- 1Women’s Hospital, School of Medicine, Zhejiang University, Hangzhou, China
- 2Key Laboratory of Women’s Reproductive Health of Zhejiang Province, Hangzhou, China
- 3Key Laboratory of Reproductive Genetics, Ministry of Education, Zhejiang University, Hangzhou, China
Galectin-14 is specifically expressed in placental trophoblasts, and its expression is reduced in trophoblasts retrieved from the cervix of women destined to develop early pregnancy loss. However, the roles of galectin-14 in regulating trophoblasts and in the pathogenesis of pregnancy complication have never been investigated. In the current research, we aimed to investigate the roles of galectin-14 in the regulation of trophoblasts. Tissues of the placenta and villi were collected. Primary trophoblasts and human trophoblast cell line HTR-8/SVneo were used. Western blotting and RT-PCR were used to quantify gene expression. The siRNA-mediated galectin-14 knockdown and lentivirus-mediated overexpression were performed to manipulate the gene expression in trophoblasts. Transwell migration and invasion assays were used to evaluate cell migration and invasion capacity. Gelatin zymography was used to determine the gelatinase activity. Galectin-14 was significantly decreased in the villi of early pregnancy loss and the placenta of preeclampsia. Knockdown of galectin-14 in primary trophoblasts inhibited cell migration and invasion, downregulated the expression of matrix metalloproteinase (MMP)-9 and N-cadherin, the activity of MMP-9, and decreased the phosphorylation of Akt. Meanwhile, the overexpression of galectin-14 in HTR-8/SVneo promoted cell migration and invasion, upregulated the expression of MMP-9 and N-cadherin, the activity of MMP-9, and increased the phosphorylation of Akt. Increased Akt phosphorylation promoted cell migration and invasion and upregulated the expression and activity of MMP-9, while decreased Akt phosphorylation inhibited cell migration and invasion and downregulated the expression and activity of MMP-9. Thus, galectin-14 promotes trophoblast migration and invasion by enhancing the expression of MMP-9 and N-cadherin through Akt phosphorylation. The dysregulation of galectin-14 is involved in the pathogenesis of early pregnancy loss and preeclampsia.
Introduction
Placenta is a temporary organ connecting the fetus and her mother. The trophoblast is the main cellular component of the placenta. The normally functioning trophoblast is crucial for the establishment and maintenance of pregnancy, development of the placenta, remodeling of the spiral artery, and subsequently fetal growth and maturation (Turco and Moffett, 2019). Dysregulation of trophoblasts is associated with pregnancy complications including early pregnancy loss (EPL) (Huppertz, 2020) and preeclampsia (Huppertz, 2018).
The function of trophoblasts is regulated by a group of genes, especially placenta-specific genes including PLAC1 and syncytin (Rawn and Cross, 2008). These genes regulate trophoblastic migration, invasion, proliferation, apoptosis, and metabolism (Bolze et al., 2017; Wan et al., 2019). Galectins are among these genes.
Galectins are a family of soluble β-galactoside-binding proteins and consist of 20 members (Blois et al., 2019). Recently, several galectin family members including galectin-1, galectin-3, galectin-9, and galectin-13 were found to be dysregulated in pregnancy complications. The placental expressions of galectin-1 and galectin-13 were decreased in early pregnancy loss and preeclampsia (Liu et al., 2006; Freitag et al., 2013; Than et al., 2014), whereas the expressions of galectin-3 and galectin-9 were increased in preeclampsia (Jeschke et al., 2007; Blois et al., 2019).
Galectin-14, a recently identified galectin family member, is specifically expressed in placenta with over 12,000 times expression level compared with other tissues (Than et al., 2009). Using immunocytochemistry, a decrease in galectin-14 was observed in trophoblasts retrieved from the cervix at the gestational age of 5–10 weeks before the onset of early pregnancy loss (Fritz et al., 2015). However, the regulatory roles of galectin-14 in trophoblasts have never been investigated yet.
In the current study, the placental expressions of galectin-14 in early pregnancy loss and severe preeclampsia were determined, the effects of galectin-14 on the migration and invasion of trophoblast were observed, and mechanisms behind were explored.
Materials and Methods
Subjects
Villi were collected from 28 women who were undergoing artificial abortion and 28 cases of early pregnancy loss. Placenta tissues were collected from 20 women of normal pregnancy and 20 with severe preeclampsia at Cesarean sections.
Early pregnancy loss was diagnosed if the ultrasound examination showed an empty sac with a mean diameter over 25 mm or an embryo with a crown–rump length over 7 mm without heartbeat within the first 12 6/7 weeks of gestation (American College of Obstetricians and Gynecologists’ Committee on Practice Bulletins—Gynecology, 2018). Women undergoing artificial abortions with a similar gestational age served as control. The pregnancies complicated by autoimmune disease, genital malformation, history of exposure to poison or X-ray, and other acute or chronic diseases were excluded. Severe preeclampsia was diagnosed according to the criteria recommended by the American College of Obstetricians and Gynecologists (ACOG) (American College of Obstetricians and Gynecologists, 2019). Exclusion criteria were multiple pregnancy, pregnancy complicated by chronic hypertension, diabetes mellitus, and other acute or chronic diseases. Demographic characteristics of the cases were described in Tables 1, 2.
This study was approved by the Institutional Ethical Committee of Women’s Hospital, School of Medicine, Zhejiang University, and informed consents were obtained.
Cell Culture
HTR-8/SVneo, Swan-71, JAR, JEG-3, and BeWo were cultured in RPMI 1640 medium, DMEM/F12 medium, or Ham’s F-12K medium (Thermo Fisher Scientific, Waltham, United States) with 10% fetal bovine serum (FBS) and antibiotics (100 U/ml penicillin and 100 mg/ml streptomycin) at 37°C with 5% CO2. HTR-8/SVneo was obtained from ATCC (Rockville, MD, United States) and authenticated by STR profiling.
Primary extravillous trophoblasts (EVTs) were isolated from the villi of artificial abortion. Briefly, tissues were washed with cold phosphate buffered saline (PBS). Amniotic membrane and decidua were removed, and villi were minced. The fragments were resuspended with high-glucose DMEM with 10% FBS. After the cells attached on the bottom, bottles were washed with PBS gently, and fresh medium was added. EVT purity was examined by immunofluorescence staining of HLA-G and CK-7. Cells with purity over 90% and within four passages were used in the experiments.
Gene Knockdown and Overexpression
Cells were transfected with a SMARTpool siRNA or scramble siRNA (GenePharma, Shanghai, China) using Lipofectamine RNAiMAX transfection reagent (Thermo Fisher Scientific, Waltham, United States) according to the manufacturer’s instructions.
For overexpression, cells were transfected with lentivirus carrying overexpression vector or empty vehicle according to the proper multiplicity of infection (MOI). Here, 5–8 mg/ml polybrene (Cell-Land, Hangzhou, China) was added in the culture medium.
Cellular Immunofluorescence Assay
Adherent cells were washed with PBS three times and fixed with 4% paraformaldehyde (Biosharp, Hefei, China) for 15 min. Then, cells were permeabilized with 0.1% Triton X-100 (Sigma-Aldrich, Temecula, United States) for 10 min, blocked for 30 min in 5% bovine serum albumin (BSA) at room temperature, and incubated with primary antibodies against CK7 (1:100), HLA-G (1:100), and galectin-14/LGALS14 (1:100) at 4°C overnight. After rinsing with PBS, cells were incubated with a secondary fluorescein isothiocyanate (FITC) antibody (1:400 MultiSciences Biotech Co., Hangzhou, China) for 1 h at room temperature. Mounting medium with 4’,6-diamidino-2-phenylindole (DAPI) was added in the amount of 1 drop per hole and rinsed off in 5 min. The fluorescence signal was detected by fluorescence microscope (Leica, Wetzlar, Germany).
Western Blotting
Tissues were homogenized in 200 μl radioimmunoprecipitation assay (RIPA) lysis buffer containing protease inhibitor cocktail (Selleck, Houston, United States) on ice. Cells were lysed with 50 μl RIPA lysis buffer containing protease inhibitor cocktail on ice.
Lysates were centrifuged at 15,000 g at 4°C for 30 min. The protein concentration was quantified using BSA kit (MultiSciences Biotech Co., Hangzhou, China). In total, 30 μg protein was separated by 12% sodium dodecyl sulfate (SDS)-polyacrylamide gel and transferred onto a polyvinylidene fluoride (PVDF) membrane (Bio-Rad, Hercules, United States) with ice. Membranes were blocked for 1 h with Tris-buffered saline containing 0.1% Tween 20 (TBS/T) containing 5% BSA (Solarbio, Beijing, China) at room temperature. Then, membranes were incubated with primary antibodies against galectin-14/LGALS14 (1:1,000), VE-cadherin (1:1,000), N-cadherin (1:1,000), matrix metalloproteinase 9 (MMP-9; 1:1,000), tissue inhibitor of matrix metalloproteinase 1 (TIMP-1; 1:1,000), MMP-2 (1:1,000), TIMP-2 (1:1,000), phospho-Akt (1:1,000), Akt (1:1,000), and glyceraldehyde 3-phosphate dehydrogenase (GAPDH; 1:1,000) at 4°C overnight. After washing in TBS/T, membranes were incubated with horseradish peroxidase (HRP)-labeled secondary antibodies (1:5,000) for 1 h. The bands were visualized with enhanced chemiluminescence (ECL) kit (Fdbio Science, Hangzhou, China) by autoradiography (ImageQuant TL Software, GE Healthcare, Pittsburgh, United States).
Reagents and Antibodies
The phosphatidylinositol 3-kinase (PI3K) inhibitor LY294002 and Akt agonist SC79 were purchased from Selleck (Houston, United States). Rabbit monoclonal anti-LGALS14 (ab150427), rabbit monoclonal anti-VE-cadherin (ab33168), rabbit monoclonal anti-N-cadherin (ab76011), mouse monoclonal anti-MMP-9 (ab119906), rabbit monoclonal anti-TIMP1 (ab109125), rabbit monoclonal anti-MMP-2 (ab92536), rabbit monoclonal anti-TIMP-2 (ab180630), rabbit monoclonal anti-GAPDH (ab181602), rabbit monoclonal anti-cytokeratin 7 (ab181598), and mouse monoclonal anti-HLA G (ab52455) antibodies were purchased from Abcam (Cambridge, United Kingdom). Rabbit monoclonal anti-p-Akt (Ser473) (9271) and rabbit monoclonal anti-Akt (9272) antibodies were purchased from Cell Signaling Technology (Danvers, United States). Goat anti-mouse IgG (H + L) HRP and goat anti-rabbit IgG (H + L) HRP secondary antibodies were purchased from MultiSciences Biotech Co. (Hangzhou, China).
RT-PCR and RT-qPCR
RNA isolation and reverse transcription were performed as previously described (Yan et al., 2016).
Reverse transcription semiquantitative PCR (RT-PCR) was performed using a PTC 200 thermal cycler, and each 25-μl reaction contained 1 × GoldStarTaq Master Mix, 100 ng cDNA, and 400 nmol/L (nM) of each specific primer. Amplifications were performed as follows: 10 min at 95°C, 30 amplification cycles (30 s at 95°C, 30 s at 55°C, and 1 min at 72°C) and final extension for 5 min at 72°C. PCR products were analyzed by 2% ethidium bromide staining agarose gel electrophoresis, quantified by densitometry (Image Lab software, Bio-Rad Laboratories Inc., Hercules, United States) and normalized against GAPDH.
The quantitative PCR (qPCR) was performed in ViiA 7 Real-Time PCR System (Thermo Fisher Scientific, Waltham, United States) by using TB Green Premix Ex Taq (Tli RNase H Plus) Kit (Takara Bio Inc., Shiga, Japan) according to the manufacturer’s instructions.
The sequences of primers were as follows:
Galectin-14 forward 5′-CATCACTACCCGTACCATACA CACT-3′,
reverse 5′-GCTGTGGGTCCTTGACAAAAGTGAG-3′;
GAPDH forward 5′-GGCAAATTCCATGG CACCGT-3′,
reverse 5′-TGGACTCCACGACGTACTCA-3′;
Galectin-1 forward 5′-GGAGGCTGTCTTTCCCTTCC-3′,
reverse 5′-GTTGATGGCCTCCAGGTTGA-3′;
Galectin-2 forward 5′-GCGAATCCACCATTGTCTGC-3′,
reverse 5′-GGCTGAAGCACAGGTGATCT-3′;
Galectin-3 forward 5′-GAGCACCTGGAGCTTATCCC-3′,
reverse 5′-GCACTTGGCTGTCCAGAAGA-3′;
Galectin-8 forward 5′-CGGTAATCCCGTTTGTTGGC-3′,
reverse 5′-CACCTGGAATCTGTCTGCGT-3′;
Galectin-9 forward 5′-GCCCCTGGACAGATGTTCTC-3′,
reverse 5′-CAGTGCCTGACAGGAGGATG-3′;
Galectin-10 forward 5′-TGACAATCAAAGGGCGAC CA-3′,
reverse 5′-CGACGACCAAAGCACACTTG-3′;
Galectin-13 forward 5′-TGTCTTCTTTACCCGTGC CA-3′,
reverse 5′-TGCAGCTGTGGGTCATTGAT-3′
Gelatin Zymography
Cells were cultured in serum-free medium for 24 h. The supernatant was collected after being centrifuged at 3,000 g for 15 min to remove the cell fragment. Then, the supernatant was concentrated by 10 times using Amicon Utra-0.5 10 kDa Centrifugal Filter Unit (Merck Millipore, Darmstadt, Germany). The protein concentration was measured by BSA kit (MultiSciences Biotech Co., Hangzhou, China). Here, 15 μg protein was separated by 7.5% SDS-polyacrylamide gel containing gelatin. Then, the gel was washed with washing buffer [50 mmol/L (mM) Tris-HCl, pH 7.5, containing 2.5% Triton X-100, 5 mM CaCl2, 1 μmol/L (μM) (ZnCl2)] at room temperature and incubated in incubating buffer (50 mM Tris-HCl, pH 7.5, containing 1% Triton X-100, 5 mM CaCl2, 1 μM ZnCl2) at 37°C for 24 h. After that, the gel was dyed by Coomassie blue staining solution (Solarbio, Beijing, China) for 1 h and was decolored by destaining solution (containing 40% methanol, 10% acetic acid) until white bands were visible.
Transwell Migration and Invasion Assays
Cells were harvested and seeded in the upper chamber with serum-free medium. For invasion assay, the upper chamber was precoated with Matrigel (BD, Franklin Lakes, United States). And the lower chamber contained 500 μl complete medium. After 8 h for migrating or 24 h for invading, the bottoms of the inserts were fixed and stained in a solution containing 0.1% Gentian violet and 20% methanol. Cells were photographed (50×, 100×) and counted (100×) with an inverted phase contrast microscope (Leica, Wetzlar, Germany).
Statistical Analysis
Kolmogorov–Smirnov test was used to test the normal distribution of the data. Levene test was used to test the homogeneity of variance. Student t-test and Mann–Whitney test were used for data comparison. p < 0.05 was considered significant. SPSS (International Business Machines Co., Armonk, United States) was used for statistical analysis.
Results
Placental Galectin-14 Is Dysregulated in Early Pregnancy Loss and Preeclampsia
Placenta tissues were obtained from women with early pregnancy loss, preeclampsia, and their controls. Demographic characteristics of the subjects were described in Tables 1, 2. There were no significant differences in maternal age and the gestational age at curettage between early pregnancy loss and its control. There were no significant differences in maternal age and fetal gender between severe preeclampsia and its control. The significant differences in blood pressure and proteinuria were consistent with the diagnosis of preeclampsia. The differences in gestational age at delivery and neonatal birth weight were due to preeclampsia. The difference in body mass index (BMI) was in accordance with the conclusion obtained in previous research that higher BMI is a risk factor for preeclampsia (Bartsch et al., 2016) (Table 2).
Galectin-14 expression was determined by Western blotting. Placental expression of galectin-14 was significantly lower in both early pregnancy loss and preeclampsia as compared with their controls (Figure 1). The dysregulation of placental galectin-14 in early pregnancy loss and preeclampsia implies its possible role in the pathogenesis of pregnancy complications and in the regulation of trophoblasts.
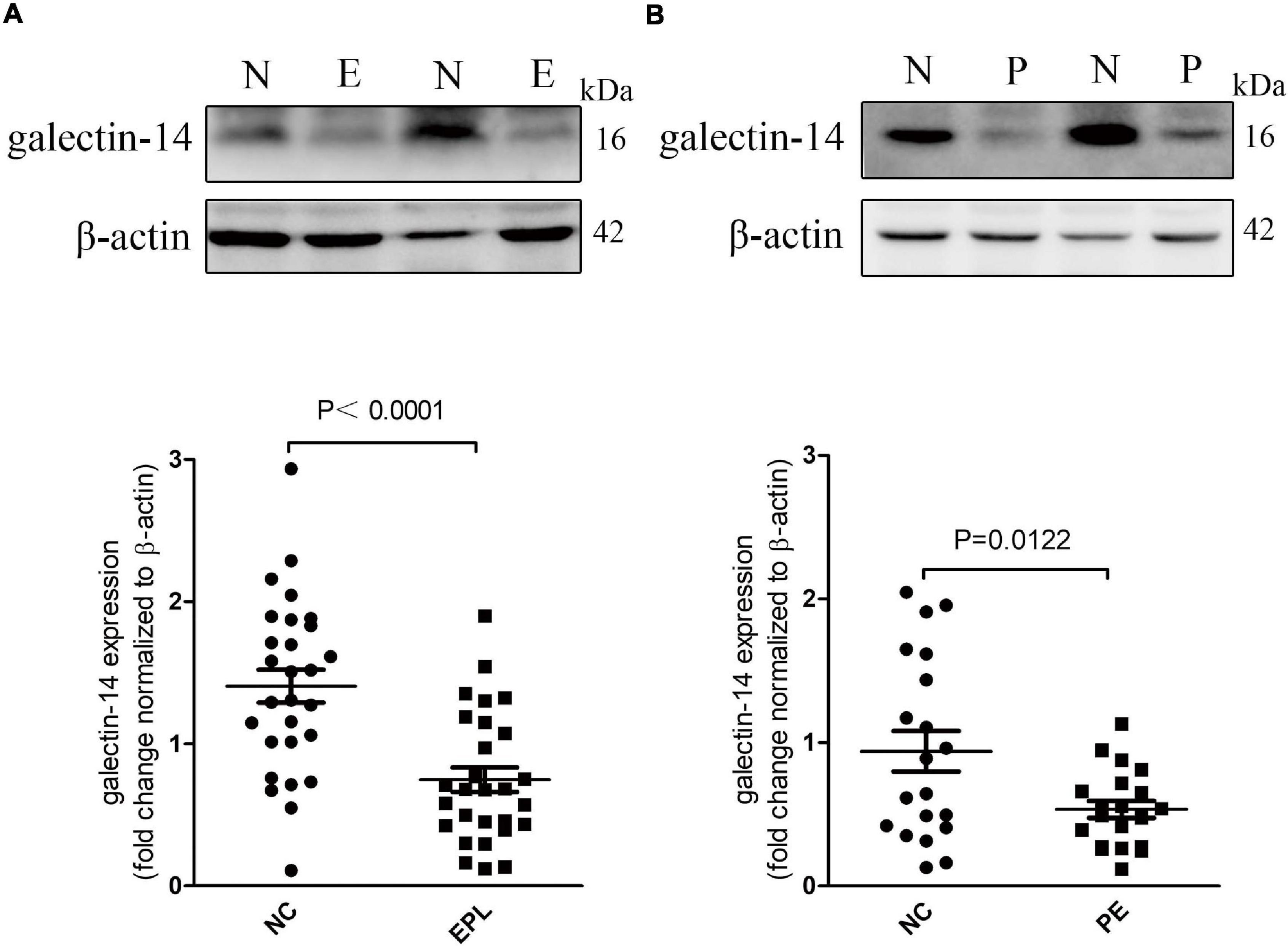
Figure 1. Galectin-14 is decreased in early pregnancy loss villi and preeclampsia placenta. (A,B) The expression level changes of galectin-14 in early pregnancy loss villi (n = 28) (A) and preeclampsia placentae (n = 20) (B) were determined by Western blotting. Dots in the graphs represent the number of biological samples analyzed. Results are presented as mean ± SEM. Statistical analysis: Student’s t-test. N or NC, control group; EPL or E, early pregnancy loss villi; P, preeclampsia placentae.
Galectin-14 Promotes Trophoblast Migration and Invasion
With RT-PCR, the expression of galectin-14 was detected in primary EVTs but not in trophoblast cell lines (Supplementary Figure S). These results were confirmed in primary EVTs and HTR-8/SVneo by immunofluorescence (Figures 2A,B) and by Western blotting (Figure 3). siRNA-mediated galectin-14 knockdown significantly decreased the migration and invasion of primary EVTs (Figure 4A). The overexpression of galectin-14 by using lentivirus transfection significantly enhanced the migration and invasion of HTR-8/SVneo cells (Figure 4B). When the overexpression of galectin-14 was knocked down by siRNA, the enhancement of migration and invasion ability was abrogated (Figure 4C).
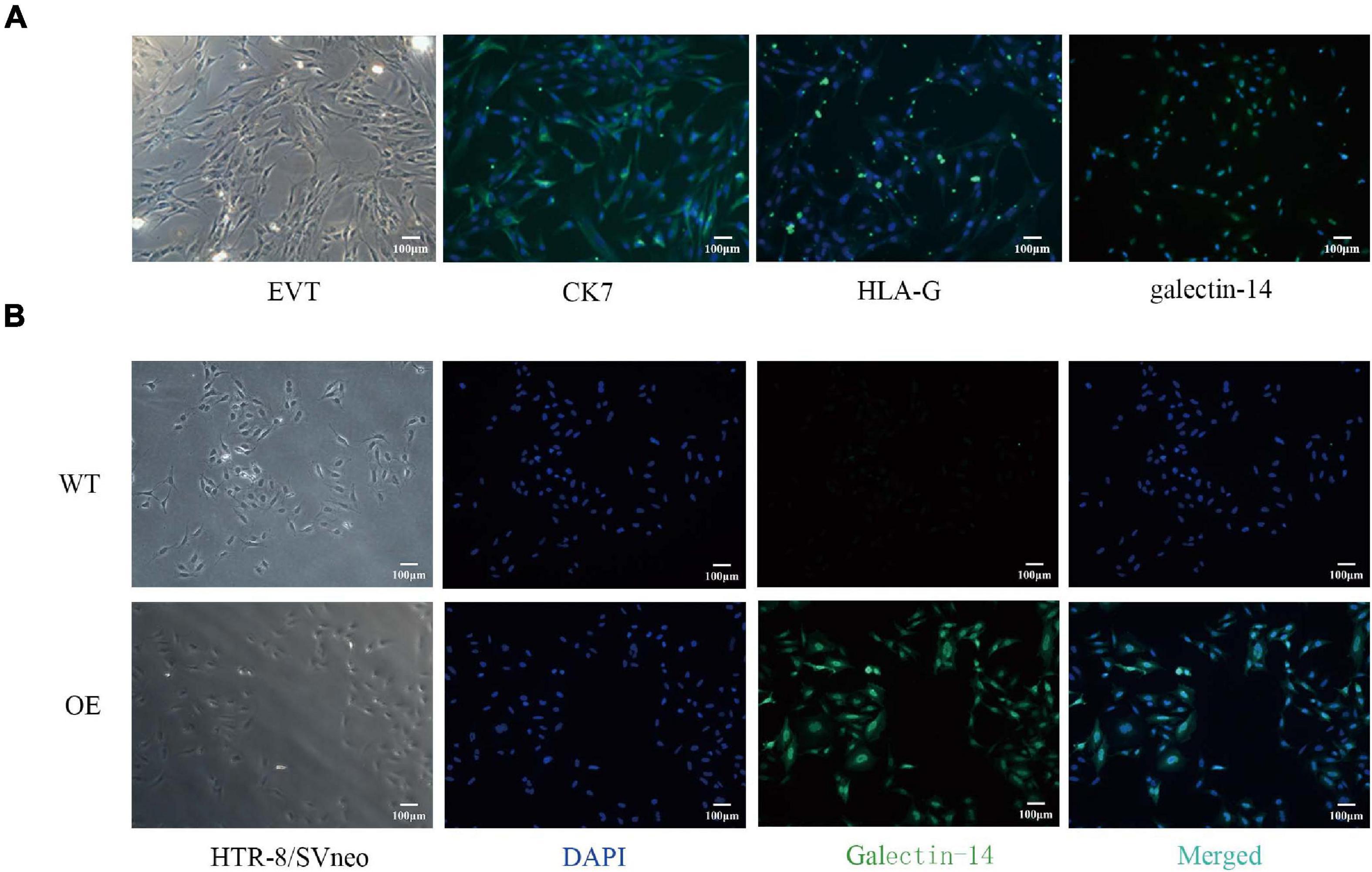
Figure 2. Distribution of galectin-14 in primary extravillous trophoblasts (EVTs) and galectin-14-overexpressed HTR-8/SVneo cell. (A) The primary EVTs were immunofluorescent stained by CK-7, HLA-G, and galectin-14 antibodies. (B) HTR-8/SVneo cell was transfected with flag-tagged galectin-14 vector or with empty vehicle. The location of galectin-14 in HTR-8/SVneo cell was identified by immunofluorescent method. Scale bar: 100 μm. EVT, primary extravillous trophoblast; CK7, cytokeratin-7; HLA-G, human leukocyte antigen-G; WT, HTR-8/SVneo transfected with empty vehicle; OE, galectin-14-overexpressed HTR-8/SVneo.
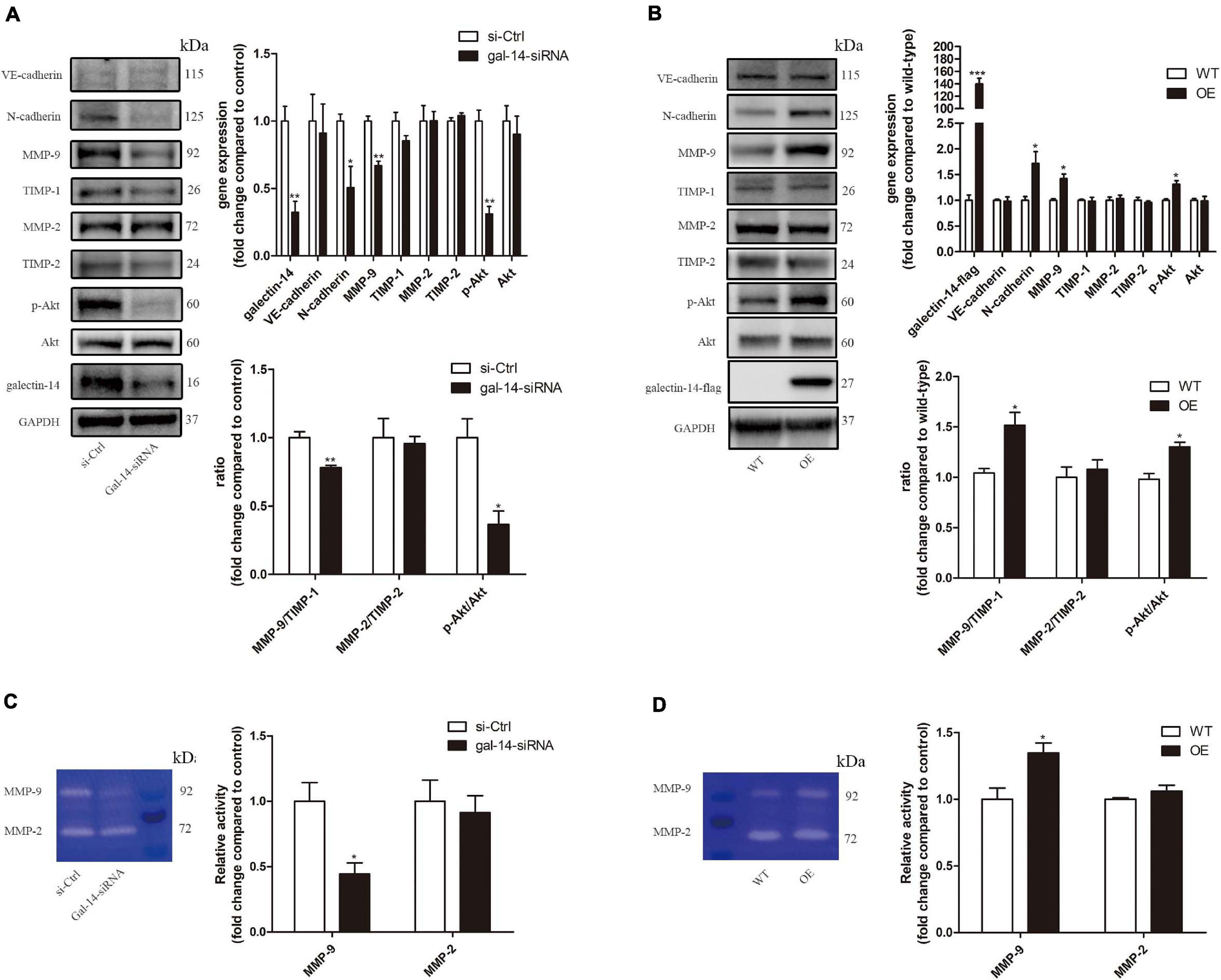
Figure 3. Galectin-14 promotes the expression of N-cadherin and matrix metalloproteinase (MMP)-9, Akt phosphorylation, and activity of MMP-9 in trophoblasts. (A,C) Primary extravillous trophoblasts (EVTs) were transfected with scramble siRNA or siRNA targeting galectin-14. (B,D) HTR-8/Svneo was transfected with empty vehicle or vector containing galectin-14 gene. (A,B) Protein expression was assessed by Western blotting with antibodies against VE-cadherin, N-cadherin, MMP-9, tissue inhibitor matrix metalloproteinase (TIMP)-1, MMP-2, TIMP-2, p-Akt, Akt, and galectin-14 and normalized to glyceraldehyde 3-phosphate dehydrogenase (GAPDH). (C,D) Gelatinase activity was determined by gelatin zymography. Results are presented as mean ± SEM of biological triplicates. Statistical analysis: Student’s t-test. *p < 0.05, **p < 0.01, ***p < 0.001. si-Ctrl, cells transfected with scramble siRNA; gal-14-siRNA, cells transfected with siRNA targeting galectin-14; WT, HTR-8/SVneo transfected with empty vehicle; OE, galectin-14-overexpressed HTR-8/SVneo.
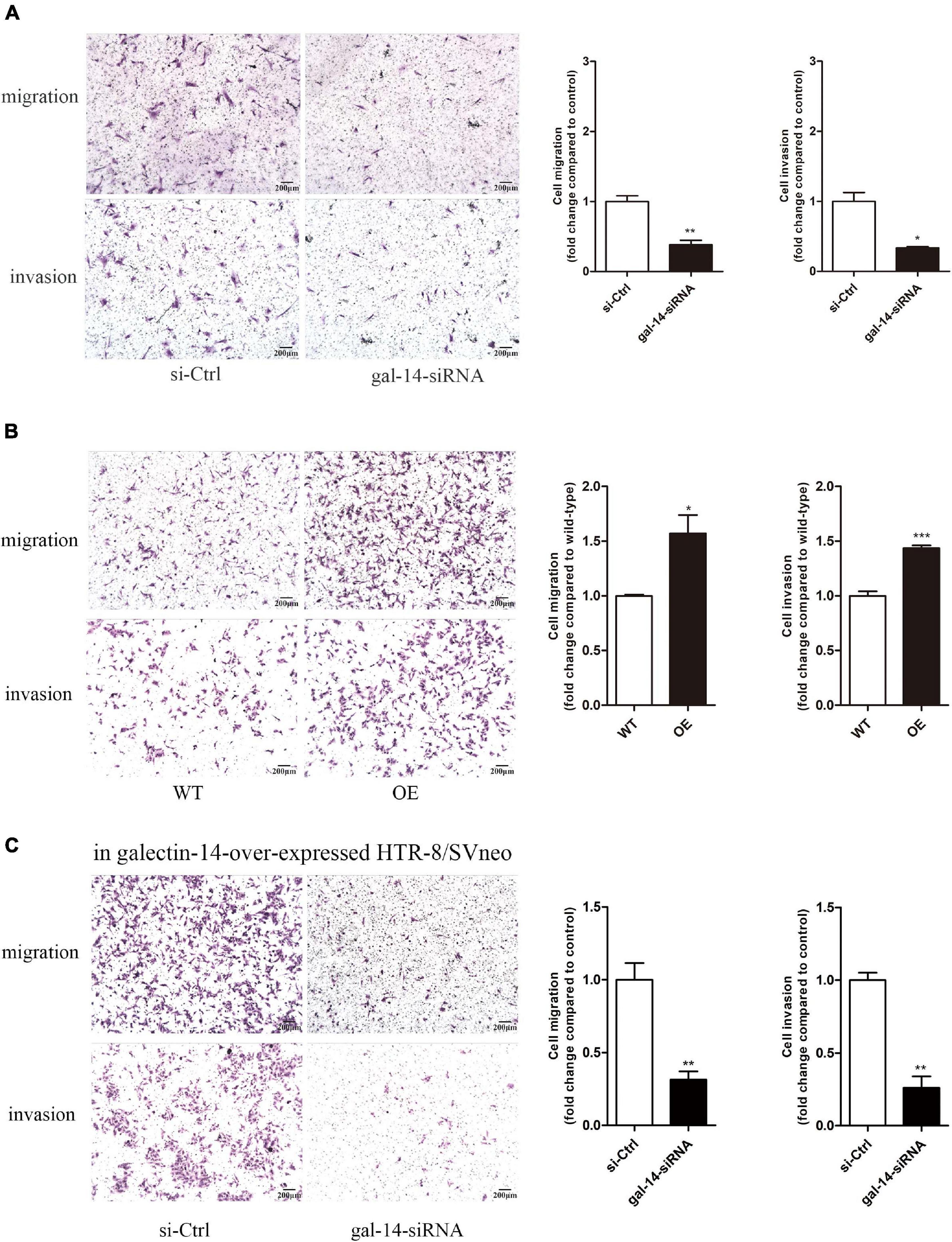
Figure 4. Galectin-14 promotes trophoblast migration and invasion. (A) Primary extravillous trophoblasts (EVTs) were transfected with scramble siRNA or siRNA targeting galectin-14. (B) HTR-8/Svneo was transfected with empty vehicle or vector containing galectin-14 gene. (C) The galectin-14-overexpressed HTR/SVneo was transfected with scramble siRNA or siRNA targeting galectin-14. (A–C) The migration and invasion ability of EVTs were examined using transwell migration and invasion assays. Results are represented as mean ± SEM of biological triplicates. Statistical analysis: Student’s t-test. *p < 0.05, **p < 0.01, ***p < 0.001. Scale bar: 200 μm. si-Ctrl, cells transfected with scramble siRNA; gal-14-siRNA, cells transfected with siRNA targeting galectin-14; WT, HTR-8/SVneo transfected with empty vehicle; OE, galectin-14-overexpressed HTR-8/SVneo.
Galectin-14 Promotes the Expression of N-Cadherin and Matrix Metalloproteinase 9 in Trophoblasts
The expressions of N-cadherin, VE-cadherin, E-cadherin, MMP-2, MMP-9, TIMP-2, and TIMP-1 were examined in galectin-14 knockdown primary EVTs and galectin-14-overexpressed HTR-8/SVneo. A decrease in N-cadherin, MMP-9 expression, MMP-9 activity, and MMP-9/TIMP-1 ratio was observed in galectin-14-knockdown primary EVTs (Figures 3A,C). On the contrary, an upregulation of N-cadherin, MMP-9 expression, MMP-9 activity, and MMP-9/TIMP-1 ratio was detected in galectin-14-overexpressed HTR-8/SVneo (Figures 3B,D). No significant change of VE-cadherin expression was detected. E-cadherin was not detectable in HTR-8/SVneo and in human primary EVTs, which might due to the hypermethylation of the promoter (Chen et al., 2013; Cheng et al., 2013; Szklanna et al., 2017).
N-Cadherin and Matrix Metalloproteinase 9 Contribute to the Promotion of Trophoblast Migration and Invasion
To investigate the involvement of N-cadherin and MMP-9 upregulation in the promotion of trophoblast migration and invasion, we performed siRNA-mediated knockdown of N-cadherin and MMP-9 in galectin-14-overexpressed HTR-8/SVneo. The N-cadherin and MMP-9 expression reduced by 75 and 60%, respectively (Figure 5A). The activity of MMP-9 reduced by 70–90% (Figure 5B). Knockdown of MMP-9 and N-cadherin decreased migration and invasion of HTR-8/SVneo (Figure 5C).
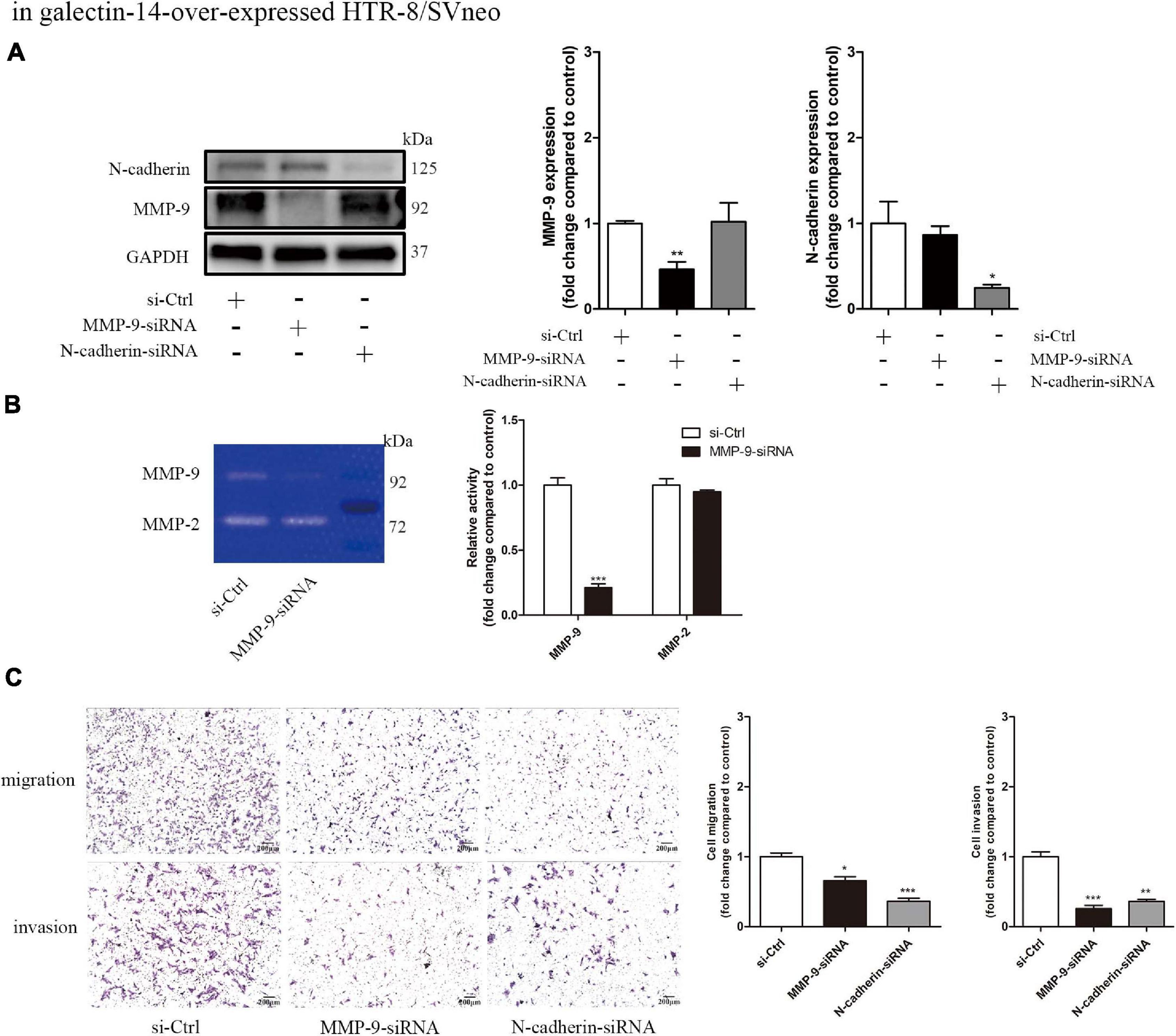
Figure 5. Knockdown of matrix metalloproteinase (MMP)-9 and N-cadherin decreases the migration and invasion of galectin-14-overexpressed HTR/Svneo. siRNA-mediated MMP-9 and N-cadhrin knockdown was performed in galectin-14-overexpressed HTR-8/Svneo. (A) Protein expression was assessed by Western blotting with antibodies against N-cadherin and MMP-9 and normalized to glyceraldehyde 3-phosphate dehydrogenase (GAPDH). (B) Gelatinase activity was determined by gelatin zymography. (C) The migration and invasion ability of extravillous trophoblasts (EVTs) were examined using transwell migration and invasion assays. Results are represented as mean ± SEM of biological triplicates. Statistical analysis: Student’s t-test. *p < 0.05, **p < 0.01, ***p < 0.001. Scale bar: 200 μm. si-Ctrl, cells transfected with scramble siRNA; MMP-09-siRNA, cells transfected with siRNA targeting MMP-9; N-cadherin-siRNA, cells transfected with siRNA targeting N-cadherin.
Akt Signaling Is Involved in Galectin-14 Promotion of Matrix Metalloproteinase 9 Expression
Inhibition of Akt phosphorylation at Ser473 was observed in galectin-14-knockdown primary EVTs, while activation was detected in galectin-14-overexpressed HTR-8/SVneo (Figures 3A,B). Akt phosphorylation agonist (SC79) enhanced the cell migration and invasion ability (Figure 6) and increased MMP-9 expression and activity and MMP-9/TIMP-1 ratio (Figure 7). On the contrary, Akt inhibitor (LY294002) inhibited the cell migration and invasion (Figure 6) and decreased MMP-9 expression and activity and MMP-9/TIMP-1 ratio (Figure 7). No significant change was detected in N-cadherin expression. These findings imply that enhancement of Akt phosphorylation is necessary for the promotion of MMP-9 by galectin-14.
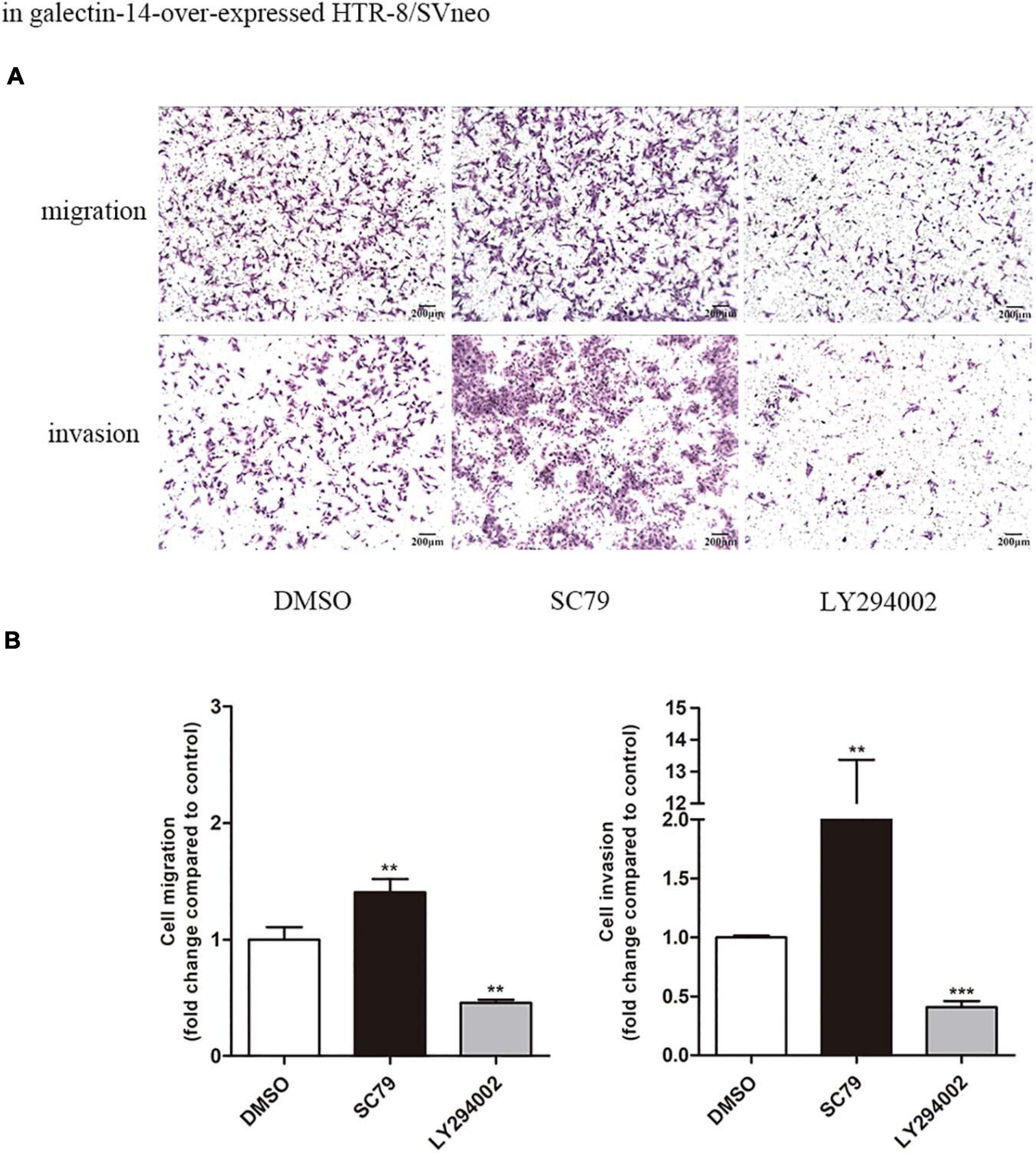
Figure 6. Increased phosphorylation of Akt promotes trophoblast migration and invasion in HTR-8/SVneo. In galectin-14-overexpressed HTR-8/SVneo, 10 μM SC79 or 10 μM LY294002 was added as the agonist or inhibitor of Akt phosphorylation. (A) The migration and invasion ability of extravillous trophoblasts (EVTs) were examined using transwell migration and invasion assays. (B) Results are represented as mean ± SEM of biological triplicates. Statistical analysis: Student’s t-test. **p < 0.01, ***p < 0.001. Scale bar: 200 μm. Dimethylsulfoxide (DMSO), cells added with DMSO of the same volume; SC79, cells added with 10 μM SC79; LY294002, cells added with 10 μM LY294002.
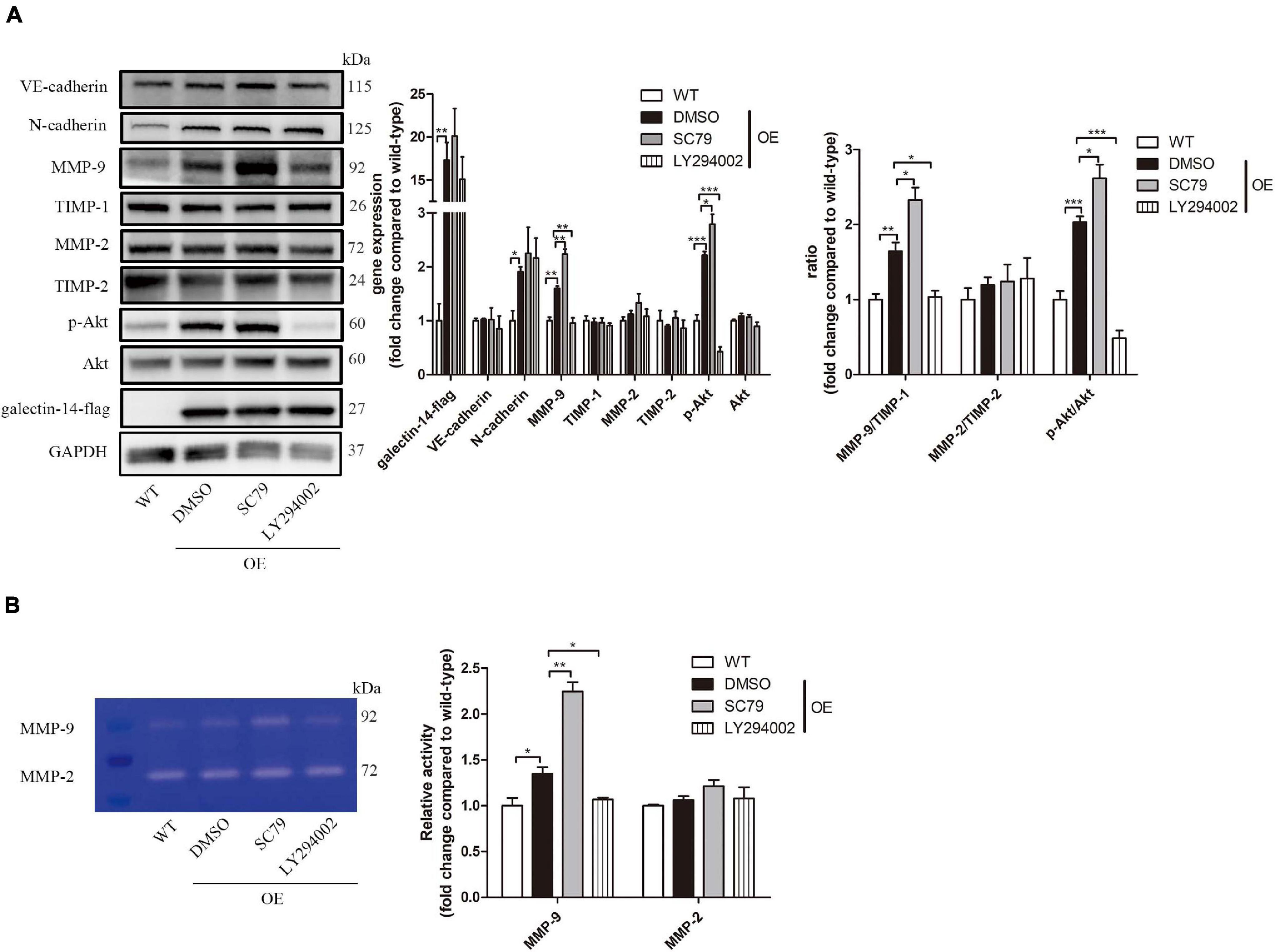
Figure 7. Galectin-14 promotes matrix metalloproteinase (MMP)-9 expression and activity through enhancing Akt phosphorylation. In galectin-14-overexpressed HTR-8/SVneo, 10 μM SC79 or 10 μM LY294002 was added as the agonist or inhibitor of Akt phosphorylation. (A) Protein expression was assessed by Western blotting with antibodies against VE-cadherin, N-cadherin, MMP-9, tissue inhibitor matrix metalloproteinase (TIMP)-1, MMP-2, TIMP-2, p-Akt, Akt, and galectin-14 and normalized to glyceraldehyde 3-phosphate dehydrogenase (GAPDH). (B) Gelatinase activity was determined by gelatin zymography. Results are presented as mean ± SEM of biological triplicates. Statistical analysis: Student’s t-test. *p < 0.05, **p < 0.01, ***p < 0.001. WT, HTR-8/SVneo transfected with empty vehicle; OE, galectin-14-overexpressed HTR-8/SVneo. Dimethylsulfoxide (DMSO), cells added with DMSO of the same volume; SC79, cells added with 10 μM SC79; LY294002, cells added with 10 μM LY294002.
Discussion
The dysfunction of trophoblasts is one of the major pathogeneses of early pregnancy loss and preeclampsia. In the current investigation, we found the decreased placental expression pattern of galectin-14 in early pregnancy loss and preeclampsia, which were consistent with previous studies (Than et al., 2014; Fritz et al., 2015; Balogh et al., 2019). These findings point to the importance of galectin-14 in these pregnancy-related diseases. We further demonstrated that galectin-14 promoted the migration and invasion of trophoblasts through enhancing the expression of MMP-9 and N-cadherin.
In the placenta, galectin-14 is expressed in STB and EVT and is necessary for the maintenance of immune tolerance at the fetal–maternal interface (Than et al., 2009, 2014; Balogh et al., 2019). However, little is known about the regulatory effects of galectin-14 on trophoblasts. In this study, the effects of galectin-14 knockdown or overexpression on trophoblast migration and invasion indicate the positive impact of galectin-14 on trophoblast migration and invasion. Successful trophoblast migration and invasion play a key role in embryonic implantation and establishment of healthy pregnancy. Thus, the dysregulation of galectin-14 in trophoblasts is involved in the pathogenesis of early pregnancy loss and preeclampsia. In addition, unlike the recombinant galectin-14 used in the previous researches, our results came from the direct knockdown of endogenic galectin-14 in primary EVTs, which are more convincing. And we further established a galectin-14-synthesizing trophoblast cell line for studies on mechanisms.
Gelatinases, including MMP-2 and MMP-9, degrade extracellular matrix (ECM) to facilitate trophoblastic migration and invasion (Shimonovitz et al., 1994). The activity of MMP-2 and MMP-9 is inhibited by TIMP-2 and TIMP-1. The different temporal expression patterns during pregnancy indicate that MMP-2 is mainly involved in embryonic implantation while MMP-9 in trophoblast invasion (Espino et al., 2017). In the placenta, MMP-9 is expressed in the EVT-rich regions (Naruse et al., 2009). In vivo, MMP-9-null mouse embryos showed deficiency of trophoblast invasion (Plaks et al., 2013). The decrease in MMP-2 and MMP-9 activity or ratios of MMP-2/TIMP-2 and MMP-9/TIMP-1 might be involved in the pathogeneses of early pregnancy loss and preeclampsia (Graham and McCrae, 1996; Choi et al., 2003; Shokry et al., 2009; Plaks et al., 2013; Gutierrez et al., 2020; Lin et al., 2020). Hereby, we found that galectin-14 promoted the expression and activity of MMP-9 and increased the MMP-9/TIMP-1 ratio while the migration and invasion of trophoblasts were enhanced.
Epithelial–mesenchymal transition (EMT) is another indispensable process for trophoblasts obtaining motility and invasion capacity. During EMT, trophoblasts lose their endothelial phenotype, loosen intercellular adhesion, and modulate cytoskeleton. The cell–cell junction proteins, such as E-cadherin, are degraded, while mesenchymal cell markers, such as N-cadherin and VE-cadherin, are upregulated (Kokkinos et al., 2010). The temporal and spatial expression of cadherins is the hallmark of trophoblast phenotype transformation, and defects in this process result in shallow trophoblast invasion, which are associated with pregnancy complications, such as early pregnancy loss and preeclampsia (Brown et al., 2005; Multhaup et al., 2018). In mice, galectin-1 improves trophoblast invasion and migration by increasing N-cadherin expression and decreasing E-cadherin expression (You et al., 2018). Herein, we observed that galectin-14 enhanced the expression of N-cadherin; meanwhile, it prompted the invasion and migration of trophoblasts, indicating that, like galectin-1, galectin-14 facilitates migration and invasion by promoting EMT in trophoblasts.
Our results indicate that galectin-14 promotes trophoblast migration and invasion through enhancing EMT and increasing the expression and activity of MMP-9. Proteomics analysis of galectin-14-overexpressed trophoblast cell line revealed the involvement of PI3K–Akt signaling pathway (data not shown). There is a significantly decreased expression of p-Akt in the villi of early pregnancy loss (Zhang et al., 2017). In our study, we found that galectin-14 promoted the phosphorylation of Akt. Increasing the phosphorylation of Akt promoted trophoblast migration and invasion and MMP-9 expression and activity, but not N-cadherin. These data suggest that galectin-14 enhances MMP-9 expression and activity through promoting Akt phosphorylation, while the mechanism through which galectin-14 promotes N-cadherin expression remains to be studied.
An in vivo research will enhance our insight into the roles of galectin-14 in pregnancy, placental development, and pathogeneses of early pregnancy loss and preeclampsia. However, there is no homologous gene of LGALS14 (the gene of galectin-14) in rat or mouse, which hammered the investigation with animal models.
In summary, galectin-14 promotes trophoblast migration and invasion by enhancing the expression of MMP-9 and N-cadherin through Akt phosphorylation (Figure 8). The dysregulation of galectin-14 is involved in the pathogenesis of early pregnancy loss and preeclampsia.
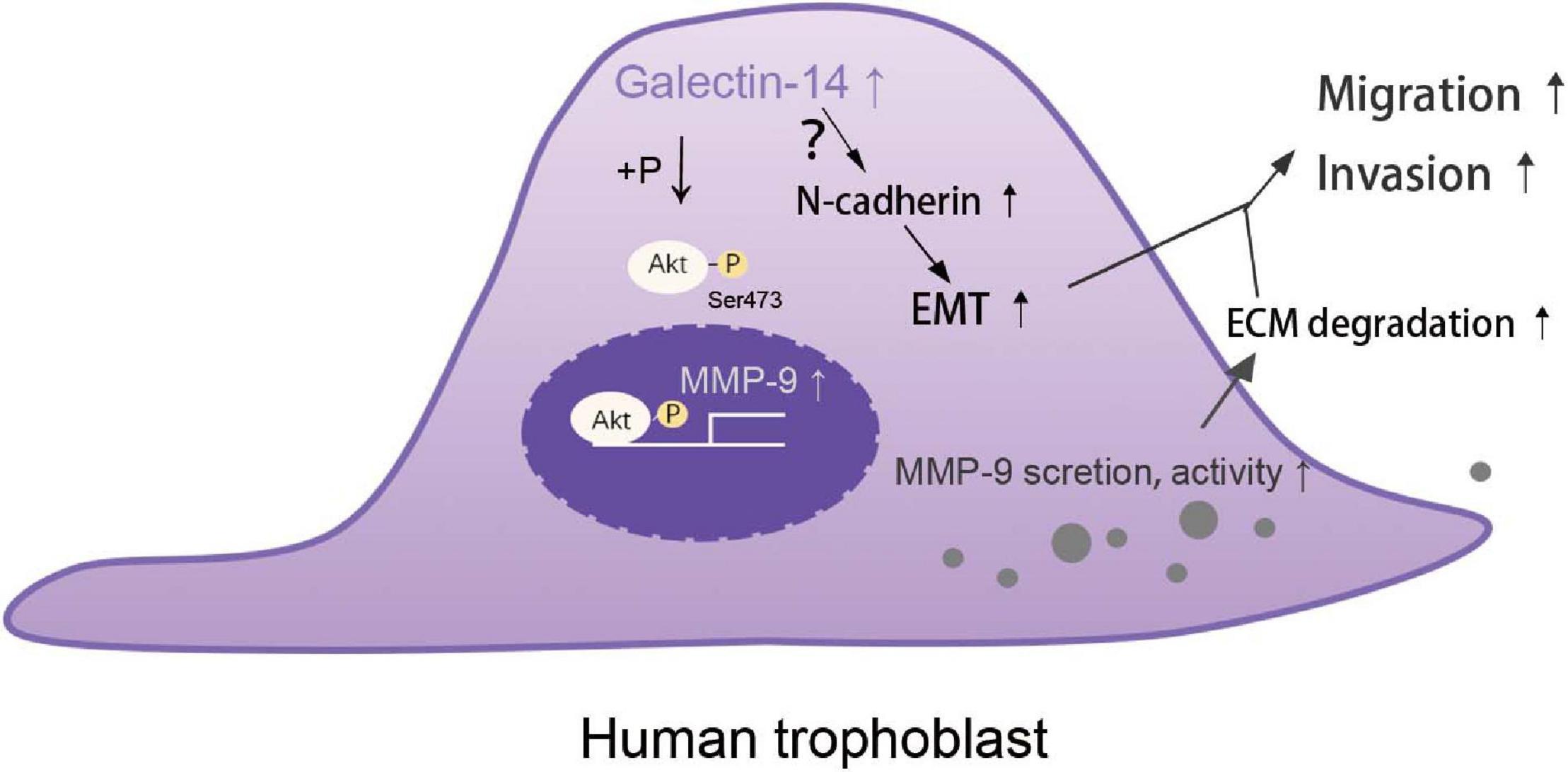
Figure 8. Schematic representation of galectin-14 enhancing trophoblast migration and invasion. The expression of galectin-14 during trophoblast differentiation promotes Akt phosphorylation, leading to increasing matrix metalloproteinase (MMP)-9 expression, secretion, and activity, thus increasing the degradation of extracellular matrix (ECM). Galectin-14 also promotes epithelial–mesenchymal transition (EMT) by upregulating N-cadherin expression. Together, the increased ECM degradation and EMT help trophoblasts obtain the capacity of migration and invasion.
Data Availability Statement
The original contributions presented in the study are included in the article/Supplementary Material, further inquiries can be directed to the corresponding author/s.
Ethics Statement
The studies involving human participants were reviewed and approved by Institutional Ethical Committee of Women’s hospital, School of Medicine, Zhejiang University. The patients/participants provided their written informed consent to participate in this study.
Author Contributions
MW, LB, and MD conceived and designed the experiments. MW, PW, YaX, and PJ performed the experiments and analyzed the data. YuX and ZW collected the samples and consents. MW wrote the draft. LB, YQ, and MD edited the manuscript. All authors contributed to the article and approved the submitted version.
Funding
This research was supported by the National Natural Science Foundation of China (No. 81370726) and the Key Research and Development Plan of Zhejiang Province (2019C03025).
Conflict of Interest
The authors declare that the research was conducted in the absence of any commercial or financial relationships that could be construed as a potential conflict of interest.
Acknowledgments
We thank Hanzhi Wang, Qi Cheng, Jiajie Shen, and Junyan Ma (Zhejinag University) for their technical assistance.
Supplementary Material
The Supplementary Material for this article can be found online at: https://www.frontiersin.org/articles/10.3389/fcell.2021.645658/full#supplementary-material
References
American College of Obstetricians and Gynecologists (2019). ACOG practice bulletin No. 202: gestational hypertension and preeclampsia. Obstet. Gynecol. 133, e1–e25. doi: 10.1097/AOG.0000000000003018
American College of Obstetricians and Gynecologists’ Committee on Practice Bulletins—Gynecology (2018). ACOG practice bulletin No. 200: early pregnancy loss. Obstet. Gynecol. 132, e197–e207. doi: 10.1097/AOG.0000000000002899
Balogh, A., Toth, E., Romero, R., Parej, K., Csala, D., Szenasi, N. L., et al. (2019). Placental galectins are key players in regulating the maternal adaptive immune response. Front. Immunol. 10:1240. doi: 10.3389/fimmu.2019.01240
Bartsch, E., Medcalf, K. E., Park, A. L., Ray, J. G., and High Risk of Pre-eclampsia Identification Group (2016). Clinical risk factors for pre-eclampsia determined in early pregnancy: systematic review and meta-analysis of large cohort studies. BMJ 353:i1753. doi: 10.1136/bmj.i1753
Blois, S. M., Dveksler, G., Vasta, G. R., Freitag, N., Blanchard, V., and Barrientos, G. (2019). Pregnancy galectinology: insights into a complex network of glycan binding proteins. Front. Immunol. 10:1166. doi: 10.3389/fimmu.2019.01166
Bolze, P. A., Mommert, M., and Mallet, F. (2017). Contribution of syncytins and other endogenous retroviral envelopes to human placenta pathologies. Prog. Mol. Biol. Transl. 145, 111–162. doi: 10.1016/bs.pmbts.2016.12.005
Brown, L. M., Lacey, H. A., Baker, P. N., and Crocker, I. P. (2005). E-cadherin in the assessment of aberrant placental cytotrophoblast turnover in pregnancies complicated by pre-eclampsia. Histochem. Cell Biol. 124, 499–506. doi: 10.1007/s00418-005-0051-7
Chen, Y., Wang, K., and Leach, R. (2013). 5-Aza-dC treatment induces mesenchymal-to-epithelial transition in 1st trimester trophoblast cell line HTR8/SVneo. Biochem. Biophys. Res. Commun. 432, 116–122. doi: 10.1016/j.bbrc.2013.01.075
Cheng, J. C., Chang, H. M., and Leung, P. C. (2013). Transforming growth factor-beta1 inhibits trophoblast cell invasion by inducing Snail-mediated down-regulation of vascular endothelial-cadherin protein. J. Biol. Chem. 288, 33181–33192. doi: 10.1074/jbc.M113.488866
Choi, H. K., Choi, B. C., Lee, S. H., Kim, J. W., Cha, K. Y., and Baek, K. H. (2003). Expression of angiogenesis- and apoptosis-related genes in chorionic villi derived from recurrent pregnancy loss patients. Mol. Reprod. Dev. 66, 24–31. doi: 10.1002/mrd.10331
Espino, Y. S. S., Flores-Pliego, A., Espejel-Nunez, A., Medina-Bastidas, D., Vadillo-Ortega, F., Zaga-Clavellina, V., et al. (2017). New insights into the role of matrix metalloproteinases in preeclampsia. Int. J. Mol. Sci. 18:1448. doi: 10.3390/ijms18071448
Freitag, N., Tirado-Gonzalez, I., Barrientos, G., Herse, F., Thijssen, V. L. J. L., Weedon-Fekjaer, S. M., et al. (2013). Interfering with Gal-1-mediated angiogenesis contributes to the pathogenesis of preeclampsia. Proc. Natl. Acad. Sci. U.S.A. 110, 11451–11456. doi: 10.1073/pnas.1303707110
Fritz, R., Kohan-Ghadr, H. R., Bolnick, J. M., Bolnick, A. D., Kilburn, B. A., Diamond, M. P., et al. (2015). Noninvasive detection of trophoblast protein signatures linked to early pregnancy loss using trophoblast retrieval and isolation from the cervix (TRIC). Fertil. Steril. 104, 339–46 e4. doi: 10.1016/j.fertnstert.2015.05.010
Graham, C. H., and McCrae, K. R. (1996). Altered expression of gelatinase and surface-associated plasminogen activator activity by trophoblast cells isolated from placentas of preeclamptic patients. Am. J. Obstet. Gynecol. 175, 555–562. doi: 10.1053/ob.1996.v175.a74404
Gutierrez, J. A., Gomez, I., Chiarello, D. I., Salsoso, R., Klein, A. D., Guzman-Gutierrez, E., et al. (2020). Role of proteases in dysfunctional placental vascular remodelling in preeclampsia. Biochim. Biophys. Acta Mol. Basis Dis. 1866:165448. doi: 10.1016/j.bbadis.2019.04.004
Huppertz, B. (2018). The critical role of abnormal trophoblast development in the etiology of preeclampsia. Curr. Pharm. Biotechnol. 19, 771–780. doi: 10.2174/1389201019666180427110547
Huppertz, B. (2020). Traditional and new routes of trophoblast invasion and their implications for pregnancy diseases. Int. J. Mol. Sci. 21:289. doi: 10.3390/ijms21010289
Jeschke, U., Mayr, D., Schiessl, B., Mylonas, I., Schulze, S., Kuhn, C., et al. (2007). Expression of Galectin-1, -3 (gal-1, gal-3) and the Thomsen-Friedenreich (TF) antigen in normal, IUGR, preeclamptic and HELLP placentas. Placenta 28, 1165–1173. doi: 10.1016/j.placenta.2007.06.006
Kokkinos, M. I., Murthi, P., Wafai, R., Thompson, E. W., and Newgreen, D. F. (2010). Cadherins in the human placenta–epithelial-mesenchymal transition (EMT) and placental development. Placenta 31, 747–755. doi: 10.1016/j.placenta.2010.06.017
Lin, C., He, H., Cui, N., Ren, Z., Zhu, M., and Khalil, R. A. (2020). Decreased uterine vascularization and uterine arterial expansive remodeling with reduced matrix metalloproteinase-2 and -9 in hypertensive pregnancy. Am. J. Physiol. Heart Circ. Physiol. 318, H165–H180. doi: 10.1152/ajpheart.00602.2019
Liu, A. X., Zhang, Y. L., Zhang, W. W., and Huang, H. F. (2006). Proteomic analysis on the alteration of protein expression in the placental villous tissue of early pregnancy loss. Fertil. Steril. 86, S38–S.
Multhaup, A., Huppertz, B., Gohner, C., Bohringer, M., Mai, M., Markert, U., et al. (2018). N-cadherin knockdown leads to disruption of trophoblastic and endothelial cell interaction in a 3D cell culture model – New insights in trophoblast invasion failure. Cell Adh. Migr. 12, 259–270. doi: 10.1080/19336918.2017.1386822
Naruse, K., Lash, G. E., Innes, B. A., Otun, H. A., Searle, R. F., Robson, S. C., et al. (2009). Localization of matrix metalloproteinase (MMP)-2, MMP-9 and tissue inhibitors for MMPs (TIMPs) in uterine natural killer cells in early human pregnancy. Hum. Reprod. 24, 553–561. doi: 10.1093/humrep/den408
Plaks, V., Rinkenberger, J., Dai, J., Flannery, M., Sund, M., Kanasaki, K., et al. (2013). Matrix metalloproteinase-9 deficiency phenocopies features of preeclampsia and intrauterine growth restriction. Proc. Natl. Acad. Sci. U.S.A. 110, 11109–11114. doi: 10.1073/pnas.1309561110
Rawn, S. M., and Cross, J. C. (2008). The evolution, regulation, and function of placenta-specific genes. Annu. Rev. Cell Dev. Biol. 24, 159–181. doi: 10.1146/annurev.cellbio.24.110707.175418
Shimonovitz, S., Hurwitz, A., Dushnik, M., Anteby, E., Gevaeldar, T., and Yagel, S. (1994). Developmental regulation of the expression of 72 and 92 Kd Type-Iv collagenases in human trophoblasts - a possible mechanism for control of trophoblast invasion. Am. J. Obstet. Gynecol. 171, 832–838. doi: 10.1016/0002-9378(94)90107-4
Shokry, M., Omran, O. M., Hassan, H. I., Elsedfy, G. O., and Hussein, M. R. A. (2009). Expression of matrix metalloproteinases 2 and 9 in human trophoblasts of normal and preeclamptic placentas: preliminary findings. Exp. Mol. Pathol. 87, 219–225. doi: 10.1016/j.yexmp.2009.08.001
Szklanna, P. B., Wynne, K., Nolan, M., Egan, K., Ainle, F. N., and Maguire, P. B. (2017). Comparative proteomic analysis of trophoblast cell models reveals their differential phenotypes, potential uses, and limitations. Proteomics 17:e1700037. doi: 10.1002/pmic.201700037
Than, N. G., Romero, R., Goodman, M., Weckle, A., Xing, J., Dong, Z., et al. (2009). A primate subfamily of galectins expressed at the maternal-fetal interface that promote immune cell death. Proc. Natl. Acad. Sci. U.S.A. 106, 9731–9736. doi: 10.1073/pnas.0903568106
Than, N. G., Romero, R., Xu, Y., Erez, O., Xu, Z., Bhatti, G., et al. (2014). Evolutionary origins of the placental expression of chromosome 19 cluster galectins and their complex dysregulation in preeclampsia. Placenta 35, 855–865. doi: 10.1016/j.placenta.2014.07.015
Turco, M. Y., and Moffett, A. (2019). Development of the human placenta. Development 146:dev163428. doi: 10.1242/dev.163428
Wan, L., Sun, D., Xie, J., Du, M., Wang, P., Wang, M., et al. (2019). Declined placental PLAC1 expression is involved in preeclampsia. Medicine 98:e17676. doi: 10.1097/MD.0000000000017676
Yan, Y., Peng, H., Wang, P., Wang, H., and Dong, M. (2016). Increased expression of fatty acid binding protein 4 in preeclamptic Placenta and its relevance to preeclampsia. Placenta 39, 94–100. doi: 10.1016/j.placenta.2016.01.014
You, J. L., Wang, W., Tang, M. Y., Ye, Y. H., Liu, A. X., and Zhu, Y. M. (2018). A potential role of galectin-1 in promoting mouse trophoblast stem cell differentiation. Mol. Cell. Endocrinol. 470, 228–239. doi: 10.1016/j.mce.2017.11.003
Keywords: early pregnancy loss, preeclampsia, epithelial-mesenchymal transition (EMT), matrix metalloproteinases (MMPs), Akt
Citation: Wang M, Xu Y, Wang P, Xu Y, Jin P, Wu Z, Qian Y, Bai L and Dong M (2021) Galectin-14 Promotes Trophoblast Migration and Invasion by Upregulating the Expression of MMP-9 and N-Cadherin. Front. Cell Dev. Biol. 9:645658. doi: 10.3389/fcell.2021.645658
Received: 23 December 2020; Accepted: 16 February 2021;
Published: 16 March 2021.
Edited by:
Wassim Abou-Kheir, American University of Beirut, LebanonReviewed by:
Hua Zhang, First Affiliated Hospital of Chongqing Medical University, ChinaNagaraj Balasubramanian, Indian Institute of Science Education and Research, India
Copyright © 2021 Wang, Xu, Wang, Xu, Jin, Wu, Qian, Bai and Dong. This is an open-access article distributed under the terms of the Creative Commons Attribution License (CC BY). The use, distribution or reproduction in other forums is permitted, provided the original author(s) and the copyright owner(s) are credited and that the original publication in this journal is cited, in accordance with accepted academic practice. No use, distribution or reproduction is permitted which does not comply with these terms.
*Correspondence: Minyue Dong, ZG9uZ215QHpqdS5lZHUuY24=