- 1Laboratory of Ocular and Systemic Autoimmune Diseases, Faculty of Medicine, Universidad de Chile, Santiago, Chile
- 2Núcleo de Ciencias Biológicas, Facultad de Estudios Interdisciplinarios, Universidad Mayor, Santiago, Chile
- 3Department de Health Science, Universidad de Aysén, Coyhaique, Chile
- 4Department of Chemical and Biological Sciences, Faculty of Health, Universidad Bernardo O’Higgins, Santiago, Chile
- 5Department of Ophthalmology, University of Chile, Santiago, Chile
- 6Faculty of Medicine, Clínica Alemana Universidad del Desarrollo, Santiago, Chile
- 7Servicio de Oftalmología, Hospital Clínico Universidad de Chile, Santiago, Chile
miRNAs, one of the members of the noncoding RNA family, are regulators of gene expression in inflammatory and autoimmune diseases. Changes in miRNA pool expression have been associated with differentiation of CD4+ T cells toward an inflammatory phenotype and with loss of self-tolerance in autoimmune diseases. Vogt–Koyanagi–Harada (VKH) disease is a chronic multisystemic pathology, affecting the uvea, inner ear, central nervous system, and skin. Several lines of evidence support an autoimmune etiology for VKH, with loss of tolerance against retinal pigmented epithelium-related self-antigens. This deleterious reaction is characterized by exacerbated inflammation, due to an aberrant TH1 and TH17 polarization and secretion of their proinflammatory hallmark cytokines interleukin 6 (IL-6), IL-17, interferon γ, and tumor necrosis factor α, and an impaired CD4+ CD25high FoxP3+ regulatory T cell function. To restrain inflammation, VKH is pharmacologically treated with corticosteroids and immunosuppressive drugs as first and second line of therapy, respectively. Changes in the expression of miRNAs related to immunoregulatory pathways have been associated with VKH development, whereas some genetic variants of miRNAs have been found to be risk modifiers of VKH. Furthermore, the drugs commonly used in VKH treatment have great influence on miRNA expression, including those miRNAs associated to VKH disease. This relationship between response to therapy and miRNA regulation suggests that these small noncoding molecules might be therapeutic targets for the development of more effective and specific pharmacological therapy for VKH. In this review, we discuss the latest evidence regarding regulation and alteration of miRNA associated with VKH disease and its treatment.
Introduction
miRNAs are short noncoding RNAs (20–23 nucleotides) that finely tune gene expression (Starega-Roslan et al., 2011). The best-known mechanism of action for gene regulation by miRNAs is post-transcriptional regulation through RISC-dependent binding or degradation of the target mRNAs, but other new functions have been described (Wu et al., 2010; Ramchandran and Chaluvally-Raghavan, 2017). Recognition of targets by the miRNA is based on the pairing of a short fragment (6–8 bases), which allows a single miRNA to bind to and regulate the activity of multiple targets, pathways, and, thus, cell function. This feature of miRNA also affects the immune system, and their dysregulation can lead to immune-related disorders such as autoimmunity.
The pathogenic role of miRNA in autoimmune diseases stems from their capability to regulate the activity of major immune-related pathways and immune cell function. For instance, Toll-like receptors (TLRs) and their signaling pathways are regulated by miRNAs, suggesting that miRNA dysregulation could reshape response toward endogenous DAMPs and foster autoimmunity (Nahid et al., 2011; He et al., 2014). Moreover, aberrant miRNA expression disrupts regulatory T cell (Treg) and tolerogenic dendritic cell function and phenotype stability (Li et al., 2014; Wu et al., 2018; Dekkema et al., 2019; Lyszkiewicz et al., 2019; Zhang et al., 2019; Chen et al., 2020; Geng et al., 2020; Tang et al., 2020). Involvement of miRNA in autoimmune diseases is also supported by the correlation of miRNA levels and many disease biomarkers. Let-7f expression is reduced in active systemic lupus erythematosus (SLE) and negatively correlates with disease activity index and proteinuria (Geng et al., 2020), whereas circulating exosomal miR-21 and miR-146a correlate with anti-SSA and anti-dsDNA levels, respectively (Li et al., 2020). Several miRNAs correlate with anti-citrullinated peptide antibodies levels and disease activity score in patients with rheumatoid arthritis (RA) (De La Rosa et al., 2020). miRNA may promote autoimmunity through direct binding to TLR-7/8 (Kim et al., 2016; Hegewald et al., 2020); therefore, general overexpression of miRNA may facilitate self-tolerance failure through mechanisms other than regulation on gene expression. In summary, miRNAs contribute to autoimmunity through the disruption of immune-related pathways, impairment of regulatory cell phenotype, or mounting immune response through TLR engagement.
Vogt–Koyanagi–Harada Disease
Vogt–Koyanagi–Harada (VKH) disease is a rare autoimmune disease with ocular and systemic compromise. Ocular manifestations are characterized mainly by severe bilateral granulomatous panuveitis, exudative retinal detachments, and optic nerve edema, with eventual development of ocular pigmentary changes as late features in advanced phases of the disease. Systemic symptoms include tinnitus, hearing loss, vertigo, meningismus, vitiligo, and poliosis (O’keefe and Rao, 2017). A recent study suggests that delayed diagnosis and inadequate treatment lead to iris deterioration in patients with chronic recurrent disease (Chee and Win, 2021). VKH disease generally affects young women and is the leading cause of noninfectious uveitis with a known etiological factor in many high-risk populations, including India, Thailand, and Chile, and a major cause of panuveitis in Tunisia, Iran, Japan, and the Hispanic population in the United States (Liberman et al., 2015; O’keefe and Rao, 2017).
VKH etiopathogenesis is only partially understood; however, several studies provide evidence that the disease is caused by the immune reaction against pigmented cell–related autoantigens (Kobayashi et al., 1998; Otani et al., 2006). Therefore, understanding the mechanisms of immune dysregulation in the context of VKH is necessary to create more specific and effective therapies.
CD4+ T Cells and Their Role in VKH Pathogenesis
As an autoimmune disorder, immune response against self is an underlying pathogenic mechanism of VKH, leading to the destruction and functional impairment of the retinal pigment epithelium and adjacent layers of the eye. Failure of tolerogenic mechanisms lead to the activation of self-reactive immune cells, including CD4+ T cells, which are major contributors to VKH. Increased CD4+ T cell population has been observed in the aqueous humor and cerebrospinal fluid of VKH patients (Norose et al., 1990, 1994; Ohta and Yoshimura, 1998). Initial phenotypical characterization of CD4+ T cells in VKH revealed an immune response shifted toward the TH1 subset with increased expression of activation markers CD25 and HLA-DR, the proinflammatory cytokine interferon γ (IFN-γ), and the transcription factor T-Bet (Norose and Yano, 1996; Li et al., 2005; Sugita et al., 2006). Notably, these TH1 cells have cytolytic activity on melanoma cells and cells expressing peptides related with pigmented tissues and express memory T cell markers, suggesting that loss of tolerance toward pigmented epithelium and long-term TH1 response are a crucial factor in VKH pathogenesis (Norose and Yano, 1996; Sugita et al., 2006). The introduction of the TH17 subset expanded the knowledge of the role of CD4+ T cells in autoimmunity. Interleukin 23 (IL-23) is a cytokine of the IL-12 family that induces differentiation of CD4+ T cells into the TH17 subpopulation to secrete the hallmark cytokine IL-17. IL-23 is increased in patients with active VKH (Wang et al., 2018) and is associated with active uveitis (Chi et al., 2008; Przepiera-Bedzak et al., 2016; Velez et al., 2016), and its administration enhances IFN-γ and IL-17 secretion in peripheral blood mononuclear cells (PBMCs) and isolated CD4+ T cells in vitro (Chi et al., 2007). Treatment-induced remission is associated with decreased expression of TH1 and TH17 cytokines and related transcription factors in PBMCs and CD4+ T cells (Liu et al., 2009).
Treg-inducing mechanisms seem to be defective in VKH; in a study published by Commodaro et al. (2010), no difference in the frequency of circulating Treg between controls and VKH patients, with or without active disease, was found. However, IL-10 and transforming growth factor β secretion was significantly stronger in the PBMCs from inactive VKH patients after in vitro stimulation, whereas IFN-γ was higher in active patients, without differences between control and inactive VKH groups (Commodaro et al., 2010). This study suggests that function, rather than number of Tregs, and maybe other regulatory cells, is impaired in VKH patients with active disease. In agreement with this, serum levels of the immunoregulatory cytokine IL-27 are decreased in patients with active VKH, which suppresses IL-17 expression and promotes IL-10 secretion in naive CD4+ T cells (Wang et al., 2012). Another immunoregulatory cytokine, IL-35, is also decreased in VKH patients, and culturing PBMCs with anti-CD3 and anti-CD28 antibodies in presence of IL-35 inhibits secretion of IFN-γ and IL-17 but enhances IL-10 release (Hu et al., 2019). Altogether, data show that deregulation of CD4+ T cells is an important event in VKH pathophysiology; therefore, understanding the mechanisms that cause these changes might be key for the development of effective therapies for this disease.
Current Pharmacological Therapies for VKH
Systemic corticosteroids (CSs) (prednisolone) are the mainstay of clinical management of VKH, with evidence endorsing the use of high doses at early phases resulting in shorter treatment periods, reduced disease severity, and better subclinical manifestations (Chee et al., 2007; Jap et al., 2008; Kitaichi et al., 2008; Kawaguchi et al., 2010), whereas CS administration for at least 6 months is key to reduce the risk of recurrence (Lai et al., 2009; Errera et al., 2011). CS therapy is known to have several side effects, including systemic (diabetes, Cushing syndrome, osteoporosis) and eye-related features (cataract, glaucoma, visual impairment) (Valenzuela et al., 2020b).
Immunomodulatory therapy (IMT), including mycophenolate mofetil (MMF), methotrexate (MTX), cyclosporin A (CsA), and azathioprine, is usually used as a CS-sparing treatment with successful visual acuity improvement and reduction of sunset glow fundus development in some reports (Agarwal et al., 2006; Shen et al., 2016; Abu El-Asrar et al., 2017; Yang et al., 2018; Ei Ei Lin et al., 2020). Early use of CS and IMT combined as a first-line therapy increases the chances of remission and lowers the risk of chronic disease compared with CS monotherapy (Herbort et al., 2017, 2019).
Biologics have been introduced in uveitis management, and guidelines recommend them upon systemic CS/IMT treatment failure (Rosenbaum et al., 2019; Valenzuela et al., 2020b). Studies have shown that the use of adalimumab [anti-tumor necrosis factor α (TNF-α) antibody] (Couto et al., 2018; Hiyama et al., 2021) and rituximab (anti-CD20 antibody) (Abu El-Asrar et al., 2020) improves visual acuity, alleviates inflammation, and allows for CS tapering. Case reports have shown favorable results for the use of infliximab (anti-TNF-α) (Wang and Gaudio, 2008; Zmuda et al., 2013) and intravitreal bevacizumab (anti-VEGF-A) (Wu et al., 2009; Park et al., 2011) as treatment of VKH.
miRNAs and Their Role in VKH Etiology and Treatment
miRNAs as Mediators of VKH Pathogenesis
miRNAs have been implicated in the development of VKH disease. Asakage and colleagues recently reported their results on differentially expressed miRNAs (DEmiRs) in the serum of patients with noninfectious uveitis, including VKH, using a microarray approach (Asakage et al., 2020). The results revealed a set of 188 DEmiRs in VKH patients when compared with healthy controls (HCs), of which 59 DEmiRs were unique to VKH when compared with sarcoidosis and Behçet’s disease (BD). The authors used several approaches such as unsupervised hierarchical analysis and principal component analysis to show that VKH is related with a distinctive miRNA expression profile compared with uveitis of different etiology. Differential expression and copy number variation (CNV) in several miRNAs between VKH and BD have been described, probably due to the difference in the nature of these immunological disorders (autoimmune/adaptive in VKH vs. autoinflammatory/innate in BD) (Qi et al., 2013; Zhou et al., 2014; Hou et al., 2016). This distinctive miRNA expression pattern suggests that a specific miRNA-mediated mechanism is central to VKH pathogenesis. A summary of the findings and a brief discussion on the role of miRNAs involved in VKH are provided in Table 1.
miR-20a: Patients with active VKH have lower expression of miR-20a-5p in CD4+ T cells compared to HC, which is associated with a hypermethylated miR-20a-5p promoter (Chang et al., 2018). Overexpression of miR-20a-5p indirectly decreases IL-17 expression in VKH CD4+ T cells through the regulation of oncostatin M and CCL1 expression (Chang et al., 2018). Accordingly, a comprehensive analysis based on literature-supported miRNA-mRNA interactions found that miR-20a may suppress TH17 differentiation through the targeting of several regulators (Honardoost et al., 2015). Moreover, miR-20a expression increases upon T cell activation and inhibits T cell receptor signaling, while also decreasing the expression of CD69, IL-2, IL-8, and, especially, IL-10 (Reddycherla et al., 2015). Altogether, evidence suggests that miR-20a participates in a negative feedback loop that modulates CD4+ T cell activation and polarization.
miR-23a: A high copy number (>2) of the miR-23a coding gene is linked to VKH. This CNV directly correlates with increased miR-23a expression in PBMCs from HC, whereas overexpression of miR-23a increases IL-6 production in human retinal pigment epithelial cells (Hou et al., 2016). Conversely, miR-23a has been shown to restrain IL-17-mediated response by inhibiting the nuclear factor κB pathway (Hu et al., 2017) and to facilitate the expression of the immunoregulatory enzyme HO-1 by targeting its inhibitor Bach-1 (Su et al., 2020). Thus, miR-23a might act as a balancing factor in inflammation with opposing proinflammatory and anti-inflammatory roles. It is possible that the effect of miR-23a depends on the tissue it is expressed or is modified by the inflammatory milieu.
miR-146a: High miR-146a encoding gene copy number has been linked to VKH disease (Hou et al., 2016). However, no association with several miR-146 single-nucleotide polymorphisms (SNPs) was found in a similar study performed by the same authors (Zhou et al., 2014). The C allele of one of these SNPs (rs2910164 C > G) impairs nuclear processing of the pri-miR-146a, leading to lower mature miR-146a expression in PBMCs (Jazdzewski et al., 2008; Zhou et al., 2014), indicating that it has a functional impact. The lack of association of SNPs with VKH and the increased number of miR-146a encoding gene copies in these patients suggest that an aberrant overexpression of mature miR-146a, not its down-regulation, may have a role in the disease. Evidence supports a tolerogenic effect of miR-146a by enhancing Treg function (Lu et al., 2010; Zhou et al., 2015) and impairing TH17 differentiation (Liu et al., 2016; Li et al., 2017). How a high CNV of miR-146a gene is linked to VKH is still unknown.
miR-182: The rs76481776 SNP, located in the MIR182 gene, is associated with a limited expression of mature miR-182 in CC in versus TT or CT genotypes (Saus et al., 2010). A significant association with VKH was found for the C allele but not the T allele of the rs76481776 SNP in a Han Chinese cohort (Yu et al., 2014). Accordingly, the authors also report that CD4+ T cells from HC with the CC genotype have a lower expression of mature miR-182 compared with cells from donors carrying at least one T allele (Yu et al., 2014). Given the association of the C allele with VKH, the evidence suggests that miR-182 has protective role.
miR-301a: A low copy number of the MIR301A gene is associated with VKH in the Han Chinese population (Hou et al., 2016). Literature shows that miR-301a promotes TH17 differentiation and TNF-α production by targeting SNIP1 and PIAS3 (Mycko et al., 2012; He et al., 2016). There seems to be a contradiction between the low copy number and the proinflammatory effects of miR-301a in the context of VKH, although the relationship between CNV and expression has not been evaluated. A low copy number could sustain enough miR-301a expression without the activation of compensating negative feedback mechanisms, promoting inflammation.
Let-7g-3p: In one study, the authors identified a predictive panel of 24 miRNA in VKH patients, with let-7g-3p being the best predictor (Asakage et al., 2020), suggesting a strong link with disease development. A decreased expression of circulating let-7g-3p was found in Graves disease patients in remission (Hiratsuka et al., 2016). However, most of the knowledge on the immunoregulatory effects of let-7g is related with let-7g-5p (Yang et al., 2020). The role of let-7g-3p in VKH is still unclear.
miRNA in Therapeutic Response
The current pharmacologic treatment for VKH includes immunosuppressive and immunomodulatory drugs, which are known to modify the expression of some of the previously reported VKH-related miRNA, accordingly to in vitro, animal model, and human studies evidence.
Corticosteroids: The expression of miR-20a, or the cluster miR17-92 in which it is located, is down-regulated by CS in mice lung tissue and murine T-cell lymphoma cell line (Moschos et al., 2007; Molitoris et al., 2011). The same effect is seen in miR-301a expression after CS stimulation (Moschos et al., 2007). Intracellular level of miR-23a is also down-regulated by dexamethasone (Dex) in both human endothelial cells and C2C12 myotubes, although the mechanism seems to be different as the one for miR-20a and miR-301a (Hudson et al., 2014b; Kwok et al., 2017). The use of Dex reverts the up-regulation of miR-146 induced by TNF-α in human bronchial epithelial cells in vitro and also in serum of pediatric patients with Crohn disease (Heier et al., 2016; Lambert et al., 2018). Finally, Dex decreases the level of miR-182 in preadipocytes, allowing C/EBPα-driven adipocyte differentiation (Dong et al., 2020).
MMF: The active metabolite of MMF, mycophenolic acid, is known to up-regulate miR-146a in T cells from SLE patients after treatment, according to microarray-based analysis and reverse transcription–quantitative polymerase chain reaction analysis (Tang et al., 2015).
MTX: RA patients who exhibit clinical improvement have higher blood levels of miR-146a-5p and other miRNAs at 4 months after MTX treatment initiation, supporting a mechanistic link between miR-146a expression and therapeutic response to MTX (Singh et al., 2019).
CsA: CsA-induced gingivae growth in rats occurs together with an up-regulation of miR-23a (Yang et al., 2017). The same effects over miR-23a expression are seen in primary mouse hepatocytes treated with CsA in vitro (Van Den Hof et al., 2014).
Adalimumab: A significant down-regulation of miR-146a-5p was observed in PBMCs from psoriasis patients after adalimumab treatment, reaching levels compared to that of the HC group (Mensa et al., 2018). Moreover, adalimumab reduced the expression of miR-146a in THP-1 and endothelial cells in vitro (Prattichizzo et al., 2016).
It is important to remark that none of the aforementioned publications established a direct relationship between changes of the miRNA expression and the therapeutic actions of the VKH-related drugs. How these drugs modify the levels of miRNAs is still under investigation, although the mechanisms behind might include (i) impairment of miRNA maturation, as CS down-regulates Dicer, Drosha, and DGCR8/Pasha and also induces G3BP1, all of them key miRNA processing enzymes (Smith et al., 2010; Kwok et al., 2017; Clayton et al., 2018); (ii) increasing exocytosis of miRNAs, making them less available intracellularly (Hudson et al., 2014a,b); (iii) histone modification in the promoter of miRNA genes by action of MMF or its active metabolite (Tang et al., 2015; Yang et al., 2015); and (iv) miRNA gene expression by indirect mechanisms that include extracellular adenosine signaling after MTX treatment (Yang et al., 2021). Less known are the mechanisms for CsA and adalimumab, although both drugs modify the profile expression of a number of different miRNAs (Gooch et al., 2017; Wcislo-Dziadecka et al., 2018).
A different but relevant aspect in the pharmacological treatment of VKH is the refractoriness to CS treatment [CS resistance (CSR)]. Recently, our group published a systematic review about CSR, a crucial issue in the management of uveitides such as VKH, which can be broadly described as refractory uveal inflammation despite the administration of high dose of CS (Valenzuela et al., 2020a). The VKH-related miRNA miR-182 confers CSR inhibiting apoptosis in lymphoma cells (Yang et al., 2012) and prevents CS-induced atrophy of skeletal muscle by targeting FOXO3a (Hudson et al., 2014a). Most data on CSR-related miRNAs involve molecules with unknown relationship with VKH; a summary of these findings is provided next as it could guide future research (Table 2). Evidence shows that a shift in the relative expression of the GR isoforms α and β after CS treatment constitutes a marker for CS sensitivity (Urzua et al., 2017, 2019). In this regard, miR-130b overexpression was found to inhibit GRα expression and conferred CSR to multiple myeloma cells (Tessel et al., 2011). Similarly, transfection of miR-331-3p mimic promotes CS sensitivity in several transformed cell lines by inhibiting JKN phosphorylation (Lucafo et al., 2020). Other pro-CSR miRNAs are miR-21, miR-29a and miR-222; conversely, miR-15b, miR-16, and miR-128b promote CS sensitivity in cancer cells (Kotani et al., 2009; Rainer et al., 2009; Wang et al., 2011; Glantschnig et al., 2019; Xu et al., 2019). Evidence for both pro- and anti-CSR effects of miR-221 and miR-124 has been reported (Kotani et al., 2009; Lv et al., 2012; Kim et al., 2015; Liang et al., 2017; Xu et al., 2019). Although CSR is a slightly different concept in the context of cancer, it also involves impairment of pharmacological response to CS; hence, some of these miRNAs might be involved in CSR in VKH patients as well.
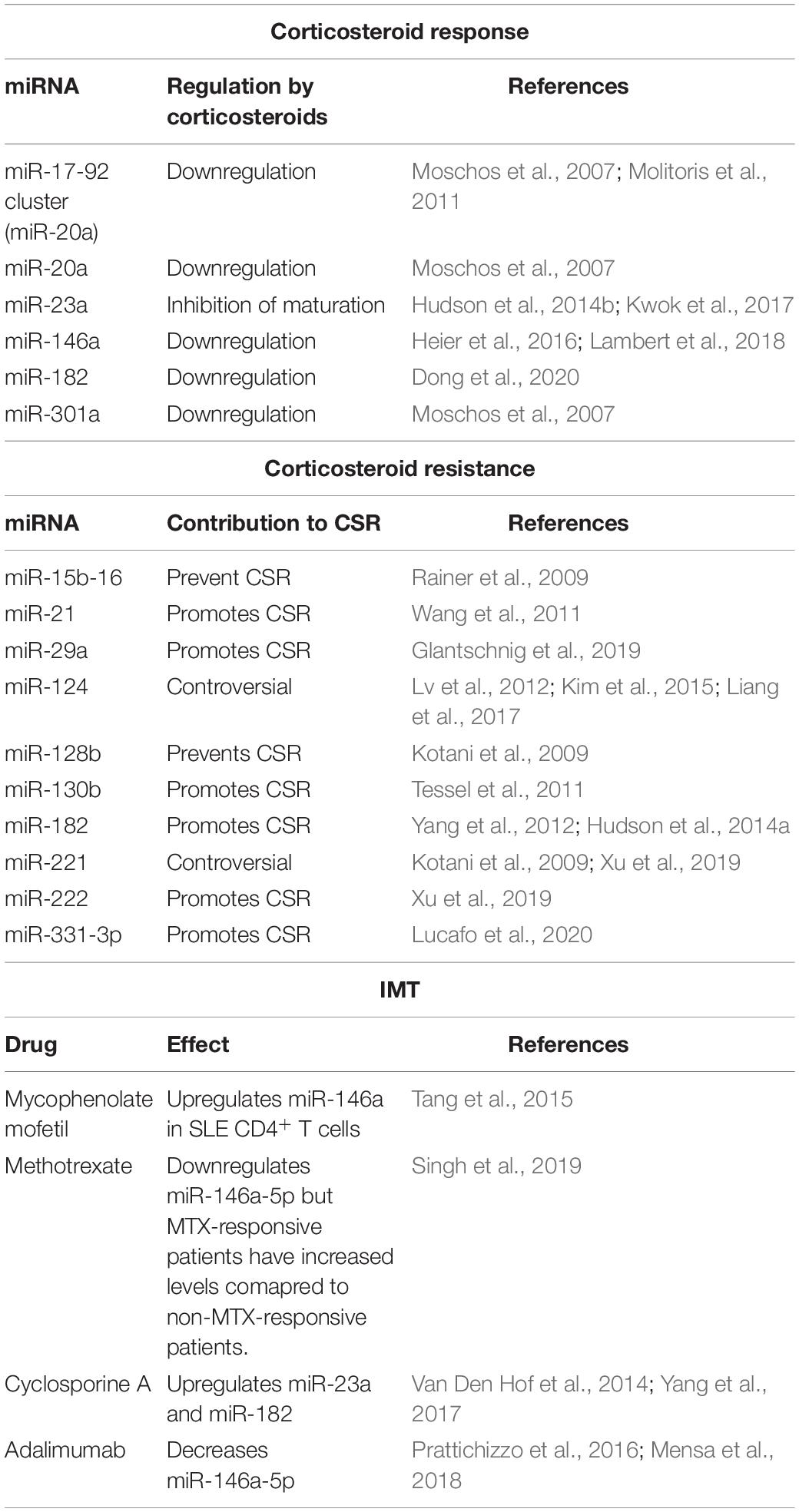
Table 2. miRNAs involved in response to pharmacological treatment in VKH and corticosteroid resistance.
Conclusion
VKH is a complex disease with incompletely understood etiopathogenesis that requires aggressive long-term CS treatment with a high risk of wasting side effects. Although scarce, evidence supports a role of miRNAs in the development of VKH disease, therapeutic response, and even therapy resistance. Future research in the subject must aim to not only find the association of miRNAs with VKH and the use of therapy but also determine potential targets and functional changes caused by the differential expression of these regulatory RNAs. Successful progress in this task will contribute to establishing new pharmacological targets and biomarkers for disease activity and therapy response.
Author Contributions
FV wrote the manuscript. MB, FV, RV, CU, and LC read, discussed, and revised the manuscript. All authors listed have made a substantial, direct and intellectual contribution to the work, and approved it for publication.
Funding
This study was supported by the National Agency for Research and Development (ANID) grant Fondecyt de Iniciación en Investigación No. 11191215 (given to LC) and Fondo de Fomento al Desarrollo Científico y Tecnológico (FONDEF) grant No. IT17I0087 (given to CU).
Conflict of Interest
The authors declare that the research was conducted in the absence of any commercial or financial relationships that could be construed as a potential conflict of interest.
References
Abu El-Asrar, A. M., Dheyab, A., Khatib, D., Struyf, S., Van Damme, J., and Opdenakker, G. (2020). Efficacy of B cell depletion therapy with rituximab in refractory chronic recurrent uveitis associated with vogt-koyanagi-harada disease. Ocul. Immunol. Inflamm. doi: 10.1080/09273948.2020.1820531 [Epub ahead of print].
Abu El-Asrar, A. M., Dosari, M., Hemachandran, S., Gikandi, P. W., and Al-Muammar, A. (2017). Mycophenolate mofetil combined with systemic corticosteroids prevents progression to chronic recurrent inflammation and development of ‘sunset glow fundus’ in initial-onset acute uveitis associated with Vogt-Koyanagi-Harada disease. Acta Ophthalmol. 95, 85–90. doi: 10.1111/aos.13189
Agarwal, M., Ganesh, S. K., and Biswas, J. (2006). Triple agent immunosuppressive therapy in Vogt-Koyanagi-Harada syndrome. Ocul. Immunol. Inflamm. 14, 333–339. doi: 10.1080/09273940600976938
Asakage, M., Usui, Y., Nezu, N., Shimizu, H., Tsubota, K., Yamakawa, N., et al. (2020). Comprehensive miRNA analysis using serum from patients with noninfectious uveitis. Invest. Ophthalmol. Vis. Sci. 61:4. doi: 10.1167/iovs.61.11.4
Chang, R., Yi, S., Tan, X., Huang, Y., Wang, Q., Su, G., et al. (2018). MicroRNA-20a-5p suppresses IL-17 production by targeting OSM and CCL1 in patients with Vogt-Koyanagi-Harada disease. Br. J. Ophthalmol. 102, 282–290. doi: 10.1136/bjophthalmol-2017-311079
Chee, S. P., Jap, A., and Bacsal, K. (2007). Spectrum of Vogt-Koyanagi-Harada disease in Singapore. Int. Ophthalmol. 27, 137–142. doi: 10.1007/s10792-006-9009-6
Chee, S. P., and Win, M. Z. A. (2021). Iris manifestations in inadequately treated chronic recurrent Vogt-Koyanagi-Harada Disease. Ocul. Immunol. Inflamm. doi: 10.1080/09273948.2020.1870701 [Epub ahead of print].
Chen, L., Hou, X., Zhang, M., Zheng, Y., Zheng, X., Yang, Q., et al. (2020). MicroRNA-223-3p modulates dendritic cell function and ameliorates experimental autoimmune myocarditis by targeting the NLRP3 inflammasome. Mol. Immunol. 117, 73–83. doi: 10.1016/j.molimm.2019.10.027
Chi, W., Yang, P., Li, B., Wu, C., Jin, H., Zhu, X., et al. (2007). IL-23 promotes CD4+ T cells to produce IL-17 in Vogt-Koyanagi-Harada disease. J. Allergy Clin. Immunol. 119, 1218–1224. doi: 10.1016/j.jaci.2007.01.010
Chi, W., Zhu, X., Yang, P., Liu, X., Lin, X., Zhou, H., et al. (2008). Upregulated IL-23 and IL-17 in Behcet patients with active uveitis. Invest. Ophthalmol. Vis. Sci. 49, 3058–3064. doi: 10.1167/iovs.07-1390
Clayton, S. A., Jones, S. W., Kurowska-Stolarska, M., and Clark, A. R. (2018). The role of microRNAs in glucocorticoid action. J. Biol. Chem. 293, 1865–1874. doi: 10.1074/jbc.R117.000366
Commodaro, A. G., Peron, J. P., Genre, J., Arslanian, C., Sanches, L., Muccioli, C., et al. (2010). IL-10 and TGF-beta immunoregulatory cytokines rather than natural regulatory T cells are associated with the resolution phase of Vogt-Koyanagi-Harada (VKH) syndrome. Scand. J. Immunol. 72, 31–37. doi: 10.1111/j.1365-3083.2010.02401.x
Couto, C., Schlaen, A., Frick, M., Khoury, M., Lopez, M., Hurtado, E., et al. (2018). Adalimumab treatment in patients with Vogt-Koyanagi-Harada disease. Ocul. Immunol. Inflamm. 26, 485–489. doi: 10.1080/09273948.2016.1236969
De La Rosa, I. A., Perez-Sanchez, C., Ruiz-Limon, P., Patino-Trives, A., Torres-Granados, C., Jimenez-Gomez, Y., et al. (2020). Impaired microRNA processing in neutrophils from rheumatoid arthritis patients confers their pathogenic profile. Modulation by biological therapies. Haematologica 105, 2250–2261. doi: 10.3324/haematol.2018.205047
Dekkema, G. J., Bijma, T., Jellema, P. G., Van Den Berg, A., Kroesen, B. J., Stegeman, C. A., et al. (2019). Increased miR-142-3p expression might explain reduced regulatory T cell function in granulomatosis with polyangiitis. Front. Immunol. 10:2170. doi: 10.3389/fimmu.2019.02170
Dong, M., Ye, Y., Chen, Z., Xiao, T., Liu, W., and Hu, F. (2020). MicroRNA 182 is a novel negative regulator of adipogenesis by targeting CCAAT/Enhancer-binding protein alpha. Obesity (Silver Spring) 28, 1467–1476. doi: 10.1002/oby.22863
Ei Ei Lin, O., Chee, S. P., Wong, K. K. Y., and Hla Myint, H. (2020). Vogt-Koyanagi-Harada disease managed with immunomodulatory therapy within 3 months of disease onset. Am. J. Ophthalmol. 220, 37–44. doi: 10.1016/j.ajo.2020.07.036
Errera, M. H., Fardeau, C., Cohen, D., Navarro, A., Gaudric, A., Bodaghi, B., et al. (2011). Effect of the duration of immunomodulatory therapy on the clinical features of recurrent episodes in Vogt–Koyanagi–Harada disease. Acta Ophthalmol. 89, e357–e366. doi: 10.1111/j.1755-3768.2010.02055.x
Geng, L., Tang, X., Wang, S., Sun, Y., Wang, D., Tsao, B. P., et al. (2020). Reduced Let-7f in bone marrow-derived mesenchymal stem cells triggers Treg/Th17 imbalance in patients with systemic lupus erythematosus. Front Immunol. 11:233. doi: 10.3389/fimmu.2020.00233
Glantschnig, C., Koenen, M., Gil-Lozano, M., Karbiener, M., Pickrahn, I., Williams-Dautovich, J., et al. (2019). A miR-29a-driven negative feedback loop regulates peripheral glucocorticoid receptor signaling. FASEB J. 33, 5924–5941. doi: 10.1096/fj.201801385RR
Gooch, J. L., King, C., Francis, C. E., Garcia, P. S., and Bai, Y. (2017). Cyclosporine a alters expression of renal microRNAs: new insights into calcineurin inhibitor nephrotoxicity. PLoS One 12:e0175242. doi: 10.1371/journal.pone.0175242
He, C., Shi, Y., Wu, R., Sun, M., Fang, L., Wu, W., et al. (2016). miR-301a promotes intestinal mucosal inflammation through induction of IL-17A and TNF-alpha in IBD. Gut 65, 1938–1950. doi: 10.1136/gutjnl-2015-309389
He, X., Jing, Z., and Cheng, G. (2014). MicroRNAs: new regulators of Toll-like receptor signalling pathways. Biomed. Res. Int. 2014, 945169. doi: 10.1155/2014/945169
Hegewald, A. B., Breitwieser, K., Ottinger, S. M., Mobarrez, F., Korotkova, M., Rethi, B., et al. (2020). Extracellular miR-574-5p induces osteoclast differentiation via TLR 7/8 in Rheumatoid arthritis. Front. Immunol. 11:585282. doi: 10.3389/fimmu.2020.585282
Heier, C. R., Fiorillo, A. A., Chaisson, E., Gordish-Dressman, H., Hathout, Y., Damsker, J. M., et al. (2016). Identification of pathway-specific serum biomarkers of response to glucocorticoid and infliximab treatment in children with inflammatory bowel disease. Clin. Transl. Gastroenterol. 7:e192. doi: 10.1038/ctg.2016.49
Herbort, C. P. Jr., Abu El Asrar, A. M., Takeuchi, M., Pavesio, C. E., Couto, C., Hedayatfar, A., et al. (2019). Catching the therapeutic window of opportunity in early initial-onset Vogt-Koyanagi-Harada uveitis can cure the disease. Int. Ophthalmol. 39, 1419–1425. doi: 10.1007/s10792-018-0949-4
Herbort, C. P. Jr., Abu El Asrar, A. M., Yamamoto, J. H., Pavesio, C. E., Gupta, V., Khairallah, M., et al. (2017). Reappraisal of the management of Vogt-Koyanagi-Harada disease: sunset glow fundus is no more a fatality. Int. Ophthalmol. 37, 1383–1395. doi: 10.1007/s10792-016-0395-0
Hiratsuka, I., Yamada, H., Munetsuna, E., Hashimoto, S., and Itoh, M. (2016). Circulating microRNAs in Graves’ disease in relation to clinical activity. Thyroid 26, 1431–1440. doi: 10.1089/thy.2016.0062
Hiyama, T., Harada, Y., and Kiuchi, Y. (2021). Efficacy and safety of adalimumab therapy for the treatment of non-infectious uveitis: efficacy comparison among uveitis aetiologies. Ocul. Immunol. Inflamm. doi: 10.1080/09273948.2020.1857791 [Epub ahead of print].
Honardoost, M. A., Naghavian, R., Ahmadinejad, F., Hosseini, A., and Ghaedi, K. (2015). Integrative computational mRNA-miRNA interaction analyses of the autoimmune-deregulated miRNAs and well-known Th17 differentiation regulators: an attempt to discover new potential miRNAs involved in Th17 differentiation. Gene 572, 153–162. doi: 10.1016/j.gene.2015.08.043
Hou, S., Ye, Z., Liao, D., Bai, L., Liu, Y., Zhang, J., et al. (2016). miR-23a, miR-146a and miR-301a confer predisposition to Vogt-Koyanagi-Harada syndrome but not to Behcet’s disease. Sci. Rep. 6:20057. doi: 10.1038/srep20057
Hu, J., Qin, Y., Yi, S., Wang, C., Yang, J., Yang, L., et al. (2019). Decreased interleukin(IL)-35 expression is associated with active intraocular inflammation in Vogt-Koyanagi-Harada (VKH) disease. Ocul. Immunol. Inflamm. 27, 595–601. doi: 10.1080/09273948.2018.1433306
Hu, J., Zhai, C., Hu, J., Li, Z., Fei, H., Wang, Z., et al. (2017). MiR-23a inhibited IL-17-mediated proinflammatory mediators expression via targeting IKKalpha in articular chondrocytes. Int. Immunopharmacol. 43, 1–6. doi: 10.1016/j.intimp.2016.11.031
Hudson, M. B., Rahnert, J. A., Zheng, B., Woodworth-Hobbs, M. E., Franch, H. A., and Price, S. R. (2014a). miR-182 attenuates atrophy-related gene expression by targeting FoxO3 in skeletal muscle. Am. J. Physiol. Cell Physiol. 307, C314–C319. doi: 10.1152/ajpcell.00395.2013
Hudson, M. B., Woodworth-Hobbs, M. E., Zheng, B., Rahnert, J. A., Blount, M. A., Gooch, J. L., et al. (2014b). miR-23a is decreased during muscle atrophy by a mechanism that includes calcineurin signaling and exosome-mediated export. Am. J. Physiol. Cell Physiol. 306, C551–C558. doi: 10.1152/ajpcell.00266.2013
Jap, A., Luu, C. D., Yeo, I., and Chee, S. P. (2008). Correlation between peripapillary atrophy and corticosteroid therapy in patients with Vogt-Koyanagi-Harada disease. Eye (Lond.) 22, 240–245. doi: 10.1038/sj.eye.6702591
Jazdzewski, K., Murray, E. L., Franssila, K., Jarzab, B., Schoenberg, D. R., and De La Chapelle, A. (2008). Common SNP in pre-miR-146a decreases mature miR expression and predisposes to papillary thyroid carcinoma. Proc. Natl. Acad. Sci. U.S.A. 105, 7269–7274. doi: 10.1073/pnas.0802682105
Kawaguchi, T., Horie, S., Bouchenaki, N., Ohno-Matsui, K., Mochizuki, M., and Herbort, C. P. (2010). Suboptimal therapy controls clinically apparent disease but not subclinical progression of Vogt-Koyanagi-Harada disease. Int. Ophthalmol. 30, 41–50. doi: 10.1007/s10792-008-9288-1
Kim, J., Jeong, D., Nam, J., Aung, T. N., Gim, J. A., Park, K. U., et al. (2015). MicroRNA-124 regulates glucocorticoid sensitivity by targeting phosphodiesterase 4B in diffuse large B cell lymphoma. Gene 558, 173–180. doi: 10.1016/j.gene.2015.01.001
Kim, S. J., Chen, Z., Essani, A. B., Elshabrawy, H. A., Volin, M. V., Volkov, S., et al. (2016). Identification of a novel toll-like receptor 7 endogenous ligand in rheumatoid arthritis synovial fluid that can provoke arthritic joint inflammation. Arthritis Rheumatol. 68, 1099–1110. doi: 10.1002/art.39544
Kitaichi, N., Horie, Y., and Ohno, S. (2008). Prompt therapy reduces the duration of systemic corticosteroids in Vogt-Koyanagi-Harada disease. Graefes Arch. Clin. Exp. Ophthalmol. 246, 1641–1642. doi: 10.1007/s00417-008-0869-5
Kobayashi, H., Kokubo, T., Takahashi, M., Sato, K., Miyokawa, N., Kimura, S., et al. (1998). Tyrosinase epitope recognized by an HLA-DR-restricted T-cell line from a Vogt-Koyanagi-Harada disease patient. Immunogenetics 47, 398–403. doi: 10.1007/s002510050375
Kotani, A., Ha, D., Hsieh, J., Rao, P. K., Schotte, D., Den Boer, M. L., et al. (2009). miR-128b is a potent glucocorticoid sensitizer in MLL-AF4 acute lymphocytic leukemia cells and exerts cooperative effects with miR-221. Blood 114, 4169–4178. doi: 10.1182/blood-2008-12-191619
Kwok, H. H., Poon, P. Y., Mak, K. H., Zhang, L. Y., Liu, P., Zhang, H., et al. (2017). Role of G3BP1 in glucocorticoid receptor-mediated microRNA-15b and microRNA-23a biogenesis in endothelial cells. Cell. Mol. Life Sci. 74, 3613–3630. doi: 10.1007/s00018-017-2540-y
Lai, T. Y., Chan, R. P., Chan, C. K., and Lam, D. S. (2009). Effects of the duration of initial oral corticosteroid treatment on the recurrence of inflammation in Vogt-Koyanagi-Harada disease. Eye (Lond) 23, 543–548. doi: 10.1038/eye.2008.89
Lambert, K. A., Roff, A. N., Panganiban, R. P., Douglas, S., and Ishmael, F. T. (2018). MicroRNA-146a is induced by inflammatory stimuli in airway epithelial cells and augments the anti-inflammatory effects of glucocorticoids. PLoS One 13:e0205434. doi: 10.1371/journal.pone.0205434
Li, B., Wang, X., Choi, I. Y., Wang, Y. C., Liu, S., Pham, A. T., et al. (2017). miR-146a modulates autoreactive Th17 cell differentiation and regulates organ-specific autoimmunity. J. Clin. Invest. 127, 3702–3716. doi: 10.1172/JCI94012
Li, B., Yang, P., Zhou, H., Huang, X., Jin, H., Chu, L., et al. (2005). Upregulation of T-bet expression in peripheral blood mononuclear cells during Vogt-Koyanagi-Harada disease. Br. J. Ophthalmol. 89, 1410–1412. doi: 10.1136/bjo.2005.074062
Li, W., Kong, L. B., Li, J. T., Guo, Z. Y., Xue, Q., Yang, T., et al. (2014). MiR-568 inhibits the activation and function of CD4(+) T cells and Treg cells by targeting NFAT5. Int. Immunol. 26, 269–281. doi: 10.1093/intimm/dxt065
Li, W., Liu, S., Chen, Y., Weng, R., Zhang, K., He, X., et al. (2020). Circulating exosomal microRNAs as biomarkers of systemic lupus erythematosus. Clinics (Sao Paulo) 75:e1528. doi: 10.6061/clinics/2020/e1528
Liang, Y. N., Tang, Y. L., Ke, Z. Y., Chen, Y. Q., Luo, X. Q., Zhang, H., et al. (2017). MiR-124 contributes to glucocorticoid resistance in acute lymphoblastic leukemia by promoting proliferation, inhibiting apoptosis and targeting the glucocorticoid receptor. J. Steroid Biochem. Mol. Biol. 172, 62–68. doi: 10.1016/j.jsbmb.2017.05.014
Liberman, P., Gauro, F., Berger, O., and Urzua, C. A. (2015). Causes of uveitis in a tertiary center in chile: a cross-sectional retrospective review. Ocul. Immunol. Inflamm. 23, 339–345. doi: 10.3109/09273948.2014.981548
Liu, L., Hua, M., Liu, C., He, N., Li, Z., and Ma, D. (2016). The aberrant expression of microRNAs and correlations with T cell subsets in patients with immune thrombocytopenia. Oncotarget 7, 76453–76463. doi: 10.18632/oncotarget.12949
Liu, X., Yang, P., Lin, X., Ren, X., Zhou, H., Huang, X., et al. (2009). Inhibitory effect of Cyclosporin a and corticosteroids on the production of IFN-gamma and IL-17 by T cells in Vogt-Koyanagi-Harada syndrome. Clin. Immunol. 131, 333–342. doi: 10.1016/j.clim.2008.12.007
Lu, L. F., Boldin, M. P., Chaudhry, A., Lin, L. L., Taganov, K. D., Hanada, T., et al. (2010). Function of miR-146a in controlling Treg cell-mediated regulation of Th1 responses. Cell 142, 914–929. doi: 10.1016/j.cell.2010.08.012
Lucafo, M., Sicari, D., Chicco, A., Curci, D., Bellazzo, A., Di Silvestre, A., et al. (2020). miR-331-3p is involved in glucocorticoid resistance reversion by rapamycin through suppression of the MAPK signaling pathway. Cancer Chemother. Pharmacol. 86, 361–374. doi: 10.1007/s00280-020-04122-z
Lv, M., Zhang, X., Jia, H., Li, D., Zhang, B., Zhang, H., et al. (2012). An oncogenic role of miR-142-3p in human T-cell acute lymphoblastic leukemia (T-ALL) by targeting glucocorticoid receptor-alpha and cAMP/PKA pathways. Leukemia 26, 769–777. doi: 10.1038/leu.2011.273
Lyszkiewicz, M., Winter, S. J., Witzlau, K., Fohse, L., Brownlie, R., Puchalka, J., et al. (2019). miR-181a/b-1 controls thymic selection of Treg cells and tunes their suppressive capacity. PLoS Biol. 17:e2006716. doi: 10.1371/journal.pbio.2006716
Mensa, E., Recchioni, R., Marcheselli, F., Giuliodori, K., Consales, V., Molinelli, E., et al. (2018). MiR-146a-5p correlates with clinical efficacy in patients with psoriasis treated with the tumour necrosis factor-alpha inhibitor adalimumab. Br. J. Dermatol. 179, 787–789. doi: 10.1111/bjd.16659
Molitoris, J. K., Mccoll, K. S., and Distelhorst, C. W. (2011). Glucocorticoid-mediated repression of the oncogenic microRNA cluster miR-17~92 contributes to the induction of Bim and initiation of apoptosis. Mol. Endocrinol. 25, 409–420. doi: 10.1210/me.2010-0402
Moschos, S. A., Williams, A. E., Perry, M. M., Birrell, M. A., Belvisi, M. G., and Lindsay, M. A. (2007). Expression profiling in vivo demonstrates rapid changes in lung microRNA levels following lipopolysaccharide-induced inflammation but not in the anti-inflammatory action of glucocorticoids. BMC Genomics 8:240. doi: 10.1186/1471-2164-8-240
Mycko, M. P., Cichalewska, M., Machlanska, A., Cwiklinska, H., Mariasiewicz, M., and Selmaj, K. W. (2012). MicroRNA-301a regulation of a T-helper 17 immune response controls autoimmune demyelination. Proc. Natl. Acad. Sci. U.S.A.j 109, E1248–E1257. doi: 10.1073/pnas.1114325109
Nahid, M. A., Satoh, M., and Chan, E. K. (2011). MicroRNA in TLR signaling and endotoxin tolerance. Cell. Mol. Immunol. 8, 388–403. doi: 10.1038/cmi.2011.26
Norose, K., and Yano, A. (1996). Melanoma specific Th1 cytotoxic T lymphocyte lines in Vogt-Koyanagi-Harada disease. Br. J. Ophthalmol. 80, 1002–1008. doi: 10.1136/bjo.80.11.1002
Norose, K., Yano, A., Aosai, F., and Segawa, K. (1990). Immunologic analysis of cerebrospinal fluid lymphocytes in Vogt-Koyanagi-Harada disease. Invest. Ophthalmol. Vis. Sci. 31, 1210–1216.
Norose, K., Yano, A., Wang, X. C., Tokushima, T., Umihira, J., Seki, A., et al. (1994). Dominance of activated T cells and interleukin-6 in aqueous humor in Vogt-Koyanagi-Harada disease. Invest. Ophthalmol. Vis. Sci. 35, 33–39.
Ohta, K., and Yoshimura, N. (1998). Expression of Fas antigen on helper T lymphocytes in Vogt-Koyanagi-Harada disease. Graefes Arch. Clin. Exp. Ophthalmol. 236, 434–439. doi: 10.1007/s004170050102
O’keefe, G. A., and Rao, N. A. (2017). Vogt-Koyanagi-Harada disease. Surv. Ophthalmol. 62, 1–25. doi: 10.1016/j.survophthal.2016.05.002
Otani, S., Sakurai, T., Yamamoto, K., Fujita, T., Matsuzaki, Y., Goto, Y., et al. (2006). Frequent immune response to a melanocyte specific protein KU-MEL-1 in patients with Vogt-Koyanagi-Harada disease. Br. J. Ophthalmol. 90, 773–777. doi: 10.1136/bjo.2005.086520
Park, H. S., Nam, K. Y., and Kim, J. Y. (2011). Intravitreal bevacizumab injection for persistent serous retinal detachment associated with Vogt-Koyanagi-Harada disease. Graefes Arch. Clin. Exp. Ophthalmol. 249, 133–136. doi: 10.1007/s00417-010-1477-8
Prattichizzo, F., Giuliani, A., Recchioni, R., Bonafe, M., Marcheselli, F., De Carolis, S., et al. (2016). Anti-TNF-alpha treatment modulates SASP and SASP-related microRNAs in endothelial cells and in circulating angiogenic cells. Oncotarget 7, 11945–11958. doi: 10.18632/oncotarget.7858
Przepiera-Bedzak, H., Fischer, K., and Brzosko, M. (2016). Extra-articular symptoms in constellation with selected serum cytokines and disease activity in spondyloarthritis. Mediators Inflamm. 2016:7617954. doi: 10.1155/2016/7617954
Qi, J., Hou, S., Zhang, Q., Liao, D., Wei, L., Fang, J., et al. (2013). A functional variant of pre-miRNA-196a2 confers risk for Behcet’s disease but not for Vogt-Koyanagi-Harada syndrome or AAU in ankylosing spondylitis. Hum. Genet. 132, 1395–1404. doi: 10.1007/s00439-013-1346-8
Rainer, J., Ploner, C., Jesacher, S., Ploner, A., Eduardoff, M., Mansha, M., et al. (2009). Glucocorticoid-regulated microRNAs and mirtrons in acute lymphoblastic leukemia. Leukemia 23, 746–752. doi: 10.1038/leu.2008.370
Ramchandran, R., and Chaluvally-Raghavan, P. (2017). miRNA-mediated RNA activation in mammalian cells. Adv. Exp. Med. Biol. 983, 81–89. doi: 10.1007/978-981-10-4310-9_6
Reddycherla, A. V., Meinert, I., Reinhold, A., Reinhold, D., Schraven, B., and Simeoni, L. (2015). miR-20a inhibits TCR-mediated signaling and cytokine production in human naive CD4+ T cells. PLoS One 10:e0125311. doi: 10.1371/journal.pone.0125311
Rosenbaum, J. T., Bodaghi, B., Couto, C., Zierhut, M., Acharya, N., Pavesio, C., et al. (2019). New observations and emerging ideas in diagnosis and management of non-infectious uveitis: a review. Semin. Arthritis Rheum. 49, 438–445. doi: 10.1016/j.semarthrit.2019.06.004
Saus, E., Soria, V., Escaramis, G., Vivarelli, F., Crespo, J. M., Kagerbauer, B., et al. (2010). Genetic variants and abnormal processing of pre-miR-182, a circadian clock modulator, in major depression patients with late insomnia. Hum. Mol. Genet. 19, 4017–4025. doi: 10.1093/hmg/ddq316
Shen, E., Rathinam, S. R., Babu, M., Kanakath, A., Thundikandy, R., Lee, S. M., et al. (2016). Outcomes of Vogt-Koyanagi-Harada disease: a subanalysis from a randomized clinical trial of antimetabolite therapies. Am. J. Ophthalmol. 168, 279–286. doi: 10.1016/j.ajo.2016.06.004
Singh, A., Patro, P. S., and Aggarwal, A. (2019). MicroRNA-132, miR-146a, and miR-155 as potential biomarkers of methotrexate response in patients with rheumatoid arthritis. Clin. Rheumatol. 38, 877–884. doi: 10.1007/s10067-018-4380-z
Smith, L. K., Shah, R. R., and Cidlowski, J. A. (2010). Glucocorticoids modulate microRNA expression and processing during lymphocyte apoptosis. J. Biol. Chem. 285, 36698–36708. doi: 10.1074/jbc.M110.162123
Starega-Roslan, J., Krol, J., Koscianska, E., Kozlowski, P., Szlachcic, W. J., Sobczak, K., et al. (2011). Structural basis of microRNA length variety. Nucleic Acids Res. 39, 257–268. doi: 10.1093/nar/gkq727
Su, D., Wang, X., Ma, Y., Hao, J., Wang, J., Lu, Y., et al. (2020). Nrf2-induced miR-23a-27a-24-2 cluster modulates damage repair of intestinal mucosa by targeting the Bach1/HO-1 axis in inflammatory bowel diseases. Free Radic. Biol. Med. 163, 1–9. doi: 10.1016/j.freeradbiomed.2020.11.006
Sugita, S., Takase, H., Taguchi, C., Imai, Y., Kamoi, K., Kawaguchi, T., et al. (2006). Ocular infiltrating CD4+ T cells from patients with Vogt-Koyanagi-Harada disease recognize human melanocyte antigens. Invest. Ophthalmol. Vis. Sci. 47, 2547–2554. doi: 10.1167/iovs.05-1547
Tang, H., Lai, Y., Zheng, J., Chen, K., Jiang, H., and Xu, G. (2020). MiR-146a promotes tolerogenic properties of dendritic cells and through targeting Notch1 signaling. Immunol. Invest. 49, 555–570. doi: 10.1080/08820139.2019.1708385
Tang, Q., Yang, Y., Zhao, M., Liang, G., Wu, H., Liu, Q., et al. (2015). Mycophenolic acid upregulates miR-142-3P/5P and miR-146a in lupus CD4+T cells. Lupus 24, 935–942. doi: 10.1177/0961203315570685
Tessel, M. A., Benham, A. L., Krett, N. L., Rosen, S. T., and Gunaratne, P. H. (2011). Role for microRNAs in regulating glucocorticoid response and resistance in multiple myeloma. Horm. Cancer 2, 182–189. doi: 10.1007/s12672-011-0072-8
Urzua, C. A., Chen, P., Chaigne-Delalande, B., Liu, B., Anguita, R., Guerrero, J., et al. (2019). Glucocorticoid receptor-alpha and MKP-1 as candidate biomarkers for treatment response and disease activity in Vogt-Koyanagi-Harada disease. Am. J. Ophthalmol. 207, 319–325. doi: 10.1016/j.ajo.2019.06.032
Urzua, C. A., Guerrero, J., Gatica, H., Velasquez, V., and Goecke, A. (2017). Evaluation of the glucocorticoid receptor as a biomarker of treatment response in Vogt-Koyanagi-Harada disease. Invest. Ophthalmol. Vis. Sci. 58, 974–980. doi: 10.1167/iovs.16-20783
Valenzuela, R. A., Flores, I., Pujol, M., Llanos, C., Carreno, E., Rada, G., et al. (2020a). Definition of uveitis refractory to treatment: a systematic review in the absence of a consensus. Ocul. Immunol. Inflamm. doi: 10.1080/09273948.2020.1793369 [Epub ahead of print].
Valenzuela, R. A., Flores, I., Urrutia, B., Fuentes, F., Sabat, P. E., Llanos, C., et al. (2020b). New pharmacological strategies for the treatment of non-infectious uveitis. a minireview. Front. Pharmacol. 11:655. doi: 10.3389/fphar.2020.00655
Van Den Hof, W. F., Van Summeren, A., Lommen, A., Coonen, M. L., Brauers, K., Van Herwijnen, M., et al. (2014). Integrative cross-omics analysis in primary mouse hepatocytes unravels mechanisms of cyclosporin A-induced hepatotoxicity. Toxicology 324, 18–26. doi: 10.1016/j.tox.2014.06.003
Velez, G., Roybal, C. N., Colgan, D., Tsang, S. H., Bassuk, A. G., and Mahajan, V. B. (2016). Precision medicine: personalized proteomics for the diagnosis and treatment of idiopathic inflammatory disease. JAMA Ophthalmol. 134, 444–448. doi: 10.1001/jamaophthalmol.2015.5934
Wang, C., Tian, Y., Lei, B., Xiao, X., Ye, Z., Li, F., et al. (2012). Decreased IL-27 expression in association with an increased Th17 response in Vogt-Koyanagi-Harada disease. Invest. Ophthalmol. Vis. Sci. 53, 4668–4675. doi: 10.1167/iovs.12-9863
Wang, C., Wang, L., Hu, J., Li, H., Kijlstra, A., and Yang, P. (2018). Increased expression of IL-23 receptor (IL-23R) in Vogt-Koyanagi-Harada (VKH) disease. Curr. Eye Res. 43, 1369–1373. doi: 10.1080/02713683.2018.1485952
Wang, X., Li, C., Ju, S., Wang, Y., Wang, H., and Zhong, R. (2011). Myeloma cell adhesion to bone marrow stromal cells confers drug resistance by microRNA-21 up-regulation. Leuk. Lymphoma 52, 1991–1998. doi: 10.3109/10428194.2011.591004
Wang, Y., and Gaudio, P. A. (2008). Infliximab therapy for 2 patients with Vogt-Koyanagi-Harada syndrome. Ocul. Immunol. Inflamm. 16, 167–171. doi: 10.1080/09273940802204527
Wcislo-Dziadecka, D., Simka, K., Kazmierczak, A., Kruszniewska-Rajs, C., Gola, J., Grabarek, B., et al. (2018). Psoriasis treatment changes the expression profile of selected caspases and their regulatory microRNAs. Cell Physiol. Biochem. 50, 525–537. doi: 10.1159/000494166
Wu, J., Zhang, H., Zheng, Y., Jin, X., Liu, M., Li, S., et al. (2018). The long noncoding RNA MALAT1 induces tolerogenic dendritic cells and regulatory T cells via miR155/dendritic cell-specific intercellular adhesion molecule-3 grabbing nonintegrin/IL10 axis. Front. Immunol. 9:1847. doi: 10.3389/fimmu.2018.01847
Wu, L., Evans, T., Saravia, M., Schlaen, A., and Couto, C. (2009). Intravitreal bevacizumab for choroidal neovascularization secondary to Vogt-Koyanagi-Harada syndrome. Jpn. J. Ophthalmol. 53, 57–60. doi: 10.1007/s10384-008-0600-4
Wu, L., Zhou, H., Zhang, Q., Zhang, J., Ni, F., Liu, C., et al. (2010). DNA methylation mediated by a microRNA pathway. Mol. Cell 38, 465–475. doi: 10.1016/j.molcel.2010.03.008
Xu, J., Su, Y., Xu, A., Fan, F., Mu, S., Chen, L., et al. (2019). miR-221/222-mediated inhibition of autophagy promotes dexamethasone resistance in multiple myeloma. Mol. Ther. 27, 559–570. doi: 10.1016/j.ymthe.2019.01.012
Yang, A., Ma, J., Wu, M., Qin, W., Zhao, B., Shi, Y., et al. (2012). Aberrant microRNA-182 expression is associated with glucocorticoid resistance in lymphoblastic malignancies. Leuk. Lymphoma 53, 2465–2473. doi: 10.3109/10428194.2012.693178
Yang, D., Haemmig, S., Zhou, H., Perez-Cremades, D., Sun, X., Chen, L., et al. (2021). Methotrexate attenuates vascular inflammation through an adenosine-microRNA-dependent pathway. Elife 10:e58064. doi: 10.7554/eLife.58064
Yang, F., Lu, J., Yu, Y., and Gong, Y. (2017). Epithelial to mesenchymal transition in cyclosporine A-induced rat gingival overgrowth. Arch. Oral Biol. 81, 48–55. doi: 10.1016/j.archoralbio.2017.04.024
Yang, P., Ye, Z., Du, L., Zhou, Q., Qi, J., Liang, L., et al. (2018). Novel treatment regimen of Vogt-Koyanagi-Harada disease with a reduced dose of corticosteroids combined with immunosuppressive agents. Curr. Eye Res. 43, 254–261. doi: 10.1080/02713683.2017.1383444
Yang, P., Zhang, M., Wang, X., Xu, A. L., Shen, M., Jiang, B., et al. (2020). MicroRNA let-7g-5p alleviates murine collagen-induced arthritis by inhibiting Th17 cell differentiation. Biochem. Pharmacol. 174:113822. doi: 10.1016/j.bcp.2020.113822
Yang, Y., Tang, Q., Zhao, M., Liang, G., Wu, H., Li, D., et al. (2015). The effect of mycophenolic acid on epigenetic modifications in lupus CD4+T cells. Clin. Immunol. 158, 67–76. doi: 10.1016/j.clim.2015.03.005
Yu, H., Liu, Y., Bai, L., Kijlstra, A., and Yang, P. (2014). Predisposition to Behcet’s disease and VKH syndrome by genetic variants of miR-182. J. Mol. Med. (Berl.) 92, 961–967. doi: 10.1007/s00109-014-1159-9
Zhang, D., Qiu, X., Li, J., Zheng, S., Li, L., and Zhao, H. (2019). MiR-23a-3p-regulated abnormal acetylation of FOXP3 induces regulatory T cell function defect in Graves’ disease. Biol. Chem. 400, 639–650. doi: 10.1515/hsz-2018-0343
Zhou, Q., Haupt, S., Kreuzer, J. T., Hammitzsch, A., Proft, F., Neumann, C., et al. (2015). Decreased expression of miR-146a and miR-155 contributes to an abnormal Treg phenotype in patients with rheumatoid arthritis. Ann. Rheum. Dis. 74, 1265–1274. doi: 10.1136/annrheumdis-2013-204377
Zhou, Q., Hou, S., Liang, L., Li, X., Tan, X., Wei, L., et al. (2014). MicroRNA-146a and Ets-1 gene polymorphisms in ocular Behcet’s disease and Vogt-Koyanagi-Harada syndrome. Ann. Rheum. Dis. 73, 170–176. doi: 10.1136/annrheumdis-2012-201627
Keywords: miRNA, VKH, autoimmunity, inflammation, therapy
Citation: Vega-Tapia F, Bustamante M, Valenzuela RA, Urzua CA and Cuitino L (2021) miRNA Landscape in Pathogenesis and Treatment of Vogt–Koyanagi–Harada Disease. Front. Cell Dev. Biol. 9:658514. doi: 10.3389/fcell.2021.658514
Received: 25 January 2021; Accepted: 22 March 2021;
Published: 10 May 2021.
Edited by:
Jingmin Ou, Shanghai Jiao Tong University, ChinaReviewed by:
Girdhari Lal, National Centre for Cell Science, IndiaClaire Joanne Stocker, Aston University, United Kingdom
Copyright © 2021 Vega-Tapia, Bustamante, Valenzuela, Urzua and Cuitino. This is an open-access article distributed under the terms of the Creative Commons Attribution License (CC BY). The use, distribution or reproduction in other forums is permitted, provided the original author(s) and the copyright owner(s) are credited and that the original publication in this journal is cited, in accordance with accepted academic practice. No use, distribution or reproduction is permitted which does not comply with these terms.
*Correspondence: Cristhian A. Urzua, Y3Jpc3RoaWFuLnVyenVhQHVjaGlsZS5jbA==; Loreto Cuitino, Y3VpdGlub2xlQGdtYWlsLmNvbQ==