- 1Department of Infectious Diseases, The First Affiliated Hospital of Zhengzhou University, Zhengzhou, China
- 2Precision Medicine Center, Gene Hospital of Henan Province, The First Affifiliated Hospital of Zhengzhou University, Zhengzhou, China
Long non-coding RNAs (lncRNAs) are a class of functional RNA molecules that do not encode proteins and are composed of more than 200 nucleotides. LncRNAs play important roles in epigenetic and gene expression regulation. The oncogenic lncRNA GACAT3 was recently discovered to be dysregulated in many tumors. Aberrant expression of GACAT3 contributes to clinical characteristics and regulates multiple oncogenic processes. The association of GACAT3 with a variety of tumors makes it a promising biomarker for diagnosis, prognosis, and targeted therapy. In this review, we integrate the current understanding of the pathological features, biological functions, and molecular mechanisms of GACAT3 in cancer. Additionally, we provide insight into the utility of GACAT3 as an effective diagnostic and prognostic marker for specific tumors, which offers novel opportunities for targeted therapeutic intervention.
Introduction
Despite the countless advances of modern medicine and technology, the incidence and mortality of cancer remain high with an estimated 18.1 million new cancer cases and 9.6 million cancer-related deaths worldwide in 2018 alone (Bray et al., 2018; Ferlay et al., 2019; Lim et al., 2019). The rising incidence of cancer reflects both the prevalence of the disease, as well as improved detection and diagnostic capabilities. In contrast, the high mortality of cancer patients underscores the progress that still needs to made, especially in advanced or recurrent cancers with poor prognoses that remain difficult to treat (Li et al., 2018b; Moody et al., 2020). Effectively combatting cancer involves detecting the malignancy as early as possible and developing novel targeted therapies to treat cancers at advanced or recurrent stages. Both of these aims would be greatly aided by the development of novel and reliable biomarkers.
High-throughput technologies, such as next-generation sequencing, have revealed a variety of non-protein coding transcripts or non-coding RNAs (ncRNAs). ncRNAs play important roles in a variety of cellular and physiological functions (Chen, 2016). The largest class of ncRNAs is long non-coding RNAs (lncRNAs), which are functional RNA molecules of more than 200 nucleotides and include intergenic transcripts, enhancer RNAs (eRNAs), and sense or antisense transcripts that overlap other genes (Kopp and Mendell, 2018). LncRNAs play important roles in epigenetic and gene expression regulation at both the transcriptional and post-transcriptional levels. LncRNAs have also been implicated in stem cell maintenance and differentiation, cell autophagy and apoptosis, and embryonic development (Qian et al., 2019). The expression of specific lncRNAs is altered in many cancers, suggesting that lncRNAs contribute to tumorigenesis and/or tumor cell function (Xia et al., 2019; Qi-Dong et al., 2020; Shen et al., 2020; Ren et al., 2021).
The lncRNA gastric cancer-associated transcript 3 (GACAT3, also known as AC130710) is one such example of a cancer-associated lncRNA (Li et al., 2020). GACAT3 functions as a competing endogenous RNA (ceRNA) and is encoded by human chromosome 2p24.3 (Xia et al., 2014; Cao et al., 2020). Previous studies have shown that GACAT3 is involved in non-malignant diseases, including osteoarthritis (Li et al., 2018a). More recently, GACAT3 was reported to be abnormally expressed in many malignant tumors, including gastric cancer, hepatocellular carcinoma, colorectal cancer, breast cancer, bladder cancer, glioma, ovarian cancer, and non-small cell lung cancer (Klymenko et al., 2017). Accumulating evidence demonstrates that GACAT3 participates in the tumorigenesis of multiple human cancers.
The molecular function of GACAT3 is well-established and suggests that GACAT3 is a promising prognostic marker and target for therapy in specific cancers. Here, we review GACAT3 expression in human cancers, the association of GACAT3 with clinical characteristics, and the biological functions and regulatory mechanisms of GACAT3 in tumorigenesis.
Expression of GACAT3 in Cancer
Many studies show that GACAT3 is dysregulated in cancers, such as gastric cancer, colorectal cancer, hepatocellular carcinoma, breast cancer, bladder cancer, and glioma. Overexpression of GACAT3 correlates with clinicopathological features, including tumor size, tumor grade, stage of development, and lymph node metastasis. Furthermore, in vitro and in vivo experiments reveal that GACAT3 enhances cellular proliferation, migration, invasion, and tumor growth, which all contribute to aggressive tumor progression. Below we review the expression of GACAT3 in specific tumors, introduce the primary clinicopathological significance of GACAT3 in these tumors, and discuss the common GACAT3-regulated biological processes. GACAT3 expression and the relevant clinicopathological features of specific cancers are summarized in Table 1.
Gastric Cancer
Based on the 2019 Global Cancer Statistics, gastric cancer (GC) remains one of the most common malignancies (De Re, 2018; Siegel et al., 2019; Smyth et al., 2020). Numerous studies have demonstrated that abnormal expression of lncRNAs is associated with the occurrence and development of GC (Song et al., 2013; Li et al., 2014; Li et al., 2016; Dragomir et al., 2020). GACAT3 was first associated with GC by Chen et al. who officially named the lncRNA “gastric cancer associated transcript 3 (GACAT3)” (Chen et al., 2014; Sun et al., 2016). Since then, an accumulation of studies have confirmed that GACAT3 is significantly upregulated in GC tissues (Xu et al., 2014; Fanelli et al., 2018; Lin et al., 2018). In follow-up cell line experiments, significantly higher expression of GACAT3 was observed in MGC-803 cells compared to the normal gastric GES-1 cell line, whereas GACAT3 was expressed at remarkably lower levels in BGC-823, SGC-7901, and AGS gastric cancer cells compared to GES-1 cells. This opposing response among cell lines has been attributed to the heterogeneity of GC tumors (Xu et al., 2014). Despite these opposing results in cell lines, high GACAT3 expression in GC tissues is positively associated with tumor size, tumor-node-metastasis (TNM) stage, and distal metastasis (Xu et al., 2014). Functionally, siRNA-mediated knockdown of GACAT3 results in inhibition of cell proliferation in HGC-27 cells. Similarly, suppression of cell growth, increased apoptosis, and drug resistance occur after knockdown of GACAT3 in SGC-7901 and BGC-823 cells (Shen et al., 2016; Lin et al., 2018). GACAT3 also influences colony formation, migration, and invasion in GC cells (Shen et al., 2016; Feng et al., 2018). In summary, although GACAT3 expression varies among GC cancer cell lines, the overwhelming majority of evidence suggests that GACAT3 functions as an oncogene in GC.
Colorectal Cancer
Colorectal cancer (CRC) is the world’s fourth most fatal malignancy, accounting for almost 900,000 deaths per year (Dekker et al., 2019; Ferlay et al., 2019; Arnold et al., 2020). Over the next decade, CRC is estimated to account for more than 2.2 million new cases and 1.1 million deaths (Arnold et al., 2017). As the clinical manifestations of CRC are only evident at advanced stages, it is critically important to develop novel sensitive biomarkers to aid the early diagnosis of CRC, which would vastly improve patient survival and prognosis (Zhou et al., 2018; Tieng et al., 2020; Shamsuddin et al., 2021). GACAT3 is a promising biomarker for CRC as it is overexpressed in CRC tissues and is upregulated in the CRC cell lines HT29, HCT116, SW480, and LoVo relative to the normal colorectal epithelial cell line FHC (Zhou et al., 2018; Ye et al., 2020). Functional studies demonstrate that siRNA-mediated knockdown of GACAT3 suppresses the growth of HCT116 cells. Moreover, GACAT3 promotes cell proliferation, colony formation, invasion, and migration in both HT29 and LoVo cells. In vivo studies have further confirmed the positive influence of GACAT3 on CRC cell growth in a nude mice model (Zhou et al., 2018). Clinicopathological analyses of CRC suggest that GACAT3 is positively related to tumor infiltration depth, TNM stage, lymph node metastasis, and CA19-9 level (Ye et al., 2020). Indeed, GACAT3 expression in CRC patients with early (T1–2) disease is elevated compared to those with other T statuses. Likewise, GACAT3 is upregulated in TNM stage III–IV tissues compared to stage I–II tissues. Together, these observations suggest that GACAT3 distinguishes CRC from normal tissue and may also indicate tumor stage, supporting the development of GACAT3 as a biomarker in CRC.
Hepatocellular Carcinoma
Liver cancer is the second major cause of cancer-related death worldwide and accounts for approximately 850,000 new cancer cases annually (Llovet et al., 2016). Hepatocellular carcinoma (HCC) is the most common type of liver cancer (Anwanwan et al., 2020), and overexpression of many lncRNAs in hepatocytes contributes to carcinogenesis in HCC (Wang et al., 2017; Feng et al., 2019; Zhang et al., 2019; Zhang et al., 2020b). GACAT3 is aberrantly overexpressed in HCC tissues and liver cancer cell lines, including HepG2, HCCLM3, SK-Hep-1, SMMC-7721, and Huh7 (Dong et al., 2020; Li et al., 2020). Importantly, GACAT3 is associated with lower OS and disease-free survival in HCC patients (Dong et al., 2020). Studies in tissues and cell lines agree that inhibition of GACAT3 suppresses the ability of hepatoma cells to proliferate and migrate and promotes cell apoptosis(Dong et al., 2020).
Breast Cancer
Breast cancer is the most frequently diagnosed cancer and is the leading cause of cancer-related deaths among females (Winters et al., 2017). Most breast cancers are estrogen-receptor positive (70% of breast cancers), followed by triple negative breast cancers (15–20% of breast cancers), and finally by human epidermal growth factor 2 positive breast cancers (10–15% of breast cancers) (Yeo and Guan, 2017; Waks and Winer, 2019). The evolution of whole genome and transcriptome sequencing techniques has made lncRNAs essential for breast cancer research (Elias-Rizk et al., 2020; Oshi et al., 2020; Tsang and Tse, 2020). Studies have observed higher GACAT3 expression in breast cancer tissues and MCF-7 cells compared to adjacent normal tissues and the normal breast epithelial MCF-10A cell line (Zhong et al., 2018; Hu et al., 2019b). Downregulation of GACAT3 significantly inhibits proliferation, migration, and invasion of breast cancer cells.
Mammography is widely used to screen and diagnose breast cancer (Gøtzsche and Jørgensen, 2013; Løberg et al., 2015). Due to the high false-positive rate of mammography, recent studies have recommended breast MRI as a more sensitive assessment of the malignancy (McDonald et al., 2016). Notably, a study that employed breast MRI and diffusion-weighted imaging found that elevated expression of GACAT3 was associated with reduced perfusion-related diffusion and increased perfusion fraction, which are indicative of aggressive tumor biology and undesirable chemotherapy effects (Hu et al., 2019b).
Glioma
Glioma is the most common primary intracranial neoplasm in adults, accounting for 81% of brain tumors (Schwartzbaum et al., 2006; Jiang et al., 2016; Gusyatiner and Hegi, 2018). Although gliomas are relatively rare, they are aggressive tumors with high morbidity and mortality rates (Ostrom et al., 2014). LncRNAs are aberrantly expressed in glioma tissues and cell lines and are thought to affect the occurrence and development of the tumor (Peng et al., 2018; Yu et al., 2021). Recent studies have shown that GACAT3 is overexpressed in glioma tissues and is incrementally elevated during disease progression. Based on these studies, GACAT3 is included in the World Health Organization grading of gliomas. GACAT3 expression is also substantially higher in the glioma cell lines U87, U251, and A172 compared to the normal brain cell line HA 1800. Experimental evidence suggests that GACAT3 functions as an oncogene by regulating proliferation, apoptosis, migration, and invasion in glioma cells (Pan et al., 2019; Wang et al., 2019a).
Bladder Cancer
Bladder cancer is one of the most common urological malignancies worldwide (Sung et al., 2021). The gold standard for bladder cancer diagnosis, urine cytology, is limited by low sensitivity (Ng et al., 2021). Emerging studies have shown that immune-related lncRNAs play fundamental roles in the prognosis and immunotherapy of bladder cancer patients (Wu et al., 2020; Zhou et al., 2021). GACAT3 is upregulated in bladder cancer tissues, and elevated GACAT3 expression positively correlates with advanced bladder cancer grade and stage. In CRISPR-Cas13-transfected bladder cancer T24 and 5637 cell lines, GACAT3 promotes cell proliferation, suppresses apoptosis, and enhances cell migration by downregulating p21, BAX, and E-Cadherin protein levels, respectively. Mechanistically, GACAT3 acts as a ceRNA by interacting with miRNA-497 in the cytoplasm (Zhang et al., 2020a). These observations indicate that GACAT3-targeted therapy is a promising strategy for bladder cancer.
Non-Small Cell Lung Cancer
Lung cancer is the most frequent cancer worldwide (Hirsch et al., 2017) with an estimated 235,760 new cases and 131,880 mortalities in 2021. Lung cancer can be divided into small cell lung cancer (SCLC) and non-small cell lung cancer (NSCLC). The most common histologic subtypes of NSCLC are adenocarcinoma, squamous cell carcinoma, and large cell lung carcinoma (Kerr et al., 2014). Similar to major biomarkers of NSCLC, including epidermal growth factor receptor (EGFR), anaplastic lymphoma kinase (ALK), and Kirsten rat sarcoma viral oncogene homolog (KRAS) (Villalobos and Wistuba, 2017), GACAT3 is upregulated in NSCLC tissues and cell lines. Clinicopathologic analyses indicate that GACAT3 overexpression is significantly associated with lymph node metastasis and TNM stage but is not correlated with age, gender, or smoking history. Functionally, GACAT3 overexpression promotes cell proliferation and migration in NSCLC (Yang et al., 2018). Of note, GACAT3 enhances the sensitivity of lung cancer cells to radiotherapy, thereby promoting the apoptosis of NSCLC cells.
Regulation of Tumorigenesis and Progression
Tumorigenesis occurs as a series of events that include proliferation, migration, and local invasion. Proliferation, migration, and invasion are the three primary features of malignant cells. GACAT3 has been implicated in these three processes as well as in tumor growth, apoptosis, metastasis, epithelial-mesenchymal transition (EMT), and drug resistance. We discuss the importance of the relationship between GACAT3 and tumor cell proliferation, apoptosis, migration, and invasion below. Additionally, we describe the molecular mechanisms underlying these biological processes, which are also summarized in Table 2.
Cell Growth and Proliferation
Tumor growth is tightly related to dysregulated cell proliferation. Unrestrained tumor cell proliferation, which is usually caused by the activation of oncogenes or inactivation of tumor suppressor genes, eventually results in tumor formation and progression (Kabała-Dzik et al., 2017; Zhang et al., 2021). Functional analyses from several tumor types suggest that GACAT3 promotes cell proliferation and tumor growth by interacting with a variety of miRNAs and signaling pathways.
In GC, GACAT3 regulates cell proliferation via multiple mechanisms (Figure 1). The interaction of GACAT3 and miR-129-5P directly promotes proliferation (Xu et al., 2014), and the interaction of GACAT3 and miR-497 induces expression of the proliferation marker Ki-67 in SGC-7901 cells (Feng et al., 2018). Additionally, GACAT3 acts as a ceRNA of HMGA1, and this interaction suppresses cell cycle inhibitors p27 and p21 in GC SGC-7901 and BGC-823 cells. Finally, GACAT3 mediates cell proliferation downstream of the IL6/STAT3 signaling pathway by inducing cyclin D expression (Shen et al., 2016; Lin et al., 2018).
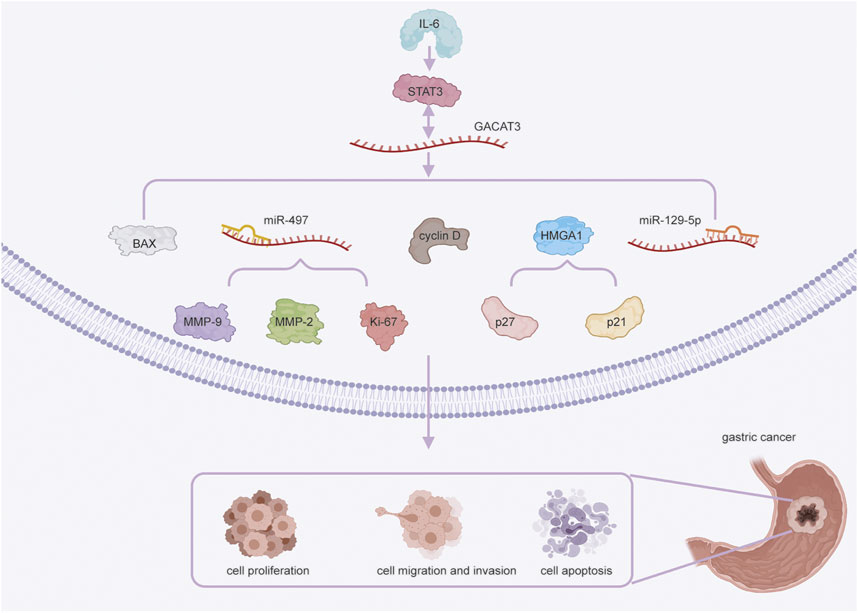
FIGURE 1. GACAT3 in gastric cancer (GC) cell proliferation, migration, invasion, and apoptosis. GACAT3 regulates cell proliferation in GC through multiple mechanisms. First, GACAT3 competitively interacts with miR-129-5P. GACAT3 also interacts with miR-497 to induce Ki-67 expression. Additionally, GACAT3 modulates the expression of HMGA1 and the levels of p27 and p21. Finally, GACAT3 upregulates cyclin D expression via IL6/STAT3 signaling. GACAT3 exerts its pro-migratory and pro-invasive effects by sponging miR-497 to downregulate the expression of MMP-2 and MMP-9. GACAT3 promotes cell apoptosis by upregulating STAT3 expression and decreasing the level of BAX.
In CRC, GACAT3 stimulates cell proliferation via competitive interaction with miR-103 as the ceRNA of LINC00152 (Ye et al., 2020). In HCC, GACAT3 facilitates cell proliferation in MHCC-97H, HepG2, and MHCC-LM3 cell lines; although, the mechanism remains unclear (Dong et al., 2020). In breast cancer, GACAT3 promotes cell proliferation by reducing the levels of caspase 9 and upregulating the expression of Bcl-2 in MCF-7 cells. Alternatively, GACAT3 can also regulate CCND2 expression by sponging miR-497 to enhance cell proliferation in MCF-7 cells (Zhong et al., 2018; Hu et al., 2019b). In glioma (Figure 2), GACAT3 targets miR-3127-5p to downregulate ELAVL1 expression, thereby significantly enhancing cell proliferation and colony formation of A172 cells, U251 cells, and xenograft tumors (Pan et al., 2019). Moreover, GACAT3 also mediates glioma cell proliferation by regulating NAMPT via competitive binding to miR-135a (Wang et al., 2019a). In NSCLC, GACAT3 plays a positive role in tumor growth and cell proliferation by directly targeting TIMP2 in xenografts and A549 cells (Yang et al., 2018). Although GACAT3 does not promote malignant cell proliferation via one consistent pathway or interaction, it does appear to influence proliferation via its ceRNA function in multiple tumor types, which may represent a therapeutic vulnerability.
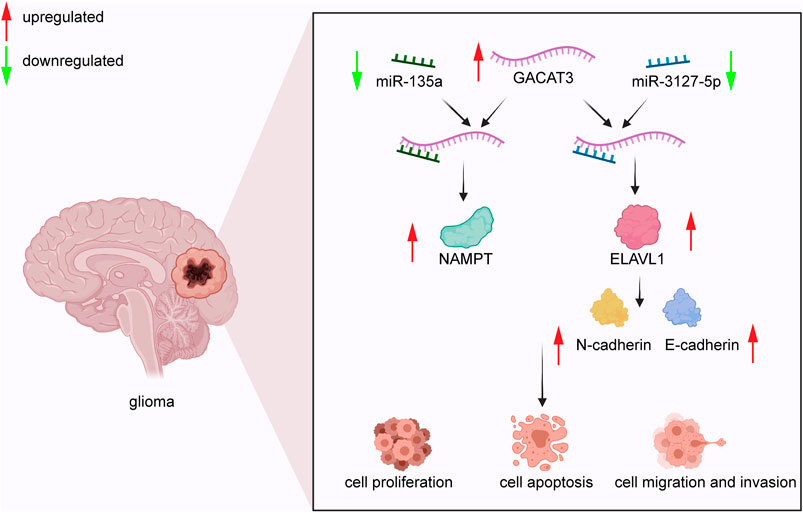
FIGURE 2. GACAT3 in glioma. GACAT3 elevates the level of NAMPT by interacting with miR-135a to mediate glioma cell proliferation. Additionally, GACAT3 inhibits cell apoptosis by binding miR-3127-5p and consequently elevating ELAVL1 levels. Elevated ELAVL1 levels also enhance EMT by altering the balance of N-cadherin and E-cadherin. As a result, the GACAT3/miR-3127-5p/ELAVL1 pathway promotes cell migration and invasion.
Cell Apoptosis
Apoptosis is a form of programmed cell death that maintains homeostasis in the body (Yue et al., 2020). Unsurprisingly, malignant cells often acquire the ability to evade apoptosis. Thus, to induce tumor cell death, many therapies target cell apoptosis or the pathways that allow tumor cells to evade apoptosis (Dong et al., 2015).
The anticancer drug cucurbitacin B promotes GC cell apoptosis, which is impaired by GACAT3 expression. Mechanistically, GACAT3 upregulates STAT3 to ultimately decrease expression of the pro-apoptotic protein bcl-2-associated X (BAX) in GC (Lin et al., 2018). In liver cancer cell lines, reduced expression of BAX and elevated expression of Bcl-2 (anti-apoptotic) support the anti-apoptotic effect of GACAT3 (Dong et al., 2020). In breast cancer, GACAT3 promotes cell apoptosis by downregulating caspases 3 and 9 as well as by upregulating BAX and Bcl-2 via miR-497 in MCF-7 cells (Zhong et al., 2018). In glioma, both in vitro and in vivo studies demonstrate that GACAT3 inhibits apoptosis by attenuating miR-3127-5p and consequently elevating ELAVL1 levels (Pan et al., 2019). In general, GACAT3 promotes the survival of malignant cells by impairing apoptosis and is a promising target to sensitive malignant cells to anti-cancer therapies.
Cell Migration and Invasion
Metastasis is a hallmark of malignant tumors and a complicated process that involves cell adhesion, migration, and invasion (van Zijl et al., 2011). Due to advanced stage, recurrence, and distant metastasis, the overall 5-year survival rate of cancer patients remains poor (Borriello et al., 2021). Although EMT is commonly accepted as a key event in metastasis (Thiery et al., 2009; van Zijl et al., 2011), understanding the molecular mechanisms of tumor cell migration and invasion remains an important focus of cancer research.
In GC, the interaction of miR-497 and GACAT3 influences cell migration and invasion by downregulating the cell invasion proteins MMP-2 and MMP-9 (Feng et al., 2018). GACAT3 acts as an endogenous miRNA sponge for miR-149 in CRC, thereby enhancing cell migration and proliferation (Zhou et al., 2018). In liver cancer, the expression of EMT factors is altered following suppression of GACAT3 in HepG2 and HCCLM3 cell lines. Specifically, N-cadherin, β-catenin, and TGF-β1 levels are reduced, and E-cadherin levels are elevated (Dong et al., 2020; Li et al., 2020), demonstrating that GACAT3 has important implications for cell migration and invasion in liver cancer. In breast cancer, wounding healing assays in MCF-7 cells show that GACAT3 knockdown dramatically decreases cell migration. Moreover, the expression of E-Cadherin was increased and the expression of N-Cadherin and Vimentin were decreased by GACAT3 knockdown. Interestingly, these effects were suppressed by a miR-497 inhibitor, indicating that miR-497 and GACAT3 cooperate to induce EMT and to enhance cell invasion and migration (Zhong et al., 2018). In glioma, GACAT3 substantially upregulates NAMPT expression, which enhances cell migration and invasion via sponging miR-135a in U87 and U251 cells (Wang et al., 2019a; Cao et al., 2020). Another study reported that silencing GACAT3 in U251 and A172 cells inhibited EMT by suppressing the levels of N-cadherin and elevating the levels of E-cadherin. Suppressed EMT further impairs cell migration and invasion via the GACAT3/miR-3127-5p/ELAVL1 pathway (Pan et al., 2019). In NSCLC A549 cells, GACAT3 directly targets TIMP2 and upregulates MMP10 to foster cell invasion and migration (Yang et al., 2018). Together, these studies suggest that GACAT3 contributes to cell invasion and migration, often by modulating the expression of EMT factors.
Future Clinical Applications
Evidence from recent decades indicates that lncRNAs are vital regulators of human cancers (Xiao et al., 2021). As such, lncRNAs have great potential for clinical applications in multiple tumor types (Hu et al., 2019a; Lou et al., 2021). Based on the above discussion, GACAT3 is a diagnostic and prognostic biomarker that has potential for next generation targeted therapies for specific tumors.
Diagnostic Biomarker
Accurate and early diagnosis is critical for prolonging the survival of cancer patients (Wang et al., 2020). GACAT3 is aberrantly expressed in GC, CRC, HCC, breast cancer, bladder cancer, and glioma. Abnormal expression of GACAT3 in specific cancer tissues can reliably differentiate normal tissue from diseased tissue, suggesting that GACAT3 is a useful diagnostic biomarker in these cancers. For example, ROC curve analysis validated the diagnostic value of GACAT3 in CRC. The study demonstrated areas under ROC curve (AUCs) of 0.8183 and 0.6238 for GACAT3 in 30 fresh CRC samples and 406 paraffin-embedded CRC samples, respectively. Co-detection of GACAT3 and LINC00152 further improved diagnostic accuracy to AUCs of 0.8411 and 0.6675, respectively (Ye et al., 2020). GACAT3 has also been confirmed as a diagnostic biomarker in glioma (Wang et al., 2019a) and is predictive of progression in breast cancer (Hu et al., 2019b).
Prognostic Indicator
In addition to its potential as a diagnostic biomarker, GACAT3 is also a promising prognostic indicator. Tumors discovered at advanced stages usually have a poor prognosis, which remains a major challenge for improving tumor outcomes. As tumor detection techniques are further developed, diverse molecular markers have become available to predict the prognosis of various tumors (Ma et al., 2020). GACAT3 is widely thought to be a prognostic biomarker for multiple tumors. For example, GACAT3 is associated with poor prognosis and shorter overall survival (OS) in GC (Xu et al., 2014; Feng et al., 2018). Moreover, GACAT3 is an independent prognostic factor for both poor OS and disease-free survival in HCC patients. Similarly, GACAT3 is associated with reduced OS and poor prognosis in breast cancer (Hu et al., 2019b). GACAT3 has also been implicated in the prognosis of CRC (Ye et al., 2020), glioma, and NSCLC (Yang et al., 2018; Wang et al., 2019a). A summary of these clinical associations can be found in Table 1.
Tumor Therapy Target
Despite decades of intensive cancer research efforts, there is no effective strategy to substantially decrease the rates of cancer recurrence and mortality (Guo et al., 2015; Song et al., 2018; Pienta et al., 2020). A recent breakthrough in cancer research has been the discovery and application of lncRNA in comprehensive tumor therapy (Bhan et al., 2017; Peng et al., 2017; Wang et al., 2019b). An abundance of evidence has demonstrated that GACAT3 is a novel oncogenic lncRNA that functions as a ceRNA to modulate the occurrence and development of various tumors (Feng et al., 2018; Zhong et al., 2018; Zhang et al., 2020a).
Knockdown of GACAT3 and overexpression of its relevant miRNA networks suppresses tumor progression, suggesting GACAT3 is a potential therapeutic target (Shen et al., 2016; Zhou et al., 2018; Dong et al., 2020). Specifically, GACAT3 is considered to be a valuable target for therapeutic intervention in GC, CRC, HCC, breast cancer, bladder cancer, NSCLC, and glioma (Pan et al., 2019). GACAT3 weakens the anti-apoptotic effects of anticancer drugs in GC (Lin et al., 2018). Moreover, GACAT3 is involved in the IL6 /STAT3 signaling pathway and therefore may be a promising biomarker for anti-cytokine treatment in GC (Shen et al., 2016). In NSCLC, overexpression of GACAT3 enhances radiotherapy efficiency, providing a new therapeutic target for NSCLC (Yang et al., 2018). Moreover, GACAT3 is included in an established ovarian cancer eight-lncRNA signature, which predicts chemotherapy response and platinum-resistance (Zhou et al., 2016; Klymenko et al., 2017). Despite these advances, the oncogenic mechanism of GACAT3 remains vague. Additional in-depth exploration of GACAT3 mechanisms are necessary to design effective cancer therapies.
Conclusion and Future Outlook
Emerging evidence demonstrates that GACAT3 is remarkably upregulated in CRC, HCC, breast cancer, NSCLC, bladder cancer, and glioma but shows diverse expression in GC cell lines. GACAT3 expression levels in tumor samples are generally correlated with tumor size, metastasis to lymph nodes, TNM stages, and patient survival. Moreover, GACAT3 is associated with CA19-9 levels in HCC and poor MRI diffusion weighted imaging in breast cancer, which are both indicative of aggressive tumors.
Functional analyses clearly demonstrate that GACAT3 acts as a ceRNA and interacts with multiple miRNAs in relevant oncogenic signaling pathways. GACAT3 exerts its carcinogenic effects by inhibiting apoptosis and enhancing cell proliferation, migration, and invasion. Thus, GACAT3 is a potential tumor biomarker for cancer diagnosis, prognosis, and targeted therapy. Detection of GACAT3 could be implemented to screen for malignancy and to monitor cancer progression. Specific GACAT3-targeted drug therapy offers a new direction and hope for cancer treatment. However, many of these findings were established in tissues and cancer cell lines. To fully explore the extent to which patients can benefit from GACAT3 monitoring and targeted treatment strategies, additional clinical validation in cancer patients is necessary.
Author Contributions
XY and ZD wrote this manuscript. SS designed the review.
Funding
This work was supported by the Science and Technology Research Project of Henan Province (202102310115), and Henan Medical Science and Technology Joint Building Program (LHGJ20200387).
Conflict of Interest
The authors declare that the research was conducted in the absence of any commercial or financial relationships that could be construed as a potential conflict of interest.
Publisher’s Note
All claims expressed in this article are solely those of the authors and do not necessarily represent those of their affiliated organizations, or those of the publisher, the editors and the reviewers. Any product that may be evaluated in this article, or claim that may be made by its manufacturer, is not guaranteed or endorsed by the publisher.
References
Anwanwan, D., Singh, S. K., Singh, S., Saikam, V., and Singh, R. (2020). Challenges in Liver Cancer and Possible Treatment Approaches. Biochim. Biophys. Acta (Bba) - Rev. Cancer 1873, 188314. doi:10.1016/j.bbcan.2019.188314
Arnold, M., Abnet, C. C., Neale, R. E., Vignat, J., Giovannucci, E. L., McGlynn, K. A., et al. (2020). Global burden of 5 Major Types of Gastrointestinal Cancer. Gastroenterology 159, 335–349. doi:10.1053/j.gastro.2020.02.068
Arnold, M., Sierra, M. S., Laversanne, M., Soerjomataram, I., Jemal, A., and Bray, F. (2017). Global Patterns and Trends in Colorectal Cancer Incidence and Mortality. Gut 66, 683–691. doi:10.1136/gutjnl-2015-310912
Bhan, A., Soleimani, M., and Mandal, S. S. (2017). Long Noncoding Rna and Cancer: A New Paradigm. Cancer Res. 77, 3965–3981. doi:10.1158/0008-5472.Can-16-2634
Borriello, L., Condeelis, J., Entenberg, D., and Oktay, M. H. (2021). Breast Cancer Cell Re-dissemination from Lung Metastases-A Mechanism for Enhancing Metastatic burden. Jcm 10, 2340. doi:10.3390/jcm10112340
Bray, F., Ferlay, J., Soerjomataram, I., Siegel, R. L., Torre, L. A., and Jemal, A. (2018). Global Cancer Statistics 2018: Globocan Estimates of Incidence and Mortality Worldwide for 36 Cancers in 185 Countries. CA: A Cancer J. Clinicians 68, 394–424. doi:10.3322/caac.21492
Cao, Z., Qiu, J., Yang, G., Liu, Y., Luo, W., You, L., et al. (2020). Mir-135a Biogenesis and Regulation in Malignancy: A new hope for Cancer Research and Therapy. Cancer Biol. Med. 17, 569–582. doi:10.20892/j.issn.2095-3941.2020.0033
Chen, L.-L. (2016). Linking Long Noncoding Rna Localization and Function. Trends Biochem. Sci. 41, 761–772. doi:10.1016/j.tibs.2016.07.003
Chen, S., Li, P., Xiao, B., and Guo, J. (2014). Long Noncoding Rna Hmlincrna717 and Ac130710 Have Been Officially Named as Gastric Cancer Associated Transcript 2 (Gacat2) and Gacat3, Respectively. Tumor Biol. 35, 8351–8352. doi:10.1007/s13277-014-2378-y
De Re, V. (2018). Molecular Features Distinguish Gastric Cancer Subtypes. Ijms 19, 3121. doi:10.3390/ijms19103121
Dekker, E., Tanis, P. J., Vleugels, J. L. A., Kasi, P. M., and Wallace, M. B. (2019). Colorectal Cancer. The Lancet 394, 1467–1480. doi:10.1016/s0140-6736(19)32319-0
Dong, L., Zhu, K., Chen, M., Li, D., Jiang, C., and Chen, L. (2020). Long Non-coding RNA GACAT3 P-romotes L-iver C-ancer P-rogression by R-egulating the P-roliferation, A-poptosis and M-igration of T-umor C-ells. Exp. Ther. Med. 19, 3377–3383. doi:10.3892/etm.2020.8604
Dong, Q.-M., Ling, C., and Zhao, L. (2015). Immunofluorescence Analysis of Cytokeratin 8/18 Staining Is a Sensitive Assay for the Detection of Cell Apoptosis. Oncol. Lett. 9, 1227–1230. doi:10.3892/ol.2015.2856
Dragomir, M. P., Kopetz, S., Ajani, J. A., and Calin, G. A. (2020). Non-coding Rnas in Gi Cancers: From Cancer Hallmarks to Clinical Utility. Gut 69, 748–763. doi:10.1136/gutjnl-2019-318279
Elias-Rizk, T., El Hajj, J., Segal-Bendirdjian, E., and Hilal, G. (2020). The Long Non Coding Rna H19 as a Biomarker for Breast Cancer Diagnosis in Lebanese Women. Sci. Rep. 10, 22228. doi:10.1038/s41598-020-79285-z
Fanelli, G. N., Gasparini, P., Coati, I., Cui, R., Pakula, H., Chowdhury, B., et al. (2018). Long-noncoding Rnas in Gastroesophageal Cancers. Non-coding RNA Res. 3, 195–212. doi:10.1016/j.ncrna.2018.10.001
Feng, J., Yang, G., Liu, Y., Gao, Y., Zhao, M., Bu, Y., et al. (2019). Lncrna Pcnap1 Modulates Hepatitis B Virus Replication and Enhances Tumor Growth of Liver Cancer. Theranostics 9, 5227–5245. doi:10.7150/thno.34273
Feng, L., Zhu, Y., Zhang, Y., and Rao, M. (2018). Lncrna Gacat3 Promotes Gastric Cancer Progression by Negatively Regulating Mir-497 Expression. Biomed. Pharmacother. 97, 136–142. doi:10.1016/j.biopha.2017.10.074
Ferlay, J., Colombet, M., Soerjomataram, I., Mathers, C., Parkin, D. M., Piñeros, M., et al. (2019). Estimating the Global Cancer Incidence and Mortality in 2018: Globocan Sources and Methods. Int. J. Cancer 144, 1941–1953. doi:10.1002/ijc.31937
Gøtzsche, P. C., and Jørgensen, K. J. (2013). Screening for Breast Cancer with Mammography. Cochrane Database Syst. Rev. CD001877. doi:10.1002/14651858.CD001877.pub5
Guo, C., Yu, H., Feng, B., Gao, W., Yan, M., Zhang, Z., et al. (2015). Highly Efficient Ablation of Metastatic Breast Cancer Using Ammonium-Tungsten-Bronze Nanocube as a Novel 1064 Nm-Laser-Driven Photothermal Agent. Biomaterials 52, 407–416. doi:10.1016/j.biomaterials.2015.02.054
Gusyatiner, O., and Hegi, M. E. (2018). Glioma Epigenetics: From Subclassification to Novel Treatment Options. Semin. Cancer Biol. 51, 50–58. doi:10.1016/j.semcancer.2017.11.010
Hirsch, F. R., Scagliotti, G. V., Mulshine, J. L., Kwon, R., Curran, W. J., Wu, Y.-L., et al. (2017). Lung Cancer: Current Therapies and New Targeted Treatments. The Lancet 389, 299–311. doi:10.1016/s0140-6736(16)30958-8
Hu, B., Wang, X., and Li, L. (2019a). Long Noncoding Rna Linc00337 Promote Gastric Cancer Proliferation through Repressing P21 Mediated by Ezh2. Am. J. Transl Res. 11, 3238–3245.
Hu, H., Wang, Y., Zhang, T., Zhang, C., Liu, Y., Li, G., et al. (2019b). Association of Lncrna-Gacat3 with Mri Features of Breast Cancer and its Molecular Mechanism. J. Buon 24, 2377–2384.
Jiang, T., Mao, Y., Ma, W., Mao, Q., You, Y., Yang, X., et al. (2016). Cgcg Clinical Practice Guidelines for the Management of Adult Diffuse Gliomas. Cancer Lett. 375, 263–273. doi:10.1016/j.canlet.2016.01.024
Kabała-Dzik, A., Rzepecka-Stojko, A., Kubina, R., Jastrzębska-Stojko, Ż., Stojko, R., Wojtyczka, R., et al. (2017). Migration Rate Inhibition of Breast Cancer Cells Treated by Caffeic Acid and Caffeic Acid Phenethyl Ester: An In Vitro Comparison Study. Nutrients 9, 1144. doi:10.3390/nu9101144
Kerr, K. M., Bubendorf, L., Edelman, M. J., Marchetti, A., Mok, T., Novello, S., et al. (2014). Second Esmo Consensus Conference on Lung Cancer: Pathology and Molecular Biomarkers for Non-small-cell Lung Cancer. Ann. Oncol. 25, 1681–1690. doi:10.1093/annonc/mdu145
Klymenko, Y., Kim, O., and Stack, M. (2017). Complex Determinants of Epithelial: Mesenchymal Phenotypic Plasticity in Ovarian Cancer. Cancers 9, 104. doi:10.3390/cancers9080104
Kopp, F., and Mendell, J. T. (2018). Functional Classification and Experimental Dissection of Long Noncoding Rnas. Cell 172, 393–407. doi:10.1016/j.cell.2018.01.011
Li, P.-F., Chen, S. C., Xia, T., Jiang, X. M., Shao, Y. F., Xiao, B. X., et al. (2014). Non-coding Rnas and Gastric Cancer. Wjg 20, 5411–5419. doi:10.3748/wjg.v20.i18.5411
Li, T., Mo, X., Fu, L., Xiao, B., and Guo, J. (2016). Molecular Mechanisms of Long Noncoding Rnas on Gastric Cancer. Oncotarget 7, 8601–8612. doi:10.18632/oncotarget.6926
Li, W., Chen, Q.-F., Huang, T., Wu, P., Shen, L., and Huang, Z.-L. (2020). Identification and Validation of a Prognostic Lncrna Signature for Hepatocellular Carcinoma. Front. Oncol. 10, 780. doi:10.3389/fonc.2020.00780
Li, X., Ren, W., Xiao, Z. Y., Wu, L. F., Wang, H., and Guo, P. Y. (2018a). Gacat3 Promoted Proliferation of Osteoarthritis Synoviocytes by Il-6/stat3 Signaling Pathway. Eur. Rev. Med. Pharmacol. Sci. 22, 5114–5120. doi:10.26355/eurrev_201808_15705
Li, Y., Shi, S., Ming, Y., Wang, L., Li, C., Luo, M., et al. (2018b). Specific Cancer Stem Cell-Therapy by Albumin Nanoparticles Functionalized with Cd44-Mediated Targeting. J. Nanobiotechnol 16, 99. doi:10.1186/s12951-018-0424-4
Lim, C., Kang, J. K., Won, W. R., Park, J. Y., Han, S. M., Le, T. N., et al. (2019). Co-delivery of D-(KLAKLAK)2 Peptide and Chlorin e6 using a Liposomal Complex for Synergistic Cancer Therapy. Pharmaceutics 11, 293. doi:10.3390/pharmaceutics11060293
Lin, Y., Li, J., Ye, S., Chen, J., Zhang, Y., Wang, L., et al. (2018). Lncrna Gacat3 Acts as a Competing Endogenous Rna of Hmga1 and Alleviates Cucurbitacin B-Induced Apoptosis of Gastric Cancer Cells. Gene 678, 164–171. doi:10.1016/j.gene.2018.08.037
Llovet, J. M., Zucman-Rossi, J., Pikarsky, E., Sangro, B., Schwartz, M., Sherman, M., et al. (2016). Hepatocellular Carcinoma. Nat. Rev. Dis. Primers 2, 16018. doi:10.1038/nrdp.2016.18
Løberg, M., Lousdal, M. L., Bretthauer, M., and Kalager, M. (2015). Benefits and Harms of Mammography Screening. Breast Cancer Res. 17, 63. doi:10.1186/s13058-015-0525-z
Lou, J., Wang, P., Chang, K., Wang, G., Geng, X., Wu, Y., et al. (2021). Knocking Down LINC01116 Can Inhibit the Regulation of TGF-β through miR-774-5p axis and Inhibit the Occurrence and Development of Glioma. Am. J. Transl Res. 13, 5702–5719.
Ma, X., Cheng, J., Zhao, P., Li, L., Tao, K., and Chen, H. (2020). DNA Methylation Profiling to Predict Recurrence Risk in Stage Ι Lung Adenocarcinoma: Development and Validation of a Nomogram to Clinical Management. J. Cell Mol Med 24, 7576–7589. doi:10.1111/jcmm.15393
McDonald, E. S., Clark, A. S., Tchou, J., Zhang, P., and Freedman, G. M. (2016). Clinical Diagnosis and Management of Breast Cancer. J. Nucl. Med. 57 (Suppl. 1), 9s–16s. doi:10.2967/jnumed.115.157834
Moody, R., Wilson, K., Jaworowski, A., and Plebanski, M. (2020). Natural Compounds with Potential to Modulate Cancer Therapies and Self-Reactive Immune Cells. Cancers 12, 673. doi:10.3390/cancers12030673
Ng, K., Stenzl, A., Sharma, A., and Vasdev, N. (2021). Urinary Biomarkers in Bladder Cancer: A Review of the Current Landscape and Future Directions. Urol. Oncol. Semin. Original Invest. 39, 41–51. doi:10.1016/j.urolonc.2020.08.016
Oshi, M., Takahashi, H., Tokumaru, Y., Yan, L., Rashid, O. M., Matsuyama, R., et al. (2020). G2m Cell Cycle Pathway Score as a Prognostic Biomarker of Metastasis in Estrogen Receptor (Er)-positive Breast Cancer. Ijms 21, 2921. doi:10.3390/ijms21082921
Ostrom, Q. T., Bauchet, L., Davis, F. G., Deltour, I., Fisher, J. L., Langer, C. E., et al. (2014). The Epidemiology of Glioma in Adults: A "state of the Science" Review. Neuro-Oncology 16, 896–913. doi:10.1093/neuonc/nou087
Pan, B., Zhao, M., and Xu, L. (2019). Long Noncoding RNA Gastric Cancer‐associated Transcript 3 Plays Oncogenic Roles in Glioma through Sponging miR‐3127‐5p. J. Cell Physiol 234, 8825–8833. doi:10.1002/jcp.27542
Peng, W.-X., Koirala, P., and Mo, Y.-Y. (2017). Lncrna-mediated Regulation of Cell Signaling in Cancer. Oncogene 36, 5661–5667. doi:10.1038/onc.2017.184
Peng, Z., Liu, C., and Wu, M. (2018). New Insights into Long Noncoding Rnas and Their Roles in Glioma. Mol. Cancer 17, 61. doi:10.1186/s12943-018-0812-2
Pienta, K. J., Hammarlund, E. U., Axelrod, R., Amend, S. R., and Brown, J. S. (2020). Convergent Evolution, Evolving Evolvability, and the Origins of Lethal Cancer. Mol. Cancer Res. 18, 801–810. doi:10.1158/1541-7786.Mcr-19-1158
Qi-Dong, X., Yang, X., Lu, J.-L., Liu, C.-Q., Sun, J.-X., Li, C., et al. (2020). Development and Validation of a Nine-Redox-Related Long Noncoding Rna Signature in Renal clear Cell Carcinoma. Oxidative Med. Cell Longevity 2020, 1–30. doi:10.1155/2020/6634247
Qian, X., Zhao, J., Yeung, P. Y., Zhang, Q. C., and Kwok, C. K. (2019). Revealing Lncrna Structures and Interactions by Sequencing-Based Approaches. Trends Biochem. Sci. 44, 33–52. doi:10.1016/j.tibs.2018.09.012
Ren, P., Chang, L., Hong, X., Xing, L., and Zhang, H. (2021). Long Non-coding Rna Linc01116 Is Activated by Egr1 and Facilitates Lung Adenocarcinoma Oncogenicity via Targeting Mir-744-5p/cdca4 axis. Cancer Cell Int 21, 292. doi:10.1186/s12935-021-01994-w
Schwartzbaum, J. A., Fisher, J. L., Aldape, K. D., and Wrensch, M. (2006). Epidemiology and Molecular Pathology of Glioma. Nat. Rev. Neurol. 2, 494–503. doi:10.1038/ncpneuro0289
Shamsuddin, S. H., Jayne, D. G., Tomlinson, D. C., McPherson, M. J., and Millner, P. A. (2021). Selection and Characterisation of Affimers Specific for Cea Recognition. Sci. Rep. 11, 744. doi:10.1038/s41598-020-80354-6
Shen, W., Yuan, Y., Zhao, M., Li, J., Xu, J., Lou, G., et al. (2016). Novel Long Non-coding Rna Gacat3 Promotes Gastric Cancer Cell Proliferation through the Il-6/stat3 Signaling Pathway. Tumor Biol. 37, 14895–14902. doi:10.1007/s13277-016-5372-8
Shen, X., Li, Y., He, F., and Kong, J. (2020). Lncrna Cdkn2b-As1 Promotes Cell Viability, Migration, and Invasion of Hepatocellular Carcinoma via Sponging Mir-424-5p. Cmar 12, 6807–6819. doi:10.2147/cmar.S240000
Siegel, R. L., Miller, K. D., and Jemal, A. (2019). Cancer Statistics, 2019. CA A. Cancer J. Clin. 69, 7–34. doi:10.3322/caac.21551
Smyth, E. C., Nilsson, M., Grabsch, H. I., van Grieken, N. C., and Lordick, F. (2020). Gastric Cancer. The Lancet 396, 635–648. doi:10.1016/s0140-6736(20)31288-5
Song, H., Sun, W., Ye, G., Ding, X., Liu, Z., Zhang, S., et al. (2013). Long Non-coding Rna Expression Profile in Human Gastric Cancer and its Clinical Significances. J. Transl Med. 11, 225. doi:10.1186/1479-5876-11-225
Song, J., Zhang, Y., Zhang, C., Du, X., Guo, Z., Kuang, Y., et al. (2018). A Microfluidic Device for Studying Chemotaxis Mechanism of Bacterial Cancer Targeting. Sci. Rep. 8, 6394. doi:10.1038/s41598-018-24748-7
Sun, W., Yang, Y., Xu, C., Xie, Y., and Guo, J. (2016). Roles of Long Noncoding Rnas in Gastric Cancer and Their Clinical Applications. J. Cancer Res. Clin. Oncol. 142, 2231–2237. doi:10.1007/s00432-016-2183-7
Sung, H., Ferlay, J., Siegel, R. L., Laversanne, M., Soerjomataram, I., Jemal, A., et al. (2021). Global Cancer Statistics 2020: Globocan Estimates of Incidence and Mortality Worldwide for 36 Cancers in 185 Countries. CA A. Cancer J. Clin. 71, 209–249. doi:10.3322/caac.21660
Thiery, J. P., Acloque, H., Huang, R. Y. J., and Nieto, M. A. (2009). Epithelial-mesenchymal Transitions in Development and Disease. Cell 139, 871–890. doi:10.1016/j.cell.2009.11.007
Tieng, F. Y. F., Abu, N., Sukor, S., Mohd Azman, Z. A., Mahamad Nadzir, N., Lee, L.-H., et al. (2020). L1cam, Ca9, Klk6, Hpn, and Aldh1a1 as Potential Serum Markers in Primary and Metastatic Colorectal Cancer Screening. Diagnostics 10, 444. doi:10.3390/diagnostics10070444
Tsang, J. Y. S., and Tse, G. M. (2020). Molecular Classification of Breast Cancer. Adv. Anat. Pathol. 27, 27–35. doi:10.1097/pap.0000000000000232
van Zijl, F., Krupitza, G., and Mikulits, W. (2011). Initial Steps of Metastasis: Cell Invasion and Endothelial Transmigration. Mutat. Research/Reviews Mutat. Res. 728, 23–34. doi:10.1016/j.mrrev.2011.05.002
Villalobos, P., and Wistuba, (2017). Lung Cancer Biomarkers. Hematology/Oncology Clin. North America 31, 13–29. doi:10.1016/j.hoc.2016.08.006
Waks, A. G., and Winer, E. P. (2019). Breast Cancer Treatment. Jama 321, 288–300. doi:10.1001/jama.2018.19323
Wang, H., Huo, X., Yang, X.-R., He, J., Cheng, L., Wang, N., et al. (2017). Stat3-mediated Upregulation of Lncrna Hoxd-As1 as a Cerna Facilitates Liver Cancer Metastasis by Regulating Sox4. Mol. Cancer 16, 136. doi:10.1186/s12943-017-0680-1
Wang, J., Zhang, M., and Lu, W. (2019a). Long Noncoding RNA GACAT3 Promotes Glioma Progression by Sponging miR‐135a. J. Cell Physiol 234, 10877–10887. doi:10.1002/jcp.27946
Wang, L., Cho, K. B., Li, Y., Tao, G., Xie, Z., and Guo, B. (2019b). Long Noncoding Rna (Lncrna)-mediated Competing Endogenous Rna Networks Provide Novel Potential Biomarkers and Therapeutic Targets for Colorectal Cancer. Ijms 20, 5758. doi:10.3390/ijms20225758
Wang, Q., Xu, K., Tong, Y., Dai, X., Xu, T., He, D., et al. (2020). Novel Mirna Markers for the Diagnosis and Prognosis of Endometrial Cancer. J. Cell Mol Med 24, 4533–4546. doi:10.1111/jcmm.15111
Winters, S., Martin, C., Murphy, D., and Shokar, N. K. (2017). Breast Cancer Epidemiology, Prevention, and Screening. Prog. Mol. Biol. Transl Sci. 151, 1–32. doi:10.1016/bs.pmbts.2017.07.002
Wu, Y., Zhang, L., He, S., Guan, B., He, A., Yang, K., et al. (2020). Identification of Immune-Related Lncrna for Predicting Prognosis and Immunotherapeutic Response in Bladder Cancer. aging 12, 23306–23325. doi:10.18632/aging.104115
Xia, L., Nie, D., Wang, G., Sun, C., and Chen, G. (2019). FER1L4/miR‐372/E2F1 Works as a ceRNA System to Regulate the Proliferation and Cell Cycle of Glioma Cells. J. Cell Mol Med 23, 3224–3233. doi:10.1111/jcmm.14198
Xia, T., Liao, Q., Jiang, X., Shao, Y., Xiao, B., Xi, Y., et al. (2014). Long Noncoding Rna Associated-Competing Endogenous Rnas in Gastric Cancer. Sci. Rep. 4, 6088. doi:10.1038/srep06088
Xiao, D., Cui, X., Fang, N., Yu, S., and Wang, X. (2021). Linc01303 Promotes the Proliferation and Migration of Laryngeal Carcinoma by Regulating Mir-200c/timp2 axis. Am. J. Transl Res. 13, 1643–1656.
Xu, C., Shao, Y., Xia, T., Yang, Y., Dai, J., Luo, L., et al. (2014). Lncrna-ac130710 Targeting by Mir-129-5p Is Upregulated in Gastric Cancer and Associates with Poor Prognosis. Tumor Biol. 35, 9701–9706. doi:10.1007/s13277-014-2274-5
Yang, X., Zhang, W., Cheng, S. Q., and Yang, R. L. (2018). High Expression of Lncrna Gacat3 Inhibits Invasion and Metastasis of Non-small Cell Lung Cancer to Enhance the Effect of Radiotherapy. Eur. Rev. Med. Pharmacol. Sci. 22, 1315–1322. doi:10.26355/eurrev_201803_14473
Ye, S., Lu, Y., Ru, Y., Wu, X., Zhao, M., Chen, J., et al. (2020). LncRNAs GACAT3 and LINC00152 Regulate Each Other through miR‐103 and Are Associated with Clinicopathological Characteristics in Colorectal Cancer. J. Clin. Lab. Anal. 34, e23378. doi:10.1002/jcla.23378
Yeo, S. K., and Guan, J.-L. (2017). Breast Cancer: Multiple Subtypes within a Tumor? Trends Cancer 3, 753–760. doi:10.1016/j.trecan.2017.09.001
Yu, S., Ruan, X., Liu, X., Zhang, F., Wang, D., Liu, Y., et al. (2021). Hnrnpd Interacts with Zhx2 Regulating the Vasculogenic Mimicry Formation of Glioma Cells via Linc00707/mir-651-3p/sp2 axis. Cell Death Dis 12, 153. doi:10.1038/s41419-021-03432-1
Yue, X., Chen, Q., and He, J. (2020). Combination Strategies to Overcome Resistance to the Bcl2 Inhibitor Venetoclax in Hematologic Malignancies. Cancer Cell Int 20, 524. doi:10.1186/s12935-020-01614-z
Zhang, Y.-Q., Liang, Y.-K., Wu, Y., Chen, M., Chen, W.-L., Li, R.-H., et al. (2021). Notch3 Inhibits Cell Proliferation and Tumorigenesis and Predicts Better Prognosis in Breast Cancer through Transactivating Pten. Cell Death Dis 12, 502. doi:10.1038/s41419-021-03735-3
Zhang, Z., Chen, J., Zhu, Z., Zhu, Z., Liao, X., Wu, J., et al. (2020a). Crispr-cas13-mediated Knockdown of Lncrna-Gacat3 Inhibited Cell Proliferation and Motility, and Induced Apoptosis by Increasing P21, Bax, and E-Cadherin Expression in Bladder Cancer. Front. Mol. Biosci. 7, 627774. doi:10.3389/fmolb.2020.627774
Zhang, Z., Wang, S., Liu, Y., Meng, Z., and Chen, F. (2019). Low lncRNA ZNF385D-AS2 E-xpression and its P-rognostic S-ignificance in L-iver C-ancer. Oncol. Rep. 42, 1110–1124. doi:10.3892/or.2019.7238
Zhang, Z., Wang, S., Yang, F., Meng, Z., and Liu, Y. (2020b). LncRNA ROR1-AS1 H-igh E-xpression and its P-rognostic S-ignificance in L-iver C-ancer. Oncol. Rep. 43, 55–74. doi:10.3892/or.2019.7398
Zhong, H., Yang, J., Zhang, B., Wang, X., Pei, L., Zhang, L., et al. (2018). Lncrna Gacat3 Predicts Poor Prognosis and Promotes Cell Proliferation in Breast Cancer through Regulation of Mir-497/ccnd2. Cbm 22, 787–797. doi:10.3233/cbm-181354
Zhou, M., Sun, Y., Sun, Y., Xu, W., Zhang, Z., Zhao, H., et al. (2016). Comprehensive Analysis of Lncrna Expression Profiles Reveals a Novel Lncrna Signature to Discriminate Nonequivalent Outcomes in Patients with Ovarian Cancer. Oncotarget 7, 32433–32448. doi:10.18632/oncotarget.8653
Zhou, M., Zhang, Z., Bao, S., Hou, P., Yan, C., Su, J., et al. (2021). Computational Recognition of Lncrna Signature of Tumor-Infiltrating B Lymphocytes with Potential Implications in Prognosis and Immunotherapy of Bladder Cancer. Brief Bioinform 22. doi:10.1093/bib/bbaa047
Keywords: lncRNA, cancer, biomarker, GACAT3, molecular mechanisms
Citation: Yuan X, Dong Z and Shen S (2022) LncRNA GACAT3: A Promising Biomarker and Therapeutic Target in Human Cancers. Front. Cell Dev. Biol. 10:785030. doi: 10.3389/fcell.2022.785030
Received: 29 September 2021; Accepted: 05 January 2022;
Published: 20 January 2022.
Edited by:
Aamir Ahmad, University of Alabama at Birmingham, United StatesReviewed by:
Dhirodatta Senapati, DIT University, IndiaZunsong Hu, City of Hope National Medical Center, United States
Copyright © 2022 Yuan, Dong and Shen. This is an open-access article distributed under the terms of the Creative Commons Attribution License (CC BY). The use, distribution or reproduction in other forums is permitted, provided the original author(s) and the copyright owner(s) are credited and that the original publication in this journal is cited, in accordance with accepted academic practice. No use, distribution or reproduction is permitted which does not comply with these terms.
*Correspondence: Shen Shen, ZmNjc2hlbmtAenp1LmVkdS5jbg==
†These authors have contributed equally to this work