- Department of General Surgery, The Second Xiangya Hospital of Central South University, Changsha, China
Cellular senescence in the intestine can induce cell death, which extends beyond the mere clearance of senescent cells. This phenomenon is prevalent in inflammatory and immune-related diseases, particularly in inflammatory bowel disease (IBD). IBD is characterized by recurrent and chronic intestinal inflammation, with the occurrence and development of the disease being influenced by multiple factors, including genetics, environment, lifestyle, intestinal immunity, and gut microbiota. Chronic intestinal inflammation drives aging of the IBD immune system, reducing its efficiency and impairing the clearance of senescent cells. The disruption of cell death regulation and the interplay between cell death and cellular senescence contribute to disease progression in IBD, with inflammaging and immunosenescence playing the key role in this process. However, the mechanisms underlying the interplay between cell death and cellular senescence in the context of IBD remain unclear. Therefore, this paper comprehensively reviews the impact of cellular death and cellular senescence on intestinal aging in IBD, emphasizing the exploration of their potential interrelationships.
1 Introduction
Inflammatory bowel disease (IBD) is a recurrent and incurable inflammatory disorder of the gastrointestinal tract, primarily comprising Crohn’s disease (CD) and Ulcerative colitis (UC) (Plevris and Lees, 2022). The clinical course of IBD is unpredictable, characterized by recurrent abdominal pain, rectal bleeding, malnutrition, and a risk of malignancy. It is influenced by multiple factors, including genetic predisposition, dietary habits, environmental triggers, psychological factors, immune dysregulation, and gut microbiota imbalance (Bisgaard et al., 2022; Liu et al., 2022).
In recent years, as the standard of living has improved, the incidence of IBD has been rising globally, especially in developing countries, posing a significant threat to global health and healthcare systems (Aniwan et al., 2022; Agrawal et al., 2022). IBD exhibits clinical features similar to intestinal aging, such as intestinal dysfunction, malabsorption, and impaired barrier function. The persistent accumulation of senescent cells and limited clearance together drive the worsening of the inflammatory microenvironment in IBD (Sangfuang et al., 2025). Cell death serves as a key mechanism for clearing senescent cells in the body, with multiple pathways interwoven to maintain the dynamic balance of intestinal cell self-renewal (Ogrodnik et al., 2024). In IBD, cellular senescence and cell death collectively drive the pathological processes of the disease (Chavan et al., 2017). To date, the interactions between cellular senescence and cell death in IBD are not fully elucidated. In this review, we discuss the latest research developments on cellular senescence and cell death, with a particular emphasis on their roles and connections in IBD.
2 Cellular senescence in IBD
2.1 Immunosenescence in IBD
Immunosenescence refers to the gradual decline in immune system function with aging or prolonged inflammatory stimulation, involving extensive changes in both adaptive and innate immunity (Liu Z. et al., 2023). Recent studies have shown that this process is not only observed in the elderly population but is also commonly present in IBD patients, manifesting as “accelerated immunosenescence.” Chronic inflammatory environments induce premature immune cell senescence, impairing immune regulatory functions and exacerbating disease progression (Meng et al., 2023). In IBD, immunosenescence primarily manifests as a decline in T cell function, chronic low-grade inflammation, compromised mucosal immune barriers, and dysbiosis, all of which may affect disease progression and treatment outcomes (Yan et al., 2025; Kosinsky et al., 2024).
Studies have found that in IBD patients, the expression of KLRG1 and CD57 on CD4+ and CD8+ T cells increases, while CD28 expression decreases, indicating T cell exhaustion, with reduced proliferation ability and immune regulatory function (Li H. et al., 2025; Strunk, 2024; Bottois et al., 2020). Additionally, regulatory T cells in CD4+ T cells show impaired function, with reduced IL-10 production, weakening immune tolerance and inflammation suppression, leading to excessive activation of the immune system (Meng et al., 2023; Xie et al., 2022). In IBD patients, T cell telomere shortening and upregulation of senescence-related genes (CDKN1A and CDKN2A) also indicate that immune cells are entering a senescent state, further aggravating chronic inflammation (Hong and Katz, 2021). Immunosenescence affects the antigen presentation capacity of mucosal dendritic cells, leading to decreased immune tolerance to the microbiota in IBD patients, as evidenced by reduced probiotics and increased pathogenic bacteria, further accelerating immunosenescence (Meng et al., 2023; Wahida et al., 2021). In our previous research, single-cell RNA sequencing data from IBD patients showed an increase in the proportion of senescent CD8+T cells (KLRG1+CD28−) and a decrease in memory T cells (CD27+ CD28+), suggesting T cell exhaustion in IBD patients (Yao et al., 2024). Meanwhile, macrophages exhibited an inflammatory phenotype (M1 type), with high expression of IL-6 and TNF-α, indicating that chronic inflammation promotes the occurrence of immunosenescence in IBD (Yao et al., 2024).
Immunosenescence is also associated with reduced response to anti-TNF-α biologics (such as infliximab) in IBD patients, with mechanisms involving B cell dysfunction, T cell exhaustion, and the effects of the chronic inflammatory environment (Faye et al., 2024). In elderly IBD patients, the proportion of senescent B cells (CD21−CD11c+) increases, while memory B cells (CD27+IgD−) decrease, potentially leading to abnormal anti-infliximab antibody production and affecting drug efficacy (Meng et al., 2023; Childs et al., 2023). Additionally, T cell senescence (particularly the dysfunction of follicular helper T cells) may weaken the regulatory effect on B cells, further contributing to drug resistance (Di Micco et al., 2021; Varricchi et al., 2020). Meanwhile, the chronic inflammatory environment (with high expression of IL-6 and TNF-α) may accelerate the metabolism and clearance of infliximab, reducing its therapeutic effect (Ben-Horin et al., 2014). Therefore, immunosenescence not only impacts the disease progression of IBD but may also impair the long-term efficacy of biologics, highlighting the need for more precise personalized treatment strategies in the future.
2.2 Inflammation-induced senescence in IBD
IBD is characterized by chronic, recurrent intestinal inflammation, with its inflammatory microenvironment filled with pro-inflammatory cytokines (IL-6, TNF-α, IL-1β) and accompanied by extensive immune cell infiltration (Onyiah and Colgan, 2016). These inflammatory factors not only cause damage to intestinal epithelial cells but also promote cellular senescence through the activation of inflammatory pathways such as p38-MAPK, JAK-STAT, and NF-κB (Sienkiewicz et al., 2023). Studies have shown that in the IBD intestine, IL-6 can upregulate the expression of CDKN1A and CDKN2A through the JAK-STAT axis, leading to cell cycle arrest and inducing senescence (Yao et al., 2014). Additionally, TNF-α can directly trigger DNA damage response (DDR), accelerate telomere shortening, and further promote cellular senescence (Maekawa et al., 2018; Zhang et al., 2016).
The senescence-associated secretory phenotype (SASP) is an important link between cellular senescence and chronic inflammation. In IBD, senescent cells release large amounts of pro-inflammatory factors through SASP, including IL-6, IL-8, CCL2 (monocyte chemoattractant protein), and MMPs, forming a vicious cycle of inflammation-senescence (Almasabi et al., 2021). The inflammatory effect induced by SASP is mainly reflected in the fact that inflammatory factors, including IL-6 and IL-8, promote further immune cell infiltration, amplify the inflammatory response, and accelerate IBD-associated senescence (Bassotti et al., 2023). MMPs (matrix metalloproteinases) degrade the extracellular matrix (ECM), weakening the intestinal barrier, increasing intestinal permeability, and making IBD more prone to relapse (Lee and Kim, 2022). SASP promotes macrophages to maintain the M1 pro-inflammatory phenotype while suppressing the anti-inflammatory function of M2 macrophages, preventing the resolution of inflammation (Wang et al., 2024).
Intestinal stem cells (ISCs) play a key role in maintaining intestinal epithelial homeostasis. However, in the IBD inflammatory environment, ISCs are continuously stimulated by pro-inflammatory factors, which may lead to their senescence (Wang X. et al., 2020). Research has shown that Lgr5+ stem cells in IBD patients exhibit limited proliferation in the chronic inflammatory environment and show a senescent phenotype (Birch and Gil, 2020; Gorgoulis et al., 2019). Moreover, inflammation-induced mitochondrial dysfunction and abnormalities in the Wnt signaling pathway further affect the regenerative capacity of stem cells (Yun et al., 2023; Watanabe et al., 2022; Risques et al., 2008). SASP-released IL-6 and IL-1β can activate the JAK-STAT signaling pathway, upregulating the expression of CDKN1A and CDKN2A, and accelerating stem cell senescence (Wang X. et al., 2020; Yun et al., 2023).
Furthermore, IBD recurrence is closely related to the accumulation of senescent cells. When IBD transitions from remission to active phase, senescent cells can release pro-inflammatory factors through SASP, further enhancing the inflammatory microenvironment, making intestinal inflammation more likely to relapse (Birch and Gil, 2020; Gorgoulis et al., 2019).
3 Cell death in IBD
The human intestinal tract serves the vital functions of digesting food, absorbing nutrients and water, while also maintaining the balance of the gut microbiota. This places a considerable demand on intestinal epithelial cells, with approximately 1010 intestinal epithelial cells shedding and undergoing passive cell death every day (Blander, 2016). The passive shedding of intestinal epithelial cells primarily occurs at the tips of villi, with apoptosis being the primary mechanism of shedding (Iwanaga and Takahashi-Iwanaga, 2022). Recently, researchers have found that shed IECs can survive for several hours in mice, stimulating the expression of antimicrobial genes at the tips of villi and contributing to the regulation of gut microbiota composition (Bahar Halpern et al., 2023). This suggests that cell apoptosis can occur after IEC shedding. Moreover, compared to the small intestine, shed cells survive longer in the colon (Bahar Halpern et al., 2023),indicating potential differences in clearance mechanisms between the small intestine and colon, possibly beyond apoptotic clearance alone. The shedding and renewal of IECs are not only related to the proliferation of stem cells in the crypts but also to the clearance rate of senescent epithelial monolayer cells (Blander, 2016). In IBD, continuous shedding of IECs is observed, along with ongoing shedding of various immune cells, particularly more pronounced during active disease phases. While apoptosis is the normal physiological mechanism for cellular renewal in the intestine, other forms of cell death also play important roles in the development of IBD. In IBD, chronic inflammation and recurrent episodes lead to excessive cell death within the intestinal tract. This excessive cell death can activate the intestinal immune system, exacerbating inflammation in the IBD intestine (Günther et al., 2013; Dagenais et al., 2014). Multiple cell death mechanisms interact in the intestinal tract of IBD, cooperatively promoting disease progression.
In addition to apoptosis, several other regulated cell death pathways mentioned earlier are involved in the development of IBD. Different modes of cell death can affect the repair and regeneration of intestinal mucosal tissue, ultimately impacting intestinal fibrosis and even increasing the long-term risk of intestinal malignancies (Patankar and Becker, 2020). Excessive apoptosis has been shown to worsen intestinal inflammation in mice and IBD patients (Zhang J. et al., 2022), while anti-apoptotic strategies can help maintain colonic epithelial cell homeostasis and reinforce epithelial barrier function (Zhang et al., 2022b). Cell pyroptosis in the IBD intestine originates from the activation of inflammasomes (such as NLRP3) (Chen et al., 2019), which are integral to innate immune responses and play crucial roles in maintaining gut microbiota and gut-brain homeostasis (Man, 2018). Cell pyroptosis mediates various damage signals, leading to persistent chronic inflammation in IBD, primarily executed by proteins like GSDMB, GADMD, and GSDME (Zhang S. et al., 2022). Furthermore, GSDMB, an executor of pyroptosis, is also a critical factor in maintaining epithelial barrier function and inflammation resolution in IBD (Rana et al., 2022).
As detection methods continue to evolve, it has been discovered that cell death induced by trace metal elements also contributes to the development of intestinal inflammation in IBD. Ferroptosis, mediated mainly by endoplasmic reticulum stress and the NF-κB pathway, can be observed in intestinal epithelial cells of IBD patients and DSS mice (Ocansey et al., 2023). There is an accumulation of ROS, increased ferrous iron levels, and excessive lipid peroxidation in the intestinal epithelium in IBD, collectively driving chronic abnormal inflammation (Ocansey et al., 2023; Chen et al., 2020). Inhibitors of ferroptosis have been shown to effectively control chronic inflammation in the intestine, a fact widely validated in IBD patients and animal models (Arab et al., 2021; Xu et al., 2021; Huang et al., 2022; Zhu et al., 2022). Similarly, cuproptosis has also been observed in the intestine of IBD patients and DSS mice (Chen et al., 2022; Liu L. et al., 2023). Cuproptosis in the IBD intestine primarily affects the composition of immune cell infiltration and indirectly influences intestinal inflammation by controlling mitochondrial metabolism (Liu L. et al., 2023; Yang et al., 2023). While SLC7A11-driven disulfidptosis has not been extensively studied in IBD, the upregulation of the key gene SLC7A11 in the intestinal mucosa of IBD is clear (Al-Mustanjid et al., 2020). Interestingly, SLC7A11 is an inhibitor of ferroptosis (Koppula et al., 2021), and ferroptosis promotes the occurrence and development of IBD intestinal inflammation (Wang et al., 2023). Therefore, it remains to be explored whether the high expression of SLC7A11 in the intestinal mucosa of IBD acts as a driver of disulfidptosis or a protector against ferroptosis. This warrants further in-depth research. To this end, our research team analyzed multiple UC datasets to identify differentially expressed genes associated with disulfidptosis and utilized lasso regression to determine key target genes. Immune infiltration analysis and the construction of clinical diagnostic models showed that five disulfidptosis-related immune-associated genes (PDLIM1, SLC7A11, MYH10, NUBPL, OXSM) are highly correlated with immune cells and inflammatory pathways in UC (Yang et al., 2024).
Lysoptosis-induced lysosomal membrane dysfunction (lysosome-dependent cell death) mediates cell death and further promotes the release of pro-inflammatory mediators, increasing the risk of colitis (Lassen et al., 2016; Wang et al., 2018). In IBD, ICD is a stress-driven form of RCD that can induce adaptive immune responses through antigen presentation and the release of danger signals (Kroemer et al., 2022). In the early stages of the disease, ICD-induced immune responses may play a regulatory role in tissue repair, helping to clear damaged cells and promote intestinal epithelial regeneration (Clucas and Meier, 2023). However, as the natural course of IBD progresses, the immune activation potential of ICD may have adverse effects in the context of chronic inflammation, such as exacerbating tissue damage or promoting carcinogenesis (Hayashi et al., 2021; Ruan et al., 2020).
Necroptosis in IBD mainly occurs in intestinal epithelial cells, and inhibiting RIPK3 can alleviate chronic intestinal inflammation induced by Necroptosis to some extent (Günther et al., 2011; Welz et al., 2011). Necroptosis can also occur in intestinal stem cells in IBD, and the deletion of the key gene SETDB1 can induce Necroptosis, affecting colonic epithelial differentiation, disrupting mucosal barrier, and promoting intestinal inflammation (Wang R. et al., 2020; Južnić et al., 2021). Unlike regulated cell death, necrosis is not commonly observed in IBD and is less involved in the regulation of chronic enteritis. It generally occurs in acute mucosal injury or extreme external stimuli (Zhou et al., 2022).
4 Correlation between cellular senescence and cell death in IBD
4.1 Cellular senescence to cell death
In the intestinal tissues of IBD patients, intestinal epithelial cells, fibroblasts, immune cells, and others often exhibit clear signs of senescence (Wei et al., 2023; Giunta et al., 2022). Senescent cells typically face three possible fates: clearance by immune cells; maintenance in a quiescent state; or entry into regulated programmed cell death (such as pyroptosis, necrosis, autophagy, or apoptosis) (Fantini et al., 2024). However, under IBD conditions, due to impaired immune clearance, a large number of senescent cells fail to be removed in time, persist for extended periods, and exacerbate the negative effects of SASP. Persistent inflammatory stimuli can induce the activation of various regulated cell death (RCD) pathways, leading to further damage to the intestinal barrier (Tower, 2015). Specifically, senescent cells release a large number of pro-inflammatory factors through SASP and activate the NF-κB and p38 MAPK pathways, enhancing inflammatory signaling, making adjacent cells more susceptible to damage and even entering Necroptosis (Zhang et al., 2022d; Bertheloot et al., 2021). The accumulation of senescent cells may amplify the TNF-α-dependent RIPK3 overexpression, thereby exacerbating intestinal epithelial programmed necrosis (Necroptosis), and the release of damage-associated molecular patterns (DAMPs) from necrotic cells will further activate NF-κB signaling, intensifying the inflammatory response and the vicious cycle of cellular senescence (Hou et al., 2024; Royce et al., 2019). Additionally, senescence leads to increased intracellular oxidative stress, such as the activation of the NOX (NADPH oxidase) family, resulting in the accumulation of reactive oxygen species (ROS) that further promote NLRP3-Caspase-1 axis-mediated pyroptosis (Zarrin et al., 2021; Yuan and Ofengeim, 2024; Hsu et al., 2021). Cytokines secreted by SASP also inhibit AMPK activity, reducing ULK1-dependent autophagy initiation, thereby impairing the autophagic clearance function of intestinal cells (Hsu et al., 2021; Cheng et al., 2024).
The accumulation of senescent cells in the intestine leads to aging-related changes, while persistent intestinal aging can reduce the expression of apoptosis-related genes in mesenchymal stem cells in IBD, affecting mucosal repair and regeneration (Alt et al., 2012). Consistent with reduced apoptosis in the state of intestinal aging, biomarkers of apoptosis in normal human serum decrease with age (Kavathia et al., 2009). Apoptosis is associated with the healing of mucosal ulcers in the intestinal tract (Wang et al., 2025). The downregulation of apoptosis gene expression in intestinal epithelial cells due to a decreased clearance rate of senescent cells may be a key factor contributing to the prolonged non-healing of intestinal ulcers in IBD (Li W. et al., 2025). In the animal models (such as the DSS-induced rat colitis model), the expression of SASP-related molecules (such as IL-1β, MMPs) is significantly elevated, accompanied by a reduction in mitochondrial membrane potential and an increased apoptosis rate in intestinal epithelial cells, further demonstrating the role of SASP in inducing cell death (Moiseeva et al., 2023). The main crosstalk mechanisms between cell death and cellular senescence in IBD are summarized in Figure 1.
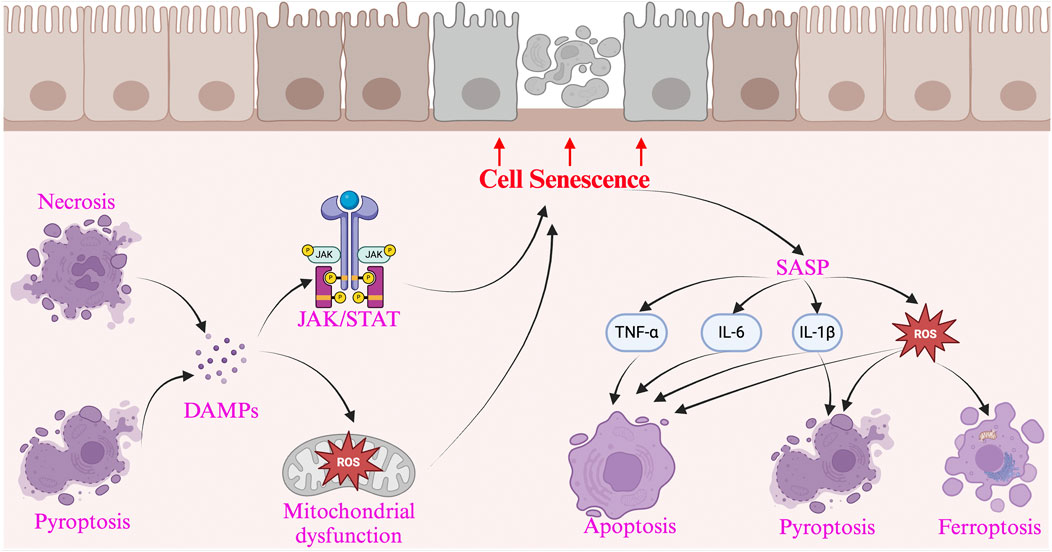
Figure 1. The interaction between cellular senescence and cell death in Inflammatory Bowel Disease and presents the key pathways and mechanisms involved. Cellular senescence promotes cell death through the SASP, which releases pro-apoptotic factors (such as TNF-α, IL-6, and IL-1β), triggering the Fas/FasL axis and Caspase cascade, leading to apoptosis. Additionally, SASP induces oxidative stress (ROS accumulation), activating the p53/p21 axis, accelerating DNA damage, and promoting cell death. It also activates the NLRP3 inflammasome via IL-1β and ATP, triggering pyroptosis, causing cell rupture and the release of DAMPs. On the other hand, cell death exacerbates cellular senescence by releasing DAMPs (such as HMGB1 and ATP), which continuously activate NF-κB, promoting chronic inflammation and inducing more cells into a senescent state. The inflammatory microenvironment activates the JAK/STAT axis, while excessive ROS accumulation leads to mitochondrial damage, accelerating DNA breaks, and further promoting the accumulation of senescent cells.
In summary, senescent cells in the IBD intestine usually accumulate due to persistent inflammatory stimuli, oxidative stress, telomere shortening, and DNA damage, exhibiting resistance to apoptosis. It is important to note that senescent cells that inhibit apoptosis may promote malignant transformation, increasing the risk of IBD patients developing colorectal cancer (Schmitt et al., 2022; Lv et al., 2024).
4.2 Cell death to cellular senescence
In the progression of IBD, cell death is not merely an endpoint but may serve as a trigger for cellular senescence. Various regulated cell death modes, such as pyroptosis, apoptosis, necroptosis, and autophagy, promote the entry of intestinal epithelial cells into a senescent state through different mechanisms (Tower, 2015).
In the early stages of IBD, intestinal epithelial cells undergo excessive apoptosis in response to external stimuli, which may deplete the intestinal stem cell pool and force the stem cells into a senescent state (Kavathia et al., 2009). In the later stages of IBD, prolonged chronic inflammation may suppress apoptosis in intestinal epithelial cells, leading to a decreased clearance rate of senescent cells (Alt et al., 2012). Some cells that fail to undergo apoptosis may become arrested in the G1 phase, displaying typical features of senescence. In IBD, the highly activated pyroptosis in intestinal epithelial cells induces the release of IL-1β, IL-18, and DAMPs, which continuously activate the NF-κB pathway, creating a chronic inflammatory environment that promotes the senescence of neighboring cells (Chen et al., 2019). Senescent cells after pyroptosis also continue to secrete SASP, leading to mitochondrial dysfunction and exacerbating IBD-related cellular senescence. Furthermore, mutations in ATG16L1 and NOD2 in IBD reduce autophagic activity, making it difficult for senescent cells in the intestine to be cleared. Necroptotic cells also induce immune cell activation of the NF-κB pathway, promoting the accumulation of senescent cells (Wang R. et al., 2020). Cell death induced by metal elements is closely related to mitochondria (Tsvetkov et al., 2022; Jiang et al., 2021), as mitochondrial dysfunction mediates insufficient cellular energy supply, closely linking it to cellular senescence (Fantini et al., 2024). In conclusion, understanding the impact of different cell death modes on cellular senescence not only helps to uncover the pathological mechanisms of IBD but may also provide new intervention strategies for future IBD treatments. The impact of various cell death mechanisms on cellular senescence and their potential mechanisms in IBD are summarized in Table 1.
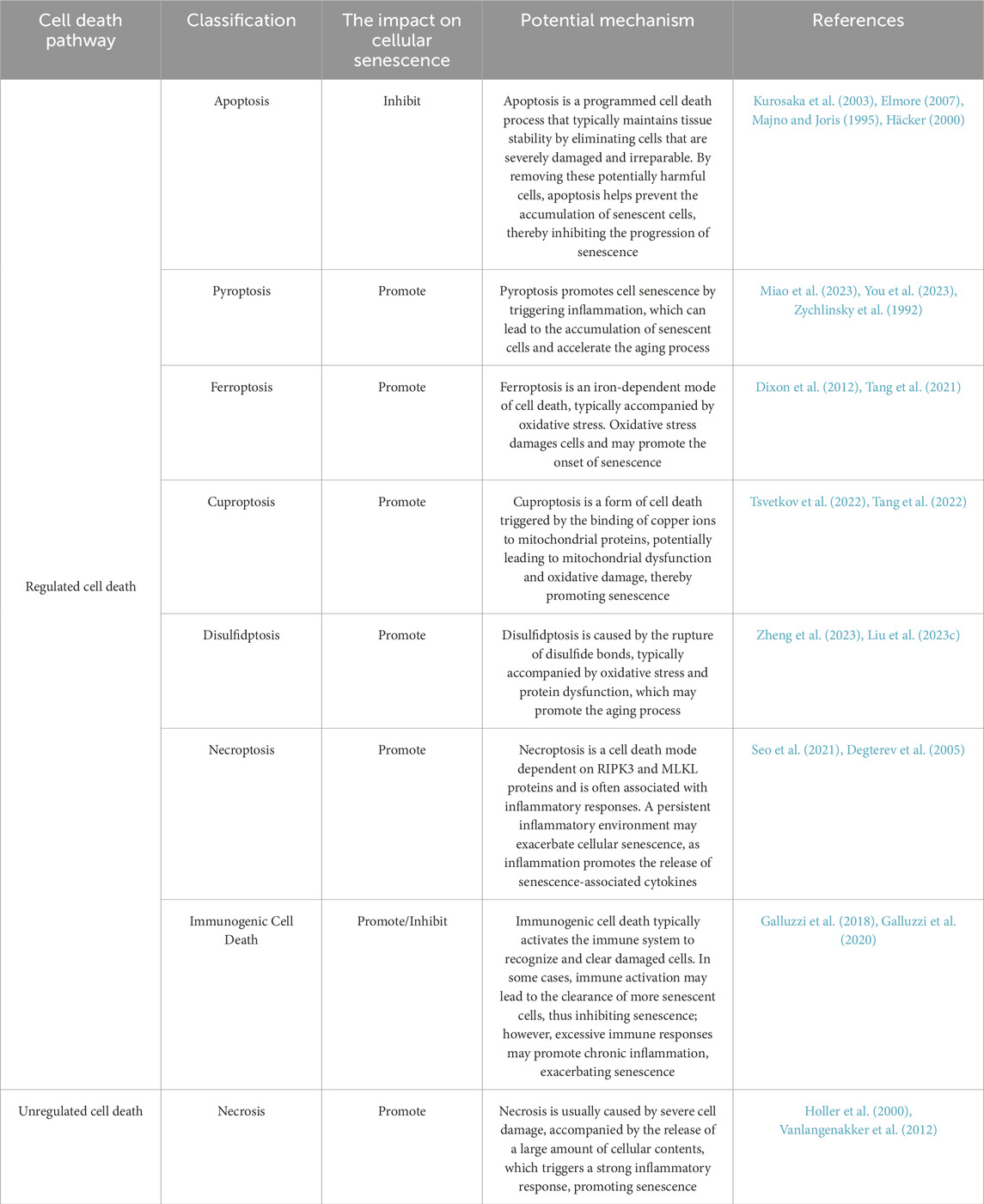
Table 1. The impact of various cell death mechanisms on cellular senescence and their potential mechanisms in IBD.
5 Conclusion and prospects
In IBD, chronic intestinal inflammation creates a pathological microenvironment that accelerates immune system aging and impairs its ability to clear senescent cells efficiently. The accumulation of senescent cells further disrupts tissue regeneration, leading to a complex interplay between cell death and cellular renewal. SASP and senescent cells modulate cell death pathways, but when the balance is lost due to chronic inflammation, cell death dysregulation can hinder senescent cell clearance, exacerbating disease progression.
Given the increasing focus on anti-aging therapies, targeting intestinal aging in IBD may provide a novel therapeutic strategy. However, research on drugs specifically targeting intestinal aging in IBD remains limited. Further basic and clinical studies are needed to explore whether preventing early intestinal aging in young IBD patients can mitigate symptoms and promote long-term remission. Although cell death regulation has been widely studied in IBD and some therapeutic interventions show promise, the complexity of IBD pathogenesis suggests that focusing solely on cell death mechanisms may not fully explain disease progression or treatment efficacy. Understanding the intrinsic link between cell death and cellular senescence is crucial for identifying new therapeutic targets, offering potential clinical and societal benefits in managing IBD.
Author contributions
LCY: Data curation, Software, Visualization, Writing – original draft, Writing – review and editing. LWY: Software, Supervision, Writing – original draft, Writing – review and editing.
Funding
The author(s) declare that financial support was received for the research and/or publication of this article. This work was supported by the National Natural Science Foundation of China (82270590) and the Fundamental Research Funds for the Central Universities of Central South University (2025ZZTS0145).
Conflict of interest
The authors declare that the research was conducted in the absence of any commercial or financial relationships that could be construed as a potential conflict of interest.
Generative AI statement
The author(s) declare that no Generative AI was used in the creation of this manuscript.
Publisher’s note
All claims expressed in this article are solely those of the authors and do not necessarily represent those of their affiliated organizations, or those of the publisher, the editors and the reviewers. Any product that may be evaluated in this article, or claim that may be made by its manufacturer, is not guaranteed or endorsed by the publisher.
References
Agrawal, M., Allin, K. H., Petralia, F., Colombel, J.-F., and Jess, T. (2022). Multiomics to elucidate inflammatory bowel disease risk factors and pathways. Nat. Rev. Gastroenterol. Hepatol. 19, 399–409. doi:10.1038/s41575-022-00593-y
Almasabi, S., Ahmed, A. U., Boyd, R., and Williams, B. R. G. (2021). A potential role for integrin-linked kinase in colorectal cancer growth and progression via regulating senescence and immunity. Front. Genet. 12, 638558. doi:10.3389/fgene.2021.638558
Al-Mustanjid, M., Mahmud, S. M. H., Royel, M. R. I., Rahman, M. H., Islam, T., Rahman, M. R., et al. (2020). Detection of molecular signatures and pathways shared in inflammatory bowel disease and colorectal cancer: a bioinformatics and systems biology approach. Genomics 112, 3416–3426. doi:10.1016/j.ygeno.2020.06.001
Alt, E. U., Senst, C., Murthy, S. N., Slakey, D. P., Dupin, C. L., Chaffin, A. E., et al. (2012). Aging alters tissue resident mesenchymal stem cell properties. Stem Cell Res. 8, 215–225. doi:10.1016/j.scr.2011.11.002
Aniwan, S., Santiago, P., Loftus, E. V., and Park, S. H. (2022). The epidemiology of inflammatory bowel disease in Asia and Asian immigrants to Western countries. United Eur. Gastroenterol. J. 10, 1063–1076. doi:10.1002/ueg2.12350
Arab, H. H., Eid, A. H., Mahmoud, A. M., and Senousy, M. A. (2021). Linagliptin mitigates experimental inflammatory bowel disease in rats by targeting inflammatory and redox signaling. Life Sci. 273, 119295. doi:10.1016/j.lfs.2021.119295
Bahar Halpern, K., Korem Kohanim, Y., Biram, A., Harnik, Y., Egozi, A., Yakubovsky, O., et al. (2023). The cellular states and fates of shed intestinal cells. Nat. Metab. 5, 1858–1869. doi:10.1038/s42255-023-00905-9
Bassotti, G., Fruganti, A., Stracci, F., Marconi, P., and Fettucciari, K. (2023). Cytotoxic synergism of Clostridioides difficile toxin B with proinflammatory cytokines in subjects with inflammatory bowel diseases. World J. Gastroenterol. 29, 582–596. doi:10.3748/wjg.v29.i4.582
Ben-Horin, S., Kopylov, U., and Chowers, Y. (2014). Optimizing anti-TNF treatments in inflammatory bowel disease. Autoimmun. Rev. 13, 24–30. doi:10.1016/j.autrev.2013.06.002
Bertheloot, D., Latz, E., and Franklin, B. S. (2021). Necroptosis, pyroptosis and apoptosis: an intricate game of cell death. Cell Mol. Immunol. 18, 1106–1121. doi:10.1038/s41423-020-00630-3
Birch, J., and Gil, J. (2020). Senescence and the SASP: many therapeutic avenues. Genes Dev. 34, 1565–1576. doi:10.1101/gad.343129.120
Bisgaard, T. H., Allin, K. H., Keefer, L., Ananthakrishnan, A. N., and Jess, T. (2022). Depression and anxiety in inflammatory bowel disease: epidemiology, mechanisms and treatment. Nat. Rev. Gastroenterol. Hepatol. 19, 717–726. doi:10.1038/s41575-022-00634-6
Blander, J. M. (2016). Death in the intestinal epithelium-basic biology and implications for inflammatory bowel disease. FEBS J. 283, 2720–2730. doi:10.1111/febs.13771
Bottois, H., Ngollo, M., Hammoudi, N., Courau, T., Bonnereau, J., Chardiny, V., et al. (2020). KLRG1 and CD103 expressions define distinct intestinal tissue-resident memory CD8 T cell subsets modulated in crohn’s disease. Front. Immunol. 11, 896. doi:10.3389/fimmu.2020.00896
Chavan, S. S., Pavlov, V. A., and Tracey, K. J. (2017). Mechanisms and therapeutic relevance of neuro-immune communication. Immunity 46, 927–942. doi:10.1016/j.immuni.2017.06.008
Chen, X., Liu, G., Yuan, Y., Wu, G., Wang, S., and Yuan, L. (2019). NEK7 interacts with NLRP3 to modulate the pyroptosis in inflammatory bowel disease via NF-κB signaling. Cell Death Dis. 10, 906. doi:10.1038/s41419-019-2157-1
Chen, Y., Li, X., Sun, R., Ji, J., Yang, F., Tian, W., et al. (2022). A broad cuproptosis landscape in inflammatory bowel disease. Front. Immunol. 13, 1031539. doi:10.3389/fimmu.2022.1031539
Chen, Y., Zhang, P., Chen, W., and Chen, G. (2020). Ferroptosis mediated DSS-induced ulcerative colitis associated with Nrf2/HO-1 signaling pathway. Immunol. Lett. 225, 9–15. doi:10.1016/j.imlet.2020.06.005
Cheng, C.-K., Ye, L., Zuo, Y., Wang, Y., Wang, L., Li, F., et al. (2024). Aged gut microbiome induces metabolic impairment and hallmarks of vascular and intestinal aging in young mice. Antioxidants (Basel) 13, 1250. doi:10.3390/antiox13101250
Childs, B. G., Graves, S. I., and Baker, D. J. (2023). B cell senescence takes guts. Nat. Cell Biol. 25, 800–801. doi:10.1038/s41556-023-01153-5
Clucas, J., and Meier, P. (2023). Roles of RIPK1 as a stress sentinel coordinating cell survival and immunogenic cell death. Nat. Rev. Mol. Cell Biol. 24, 835–852. doi:10.1038/s41580-023-00623-w
Dagenais, M., Douglas, T., and Saleh, M. (2014). Role of programmed necrosis and cell death in intestinal inflammation. Curr. Opin. Gastroenterol. 30, 566–575. doi:10.1097/MOG.0000000000000117
Degterev, A., Huang, Z., Boyce, M., Li, Y., Jagtap, P., Mizushima, N., et al. (2005). Chemical inhibitor of nonapoptotic cell death with therapeutic potential for ischemic brain injury. Nat. Chem. Biol. 1, 112–119. doi:10.1038/nchembio711
Di Micco, R., Krizhanovsky, V., Baker, D., and d’Adda di Fagagna, F. (2021). Cellular senescence in ageing: from mechanisms to therapeutic opportunities. Nat. Rev. Mol. Cell Biol. 22, 75–95. doi:10.1038/s41580-020-00314-w
Dixon, S. J., Lemberg, K. M., Lamprecht, M. R., Skouta, R., Zaitsev, E. M., Gleason, C. E., et al. (2012). Ferroptosis: an iron-dependent form of nonapoptotic cell death. Cell 149, 1060–1072. doi:10.1016/j.cell.2012.03.042
Elmore, S. (2007). Apoptosis: a review of programmed cell death. Toxicol. Pathol. 35, 495–516. doi:10.1080/01926230701320337
Fantini, M. C., Onali, S., Gasbarrini, A., and Lopetuso, L. R. (2024). Immune system and gut microbiota senescence in elderly IBD patients. Minerva Gastroenterol Torino 70 (1), 59–67. doi:10.23736/S2724-5985.21.02934-X
Faye, A. S., Lee, K. E., Hudesman, D., and Dervieux, T. (2024). Older adults with inflammatory bowel disease are at higher risk of developing antibodies to infliximab. Inflamm. Bowel Dis. 30, 2509–2511. doi:10.1093/ibd/izad305
Galluzzi, L., Vitale, I., Aaronson, S. A., Abrams, J. M., Adam, D., Agostinis, P., et al. (2018). Molecular mechanisms of cell death: recommendations of the nomenclature committee on cell death 2018. Cell Death Differ. 25, 486–541. doi:10.1038/s41418-017-0012-4
Galluzzi, L., Vitale, I., Warren, S., Adjemian, S., Agostinis, P., Martinez, A. B., et al. (2020). Consensus guidelines for the definition, detection and interpretation of immunogenic cell death. J. Immunother. Cancer 8, e000337. doi:10.1136/jitc-2019-000337
Giunta, S., Wei, Y., Xu, K., and Xia, S. (2022). Cold-inflammaging: when a state of homeostatic-imbalance associated with aging precedes the low-grade pro-inflammatory-state (inflammaging): meaning, evolution, inflammaging phenotypes. Clin. Exp. Pharmacol. Physiol. 49, 925–934. doi:10.1111/1440-1681.13686
Gorgoulis, V., Adams, P. D., Alimonti, A., Bennett, D. C., Bischof, O., Bishop, C., et al. (2019). Cellular senescence: defining a path forward. Cell 179, 813–827. doi:10.1016/j.cell.2019.10.005
Günther, C., Martini, E., Wittkopf, N., Amann, K., Weigmann, B., Neumann, H., et al. (2011). Caspase-8 regulates TNF-α-induced epithelial necroptosis and terminal ileitis. Nature 477, 335–339. doi:10.1038/nature10400
Günther, C., Neumann, H., Neurath, M. F., and Becker, C. (2013). Apoptosis, necrosis and necroptosis: cell death regulation in the intestinal epithelium. Gut 62, 1062–1071. doi:10.1136/gutjnl-2011-301364
Häcker, G. (2000). The morphology of apoptosis. Cell Tissue Res. 301, 5–17. doi:10.1007/s004410000193
Hayashi, K., Nikolos, F., and Chan, K. S. (2021). Inhibitory DAMPs in immunogenic cell death and its clinical implications. Cell Stress 5, 52–54. doi:10.15698/cst2021.04.247
Holler, N., Zaru, R., Micheau, O., Thome, M., Attinger, A., Valitutti, S., et al. (2000). Fas triggers an alternative, caspase-8-independent cell death pathway using the kinase RIP as effector molecule. Nat. Immunol. 1, 489–495. doi:10.1038/82732
Hong, S. J., and Katz, S. (2021). The elderly IBD patient in the modern era: changing paradigms in risk stratification and therapeutic management. Ther. Adv. Gastroenterol. 14, 17562848211023399. doi:10.1177/17562848211023399
Hou, S., Zhang, J., Jiang, X., Yang, Y., Shan, B., Zhang, M., et al. (2024). PARP5A and RNF146 phase separation restrains RIPK1-dependent necroptosis. Mol. Cell 84, 938–954.e8. doi:10.1016/j.molcel.2023.12.041
Hsu, S.-K., Li, C.-Y., Lin, I.-L., Syue, W.-J., Chen, Y.-F., Cheng, K.-C., et al. (2021). Inflammation-related pyroptosis, a novel programmed cell death pathway, and its crosstalk with immune therapy in cancer treatment. Theranostics 11, 8813–8835. doi:10.7150/thno.62521
Huang, F., Zhang, S., Li, X., Huang, Y., He, S., and Luo, L. (2022). STAT3-mediated ferroptosis is involved in ulcerative colitis. Free Radic. Biol. Med. 188, 375–385. doi:10.1016/j.freeradbiomed.2022.06.242
Iwanaga, T., and Takahashi-Iwanaga, H. (2022). Disposal of intestinal apoptotic epithelial cells and their fate via divergent routes. Biomed. Res. 43, 59–72. doi:10.2220/biomedres.43.59
Jiang, X., Stockwell, B. R., and Conrad, M. (2021). Ferroptosis: mechanisms, biology and role in disease. Nat. Rev. Mol. Cell Biol. 22, 266–282. doi:10.1038/s41580-020-00324-8
Južnić, L., Peuker, K., Strigli, A., Brosch, M., Herrmann, A., Häsler, R., et al. (2021). SETDB1 is required for intestinal epithelial differentiation and the prevention of intestinal inflammation. Gut 70, 485–498. doi:10.1136/gutjnl-2020-321339
Kavathia, N., Jain, A., Walston, J., Beamer, B. A., and Fedarko, N. S. (2009). Serum markers of apoptosis decrease with age and cancer stage. Aging (Albany NY) 1, 652–663. doi:10.18632/aging.100069
Koppula, P., Zhuang, L., and Gan, B. (2021). Cystine transporter SLC7A11/xCT in cancer: ferroptosis, nutrient dependency, and cancer therapy. Protein Cell 12, 599–620. doi:10.1007/s13238-020-00789-5
Kosinsky, R. L., Gonzalez, M. M., Saul, D., Barros, L. L., Sagstetter, M. R., Fedyshyn, Y., et al. (2024). The FOXP3+ pro-inflammatory T cell: a potential therapeutic target in crohn’s disease. Gastroenterology 166, 631–644.e17. doi:10.1053/j.gastro.2024.01.007
Kroemer, G., Galassi, C., Zitvogel, L., and Galluzzi, L. (2022). Immunogenic cell stress and death. Nat. Immunol. 23, 487–500. doi:10.1038/s41590-022-01132-2
Kurosaka, K., Takahashi, M., Watanabe, N., and Kobayashi, Y. (2003). Silent cleanup of very early apoptotic cells by macrophages. J. Immunol. 171, 4672–4679. doi:10.4049/jimmunol.171.9.4672
Lassen, K. G., McKenzie, C. I., Mari, M., Murano, T., Begun, J., Baxt, L. A., et al. (2016). Genetic coding variant in GPR65 alters lysosomal pH and links lysosomal dysfunction with colitis risk. Immunity 44, 1392–1405. doi:10.1016/j.immuni.2016.05.007
Lee, H. S., and Kim, W. J. (2022). The role of matrix metalloproteinase in inflammation with a focus on infectious diseases. Int. J. Mol. Sci. 23, 10546. doi:10.3390/ijms231810546
Li, H., Cai, X., Xu, C., Yang, X., Song, X., Kong, Y., et al. (2025a). RNA cytidine acetyltransferase NAT10 maintains T cell pathogenicity in inflammatory bowel disease. Cell Discov. 11, 19. doi:10.1038/s41421-025-00781-5
Li, W., Zeng, Y., Zhong, J., Hu, Y., Xiong, X., Zhou, Y., et al. (2025b). Probiotics exert gut immunomodulatory effects by regulating the expression of host miRNAs. Probiotics Antimicrob. Proteins 17, 557–568. doi:10.1007/s12602-024-10443-9
Liu, D., Saikam, V., Skrada, K. A., Merlin, D., and Iyer, S. S. (2022). Inflammatory bowel disease biomarkers. Med. Res. Rev. 42, 1856–1887. doi:10.1002/med.21893
Liu, L., Liang, L., Yang, C., and Chen, Y. (2023b). Machine learning-based solution reveals cuproptosis features in inflammatory bowel disease. Front. Immunol. 14, 1136991. doi:10.3389/fimmu.2023.1136991
Liu, X., Nie, L., Zhang, Y., Yan, Y., Wang, C., Colic, M., et al. (2023c). Actin cytoskeleton vulnerability to disulfide stress mediates disulfidptosis. Nat. Cell Biol. 25, 404–414. doi:10.1038/s41556-023-01091-2
Liu, Z., Liang, Q., Ren, Y., Guo, C., Ge, X., Wang, L., et al. (2023a). Immunosenescence: molecular mechanisms and diseases. Signal Transduct. Target Ther. 8, 200. doi:10.1038/s41392-023-01451-2
Lv, H., Mu, Y., Zhang, C., Zhao, M., Jiang, P., Xiao, S., et al. (2024). Comparative analysis of single-cell transcriptome reveals heterogeneity and commonality in the immune microenvironment of colorectal cancer and inflammatory bowel disease. Front. Immunol. 15, 1356075. doi:10.3389/fimmu.2024.1356075
Maekawa, T., Liu, B., Nakai, D., Yoshida, K., Nakamura, K.-I., Yasukawa, M., et al. (2018). ATF7 mediates TNF-α-induced telomere shortening. Nucleic Acids Res. 46, 4487–4504. doi:10.1093/nar/gky155
Majno, G., and Joris, I. (1995). Apoptosis, oncosis, and necrosis. An overview of cell death. Am. J. Pathol. 146, 3–15.
Man, S. M. (2018). Inflammasomes in the gastrointestinal tract: infection, cancer and gut microbiota homeostasis. Nat. Rev. Gastroenterol. Hepatol. 15, 721–737. doi:10.1038/s41575-018-0054-1
Meng, G., Monaghan, T. M., Duggal, N. A., Tighe, P., and Peerani, F. (2023). Microbial-immune crosstalk in elderly-onset inflammatory bowel disease: unchartered territory. J. Crohns Colitis 17, 1309–1325. doi:10.1093/ecco-jcc/jjad025
Miao, R., Jiang, C., Chang, W. Y., Zhang, H., An, J., Ho, F., et al. (2023). Gasdermin D permeabilization of mitochondrial inner and outer membranes accelerates and enhances pyroptosis. Immunity 56, 2523–2541.e8. doi:10.1016/j.immuni.2023.10.004
Moiseeva, V., Cisneros, A., Sica, V., Deryagin, O., Lai, Y., Jung, S., et al. (2023). Senescence atlas reveals an aged-like inflamed niche that blunts muscle regeneration. Nature 613, 169–178. doi:10.1038/s41586-022-05535-x
Ocansey, D. K. W., Yuan, J., Wei, Z., Mao, F., and Zhang, Z. (2023). Role of ferroptosis in the pathogenesis and as a therapeutic target of inflammatory bowel disease (Review). Int. J. Mol. Med. 51, 53. doi:10.3892/ijmm.2023.5256
Ogrodnik, M., Carlos Acosta, J., Adams, P. D., d’Adda di Fagagna, F., Baker, D. J., Bishop, C. L., et al. (2024). Guidelines for minimal information on cellular senescence experimentation in vivo. Cell 187, 4150–4175. doi:10.1016/j.cell.2024.05.059
Onyiah, J. C., and Colgan, S. P. (2016). Cytokine responses and epithelial function in the intestinal mucosa. Cell Mol. Life Sci. 73, 4203–4212. doi:10.1007/s00018-016-2289-8
Patankar, J. V., and Becker, C. (2020). Cell death in the gut epithelium and implications for chronic inflammation. Nat. Rev. Gastroenterol. Hepatol. 17, 543–556. doi:10.1038/s41575-020-0326-4
Plevris, N., and Lees, C. W. (2022). Disease monitoring in inflammatory bowel disease: evolving principles and possibilities. Gastroenterology 162, 1456–1475.e1. doi:10.1053/j.gastro.2022.01.024
Rana, N., Privitera, G., Kondolf, H. C., Bulek, K., Lechuga, S., De Salvo, C., et al. (2022). GSDMB is increased in IBD and regulates epithelial restitution/repair independent of pyroptosis. Cell 185, 283–298.e17. doi:10.1016/j.cell.2021.12.024
Risques, R. A., Lai, L. A., Brentnall, T. A., Li, L., Feng, Z., Gallaher, J., et al. (2008). Ulcerative colitis is a disease of accelerated colon aging: evidence from telomere attrition and DNA damage. Gastroenterology 135, 410–418. doi:10.1053/j.gastro.2008.04.008
Royce, G. H., Brown-Borg, H. M., and Deepa, S. S. (2019). The potential role of necroptosis in inflammaging and aging. Geroscience 41, 795–811. doi:10.1007/s11357-019-00131-w
Ruan, H., Leibowitz, B. J., Zhang, L., and Yu, J. (2020). Immunogenic cell death in colon cancer prevention and therapy. Mol. Carcinog. 59, 783–793. doi:10.1002/mc.23183
Sangfuang, N., McCoubrey, L. E., Awad, A., Marzorati, M., Ghyselinck, J., Verstrepen, L., et al. (2025). Effects of senotherapeutics on gut microbiome dysbiosis and intestinal inflammation in Crohn’s disease: a pilot study. Transl. Res. 278, 36–47. doi:10.1016/j.trsl.2025.02.004
Schmitt, C. A., Wang, B., and Demaria, M. (2022). Senescence and cancer - role and therapeutic opportunities. Nat. Rev. Clin. Oncol. 19, 619–636. doi:10.1038/s41571-022-00668-4
Seo, J., Nam, Y. W., Kim, S., Oh, D.-B., and Song, J. (2021). Necroptosis molecular mechanisms: recent findings regarding novel necroptosis regulators. Exp. Mol. Med. 53, 1007–1017. doi:10.1038/s12276-021-00634-7
Sienkiewicz, M., Sroka, K., Binienda, A., Jurk, D., and Fichna, J. (2023). A new face of old cells: an overview about the role of senescence and telomeres in inflammatory bowel diseases. Ageing Res. Rev. 91, 102083. doi:10.1016/j.arr.2023.102083
Strunk, F. J., Die Rolle des Signalnetzwerks von CD226, TIGIT und CD96 für die humane T-Zell-Antwort und die Pathogenese von chronisch entzündlichen Darmerkrankungen, (2024). doi:10.17169/refubium-42936
Tang, D., Chen, X., Kang, R., and Kroemer, G. (2021). Ferroptosis: molecular mechanisms and health implications. Cell Res. 31, 107–125. doi:10.1038/s41422-020-00441-1
Tang, D., Chen, X., and Kroemer, G. (2022). Cuproptosis: a copper-triggered modality of mitochondrial cell death. Cell Res. 32, 417–418. doi:10.1038/s41422-022-00653-7
Tower, J. (2015). Programmed cell death in aging. Ageing Res. Rev. 23, 90–100. doi:10.1016/j.arr.2015.04.002
Tsvetkov, P., Coy, S., Petrova, B., Dreishpoon, M., Verma, A., Abdusamad, M., et al. (2022). Copper induces cell death by targeting lipoylated TCA cycle proteins. Science 375, 1254–1261. doi:10.1126/science.abf0529
Vanlangenakker, N., Vanden Berghe, T., and Vandenabeele, P. (2012). Many stimuli pull the necrotic trigger, an overview. Cell Death Differ. 19, 75–86. doi:10.1038/cdd.2011.164
Varricchi, G., Bencivenga, L., Poto, R., Pecoraro, A., Shamji, M. H., and Rengo, G. (2020). The emerging role of T follicular helper (TFH) cells in aging: influence on the immune frailty. Ageing Res. Rev. 61, 101071. doi:10.1016/j.arr.2020.101071
Wahida, A., Müller, M., Hiergeist, A., Popper, B., Steiger, K., Branca, C., et al. (2021). XIAP restrains TNF-driven intestinal inflammation and dysbiosis by promoting innate immune responses of Paneth and dendritic cells. Sci. Immunol. 6, eabf7235. doi:10.1126/sciimmunol.abf7235
Wang, F., Gómez-Sintes, R., and Boya, P. (2018). Lysosomal membrane permeabilization and cell death. Traffic 19, 918–931. doi:10.1111/tra.12613
Wang, H., Sun, Y., Xiao, F.-J., Zhao, X., Zhang, W.-Y., Xia, Y.-J., et al. (2023). Mesenchymal stem cells ameliorate DSS-induced experimental colitis by modulating the gut microbiota and MUC-1 pathway. J. Inflamm. Res. 16, 2023–2039. doi:10.2147/JIR.S402592
Wang, L., Hong, W., Zhu, H., He, Q., Yang, B., Wang, J., et al. (2024). Macrophage senescence in health and diseases. Acta Pharm. Sin. B 14, 1508–1524. doi:10.1016/j.apsb.2024.01.008
Wang, R., Li, H., Wu, J., Cai, Z.-Y., Li, B., Ni, H., et al. (2020b). Gut stem cell necroptosis by genome instability triggers bowel inflammation. Nature 580, 386–390. doi:10.1038/s41586-020-2127-x
Wang, X., Bootsma, H., Kroese, F., Dijkstra, G., and Pringle, S. (2020a). Senescent stem and transient amplifying cells in crohn’s disease intestine. Inflamm. Bowel Dis. 26, e8–e9. doi:10.1093/ibd/izz295
Wang, Z., Wu, H., Chang, X., Song, Y., Chen, Y., Yan, Z., et al. (2025). CKMT1 deficiency contributes to mitochondrial dysfunction and promotes intestinal epithelial cell apoptosis via reverse electron transfer-derived ROS in colitis. Cell Death Dis. 16, 177. doi:10.1038/s41419-025-07504-4
Watanabe, S., Hibiya, S., Katsukura, N., Kitagawa, S., Sato, A., Okamoto, R., et al. (2022). Importance of telomere shortening in the pathogenesis of ulcerative colitis: a new treatment from the aspect of telomeres in intestinal epithelial cells. J. Crohns Colitis 16, 109–121. doi:10.1093/ecco-jcc/jjab115
Wei, Y., Jia, S., Ding, Y., Xia, S., and Giunta, S. (2023). Balanced basal-levels of ROS (redox-biology), and very-low-levels of pro-inflammatory cytokines (cold-inflammaging), as signaling molecules can prevent or slow-down overt-inflammaging, and the aging-associated decline of adaptive-homeostasis. Exp. Gerontol. 172, 112067. doi:10.1016/j.exger.2022.112067
Welz, P.-S., Wullaert, A., Vlantis, K., Kondylis, V., Fernández-Majada, V., Ermolaeva, M., et al. (2011). FADD prevents RIP3-mediated epithelial cell necrosis and chronic intestinal inflammation. Nature 477, 330–334. doi:10.1038/nature10273
Xie, X., Wu, Z., Wu, Y., Liu, J., Chen, X., Shi, X., et al. (2022). Cysteine protease of Clonorchis sinensis alleviates DSS-induced colitis in mice. PLoS Negl. Trop. Dis. 16, e0010774. doi:10.1371/journal.pntd.0010774
Xu, J., Liu, S., Cui, Z., Wang, X., Ning, T., Wang, T., et al. (2021). Ferrostatin-1 alleviated TNBS induced colitis via the inhibition of ferroptosis. Biochem. Biophys. Res. Commun. 573, 48–54. doi:10.1016/j.bbrc.2021.08.018
Yan, R., Jia, D., Qi, Y., Wang, Q., and Chen, S. (2025). Intestinal tissue-resident memory T cells: characteristics, functions under physiological and pathological conditions and spatial specificity. J. Adv. Res. S2090-1232 (25). doi:10.1016/j.jare.2025.03.021
Yang, C., Wang, W., Li, S., Qiao, Z., Ma, X., Yang, M., et al. (2023). Identification of cuproptosis hub genes contributing to the immune microenvironment in ulcerative colitis using bioinformatic analysis and experimental verification. Front. Immunol. 14, 1113385. doi:10.3389/fimmu.2023.1113385
Yang, L., Yuan, L., and Liu, G. (2024). Comprehensive evaluation of disulfidptosis in intestinal immunity and biologic therapy response in Ulcerative Colitis. Heliyon 10, e34516. doi:10.1016/j.heliyon.2024.e34516
Yao, B., Zhang, Y., Wu, Q., Yao, H., Peng, L., Jiang, Z., et al. (2024). Comprehensive assessment of cellular senescence in intestinal immunity and biologic therapy response in ulcerative colitis. Sci. Rep. 14, 28127. doi:10.1038/s41598-024-79607-5
Yao, X., Huang, J., Zhong, H., Shen, N., Faggioni, R., Fung, M., et al. (2014). Targeting interleukin-6 in inflammatory autoimmune diseases and cancers. Pharmacol. Ther. 141, 125–139. doi:10.1016/j.pharmthera.2013.09.004
You, H.-M., Wang, L., Meng, H.-W., Huang, C., Fang, G.-Y., and Li, J. (2023). Pyroptosis: shedding light on the mechanisms and links with cancers. Front. Immunol. 14, 1290885. doi:10.3389/fimmu.2023.1290885
Yuan, J., and Ofengeim, D. (2024). A guide to cell death pathways. Nat. Rev. Mol. Cell Biol. 25, 379–395. doi:10.1038/s41580-023-00689-6
Yun, J., Hansen, S., Morris, O., Madden, D. T., Libeu, C. P., Kumar, A. J., et al. (2023). Senescent cells perturb intestinal stem cell differentiation through Ptk7 induced noncanonical Wnt and YAP signaling. Nat. Commun. 14, 156. doi:10.1038/s41467-022-35487-9
Zarrin, A. A., Bao, K., Lupardus, P., and Vucic, D. (2021). Kinase inhibition in autoimmunity and inflammation. Nat. Rev. Drug Discov. 20, 39–63. doi:10.1038/s41573-020-0082-8
Zhang, J., Cen, L., Zhang, X., Tang, C., Chen, Y., Zhang, Y., et al. (2022a). MPST deficiency promotes intestinal epithelial cell apoptosis and aggravates inflammatory bowel disease via AKT. Redox Biol. 56, 102469. doi:10.1016/j.redox.2022.102469
Zhang, J., Rane, G., Dai, X., Shanmugam, M. K., Arfuso, F., Samy, R. P., et al. (2016). Ageing and the telomere connection: an intimate relationship with inflammation. Ageing Res. Rev. 25, 55–69. doi:10.1016/j.arr.2015.11.006
Zhang, S., Liang, Y., Yao, J., Li, D.-F., and Wang, L.-S. (2022c). Role of pyroptosis in inflammatory bowel disease (IBD): from gasdermins to DAMPs. Front. Pharmacol. 13, 833588. doi:10.3389/fphar.2022.833588
Zhang, T., Ding, C., Chen, H., Zhao, J., Chen, Z., Chen, B., et al. (2022b). m6A mRNA modification maintains colonic epithelial cell homeostasis via NF-κB-mediated antiapoptotic pathway. Sci. Adv. 8, eabl5723. doi:10.1126/sciadv.abl5723
Zhang, T., Wang, Y., Inuzuka, H., and Wei, W. (2022d). Necroptosis pathways in tumorigenesis. Semin. Cancer Biol. 86, 32–40. doi:10.1016/j.semcancer.2022.07.007
Zheng, T., Liu, Q., Xing, F., Zeng, C., and Wang, W. (2023). Disulfidptosis: a new form of programmed cell death. J. Exp. Clin. Cancer Res. 42, 137. doi:10.1186/s13046-023-02712-2
Zhou, L., Zhou, W., Joseph, A. M., Chu, C., Putzel, G. G., Fang, B., et al. (2022). Group 3 innate lymphoid cells produce the growth factor HB-EGF to protect the intestine from TNF-mediated inflammation. Nat. Immunol. 23, 251–261. doi:10.1038/s41590-021-01110-0
Zhu, Y., Qin, H., Sun, C., Shao, B., Li, G., Qin, Y., et al. (2022). Endometrial regenerative cell-derived exosomes attenuate experimental colitis through downregulation of intestine ferroptosis. Stem Cells Int. 2022, 3014123. doi:10.1155/2022/3014123
Keywords: inflammatory bowel disease, cellular senescence, cell death, immunosenescence, inflammaging
Citation: Yang L and Yuan L (2025) The role and intrinsic connection of cellular senescence and cell death in inflammatory bowel disease. Front. Cell Dev. Biol. 13:1502531. doi: 10.3389/fcell.2025.1502531
Received: 30 September 2024; Accepted: 09 April 2025;
Published: 24 April 2025.
Edited by:
Yan Chun Li, The University of Chicago, United StatesReviewed by:
Bianca Vezzani, University of Parma, ItalyAtsushi Kitani, National Institute of Allergy and Infectious Diseases (NIH), United States
Copyright © 2025 Yang and Yuan. This is an open-access article distributed under the terms of the Creative Commons Attribution License (CC BY). The use, distribution or reproduction in other forums is permitted, provided the original author(s) and the copyright owner(s) are credited and that the original publication in this journal is cited, in accordance with accepted academic practice. No use, distribution or reproduction is permitted which does not comply with these terms.
*Correspondence: Lianwen Yuan, eXVhbmxpYW53ZW5AY3N1LmVkdS5jbg==