- Department of Otorhinolaryngology Head and Neck Surgery, Shanghai Children’s Hospital, Shanghai Jiao Tong University School of Medicine, Shanghai, China
ALKBH5 is one of the demethylases involved in the regulation of RNA m6A modification. In addition to its role in the dynamic regulation of RNA m6A modification, ALKBH5 has been found to play important roles in various tissues fibrosis processes in recent years. However, the mechanisms and effects of ALKBH5 in fibrosis have been reported inconsistently. Multiple cell types, including parenchymal cells, immune cells (neutrophils and T cells), macrophages, endothelial cells, and fibroblasts, play roles in various stages of fibrosis. Therefore, this review analyzes the mechanisms by which ALKBH5 regulates these cells, its impact on their functions, and the outcomes of fibrosis. Furthermore, this review summarizes the role of ALKBH5 in fibrotic diseases such as pulmonary fibrosis, liver fibrosis, cardiac fibrosis, and renal fibrosis, and discusses various ALKBH5 inhibitors that have been discovered to date, exploring the potential of ALKBH5 as a clinical target for fibrosis.
1 Introduction
ALKBH5 is one of the demethylases involved in the regulation of RNA N6-methyladenosine (m6A) modification. Its main function is to remove the m6A modification from RNA (Aik et al., 2014). m6A modification is a common form of RNA chemical modification that plays important roles in RNA stability, translational regulation, and post-transcriptional modification. ALKBH5 catalyzes the reaction that converts m6A modification back to adenosine, thereby participating in the dynamic regulation of RNA (Toh et al., 2020; Yu et al., 2021). In recent years, research on ALKBH5 has attracted widespread attention and has made significant progress in the field of life sciences.
Studies on ALKBH5 have demonstrated its crucial roles in multiple biological processes. Firstly, ALKBH5 is involved in the regulation of RNA metabolic homeostasis. By regulating the level of m6A modification, ALKBH5 can influence RNA degradation rate and translation efficiency, thereby controlling gene expression level and cellular functions (Zheng et al., 2013; Yu et al., 2021). Secondly, ALKBH5 plays an important role in germ cells, participating in germ cell development and maturation processes. Studies have shown that the loss of ALKBH5 can lead to germ cell apoptosis and infertility (Tang et al., 2018; Cai et al., 2022). Furthermore, ALKBH5 is closely associated with the occurrence and progression of tumors, and its expression level in tumor cells are related to prognosis and treatment outcomes (Hu et al., 2022; Zhai et al., 2023).
In recent years, ALKBH5 has been found to play important regulatory roles in various tissues fibrosis processes, although the mechanisms of ALKBH5 in fibrosis have been reported inconsistently (Ning et al., 2020; Sun et al., 2022a; Yang et al., 2022). Therefore, this review summarizes the research progress of ALKBH5 in various fibrosis-related cells and diseases such as pulmonary fibrosis, liver fibrosis, cardiac fibrosis, and renal fibrosis. Understanding the role of ALKBH5 in fibrosis not only contributes to a deeper understanding of its biological processes but also provides potential targets for the treatment and drug development of fibrotic diseases (Figure 1).
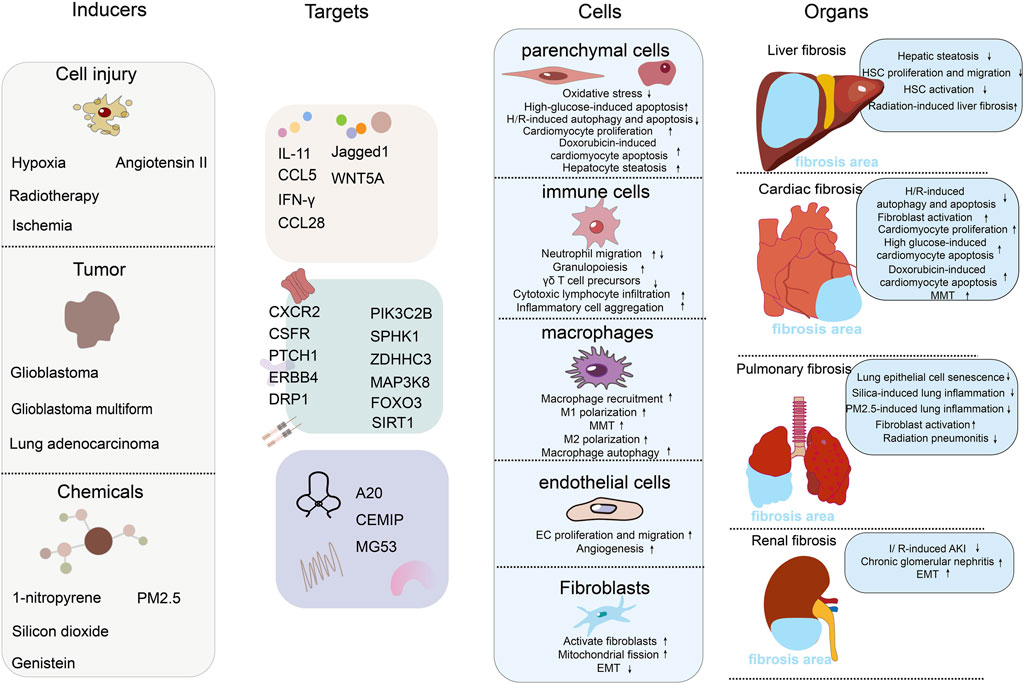
Figure 1. The role of RNA m6A demethylase ALKBH5 in the mechanisms of fibrosis. The figure includes 4 columns: inducers, targets, cells, organs. Inducers: this column lists the factors or conditions that can affect or activate the function of ALKBH5, including chemicals, tumor, and cell injure. Each inducer may modulate ALKBH5 activity or expression level in different ways. Targets: this column shows the targets of ALKBH5. We have divided the targets into three major categories: secreted proteins (such as interleukins, chemokines, and ligand proteins), cell membrane receptor proteins and their downstream signaling pathway proteins, and extracellular matrix proteins. Cells: this column shows the effects of ALKBH5 on different types of cells, including parenchymal cells (hepatocytes and cardiomyocytes), immune cells (neutrophils, T cells, etc.), macrophages, endothelial cells, and fibroblasts. We list the effects of ALKBH5 on cells functions. Organs: in this column, we show the effects of ALKBH5 on the fibrosis of different organs, including liver fibrosis, cardiac fibrosis, pulmonary fibrosis, and renal fibrosis. We list specific induction models and the mechanisms of fibrosis development.
2 The structure and function of ALKBH5
ALKBH5 belonged to the iron (II)- and 2-oxoglutarate-dependent AlkB oxygenase family (Aik et al., 2014). Endogenous ALKBH5 was primarily found in nuclear speckles, where facilitated mRNA processing. ALKBH5 was an mRNA-binding protein, and newly synthesized RNA was its main substrate (Meyer et al., 2012; Zheng et al., 2013; Feng et al., 2014). Structurally, the catalytic center of ALKBH5 contained a double-stranded β-helix domain (DSBH structure), consisting of 11 β strands and 5 α helices. The DSBH domain could bind to Fe2+ and 2OG. The binding of Fe2+ and 2OG directly affected the function of ALKBH5. The binding of Fe2+ and 2OG occurred prior to the binding of primary substrates (m6A-modified single-stranded nucleic acids) to the active site of ALKBH5. Studies had found that ALKBH5 was more disordered in solution than observed in its crystal structure. These differences were likely due to the absence of the Cys230-Cys267 disulfide bond in solution, which restricted the binding of 2OG to the “catalytic pocket” of ALKBH5. After 2OG binding to the “catalytic pocket,” the enzyme underwent a conformational change, enlarging the active site and increasing the enzyme’s affinity for substrates, allowing small molecule substrates to enter the active site (Purslow et al., 2018). Studies had also revealed the influence of Fe2+ on the function of ALKBH5: the octahedral structure formed by the binding of Fe2+ to the enzyme could promote its stability, and make residues near the key motif HX (D/E) in the catalytic pocket more hydrophobic (Feng et al., 2014). The hydrophobic environment was favorable for the enzyme to bind to substrates and catalyze reactions. Additionally, the binding of ALKBH5 to primary and secondary substrates required the mediation of Fe2+ (Zheng et al., 2014). Research had found that ALKBH5 could regulate ferroptosis (Lv et al., 2023). However, whether Fe metabolism and ferroptosis affect the function of ALKBH5 remained unreported at present (Figure 2).
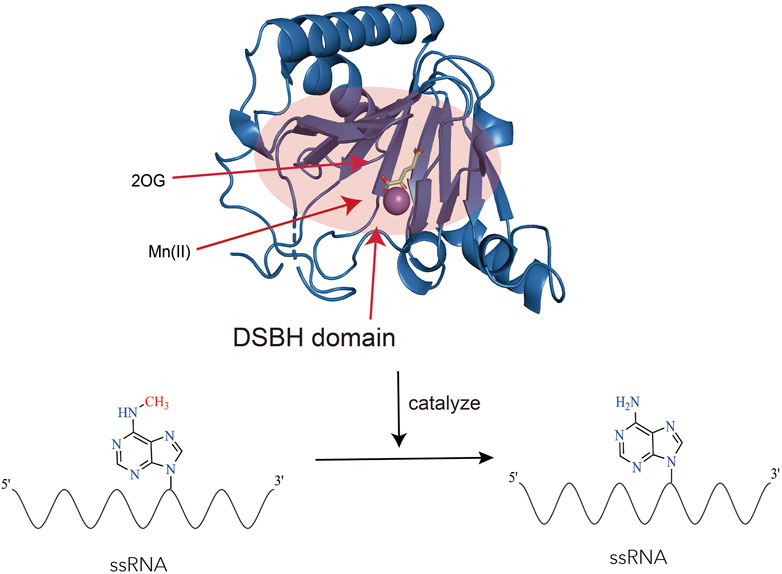
Figure 2. The structure and function of ALKBH5. The figure showcases the structure of ALKBH5 (Protein Data Bank ID: 4OCT) in complex with Mn(II) and 2-oxoglutarate (2OG). Mn(II) is a substitute for Fe(II) (Xu et al., 2014). The highlighted red area corresponds to the DSBH (Double-Stranded Beta-Helix) domain, which possesses the ability to bind to Fe(II), 2-oxoglutarate (2OG), and single-stranded RNA (ssRNA). Within this region, ALKBH5 performs a vital function by catalyzing the removal of N6-methyladenosine (m6A) modifications from RNA.
3 The impact of ALKBH5 on cellular functions in fibrosis
Fibrosis referred to the excessive activation of fibroblasts, leading to the deposition of extracellular matrix components, including collagen, in response to various stimulus. The activation and transformation of fibroblasts into myofibroblasts were key events in fibrosis. Myofibroblasts could also undergo transformation from other cell types, such as epithelial/endothelial cells through epithelial/endothelial-mesenchymal transition (EMT/EndMT) and from macrophages through macrophage-to-myofibroblast transition (MMT). Additionally, various cell types, including parenchymal cells, immune cells, macrophages, and endothelial cells (ECs), played important roles in various stages of fibrosis process. Current research had found that ALKBH5 regulated the functions of these cells in different ways (Table 1).
3.1 The impact of ALKBH5 on parenchymal cells
ALKBH5 played significant roles in the repair process of cardiomyocytes injury, which was essentially a tissue damage repair mechanism. In general, it was believed that in adult mammals, when cardiomyocytes suffered significant and sustained damage, fibrosis was the main mechanism for repair, due to their lack of regenerative capacity (Henderson et al., 2020).
Mitsugumin 53 (MG53), also known as TRIM72, was a potential cardiac protective protein (Zhao et al., 2024). When cardiomyocytes experienced acute ischemia and hypoxia, ALKBH5 inhibited the m6A methylation of MG53, preventing its degradation. This inhibition suppressed apoptosis and oxidative stress in cardiomyocytes, thereby reducing cell death (Li et al., 2024a). Following the restoration of blood supply, there was a high level of oxidative stress in the tissues, resulting in ischemia/reperfusion (I/R) injury (Metra and Teerlink, 2017). ALKBH5 had protective properties on cell proliferation, injury, and apoptosis during myocardial I/R injury (Li et al., 2022). ALKBH5 could also inhibit I/R-induced autophagy and apoptosis through the EGFR/PI3K/AKT/mTOR pathway (Wang et al., 2024). Additionally, ALKBH5 stabilized SIRT1 mRNA, inhibiting oxidative stress and apoptosis in cardiomyocytes induced by I/R (Liu and Liu, 2023). STAT3 downregulation could aggravate I/R injury in aging cardiomyocytes, while ALKBH5 regulated STAT3 expression by mediating long non-coding RNA (lncRNA) H19/miR-124-3p, thereby alleviating cardiomyocytes damage (Zhang et al., 2023). Therefore, ALKBH5 exerted a protective effect on cardiomyocytes by controlling the stability of various RNAs, potentially reducing the occurrence of cardiac fibrosis following myocardial infarction (MI). However, different results had also been reported. In diabetic cardiomyopathy, ALKBH5 regulated FOXO3 through m6A demethylation in m6A-YTHDF2-dependent manner, activating the CDR1/Hippo signaling pathway ultimately aggravating high glucose-induced cardiomyocytes apoptosis (Shao et al., 2022). ALKBH5 could exacerbate doxorubicin-induced cardiomyocytes apoptosis by regulating AT-Rich Interaction Domain 2 (ARID2) expression (Chen et al., 2024).
ALKBH5 also played a significant role in hepatocytes. In the mouse model of non-alcoholic fatty liver disease (NAFLD), inhibiting the activity of ALKBH5 significantly induced hepatocytes autophagy by reducing the stability of AXL mRNA (Meng et al., 2023).
3.2 The impact of ALKBH5 on immune cells
ALKBH5 played crucial roles in regulating the functions of inflammatory cells such as neutrophils and T lymphocytes, which were important in fibrosis. ALKBH5 played important roles in emergency granulopoiesis and neutrophil mobilization. In a mouse model of polymicrobial sepsis induced by cecal ligation puncture (CLP), ALKBH5 promoted G-CSF factor-driven emergency granulopoiesis and neutrophil mobilization by upregulating G-CSFR expression. ALKBH5 depletion significantly impaired the production of immature neutrophils and leaded to higher retention of mature neutrophils in the bone marrow (Liu et al., 2024). Furthermore, in a mouse model of polymicrobial sepsis induced by CLP, ALKBH5 regulated the expression of molecules involved in neutrophil migration, increasing the expression of CXCR2 and NLRP12, which promoted neutrophil migration, while decreasing the expression of PTGER4, TNC, and WNK1, which inhibited neutrophil migration. Deficiency of ALKBH5 impaired neutrophil migration (Liu et al., 2022). It seemed that ALKBH5 could promote the infiltration of neutrophils.
Different types of T lymphocytes also played important roles during the inflammatory phase of fibrosis. CD4+ T lymphocytes could produce the cytokine IFN-γ, which further activated macrophages (Mosser and Edwards, 2008). During neuroinflammation, ALKBH5 decreased m6A modification of IFNG mRNA of CD4+ T cells, leading to increased mRNA stability, thus promoting the secretion of IFN-γ (Zhou et al., 2021). At the same time, ALKBH5 mediated the stability of CXCL2 mRNA in CD4+ T cells through m6A, affecting CXCL2 expression in CD4+ T cells, resulting in promoted CD4+ T cell response and neutrophil recruitment (Zhou et al., 2021). ALKBH5 had additional functions in regulating the development and function of γδ T cells and mature T cells. ALKBH5 regulated the expression levels of Jagged1 and Notch2 in lymphocytes, changing the Jagged1/Notch2 signaling pathway, affecting the development of γδ T cell precursors, and thus reduce the number of mature γδ T cells (Ding et al., 2022). Furthermore, in a case-control study of lupus erythematosus, it was found that overexpression of ALKBH5 promoted apoptosis of mature T cells and inhibit T cell proliferation (Deng et al., 2022). The interaction between programmed death-ligand 1 (PD-L1) and programmed cell death protein 1 (PD-1) triggered immune inhibitory signals, suppressing the activity of T cells and other immune cells (Perry et al., 2022). ALKBH5 decreased the expression of PD-L1 in glioblastoma (GB) by regulating the stability of ZDHHC3 mRNA. As a result, there was increased infiltration of cytotoxic lymphocytes and pro-inflammatory cytokines in the cerebrospinal fluid (Tang et al., 2022). In a male mouse model of kidney injury, it was found that inhibiting ALKBH5 enhanced the m6A modification and stability of CCL28 mRNA, thereby regulating the recruitment of Treg cells, ultimately alleviating macrophage and neutrophil infiltration (Chen et al., 2023a). In summary, ALKBH5 could regulate the function of neutrophils and various types of T cells, including CD4+ T cells, γδ T cells and mature T cells. However, further research was needed to fully understand the impact of ALKBH5-mediated regulation of these immune cell functions on fibrosis.
3.3 The impact of ALKBH5 on macrophages
The macrophages derived from monocytes were crucial in fibrosis (Gordon and Taylor, 2005; Misharin et al., 2017). ALKBH5 regulated the function of macrophages through various mechanisms. Firstly, ALKBH5 promoted the recruitment of macrophages (Wei et al., 2022). In glioblastoma multiforme (GBM), ALKBH5 regulated the m6A modification of lncRNA NEAT1, leading to increased expression of interleukin-8 (IL-8), which promoted macrophage recruitment (Dong et al., 2021). Research had also found that ALKBH5 regulated the expression of MAP3K8 in hepatocellular carcinoma (HCC) cells and modulated IL-8 expression through the JNK and ERK pathways, promoting macrophage recruitment (You et al., 2022).
Secondly, ALKBH5 affected macrophage polarization. Activated macrophages could polarize into M1 (proinflammatory) or M2 (anti-inflammatory/pro-fibrotic) macrophages and performed different functions in different conditions and stages (Adhyatmika et al., 2015; Wang Y. et al., 2023). Several studies had shown that ALKBH5 played significant roles in macrophage polarization. A20 was considered as an anti-inflammatory molecule that inhibited inflammation and blocked the activation of various signaling pathways. In the diabetic retinopathy, ALKBH5-mediated m6A modification leaded to decreased A20 expression, ultimately enhancing M1 polarization of retinal microglia (Chen et al., 2022). However, the impact of ALKBH5 on macrophage polarization seemed to be bidirectional (Jiang et al., 2020). Research had found that ALKBH5 promoted monocyte infiltration and M2 polarization through the regulation of CCL5 production in radiation-induced-Hepatic stellate cells (HSCs), thereby promoted radiation-induced liver fibrosis (RILF) (Chen Y. et al., 2023). ALKBH5 could also affect macrophage recruitment and M2 differentiation by regulating the secretion of vascular endothelial growth factor (VEGF) (Zhao et al., 2022). In lung adenocarcinoma (LUAD), ALKBH5 regulated macrophages M2 polarization through CDCA4 (Tan et al., 2024). Therefore, the impact of ALKBH5 on macrophage polarization stilled remain controversial and might be influenced by different conditions and stages.
Besides, ALKBH5 affected MMT and macrophage autophagy, thereby regulating fibrosis. MMT was an important source of myofibroblasts. Under angiotensin II (AngII)-induced hypertension, cardiac macrophages derived from circulating monocytes preferentially underwent MMT. ALKBH5 mediated m6A demethylation of IL-11 mRNA, leading to increased stability and protein level of IL-11, promoting AngII-induced MMT, and thus resulted in cardiac fibrosis and dysfunction (Zhuang et al., 2024). There was also research suggesting that ALKBH5 reduced Slamf7 mRNA stability in an m6A-dependent manner, promoting macrophage autophagy and reducing the secretion of pro-inflammatory cytokines, thereby mediated silica particles-induced pulmonary inflammation (Yin et al., 2024).
3.4 The impact of ALKBH5 on endothelial cells
Vascular ECs and angiogenesis played important roles in fibrosis (Fan et al., 2023). Studies suggested that ALKBH5 might regulate the function of vascular ECs and thus modulated angiogenesis. VEGF, WNT signaling pathway, and hypoxia signaling were known regulators of angiogenesis (Carmeliet and Jain, 2011; Cui et al., 2018). Research had shown that overexpression of ALKBH5 increased VEGF-A secretion, promoted EC proliferation, migration, and tube formation, and regulated vessel formation. ALKBH5 knockdown impaired VEGF-A secretion in both in vitro and in vivo settings in GBM cells, decreasing the pro-angiogenesis ability of GBM cells (Tao et al., 2022; Fan et al., 2024). ALKBH5 overexpression elevated VEGF-A secretion in retinal pigment epitheliums (RPEs), thereby accelerating choroidal neovascularization progression in age-related macular degeneration (Sun et al., 2023). During ischemia, ALKBH5 decreased the m6A level on sphingosine kinase 1 (SPHK1) mRNA in ECs, increasing its stability and protein level, promoting vascular generation after acute ischemic stress (Kumari et al., 2021). ALKBH5 had also been found to regulate other extracellular mediators that affected angiogenesis. For example, connective tissue growth factor (CTGF), a vascular growth factor, showed significantly reduced expression in ALKBH5 knocked-out breast cancer cells (Panneerdoss et al., 2018). Increasing ALKBH5 level promoted angiogenesis by regulating CTGF production. ALKBH5 also influenced angiogenesis by modulating m6A level on non-coding RNA. lncRNA PVT1 promoted vascular generation in lung cancer tissue, and ALKBH5 reduced m6A modification on PVT1, thereby facilitating vascular generation in lung cancer (Shen et al., 2022). ALKBH5 could also affect angiogenesis by regulating circular RNA. In HCC, ALKBH5 regulated m6A modification on circ-CCT3, affecting angiogenesis (Liu et al., 2023). These studies suggested a potential role of ALKBH5 in promoting angiogenesis. However, there were also reports with opposing findings. For instance, it had been found that downregulation of ALKBH5 in vascular ECs under hypoxic conditions regulated the expression of WNT5A in an m6A-dependent manner, increasing its stability and promoting vascular generation phenotypes (Zhao et al., 2021).
3.5 The impact of ALKBH5 on fibroblasts
Activation of fibroblasts and their transformation into myofibroblasts were crucial steps in fibrosis (Frangogiannis, 2021). ALKBH5 had been found to regulate the activation of fibroblasts. During the post- MI healing process, ALKBH5 promoted the transformation of cardiac fibroblasts into myofibroblasts and improved collagen repair after MI by enhancing the stability of ErbB4 mRNA (Yang et al., 2023). In a mouse model of silica-induced pulmonary fibrosis, ALKBH5 promoted lung fibroblast activation and silica-induced pulmonary fibrosis via the miR-320a-3p/FOXM1 axis or targeting FOXM1 directly (Sun et al., 2022b).
ALKBH5 had also been found to regulate the transformation of multiple mesenchymal cell types into myofibroblasts. HSCs could transit from a quiescent state to a proliferative myofibroblast phenotype in response to liver injury (Trivedi et al., 2021). In vitro and in vivo models, including HSCs and clinical cases or CCl4-induced mice liver fibrosis, ALKBH5 triggered PTCH1 activation in an m6A-dependent manner, leading to hedgehog signaling inactivation, which inhibited the transformation of HSCs into myofibroblasts and ameliorated liver fibrosis (Yang et al., 2022). Furthermore, ALKBH5 reduced Drp1 methylation in an m6A-YTHDF1-dependent manner, inhibiting HSC proliferation, and migration, thereby ameliorating liver fibrosis (Wang J. et al., 2023).
Myofibroblasts could also undergo transformation from epithelial cells and macrophages. In a mouse model of renal fibrosis induced by unilateral ureteral obstruction (UUO). Knockdown of ALKBH5 enhanced the expression of mesenchymal markers, such as α-smooth muscle actin and snail, while overexpression of ALKBH5 increased the expression of the epithelial adhesion molecule E-cadherin and decreases snail expression, alleviating renal fibrosis (Ning et al., 2020), suggesting that ALKBH5 might play an important role in the EMT process. MMT was another important source of myofibroblasts. Under AngII-induced hypertension, ALKBH5 mediated the m6A demethylation of IL-11 mRNA, leading to increased stability and protein level of IL-11, promoting AngII-induced MMT, resulting in cardiac fibrosis and functional impairment (Zhuang et al., 2024).
4 The impact of ALKBH5 on organ fibrosis
4.1 The impact of ALKBH5 on pulmonary fibrosis
The occurrence of pulmonary fibrosis could be caused by factors such as radiation, inhalation of substances (crystalline silica, PM2.5), and chronic inflammation. ALKBH5 had been found to regulate pulmonary fibrosis in various animal models. Long-term exposure to crystalline silica could cause chronic respiratory disease and lead to silicosis, a disease characterized by diffuse fibrosis of the lung tissue (Hornung et al., 2008). Studie had discovered that ALKBH5 could regulate fibroblast-to-myofibroblast differentiation via the miR-320a-3p/FOXM1 axis or targeting FOXM1 directly, promoting silica-induced pulmonary fibrosis (Sun et al., 2022b). These studies suggested that ALKBH5 might promote the occurrence of pulmonary fibrosis.
However, some studies had also found that downregulating ALKBH5 might promote the development of pulmonary fibrosis. Exposure to 1-nitropyrene (1-NP) could cause pulmonary fibrosis in mice. Research had found that 1-NP promoted ALKBH5 degradation, regulating lung epithelial cells senescence through FBXW7 m6A modification, ultimately leading to 1-NP-induced pulmonary fibrosis (Li SR. et al., 2024). Radiation-induced pulmonary fibrosis (RIPF) was a common complication of thoracic radiation therapy (Hanania et al., 2019). ALKBH5 mediated the demethylation of IL-6 mRNA by silencing zinc finger and BTB domain-containing protein 7B (Zbtb7b), inhibiting its nuclear export, and suppressing the production of IL-6 in the lung, thereby slowing the development of RIPF (Zhao et al., 2020). In silica-induced pulmonary fibrotic tissue, ALKBH5 reduced Slamf7 through an m6A-dependent mechanism, promoting macrophage autophagy and reducing the secretion of pro-inflammatory cytokines, thereby inhibiting silica-induced lung inflammation (Yin et al., 2024). Similar to inhalation of crystalline silica, long-term exposure to PM2.5 could lead to pulmonary fibrosis. Knockdown of ALKBH5 significantly upregulated the YAP1 signaling pathway in NIH3T3 cells and lung tissue, promoting extracellular matrix deposition in PM2.5 exposure-induced pulmonary fibrosis of mice (Zhang et al., 2022). Therefore, the impact of ALKBH5 on pulmonary fibrosis still requires further elucidation (Table 2).
4.2 The impact of ALKBH5 on liver fibrosis
The occurrence of liver fibrosis could be caused by multiple factors, and ALKBH5 played significant roles in this process (Paternostro and Trauner, 2022; Chen et al., 2023c). In a mouse model of NAFLD, inhibiting ALKBH5 regulated hepatic autophagy flux, thereby alleviating hepatic steatosis and fibrosis (Meng et al., 2023). HSCs were the major fibrotic cells in the liver and could transform into activated myofibroblast-like cells upon activation, secreting extracellular matrix components involved in the formation of liver fibrosis and structural remodeling (Tsuchida and Friedman, 2017). Mitochondrial homeostasis played an important role in the progression of liver fibrosis (An et al., 2020; Wu et al., 2023). ALKBH5 inhibited mitochondrial fission and HSC proliferation and migration by reducing Drp1 methylation in an m6A-YTHDF1-dependent manner, ultimately ameliorating liver fibrosis (Wang J. et al., 2023). Furthermore, ALKBH5 could mediate the activation of PTCH1 through an m6A-dependent mechanism. Upregulation of PTCH1 leaded to inactivation of the Hedgehog signaling pathway, inhibiting HSC activation and ameliorating liver fibrosis (Yang et al., 2022). It seemed that ALKBH5 ameliorated liver fibrosis. However, in radiation-induced HSCs (radiation could induce HSC activation and promote RILF, which was a common complication of HCC radiotherapy (Kim and Jung, 2017). ALKBH5 mediated the demethylation of toll-interleukin 1 receptor domain-containing adaptor protein (TIRAP) mRNA through m6A modification, activating the downstream NF-κB and JNK/Smad2 pathways, and promoting radiation-induced HSC activation. Knockdown of ALKBH5 significantly alleviated RILF in mice (Chen Y. et al., 2023). These suggested that ALKBH5 could improve RILF (Table 2).
4.3 The impact of ALKBH5 on cardiac fibrosis
The injured myocardium was replaced by scar tissue, and excessive myocardial injury could lead to cardiac fibrosis (Bergmann et al., 2009). Myocardial ischemia and hypoxia were the primary causes of myocardial injury and cardiac fibrosis. During acute ischemia and hypoxia in the myocardium, ALKBH5 could influence the extent of MI and cardiac fibrosis through multiple mechanisms (Wang et al., 2021; Cheng et al., 2022; Li et al., 2024b). After the ischemic myocardium was reperfused, ALKBH5 inhibited H/R-induced autophagy and apoptosis in cardiomyocytes through pathways such as EGFR/PI3K/AKT/mTOR (Liu and Liu, 2023; Wang et al., 2024). During the repair phase of MI, ALKBH5 enhanced the stability of ErbB4 mRNA through m6A demethylation, promoting the transformation of fibroblasts into myofibroblasts and improving post-MI fibrotic repair (Yang et al., 2023). ALKBH5 also contributed to maintaining post-acute ischemic stress-induced angiogenesis by reducing SPHK1 mRNA m6A methylation, thereby improving post-MI fibrosis progression (Kumari et al., 2021). While it had long been believed that mammalian cardiomyocytes had limited ability to re-enter the cell cycle and primarily relied on fibrotic repair after myocardial injury (Bergmann et al., 2009; Weinberger and Riley, 2024), the concept of cardiac regeneration had gained attention among researchers with advancements in scientific technology (Weinberger and Riley, 2024). Studies had reported that ALKBH5, through the ALKBH5-m6A-YTHDF1-YAP axis, regulated cardiomyocytes re-entry into the cell cycle and promoted cardiomyocyte proliferation after MI (Han et al., 2021). These studies suggested that ALKBH5 was beneficial for the repair of ischemia-induced myocardial injury and improvement of cardiac fibrosis.
Diabetic cardiomyopathy was also a common cause of cardiac fibrosis (Zhang et al., 2019). ALKBH5 activated FOXO3 through m6A demethylation in an m6A-YTHDF2-dependent manner, activating the CDR1/Hippo signaling pathway, ultimately aggravating high glucose-induced cardiomyocytes apoptosis (Shao et al., 2022). Drug toxicity could also lead to cardiac fibrosis, with drugs like doxorubicin exhibiting dose-dependent cardiac toxicity that could result in heart failure and interstitial fibrosis (Deng et al., 2007). ARID2, serving as a downstream effector of ALKBH5 in cardiomyocytes, regulated the DNA damage response and enhanced doxorubicin-induced cardiomyocyte apoptosis, ALKBH5 could affect the role of doxorubicin-induced cardiac dysfunction, remodeling, and cardiomyocyte apoptosis by regulating ARID2 expression (Chen et al., 2024). Under AngII-induced hypertension, ALKBH5 mediated m6A demethylation of IL-11 mRNA to increase the stability IL-11, promoting AngII-induced MMT, and resulted in cardiac fibrosis (Zhuang et al., 2024).
4.4 The impact of ALKBH5 on renal fibrosis
In a mouse model of renal fibrosis induced by UUO, overexpression of ALKBH5 could increase the expression of the renal epithelial adhesion molecule E-cadherin while decreasing the expression of snail, thereby alleviating UUO-induced renal fibrosis by affecting the EMT process. This suggested that ALKBH5 could slow down the progression of kidney fibrosis (Ning et al., 2020). However, different results had also been reported. For example, studies found that inhibiting ALKBH5 reduced glomerular inflammation and excessive proliferation of mesangial cells through the modification of TRIM13-m6A in glomerular mesangial cells, thereby slowing the development of chronic glomerulonephritis. This suggested that inhibiting ALKBH5 could slow down the progression of kidney fibrosis (Hu et al., 2024). In the I/R-induced renal injury model of male mice, researchers found that knocking down ALKBH5 increased the expression of CCL28 through m6A modification, regulated the downstream CCL28/Treg/inflammatory cell axis, thereby alleviating I/R-induced acute kidney injury and renal fibrosis. This also suggested that inhibiting ALKBH5 could slow down the progression of renal fibrosis (Chen et al., 2023a). Therefore, the effect of ALKBH5 on renal fibrosis needed further study (Table 2).
5 Applications of ALKBH5 research: inhibitors
Several inhibitors of ALKBH5 had been discovered in current research. 2OG-dependent oxygenase’s inhibitors such as NOG, PDCA, and HIF PHD inhibitor IOX3 could weakly inhibit ALKBH5 activity by competing with 2OG, but they lack selectivity (Aik et al., 2014). Among them, 4-PDCA exhibited the strongest inhibitory effect, while NOG had the weakest inhibitory effect. The imidazobenzoxazin-5-thione (MV1035), a novel sodium channel blocker, partially overlapped with the 2OG binding site of ALKBH5. MV1035 inhibited the catalytic activity of ALKBH5 by competing with 2OG at the active site, reducing GBM cells migration and invasiveness (Malacrida et al., 2020; Malacrida et al., 2022). Dexmedetomidine had been found to inhibit ALKBH5 expression in vitro and decrease inflammation cytokine production of LPS-treated HK-2 cells (Zhu and Lu, 2020). However, these inhibitors were not primarily targeted at ALKBH5 demethylase.
Specific inhibitors targeting ALKBH5 were gradually being discovered. Researchers identified two novel ALKBH5 inhibitors, Ena15 and Ena21. Ena21 was a selective competitive inhibitor of ALKBH5, which could compete with 2OG for the active site of ALKBH5 and inhibit ALKBH5 activity, while Ena15 was a non-competitive inhibitor of ALKBH5. Both of them could inhibit the growth activity of GBM (Takahashi et al., 2022). Pyrazolo [1,5-a]pyrimidine Derivative DDO-2728 had also been found to act as a selective inhibitor of ALKBH5, significantly suppressing tumor growth in the MV4-11 xenograft mouse model (Wang YZ. et al., 2023). A drug with a 1-phenyl-1H-pyrazole scaffold called 20m, which had been identified as an effective, selective, and cell-active ALKBH5 inhibitor (Fang et al., 2022). Scientists had also shared a novel small molecule RNA demethylase ALKBH5 inhibitor for targeted cancer therapy (Sabnis, 2021). The selection of ALKBH5 inhibitors specific to specific cells or even specific mechanisms of action would be the focus of future research, relying on a deeper understanding of the mechanisms of ALKBH5 action.
In fact, there was a natural inhibitor of ALKBH5 in cellular environments: citrate. Citrate molecules bound to ALKBH5 demethylase, displacing the metal ions and 2OG in ALKBH5, thereby inhibiting its demethylation activity (Xu et al., 2014). Chlorogenic acid (CGA), a naturally occurring plant component, could inhibit ALKBH5 activity to regulate autophagy and alleviate hepatic steatosis (Meng et al., 2023) (Table 3).
6 Conclusion
ALKBH5 can regulate the functions of various cells, including parenchymal cells (hepatocytes and cardiomyocytes), immune cells (neutrophils and T cells), macrophages, endothelial cells, and fibroblasts, through different signaling pathways and mechanisms, ultimately affecting tissue fibrosis in organs such as the heart, liver, lung and kidney. The impact of ALKBH5 on fibrotic outcomes varies and may be influenced by different cell types, stimulation conditions, and research models. The focus of future research will be how to select ALKBH5 inhibitors targeting specific cells or even specific mechanisms of action, which relies on a deeper understanding of the mechanisms of ALKBH5.
Author contributions
ZL: Writing–original draft. JW: Writing–original draft. MX: Writing–original draft. XL: Writing–review and editing. HX: Writing–review and editing.
Funding
The author(s) declare that financial support was received for the research, authorship, and/or publication of this article. This study was supported by National Natural Science Foundation of China (Nos. 82272270 and 82171121) and the Science and Technology Commission of Shanghai Municipality Funds (22ZR1451700).
Conflict of interest
The authors declare that the research was conducted in the absence of any commercial or financial relationships that could be construed as a potential conflict of interest.
Publisher’s note
All claims expressed in this article are solely those of the authors and do not necessarily represent those of their affiliated organizations, or those of the publisher, the editors and the reviewers. Any product that may be evaluated in this article, or claim that may be made by its manufacturer, is not guaranteed or endorsed by the publisher.
References
Adhyatmika, A., Putri, K. S., Beljaars, L., and Melgert, B. N. (2015). The elusive antifibrotic macrophage. Front. Med. (Lausanne) 2, 81. doi:10.3389/fmed.2015.00081
Aik, W., Scotti, J. S., Choi, H., Gong, L., Demetriades, M., Schofield, C. J., et al. (2014). Structure of human RNA N⁶-methyladenine demethylase ALKBH5 provides insights into its mechanisms of nucleic acid recognition and demethylation. Nucleic Acids Res. 42 (7), 4741–4754. doi:10.1093/nar/gku085
An, P., Wei, L. L., Zhao, S., Sverdlov, D. Y., Vaid, K. A., Miyamoto, M., et al. (2020). Hepatocyte mitochondria-derived danger signals directly activate hepatic stellate cells and drive progression of liver fibrosis. Nat. Commun. 11 (1), 2362. doi:10.1038/s41467-020-16092-0
Bergmann, O., Bhardwaj, R. D., Bernard, S., Zdunek, S., Barnabé-Heider, F., Walsh, S., et al. (2009). Evidence for cardiomyocyte renewal in humans. Science 324 (5923), 98–102. doi:10.1126/science.1164680
Cai, Z., Zhang, Y., Yang, L., Ma, C., Fei, Y., Ding, J., et al. (2022). ALKBH5 in mouse testicular Sertoli cells regulates Cdh2 mRNA translation to maintain blood-testis barrier integrity. Cell Mol. Biol. Lett. 27 (1), 101. doi:10.1186/s11658-022-00404-x
Carmeliet, P., and Jain, R. K. (2011). Molecular mechanisms and clinical applications of angiogenesis. Nature 473 (7347), 298–307. doi:10.1038/nature10144
Chen, J., Xu, C., Yang, K., Gao, R., Cao, Y., Liang, L., et al. (2023a). Inhibition of ALKBH5 attenuates I/R-induced renal injury in male mice by promoting Ccl28 m6A modification and increasing Treg recruitment. Nat. Commun. 14 (1), 1161. doi:10.1038/s41467-023-36747-y
Chen, J., Ye, M., Bai, J., Gong, Z., Yan, L., Gu, D., et al. (2023c). ALKBH5 enhances lipid metabolism reprogramming by increasing stability of FABP5 to promote pancreatic neuroendocrine neoplasms progression in an m6A-IGF2BP2-dependent manner. J. Transl. Med. 21 (1), 741. doi:10.1186/s12967-023-04578-6
Chen, R., Zhang, G., Sun, K., and Chen, A. F. (2024). Aging-associated ALKBH5-m(6)A modification exacerbates doxorubicin-induced cardiomyocyte apoptosis via AT-rich interaction domain 2. J. Am. Heart Assoc. 13 (1), e031353. doi:10.1161/JAHA.123.031353
Chen, T., Zhu, W., Wang, C., Dong, X., Yu, F., Su, Y., et al. (2022). ALKBH5-Mediated m(6)A modification of A20 regulates microglia polarization in diabetic retinopathy. Front. Immunol. 13, 813979. doi:10.3389/fimmu.2022.813979
Chen, Y., Zhou, P., Deng, Y., Cai, X., Sun, M., Sun, Y., et al. (2023b). ALKBH5-mediated m(6) A demethylation of TIRAP mRNA promotes radiation-induced liver fibrosis and decreases radiosensitivity of hepatocellular carcinoma. Clin. Transl. Med. 13 (2), e1198. doi:10.1002/ctm2.1198
Cheng, P., Han, H., Chen, F., Cheng, L., Ma, C., Huang, H., et al. (2022). Amelioration of acute myocardial infarction injury through targeted ferritin nanocages loaded with an ALKBH5 inhibitor. Acta Biomater. 140, 481–491. doi:10.1016/j.actbio.2021.11.041
Cui, X., Morales, R. T., Qian, W., Wang, H., Gagner, J. P., Dolgalev, I., et al. (2018). Hacking macrophage-associated immunosuppression for regulating glioblastoma angiogenesis. Biomaterials 161, 164–178. doi:10.1016/j.biomaterials.2018.01.053
Deng, L. J., Fang, X. Y., Wu, J., Li, Q. R., Mao, Y. M., Leng, R. X., et al. (2022). ALKBH5 expression could affect the function of T cells in systemic lupus erythematosus patients: a case-control study. Curr. Pharm. Des. 28 (27), 2270–2278. doi:10.2174/1381612828666220617154204
Deng, S., Kulle, B., Hosseini, M., Schlüter, G., Hasenfuss, G., Wojnowski, L., et al. (2007). Dystrophin-deficiency increases the susceptibility to doxorubicin-induced cardiotoxicity. Eur. J. Heart Fail 9 (10), 986–994. doi:10.1016/j.ejheart.2007.07.016
Ding, C., Xu, H., Yu, Z., Roulis, M., Qu, R., Zhou, J., et al. (2022). RNA m(6)A demethylase ALKBH5 regulates the development of γδ T cells. Proc. Natl. Acad. Sci. U. S. A. 119 (33), e2203318119. doi:10.1073/pnas.2203318119
Dong, F., Qin, X., Wang, B., Li, Q., Hu, J., Cheng, X., et al. (2021). ALKBH5 facilitates hypoxia-induced paraspeckle assembly and IL8 secretion to generate an immunosuppressive tumor microenvironment. Cancer Res. 81 (23), 5876–5888. doi:10.1158/0008-5472.CAN-21-1456
Fan, M., Yang, K., Wang, X., Chen, L., Gill, P. S., Ha, T., et al. (2023). Lactate promotes endothelial-to-mesenchymal transition via Snail1 lactylation after myocardial infarction. Sci. Adv. 9 (5), eadc9465. doi:10.1126/sciadv.adc9465
Fan, Y., Yan, D., Ma, L., Liu, X., Luo, G., Hu, Y., et al. (2024). ALKBH5 is a prognostic factor and promotes the angiogenesis of glioblastoma. Sci. Rep. 14 (1), 1303. doi:10.1038/s41598-024-51994-9
Fang, Z., Mu, B., Liu, Y., Guo, N., Xiong, L., Guo, Y., et al. (2022). Discovery of a potent, selective and cell active inhibitor of m(6)A demethylase ALKBH5. Eur. J. Med. Chem. 238, 114446. doi:10.1016/j.ejmech.2022.114446
Feng, C., Liu, Y., Wang, G., Deng, Z., Zhang, Q., Wu, W., et al. (2014). Crystal structures of the human RNA demethylase Alkbh5 reveal basis for substrate recognition. J. Biol. Chem. 289 (17), 11571–11583. doi:10.1074/jbc.M113.546168
Frangogiannis, N. G. (2021). Cardiac fibrosis. Cardiovasc Res. 117 (6), 1450–1488. doi:10.1093/cvr/cvaa324
Gordon, S., and Taylor, P. R. (2005). Monocyte and macrophage heterogeneity. Nat. Rev. Immunol. 5 (12), 953–964. doi:10.1038/nri1733
Han, Z., Wang, X., Xu, Z., Cao, Y., Gong, R., Yu, Y., et al. (2021). ALKBH5 regulates cardiomyocyte proliferation and heart regeneration by demethylating the mRNA of YTHDF1. Theranostics 11 (6), 3000–3016. doi:10.7150/thno.47354
Hanania, A. N., Mainwaring, W., Ghebre, Y. T., Hanania, N. A., and Ludwig, M. (2019). Radiation-induced lung injury: assessment and management. Chest 156 (1), 150–162. doi:10.1016/j.chest.2019.03.033
Henderson, N. C., Rieder, F., and Wynn, T. A. (2020). Fibrosis: from mechanisms to medicines. Nature 587 (7835), 555–566. doi:10.1038/s41586-020-2938-9
Hornung, V., Bauernfeind, F., Halle, A., Samstad, E. O., Kono, H., Rock, K. L., et al. (2008). Silica crystals and aluminum salts activate the NALP3 inflammasome through phagosomal destabilization. Nat. Immunol. 9 (8), 847–856. doi:10.1038/ni.1631
Hu, X., Liu, T., Zhuang, X., Wei, L., and Gao, J. (2024). Inhibition of ALKBH5 inhibits inflammation and excessive proliferation by promoting TRIM13 m6A modifications in glomerular mesangial cells. Naunyn Schmiedeb. Arch. Pharmacol. doi:10.1007/s00210-024-03057-z
Hu, Y., Gong, C., Li, Z., Liu, J., Chen, Y., Huang, Y., et al. (2022). Demethylase ALKBH5 suppresses invasion of gastric cancer via PKMYT1 m6A modification. Mol. Cancer 21 (1), 34. doi:10.1186/s12943-022-01522-y
Jiang, Y., Wan, Y., Gong, M., Zhou, S., Qiu, J., and Cheng, W. (2020). RNA demethylase ALKBH5 promotes ovarian carcinogenesis in a simulated tumour microenvironment through stimulating NF-κB pathway. J. Cell Mol. Med. 24 (11), 6137–6148. doi:10.1111/jcmm.15228
Jin, D., Guo, J., Wu, Y., Yang, L., Wang, X., Du, J., et al. (2020). m(6)A demethylase ALKBH5 inhibits tumor growth and metastasis by reducing YTHDFs-mediated YAP expression and inhibiting miR-107/LATS2-mediated YAP activity in NSCLC. Mol. Cancer 19 (1), 40. doi:10.1186/s12943-020-01161-1
Kim, J., and Jung, Y. (2017). Radiation-induced liver disease: current understanding and future perspectives. Exp. Mol. Med. 49 (7), e359. doi:10.1038/emm.2017.85
Kumari, R., Dutta, R., Ranjan, P., Suleiman, Z. G., Goswami, S. K., Li, J., et al. (2021). ALKBH5 regulates SPHK1-dependent endothelial cell angiogenesis following ischemic stress. Front. Cardiovasc Med. 8, 817304. doi:10.3389/fcvm.2021.817304
Li, D., Li, L., Dong, S., Yu, Y., Zhang, L., and Jiang, S. (2024a). Alkylation repair homolog 5 regulates N(6)-methyladenosine (m6A) methylation of mitsugumin 53 to attenuate myocardial infarction by inhibiting apoptosis and oxidative stress. J. Cardiovasc Pharmacol. 83 (2), 183–192. doi:10.1097/FJC.0000000000001515
Li, J., Chen, J., Zhao, M., Li, Z., Liu, N., Fang, H., et al. (2022). Downregulated ALKBH5 contributes to myocardial ischemia/reperfusion injury by increasing m(6)A modification of Trio mRNA. Ann. Transl. Med. 10 (7), 417. doi:10.21037/atm-22-1289
Li, S. R., Kang, N. N., Wang, R. R., Li, M. D., Chen, L. H., Zhou, P., et al. (2024b). ALKBH5 SUMOylation-mediated FBXW7 m6A modification regulates alveolar cells senescence during 1-nitropyrene-induced pulmonary fibrosis. J. Hazard Mater 468, 133704. doi:10.1016/j.jhazmat.2024.133704
Liu, H., Jiang, Y., Lu, J., Peng, C., Ling, Z., Chen, Y., et al. (2023). m(6)A-modification regulated circ-CCT3 acts as the sponge of miR-378a-3p to promote hepatocellular carcinoma progression. Epigenetics 18 (1), 2204772. doi:10.1080/15592294.2023.2204772
Liu, L., and Liu, Z. (2023). m(6)A eraser ALKBH5 mitigates the apoptosis of cardiomyocytes in ischemia reperfusion injury through m(6)A/SIRT1 axis. PeerJ 11, e15269. doi:10.7717/peerj.15269
Liu, Y., Song, R., Lu, Z., Zhao, L., Zhan, X., Li, Y., et al. (2024). The RNA m(6)A demethylase ALKBH5 drives emergency granulopoiesis and neutrophil mobilization by upregulating G-CSFR expression. Cell Mol. Immunol. 21 (1), 6–18. doi:10.1038/s41423-023-01115-9
Liu, Y., Song, R., Zhao, L., Lu, Z., Li, Y., Zhan, X., et al. (2022). m(6)A demethylase ALKBH5 is required for antibacterial innate defense by intrinsic motivation of neutrophil migration. Signal Transduct. Target Ther. 7 (1), 194. doi:10.1038/s41392-022-01020-z
Lv, D., Zhong, C., Dixit, D., Yang, K., Wu, Q., Godugu, B., et al. (2023). EGFR promotes ALKBH5 nuclear retention to attenuate N6-methyladenosine and protect against ferroptosis in glioblastoma. Mol. Cell 83 (23), 4334–4351.e7. doi:10.1016/j.molcel.2023.10.025
Malacrida, A., Di Domizio, A., Bentivegna, A., Cislaghi, G., Messuti, E., Tabano, S. M., et al. (2022). MV1035 overcomes temozolomide resistance in patient-derived glioblastoma stem cell lines. Biol. (Basel). 11 (1), 70. doi:10.3390/biology11010070
Malacrida, A., Rivara, M., Di Domizio, A., Cislaghi, G., Miloso, M., Zuliani, V., et al. (2020). 3D proteome-wide scale screening and activity evaluation of a new ALKBH5 inhibitor in U87 glioblastoma cell line. Bioorg Med. Chem. 28 (4), 115300. doi:10.1016/j.bmc.2019.115300
Meng, F., Song, C., Liu, J., Chen, F., Zhu, Y., Fang, X., et al. (2023). Chlorogenic acid modulates autophagy by inhibiting the activity of ALKBH5 demethylase, thereby ameliorating hepatic steatosis. J. Agric. Food Chem. 71 (41), 15073–15086. doi:10.1021/acs.jafc.3c03710
Metra, M., and Teerlink, J. R. (2017). Heart failure. Lancet 390 (10106), 1981–1995. doi:10.1016/S0140-6736(17)31071-1
Meyer, K. D., Saletore, Y., Zumbo, P., Elemento, O., Mason, C. E., and Jaffrey, S. R. (2012). Comprehensive analysis of mRNA methylation reveals enrichment in 3' UTRs and near stop codons. Cell 149 (7), 1635–1646. doi:10.1016/j.cell.2012.05.003
Misharin, A. V., Morales-Nebreda, L., Reyfman, P. A., Cuda, C. M., Walter, J. M., McQuattie-Pimentel, A. C., et al. (2017). Monocyte-derived alveolar macrophages drive lung fibrosis and persist in the lung over the life span. J. Exp. Med. 214 (8), 2387–2404. doi:10.1084/jem.20162152
Mosser, D. M., and Edwards, J. P. (2008). Exploring the full spectrum of macrophage activation. Nat. Rev. Immunol. 8 (12), 958–969. doi:10.1038/nri2448
Ning, Y., Chen, J., Shi, Y., Song, N., Yu, X., Fang, Y., et al. (2020). Genistein ameliorates renal fibrosis through regulation snail via m6A RNA demethylase ALKBH5. Front. Pharmacol. 11, 579265. doi:10.3389/fphar.2020.579265
Panneerdoss, S., Eedunuri, V. K., Yadav, P., Timilsina, S., Rajamanickam, S., Viswanadhapalli, S., et al. (2018). Cross-talk among writers, readers, and erasers of m(6)A regulates cancer growth and progression. Sci. Adv. 4 (10), eaar8263. doi:10.1126/sciadv.aar8263
Paternostro, R., and Trauner, M. (2022). Current treatment of non-alcoholic fatty liver disease. J. Intern Med. 292 (2), 190–204. doi:10.1111/joim.13531
Perry, J. A., Shallberg, L., Clark, J. T., Gullicksrud, J. A., DeLong, J. H., Douglas, B. B., et al. (2022). PD-L1-PD-1 interactions limit effector regulatory T cell populations at homeostasis and during infection. Nat. Immunol. 23 (5), 743–756. doi:10.1038/s41590-022-01170-w
Purslow, J. A., Nguyen, T. T., Egner, T. K., Dotas, R. R., Khatiwada, B., and Venditti, V. (2018). Active site breathing of human Alkbh5 revealed by solution NMR and accelerated molecular dynamics. Biophys. J. 115 (10), 1895–1905. doi:10.1016/j.bpj.2018.10.004
Sabnis, R. W. (2021). Novel small molecule RNA m6A demethylase AlkBH5 inhibitors for treating cancer. ACS Med. Chem. Lett. 12 (6), 856–857. doi:10.1021/acsmedchemlett.1c00102
Shao, Y., Li, M., Yu, Q., Gong, M., Wang, Y., Yang, X., et al. (2022). CircRNA CDR1as promotes cardiomyocyte apoptosis through activating hippo signaling pathway in diabetic cardiomyopathy. Eur. J. Pharmacol. 922, 174915. doi:10.1016/j.ejphar.2022.174915
Shen, W., Pu, J., Zuo, Z., Gu, S., Sun, J., Tan, B., et al. (2022). The RNA demethylase ALKBH5 promotes the progression and angiogenesis of lung cancer by regulating the stability of the LncRNA PVT1. Cancer Cell Int. 22 (1), 353. doi:10.1186/s12935-022-02770-0
Sun, R. X., Zhu, H. J., Zhang, Y. R., Wang, J. N., Wang, Y., Cao, Q. C., et al. (2023). ALKBH5 causes retinal pigment epithelium anomalies and choroidal neovascularization in age-related macular degeneration via the AKT/mTOR pathway. Cell Rep. 42 (7), 112779. doi:10.1016/j.celrep.2023.112779
Sun, W., Li, Y., Ma, D., Liu, Y., Xu, Q., Cheng, D., et al. (2022a). ALKBH5 promotes lung fibroblast activation and silica-induced pulmonary fibrosis through miR-320a-3p and FOXM1. Cell. and Mol. Biol. Lett. 27 (1), 26. doi:10.1186/s11658-022-00329-5
Sun, W., Li, Y., Ma, D., Liu, Y., Xu, Q., Cheng, D., et al. (2022b). ALKBH5 promotes lung fibroblast activation and silica-induced pulmonary fibrosis through miR-320a-3p and FOXM1. Cell Mol. Biol. Lett. 27 (1), 26. doi:10.1186/s11658-022-00329-5
Takahashi, H., Hase, H., Yoshida, T., Tashiro, J., Hirade, Y., Kitae, K., et al. (2022). Discovery of two novel ALKBH5 selective inhibitors that exhibit uncompetitive or competitive type and suppress the growth activity of glioblastoma multiforme. Chem. Biol. Drug Des. 100 (1), 1–12. doi:10.1111/cbdd.14051
Tan, J., Chen, F., Wang, J., Li, J., Ouyang, B., Li, X., et al. (2024). ALKBH5 promotes the development of lung adenocarcinoma by regulating the polarization of M2 macrophages through CDCA4. Gene 895, 147975. doi:10.1016/j.gene.2023.147975
Tang, C., Klukovich, R., Peng, H., Wang, Z., Yu, T., Zhang, Y., et al. (2018). ALKBH5-dependent m6A demethylation controls splicing and stability of long 3'-UTR mRNAs in male germ cells. Proc. Natl. Acad. Sci. U. S. A. 115 (2), E325–e33. doi:10.1073/pnas.1717794115
Tang, W., Xu, N., Zhou, J., He, Z., Lenahan, C., Wang, C., et al. (2022). ALKBH5 promotes PD-L1-mediated immune escape through m6A modification of ZDHHC3 in glioma. Cell Death Discov. 8 (1), 497. doi:10.1038/s41420-022-01286-w
Tao, M., Li, X., He, L., Rong, X., Wang, H., Pan, J., et al. (2022). Decreased RNA m(6)A methylation enhances the process of the epithelial mesenchymal transition and vasculogenic mimicry in glioblastoma. Am. J. Cancer Res. 12 (2), 893–906.
Toh, J. D. W., Crossley, S. W. M., Bruemmer, K. J., Ge, E. J., He, D., Iovan, D. A., et al. (2020). Distinct RNA N-demethylation pathways catalyzed by nonheme iron ALKBH5 and FTO enzymes enable regulation of formaldehyde release rates. Proc. Natl. Acad. Sci. U. S. A. 117 (41), 25284–25292. doi:10.1073/pnas.2007349117
Trivedi, P., Wang, S., and Friedman, S. L. (2021). The power of plasticity-metabolic regulation of hepatic stellate cells. Cell Metab. 33 (2), 242–257. doi:10.1016/j.cmet.2020.10.026
Tsuchida, T., and Friedman, S. L. (2017). Mechanisms of hepatic stellate cell activation. Nat. Rev. Gastroenterol. Hepatol. 14 (7), 397–411. doi:10.1038/nrgastro.2017.38
Wang, D., Wang, D., Jin, Q., and Wang, X. (2024). Suxiao Jiuxin Pill alleviates myocardial ischemia/reperfusion-induced autophagy via miR-193a-3p/ALKBH5 pathway. Phytomedicine 125, 155359. doi:10.1016/j.phymed.2024.155359
Wang, J., Yang, Y., Sun, F., Luo, Y., Yang, Y., Li, J., et al. (2023b). ALKBH5 attenuates mitochondrial fission and ameliorates liver fibrosis by reducing Drp1 methylation. Pharmacol. Res. 187, 106608. doi:10.1016/j.phrs.2022.106608
Wang, Y., Zhang, Y., Li, J., Li, C., Zhao, R., Shen, C., et al. (2023a). Hypoxia induces M2 macrophages to express VSIG4 and mediate cardiac fibrosis after myocardial infarction. Theranostics 13 (7), 2192–2209. doi:10.7150/thno.78736
Wang, Y. J., Yang, B., Lai, Q., Shi, J. F., Peng, J. Y., Zhang, Y., et al. (2021). Reprogramming of m(6)A epitranscriptome is crucial for shaping of transcriptome and proteome in response to hypoxia. RNA Biol. 18 (1), 131–143. doi:10.1080/15476286.2020.1804697
Wang, Y. Z., Li, H. Y., Zhang, Y., Jiang, R. X., Xu, J., Gu, J., et al. (2023c). Discovery of pyrazolo[1,5-a]pyrimidine derivative as a novel and selective ALKBH5 inhibitor for the treatment of AML. J. Med. Chem. 66 (23), 15944–15959. doi:10.1021/acs.jmedchem.3c01374
Wei, C., Wang, B., Peng, D., Zhang, X., Li, Z., Luo, L., et al. (2022). Pan-cancer analysis shows that ALKBH5 is a potential prognostic and immunotherapeutic biomarker for multiple cancer types including gliomas. Front. Immunol. 13, 849592. doi:10.3389/fimmu.2022.849592
Weinberger, M., and Riley, P. R. (2024). Animal models to study cardiac regeneration. Nat. Rev. Cardiol. 21 (2), 89–105. doi:10.1038/s41569-023-00914-x
Wu, N. N., Wang, L., Wang, L., Xu, X., Lopaschuk, G. D., Zhang, Y., et al. (2023). Site-specific ubiquitination of VDAC1 restricts its oligomerization and mitochondrial DNA release in liver fibrosis. Exp. Mol. Med. 55 (1), 269–280. doi:10.1038/s12276-022-00923-9
Xu, C., Liu, K., Tempel, W., Demetriades, M., Aik, W., Schofield, C. J., et al. (2014). Structures of human ALKBH5 demethylase reveal a unique binding mode for specific single-stranded N6-methyladenosine RNA demethylation. J. Biol. Chem. 289 (25), 17299–17311. doi:10.1074/jbc.M114.550350
Yang, J. J., Wang, J., Yang, Y., Yang, Y., Li, J., Lu, D., et al. (2022). ALKBH5 ameliorated liver fibrosis and suppressed HSCs activation via triggering PTCH1 activation in an m(6)A dependent manner. Eur. J. Pharmacol. 922, 174900. doi:10.1016/j.ejphar.2022.174900
Yang, K., Zhao, Y., Hu, J., Gao, R., Shi, J., Wei, X., et al. (2023). ALKBH5 induces fibroblast-to-myofibroblast transformation during hypoxia to protect against cardiac rupture after myocardial infarction. J. Adv. Res. 61, 193–209. doi:10.1016/j.jare.2023.09.004
Yin, H., Gu, P., Xie, Y., You, X., Zhang, Y., Yao, Y., et al. (2024). ALKBH5 mediates silica particles-induced pulmonary inflammation through increased m(6)A modification of Slamf7 and autophagy dysfunction. J. Hazard Mater 462, 132736. doi:10.1016/j.jhazmat.2023.132736
You, Y., Wen, D., Zeng, L., Lu, J., Xiao, X., Chen, Y., et al. (2022). ALKBH5/MAP3K8 axis regulates PD-L1+ macrophage infiltration and promotes hepatocellular carcinoma progression. Int. J. Biol. Sci. 18 (13), 5001–5018. doi:10.7150/ijbs.70149
Yu, F., Wei, J., Cui, X., Yu, C., Ni, W., Bungert, J., et al. (2021). Post-translational modification of RNA m6A demethylase ALKBH5 regulates ROS-induced DNA damage response. Nucleic Acids Res. 49 (10), 5779–5797. doi:10.1093/nar/gkab415
Zhai, J., Chen, H., Wong, C. C., Peng, Y., Gou, H., Zhang, J., et al. (2023). ALKBH5 drives immune suppression via targeting AXIN2 to promote colorectal cancer and is a target for boosting immunotherapy. Gastroenterology 165 (2), 445–462. doi:10.1053/j.gastro.2023.04.032
Zhang, W., Xu, W., Feng, Y., and Zhou, X. (2019). Non-coding RNA involvement in the pathogenesis of diabetic cardiomyopathy. J. Cell Mol. Med. 23 (9), 5859–5867. doi:10.1111/jcmm.14510
Zhang, X., Li, F., Ma, J., Zhang, X., and Yin, Y. (2023). ALKBH5 alleviates hypoxia postconditioning injury in D-galactose-induced senescent cardiomyocytes by regulating stat3. Shock 59 (1), 91–98. doi:10.1097/shk.0000000000002031
Zhang, Y., Liu, Q., Ning, J., Jiang, T., Kang, A., Li, L., et al. (2022). The proteasome-dependent degradation of ALKBH5 regulates ECM deposition in PM(2.5) exposure-induced pulmonary fibrosis of mice. J. Hazard Mater 432, 128655. doi:10.1016/j.jhazmat.2022.128655
Zhao, J., Han, D. X., Wang, C. B., and Wang, X. L. (2020). Zbtb7b suppresses aseptic inflammation by regulating m(6)A modification of IL6 mRNA. Biochem. Biophys. Res. Commun. 530 (1), 336–341. doi:10.1016/j.bbrc.2020.07.011
Zhao, Q., Zhang, Q., Zhao, X., Tian, Z., Sun, M., and He, L. (2024). MG53: a new protagonist in the precise treatment of cardiomyopathies. Biochem. Pharmacol. 222, 116057. doi:10.1016/j.bcp.2024.116057
Zhao, Y., Hu, J., Sun, X., Yang, K., Yang, L., Kong, L., et al. (2021). Loss of m6A demethylase ALKBH5 promotes post-ischemic angiogenesis via post-transcriptional stabilization of WNT5A. Clin. Transl. Med. 11 (5), e402. doi:10.1002/ctm2.402
Zhao, Y., Sun, J., and Jin, L. (2022). The N6-methyladenosine regulator ALKBH5 mediated stromal cell-macrophage interaction via VEGF signaling to promote recurrent spontaneous abortion: a bioinformatic and in vitro study. Int. J. Mol. Sci. 23 (24), 15819. doi:10.3390/ijms232415819
Zheng, G., Dahl, J. A., Niu, Y., Fedorcsak, P., Huang, C. M., Li, C. J., et al. (2013). ALKBH5 is a mammalian RNA demethylase that impacts RNA metabolism and mouse fertility. Mol. Cell 49 (1), 18–29. doi:10.1016/j.molcel.2012.10.015
Zheng, G., Fu, Y., and He, C. (2014). Nucleic acid oxidation in DNA damage repair and epigenetics. Chem. Rev. 114 (8), 4602–4620. doi:10.1021/cr400432d
Zhou, J., Zhang, X., Hu, J., Qu, R., Yu, Z., Xu, H., et al. (2021). m(6)A demethylase ALKBH5 controls CD4(+) T cell pathogenicity and promotes autoimmunity. Sci. Adv. 7 (25), eabg0470. doi:10.1126/sciadv.abg0470
Zhu, S., and Lu, Y. (2020). Dexmedetomidine suppressed the biological behavior of HK-2 cells treated with LPS by down-regulating ALKBH5. Inflammation 43 (6), 2256–2263. doi:10.1007/s10753-020-01293-y
Keywords: ALKBH5, fibrosis, m6A modification, fibroblasts, RNA modification
Citation: Liao Z, Wang J, Xu M, Li X and Xu H (2024) The role of RNA m6A demethylase ALKBH5 in the mechanisms of fibrosis. Front. Cell Dev. Biol. 12:1447135. doi: 10.3389/fcell.2024.1447135
Received: 25 June 2024; Accepted: 06 August 2024;
Published: 16 August 2024.
Edited by:
Guan-Jun Yang, Ningbo University, ChinaReviewed by:
Liu Ouyang, Georgia State University, United StatesRohit Anthony Sinha, Sanjay Gandhi Post Graduate Institute of Medical Sciences (SGPGI), India
Han Sheng-Na, Zhengzhou University, China
Copyright © 2024 Liao, Wang, Xu, Li and Xu. This is an open-access article distributed under the terms of the Creative Commons Attribution License (CC BY). The use, distribution or reproduction in other forums is permitted, provided the original author(s) and the copyright owner(s) are credited and that the original publication in this journal is cited, in accordance with accepted academic practice. No use, distribution or reproduction is permitted which does not comply with these terms.
*Correspondence: Xiaoyan Li, Y2hoc2hlbnRAMTI2LmNvbQ==; Hongming Xu, eHVob25nbWluZ0AxODguY29t
†These authors have contributed equally to this work and share first authorship