright owner(s) are credited and that the original publication in this journal is cited, in accordance with accepted academic practice. No use, distribution or reproduction is permitted which does not comply with these terms.
*Correspondence: Ryan W. Pfeifle, ryan.w.pfeifle@nasa.gov