- 1Zhongshan Clinical College, Dalian University, Dalian, China
- 2National and Local Joint Engineering Laboratory for Orthopedic Implant Material Development, Affiliated Zhongshan Hospital of Dalian University, Dalian, China
Annexin A5 (ANXA5), also known as Annexin V, is a calcium-dependent phospholipid-binding protein and has a high affinity with phosphatidylserine (PS). This characteristic facilitates its involvement in a wide range of biological functions, including vesicle transport, the formation of mineral phases in the extracellular matrix, anticoagulation and antithrombotic, the inhibition of tumor growth, and apoptosis regulation. ANXA5 plays a role in anti-inflammatory and antithrombotic properties. It also has protective effects on the nervous system. ANXA5 has been reported to facilitate osteogenic differentiation and take part in chondrocyte apoptosis and mineralization. More and more attention is paid to the potential of ANXA5 for bone defect repair. Most current studies on ANXA5 mainly concentrate on immune disorders, pregnancy disorders and serve as a biomarker for various diseases as well as apoptosis detection. However, there is still a lack of systematic studies on ANXA5 involving multiple tissues, including bone, cartilage, vessels, and nerves in the process of bone regeneration. Our study aims to summarize the biological functions in bone tissue and the related signaling pathways of ANXA5. This work provides a theoretical foundation for applying ANXA5 in clinical orthopedics in the future.
1 Introduction
Annexins (ANXs) comprise a multigene family consisting of approximately 500 members, which are found in vertebrates, invertebrates, fungi, plants, and protozoa. Twelve kinds of common Annexins in vertebrates belong to the Annexin A family, named ANXA1 to ANXA11 and ANXA13 (Moss and Morgan, 2004). Annexins are classified into several families: the B family (invertebrates), the C family (fungi and certain unicellular eukaryotes), the D family (plants) and the E family (protozoa) (Gerke et al., 2005; Xi et al., 2020).
ANXA5 (Annexin A5), also known as Annexin V, is a key member of the Annexin A family. ANXA5 was first found by Bohn et al., who isolated the ANXA5 protein from the human placenta and designated it as placental protein 4 (PP4) (Inaba et al., 1984). It is also called calphobindin-I(CPB-I), lipocortin V, placental anticoagulant protein I (PAP-I), endonexin II(E-II), vascular anticoagulant-α(VAC α) and anchorin CII. ANXA5 is a single-chain protein weighing 35–36 kD (Jing, 2024), and it is encoded by a gene located on chromosomes 4q26-q28 (Tait et al., 1991). ANXA5 forms protein-protein interactions by binding to specific sites of the S100 dimer (Rescher and Gerke, 2004; Rintala-Dempsey et al., 2008). The ANXA5 has a conserved C-terminal domain and an N-terminal domain. The C-terminal is a core domain composed of four repeated sequences. The core domain contains approximately 70 amino acid residues (Gerke and Moss, 2002; Lizarbe et al., 2013; Xi et al., 2020). Each repeated sequence of the C-terminal has five α-helices, including calcium-binding motifs and mediating the binding to negatively charged phospholipids. The N-terminal domain of ANXA5 contains calcium-binding sites and phosphorylation sites (Qi et al., 2015; Woodward et al., 2022). (Figure 1A)
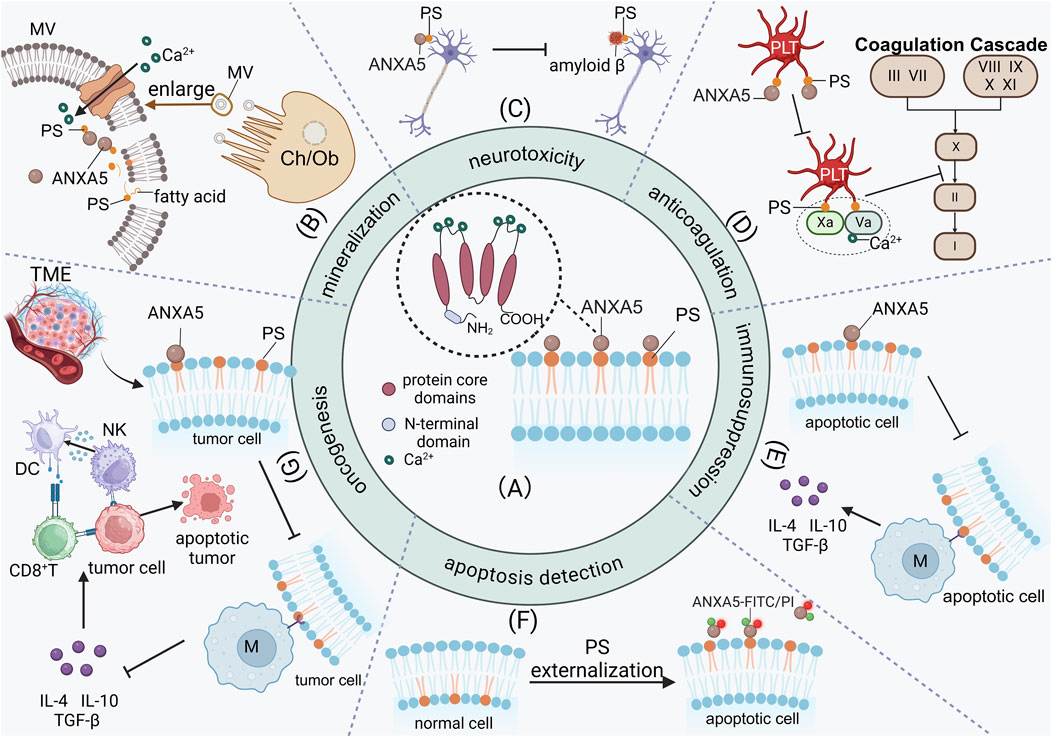
Figure 1. The high affinity of ANXA5 with PS facilitates its participation in a wide range of biological processes. (A) The ANXA5 has a conserved C-terminal core domain and an N-terminal domain. The C-terminal is a core domain composed of four repeated sequences. The C-terminal has calcium-binding motifs and mediates the binding to negatively charged phospholipids. (B) In the extracellular matrix of osteoblasts and chondrocytes, ANXA5 facilitates mineralization by binding to PS-rich matrix vesicle membranes, acceleratingCa2+ influx and subsequently promoting hydroxyapatite deposition in the extracellular matrix. (C) The characteristic of ANXA5 inhibits the binding between amyloid β and PS, resulting in the accumulation of neurotoxic amyloid β in the choroid plexus of Alzheimer’s disease patients. (D) ANXA5 binds to and interacts with activated platelets, competitively inhibiting the binding of coagulation factors (Va and Xa) to activated platelets, thus preventing the initiation of the coagulation cascade. (E) ANXA5 inhibits macrophages phagocytosis of apoptotic cells and promotes the release of immunosuppressive cytokines, including IL-4, IL-10, and TGF-β thereby exerting immunosuppression. (F) The characteristic of ANXA5 is particularly significant because of PS externalization during cell apoptosis, making ANXA5 a key agent for detecting cell apoptosis in vivo. (G) In tumor cells, ANXA5 inhibits macrophages from secreting immunosuppressive cytokines IL-4, IL-10, and TGF-β by blocking PS exposure on tumor cells. Dendritic cells regain the ability to present antigens to CD8+T cells, shifting the immune profile from immunosuppressive to immune-active. MV, Matrix vesicle; PS, Phosphatidylserine; PLT, Platelet; Ch, Chondrocytes; Ob, Osteoblasts; M, Macrophage; TME, Tumor microenvironment; DC, Dendritic cells; NK, Natural killer cell.
ANXA5 is found in different tissues. Noteworthily, ANXA5 is known for its ability to bind to phosphatidylserine (PS) on cell membranes with high affinity in a calcium-dependent manner (Boersma et al., 2005; Schutters and Reutelingsperger, 2010). This characteristic is particularly significant, making ANXA5 participate in various biological processes in vivo, including vesicle transport, mineralization in the extracellular matrix, neurotoxicity in Alzheimer’s disease, anticoagulation and antithrombotic, apoptosis regulation and detection, and inhibition of tumor cell growth (Krey et al., 2016). In the extracellular matrix of osteocytes and chondrocytes, ANXA5 facilitates mineralization through its binding to PS-rich matrix vesicle membranes, which accelerates Ca2+ influx and subsequently promotes the deposition of hydroxyapatite crystals in the extracellular matrix (Ansari et al., 2021; Wuthier and Lipscomb, 2011; Genge et al., 2008) (Figure 1B). Furthermore, the characteristic of ANXA5 for binding to PS also plays a role in neurological disorders. For example, the characteristic of ANXA5 inhibits the binding between amyloid β and PS, resulting in the accumulation of neurotoxic amyloid β in the choroid plexus of Alzheimer’s disease patients, ultimately leading to cellular death (Bartolome et al., 2020; Lee et al., 2002) (Figure 1C). Additionally, ANXA5 has an antithrombotic function in the blood vessels. ANXA5 can displace coagulation factors from procoagulant phospholipids, thereby inhibiting the coagulation cascade in vitro (Cederholm and Frostegård, 2007; Reddy and Rand, 2020; Ravanat et al., 1992) (Figure 1D). The disruption of the anticoagulation pathway involving ANXA5 is associated with antiphospholipid syndrome (Bogdanova et al., 2012; Mineo et al., 2023) and its pregnancy complications, including recurrent pregnancy loss (Aranda et al., 2018; Rogenhofer et al., 2018; Ang et al., 2017). ANXA5 also has anti-inflammatory properties. The binding between ANXA5 and PS effectively inhibits PS-mediated adhesion of platelets and white blood cells to the endothelium, consequently mitigating systemic inflammation (Ewing et al., 2011; Ewing et al., 2012). PS mainly exists in the inner leaflets of cells. The characteristic of ANXA5 is particularly significant because of PS externalization during cell apoptosis, making ANXA5 a key agent for detecting cell apoptosis in vivo (Rieger et al., 2011; Kumar et al., 2021; Krysko et al., 2004; Miyagishima et al., 2024) (Figure 1F). PS externalization serves as a primary signal for macrophages to recognize and clear apoptotic cells (Munoz et al., 2007). ANXA5 selectively binds to PS on the surface of apoptotic cells with high affinity, thereby competitively inhibiting the phagocytosis of apoptotic cells by macrophages and consequently exerting an immunosuppressive effect (Stach et al., 2000; Böttcher et al., 2006; Munoz et al., 2007) (Figure 1E). In tumor cells, ANXA5 enables dendritic cells to regain their capacity for antigen presentation to CD8+ T cells by blocking PS exposure on tumor cells. Furthermore, ANXA5 inhibits the secretion of immunosuppressive cytokines in macrophages, thereby shifting the immune profile from immunosuppressive to immune-active. (Yan et al., 2012; Bondanza et al., 2004; Kang et al., 2020) (Figure 1G). Through this mechanism, ANXA5 alleviates the immunosuppressive effects induced by chemotherapy and enhances the antitumor efficacy and immunogenicity of tumor antigen-specific immune responses (Bondanza et al., 2004; Chaurio et al., 2009; Frey et al., 2009; Gray et al., 2016).
Bone regeneration is a complex physiological process that involves the co-regulation of various phenomena, including endochondral ossification, osteogenic differentiation, angiogenesis, neurogenesis, and so on (Salhotra et al., 2020). Numerous studies have indicated that ANXA5 is able to promote osteoblast differentiation and prevent osteoporosis (Su et al., 2023; Genetos et al., 2014; Shimada et al., 2018). Additionally, ANXA5 facilitates the apoptosis and terminal differentiation of chondrocytes and promotes chondrocyte mineralization, indicating its significant role in bone regeneration (Shimada et al., 2018; Wang and Kirsch, 2006; Kirsch et al., 2000). Currently, there are no reviews on ANXA5 in bone tissue. Most reviews focus on cardiovascular disease (Cederholm and Frostegård, 2007), immune disorders (Bećarević, 2016; Rand et al., 2010), tumorigenesis (Woodward et al., 2022; Peng et al., 2014), pregnancy complications (Bogdanova et al., 2012; Peng et al., 2022)and the detection of apoptosis (Laufer et al., 2008). In this study, we provide a detailed description of these physiological processes and summarize the biological functions and associated signaling pathways of ANXA5 within the field of bone tissue. We aim to provide a theoretical foundation for applying ANXA5 in clinical orthopedics in the future.
2 The function of ANXA5 in bone tissue
2.1 The expression of ANXA5 in bone tissue
Suarez et al. isolated osteoblasts from the skull of newborn rats and detected the cellular protein extracts of these osteoblasts by Western blotting. They found that ANXA5 was expressed in the osteoblast cell lineage (Suarez et al., 1993). Brachvogel et al. analyzed ANXA5 in frozen sections of mouse embryos through immunohistochemistry, discovering that ANXA5 was present in frozen sections of the developing lumbar arch. The ANXA5 gene plays a role in the cellular lineage of skeletal system development, and the researchers believe it may represent a novel marker characterizing involved in this process (Brachvogel et al., 2001). Su et al. detected the expression of ANXA5 in bone tissue in vivo and primary osteoblasts in vitro from both sham and osteoporotic mice by Western blotting (Su et al., 2023). Mohiti et al. obtained bone tissue from human knee joints and then isolated osteoblasts, confirming the presence of ANXA5 in these cells through Western blotting. They determined that the cellular content of ANXA5 was found to be 0.18% ± 0.010% (n = 9) of total cell protein in primary cultures of osteoblasts using quantitative immunoblotting. Additionally, the localization of ANXA5 in MG-63 osteosarcoma cells was determined using immunofluorescence microscopy, which revealed that ANXA5 was always strongly present in the nucleus with additional cytoplasmic staining (Mohiti et al., 1995).
2.2 ANXA5 promotes the proliferation and differentiation of osteogenic cells
After knocking down the ANXA5 gene in preosteoblast MC3T3 cells, Genetos et al. observed a reduction in cell proliferation, as measured by Calcein-AM and Alamar Blue staining. When MC3T3 cells were cultured under osteogenic differentiation-inducing conditions, ANXA5 revealed maximal expression at 14 days. These findings suggest that ANXA5 can influence bone formation via the regulation of osteoprogenitor proliferation and differentiation in addition to the function in matrix vesicles (MVs) (Genetos et al., 2014). Shimada et al. reported that after knocking down the ANXA5 gene in primary cultures of osteoblasts in vitro, subsequent qPCR analysis resulted in decreased Runx2 and osteopontin expression. This suggests that ANXA5 plays a role in promoting osteoblast differentiation (Shimada et al., 2018). Furthermore, Su et al. found that ANXA5 expression was significantly downregulated in bone tissue and isolated osteoblasts of osteoporosis mice compared to those of the sham mice. After transfecting the shANXA5 plasmid into the preosteoblastic cell line MC3T3, Western blot analysis indicated a significant reduction in osteogenic differentiation-related markers (Su et al., 2023).
However, further research conducted by Brachvogel et al. indicates that ANXA5 is not essential for bone development. In ANXA5-deficient mouse mutants, no serious defects related to the ossification process were observed. X-ray analyses of the skeleton from 6-month-old mice revealed no significant differences in size or the density of the bone. Additionally, histological analysis of the tibia from newborn mice displayed no overt changes in the organization of the growth plate in the absence of ANXA5. These findings demonstrate that mice lacking ANXA5 can develop normally and reveal no significant alterations in the biochemical parameters characteristic of metabolic or functional defects. This may be due to a compensatory effect of other members from the annexin family arising from the high functional and structural similarity (Brachvogel et al., 2003).
2.3 ANXA5 enhances the mineralization process in osteoblasts
Su et al. conducted a study in which they transfected the shANXA5 plasmid into the preosteoblast cell line MC3T3. The results from ALP staining and alizarin red staining showed that shANXA5 decreased the number of ALP-positive cells and inhibited the formation of mineralized nodules. Conversely, in cells that overexpressed ANXA5, these results were significantly enhanced, confirming that ANXA5 mediates the mineralization of the precursor osteoblast lineage (Su et al., 2023). Additionally, when Genetos et al. applied ANXA2 siRNA and ANXA5 siRNA to preosteoblast MC3T3 cells, they observed a decrease in osteogenic marker ALP staining and a significant reduction in mineralized nodule formation after knockdown. This further supports that ANXA5 plays a significant role in promoting osteogenic differentiation (Genetos et al., 2014).
Su also found that ANXA5 is highly expressed in osteoblast adhesion MVs (Su et al., 2023). It is widely known that type I collagen is the main organic extracellular matrix component in osteoblasts (Kim and Kirsch, 2008) (Figure 2C). In MC3T3 cells with ANXA5 knockdown, there was a decrease in the number of MVs attaching to the cellular matrix, which suggests that ANXA5 may regulate the interaction between MVs and the extracellular matrix. In both in vivo bone tissue and in vitro osteoblasts, immunofluorescence double staining revealed colocalization between ANXA5 and type I collagen. Subsequent co-immunoprecipitation experiments demonstrated the direct binding between MVs and type I collagen. These results further confirm that ANXA5, located on the MVs membrane, can directly attach to type I collagen. Therefore, it is suggested that ANXA5 may play a protective role against bone loss by promoting MVs adhesion to the extracellular matrix through its interaction with type I collagen (Su et al., 2023) (Figure 2D).
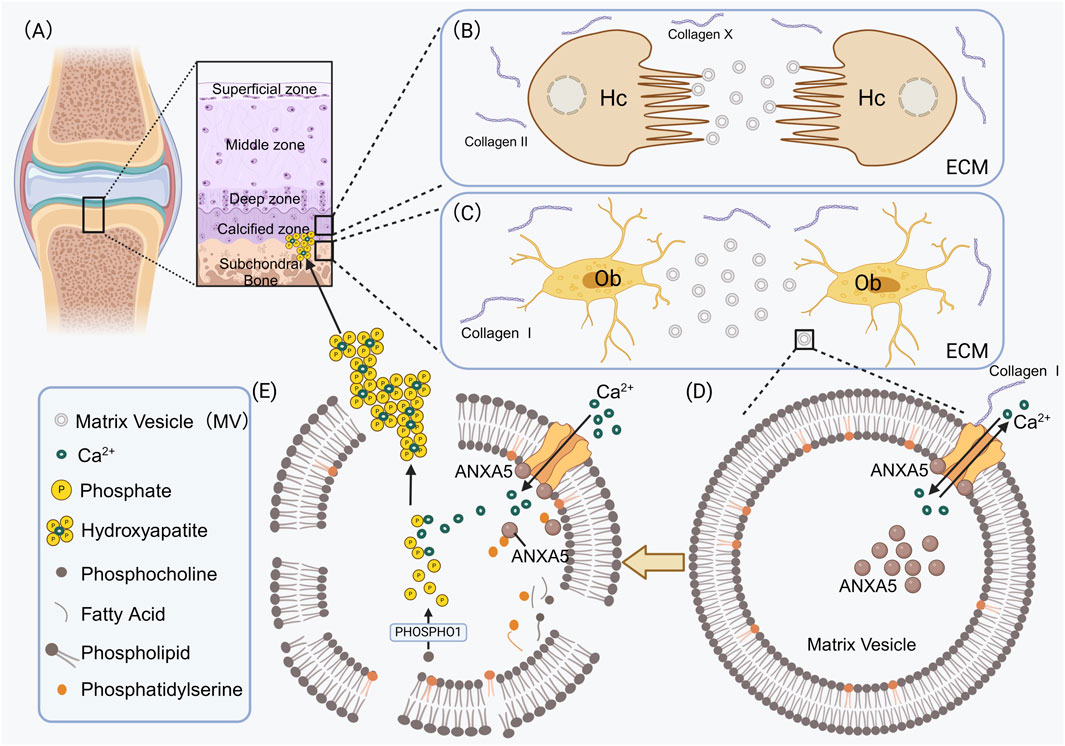
Figure 2. ANXA5 participates in the mineralization process of the extracellular matrix in osteoblasts and chondrocytes. (A) Hypertrophic chondrocytes form mineralized cartilage tissue in the calcified zone; osteoblasts form mineralized bone tissue in the subchondral bone. (B) Type II and Type X collagens constitute the primary components of the extracellular matrix in hypertrophic chondrocytes. (C) Type I collagens constitute the primary components of the extracellular matrix in osteoblasts. MVs sprout from the surface of osteoblasts and subsequently enter the extracellular matrix. (D) In the MVs secreted by osteoblasts or hypertrophic chondrocytes, Ca2+ transport ANXA5-mediated maintains MVs homeostasis. For instance, in MVs secreted by osteoblasts, type I collagens bind to ANXA5 on the surface of MVs, thereby accelerating Ca2+ influx. ANXA5 serves not only as a transmembrane calcium channel but also as a calcium-binding protein that accumulates within the MVs. (E) The membranes of MVs are abundant in PS. In the presence of calcium, ANXA5 binds to PS, thereby accelerating the Ca2+ influx. Phosphocholines release phosphates through PHOSPHO1, which combines with calcium ions to form hydroxyapatite crystals inside the MVs. Hydroxyapatite crystals are deposited in the extracellular matrix to complete the mineralization of cartilage. Hc, Hypertrophic chondrocytes; Ob, Osteoblasts; ECM, Extracellular matrix; PHOSPHO1, Phosphoethanolamine/Phosphocholine phosphatase1; Collagen I, type I collagen; Collagen II, type II collagen.
3 The function of ANXA5 in cartilage tissue
3.1 The expression of ANXA5 in cartilage tissue
ANXA5 is essential for the normal proliferation and hypertrophy of chondrocytes. Castagnola et al. demonstrated that ANXA5 mRNA reaches its maximum level in hypertrophic stage II chondrocytes (Castagnola and Cancedda, 1991). Rahman and Giambanco showed that ANXA5 expression is closely linked to the differentiation of chondrocytes and skeletal muscle cells during limb development (Rahman et al., 1997; Giambanco et al., 1991). Shimada et al. examined the entire tibia and femur of ANXA5+/−mice. They found that ANXA5 is expressed at the tibial attachment site, the periosteum, the articular cartilage surface, and the growth plate cartilage (Shimada et al., 2018).
3.2 ANXA5 enhances chondrocyte mineralization
3.2.1 ANXA5 interacts with type II and type X collagen, accelerating the Ca2+ influx and promoting chondrocyte mineralization
Unlike osteoblasts, ANXA5 on the surface of MVs secreted by chondrocytes plays a crucial role in chondrocyte mineralization (Figure 2A). This function is achieved through its interaction with extracellular matrix components, specifically type II collagen and type X collagen (Wuthier and Lipscomb, 2011). (Figure 2B) Several researchers have shown that ANXA5 is involved in the interaction between chondrocytes and extracellular collagen, contributing to the mineral deposition process in primary cultures of chicken growth plate chondrocytes grown in ascorbate-containing media (Mollenhauer et al., 1984; Castagnola and Cancedda, 1991; Pfäffle et al., 1988; Mebarek et al., 2023). Kirsch et al. provided evidence using slot blot assays that ANXA5 not only binds to native type II and X collagen but also to chondrocalcin, the C-terminal extension of type II collagen in a calcium-independent manner (Kirsch and Pfäffle, 1992). Bolean et al. found that ANXA5-lipoprotein complexes have the highest affinity for type II collagen deposited during chondrocyte mineralization in articular cartilage (Bolean et al., 2020). King et al. discovered that in vitro cultured Swarm rat chondrosarcoma cells lack the ability to bind significant amounts of type II collagen to their surfaces, as compared to normal rat chondrocytes, which correlates with a deficiency of ANXA5 on these cell surfaces (King et al., 1997). Lucic et al. discovered that the N-terminal peptide of type II collagen binds to ANXA5. The binding of C-terminal peptides and triple helical peptide to the chondrocyte surface may occur through other collagen receptors, such as integrins or cell-associated matrix proteins (Lucic et al., 2003). Furthermore, von der Mark K et al. demonstrated that during matrix vesicle-initiated cartilage mineralization, the binding of ANXA5 to collagen significantly facilitates calcium influx into MVs (von der Mark and Mollenhauer, 1997).
The N-terminal domain of ANXA5 has been shown to contain calcium-binding sites that facilitate the influx of calcium (Kim and Kirsch, 2008). ANXA5, which is expressed on the surface of MVs secreted by chondrocytes, interacts with type II and type X collagen. This interaction mediates the flow of Ca2+ into the MVs released by hypertrophic cartilage in the growth plate (Boyan et al., 2022; Anderson, 2003). Phosphocholines release phosphates through PHOSPHO1 (Phosphoethanolamine/Phosphocholine phosphatase), which combines with calcium ions to form Hydroxyapatite crystals inside of the MV. Hydroxyapatite crystals are deposited in the extracellular matrix to complete the mineralization of cartilage (Bolean et al., 2017; Wuthier and Lipscomb, 2011; Chaudhary et al., 2016; Millán, 2013) (Figure 2E). Additionally, due to the close association of matrix vesicles with the extracellular matrix rich in collagen and proteoglycans, Genge BR et al. speculate that this MV protein may be a stretch-activated ion channel component that enhances Ca2+ uptake during mechanical stress (Genge et al., 1992).
3.2.2 ANXA5 interacts with PS in MVs to accelerate the Ca2+ influx and enhance the mineralization of chondrocytes
ANXA5 is known for its high-affinity binding to PS on cell membranes, a process that depends on calcium concentrations (von der Mark and Mollenhauer, 1997). Research by Köhler et al. has shown that the binding affinity of recombinant ANXA5 proteins to PS-rich MVs is stronger at low pH compared to neutral pH (Köhler et al., 1997). Furthermore, studies by Genge BR and Kirsch T et al. have demonstrated that the membranes of MVs in vivo are rich in PS, which binds to ANXA5. This interaction enhances calcium (Ca2+) influx and promotes intracavicular crystal growth, thereby playing a critical role in the mineralization of the cartilage matrix (Genge et al., 2007; Kirsch et al., 1997).
3.3 ANXA5 promotes apoptosis in cartilage
ANXA5 plays a crucial role in promoting chondrocyte mineralization, which ultimately leads to apoptosis. A key characteristic of osteoarthritis is the eventual apoptosis of chondrocytes following mineralization (Wang et al., 2023a). Research by Mollenhauer has demonstrated that ANXA5 is significantly upregulated in the cartilage of patients with osteoarthritis. The expression and distribution of ANXA5 could serve as a histological marker for metabolic alterations and changes in cell phenotype associated with osteoarthritis (Mollenhauer et al., 1999). Shimada and colleagues have shown that ANXA5 inhibits the proliferation of fibrocartilage and prevents the excessive growth of bone ends (Shimada et al., 2018). Wang et al. discovered that ANXA5 alters Ca2+ homeostasis in growth plate chondrocytes, thereby regulating terminal differentiation and mineralization events (Wang et al., 2005). Additionally, they also found that the binding of ANXA5 to active protein kinase Cα (PKCα) stimulates apoptosis in growth plate chondrocytes. Meanwhile, the interaction of ANXA5 with β5 integrin regulates these processes, ultimately leading to apoptosis (Wang and Kirsch, 2006). Furthermore, research by Kirsch et al. found that human osteoarthritic chondrocytes adjacent to the joint space undergo terminal differentiation and release MVs containing ANXA5, which initiate mineral formation and eventually die by apoptosis (Kirsch et al., 2000).
4 The function of ANXA5 in vessels
4.1 The expression of ANXA5 in vessels
Brachvogel et al. used mice with the ANXA5-lacZ fusion gene to investigate the expression of ANXA5 in mouse blood vessels. Their initial findings revealed that the fusion gene is expressed in cells associated with the embryonic vascular network (Brachvogel et al., 2001). Further research demonstrated that, following X-gal staining of embryonic sections, ANXA5 expression is limited to the primary capillary plexus, the dorsal aorta and extraembryonic tissue during early embryonic development. Perivascular cells (PVCs) are crucial for proper vascular development and play a significant role in maintaining both the structural integrity and contractility of vessels (Brachvogel et al., 2005).
4.2 ANXA5 reduces inflammation in endothelial
Ewing et al. conducted a study demonstrating that ANXA5 reduces local vascular and systemic inflammation and vascular remodeling and improves vascular function, indicating that it has a therapeutic potential against atherosclerotic cardiovascular diseases (Ewing et al., 2011). Tschirhart et al. found that recombinant human ANXA5 protein, by binding to PS, can inhibit endothelial inflammation induced by microvesicles in septic patients (Tschirhart et al., 2023). Additionally, in vitro experiments by Burgmaier et al. revealed that ANXA5 significantly inhibits the capture, rolling, adhesion, and migration of peripheral blood mononuclear cells on TNF-α-activated endothelial cells within inflammatory lesions. The research team also observed that short-term treatment with ANXA5 can decrease inflammation in plaque lesions of atherosclerotic mice by interfering with the recruitment and activation of monocytes at sites of inflammation (Burgmaier et al., 2014).
4.3 ANXA5 suppresses the apoptosis of endothelial cells
Liu et al. demonstrated that knocking out the ANXA5 gene in human umbilical vein endothelial cells (HUVECs) led to a decline in cell viability. Flow cytometry analysis showed that the knockout of ANXA5 promotes apoptosis in HUVECs (Liu et al., 2024). Additionally, it has been reported that anti-ANXA5 antibodies can induce apoptosis in vascular endothelial cells. The clinical study by Habeeb et al. indicated that patients with systemic sclerosis (SSc) have anti-ANXA5 antibodies in their serum and that higher antibody titers are associated with more severe vascular damage (Habeeb et al., 2010; Sugiura and Muro, 1999). Furthermore, Tripathy et al. observed a significant increase in anti-ANXA5 antibody levels in patients with Takayasu’s arteritis (TA), and a corresponding increase in the number of anti-endothelial cell antibodies (AECA) positively correlated. Anti-ANXA5 antibodies were also positively correlated with disease activity, suggesting they play a pathogenic role in the disease (Tripathy et al., 2003).
4.4 ANXA5 promotes angiogenesis and vascular differentiation
Brachvogel et al. isolated perivascular cells (PVC) from ANXA 5-LacZ + mice that specifically expressed ANXA 5 and discovered that they possessed the ability to differentiate into various mesenchymal lineages (Brachvogel et al., 2005). Subsequently, they added growth factors, namely, vascular endothelial growth factor (VEGF) and platelet-derived growth factor (PDGF), to the cell culture medium of PVC from ANXA 5-LacZ + mice. As a consequence, characteristic basement membrane proteins were detected in PVC, which are indicators of mature vascular structures (Brachvogel et al., 2007). Sun et al. discovered that the knockdown of ANXA5 was positively associated with the decrease in the levels of CD34 and VEGF-3, two indicators of angiogenesis, in mice transplanted with liver cancer cells where ANXA5 was knocked down, thereby inhibiting the progression and metastasis of liver cancer in vivo (Sun et al., 2018). The study conducted by Zheng et al. demonstrated that ANXA5 expression is positively correlated with the total vessel length per field in patient liver cancer tissue. Co-culturing human umbilical HUVEC with liver cancer cells HuH-7 of different ANXA5 expression levels revealed that overexpression of ANXA 5 in liver cancer cells enhances the tubulogenic ability of endothelial cells. Conversely, co-culturing with ANX5-knockout liver cancer cells reduced the tubulogenic ability of endothelial cells.
4.5 The anticoagulant and antithrombotic functions of ANXA5
The exposure of PS is crucial for the binding and activity of the prothrombinase complex on activated platelets (Thiagarajan and Tait, 1990; Sang et al., 2021; Zhao et al., 2016). ANXA5, which has a high affinity with PS, can bind to activated platelets and interact with them in a calcium-dependent manner (Connor et al., 2010; Thiagarajan and Tait, 1991). This binding competitively inhibits the attachment of coagulation factors to activated platelets, thereby reducing coagulation. As a result, ANXA5 is recognized as a highly effective anticoagulant protein. In a study by Van Ryn-McKenna et al., heparin and ANXA5 were injected into the injured carotid vein of a denuded rabbit. They discovered that ANXA5 prevented the formation of the prothrombinase complex and significantly decreased thrombus formation (Van Ryn-McKenna et al., 1993). Additionally, research conducted by Li et al. found that ANXA5 can inhibit the expression and activity of tissue factor (TF) and its release induced by homocysteine (Hcy) in vascular smooth muscle cells (VSMCs) (Li et al., 2009).
Bone regeneration is also a critical aspect of vascular development. Kusumbe and his colleagues have demonstrated that the blood vessels in the bone comprise endothelial cells that specifically facilitate bone maturation and regeneration (Kusumbe et al., 2014). While numerous studies have explored the role of ANXA5 in vascular endothelium, and several ANXA5-based drugs have been developed for anticoagulation and antithrombosis—such as recombinant ANXA5 (rANV) (van Heerde et al., 1994; Thiagarajan and Benedict, 1997), ANXA5 derivative (AND) (Ju et al., 2004; Huang et al., 2006), and recombinant ANXA5 anticoagulation fusion protein (Quan et al., 2022). However, the function of ANXA5 in angiogenesis during bone formation has not been investigated. Therefore, research in this aspect might be one of the new directions in the field of bone regeneration.
5 The function of ANXA5 in the nervous system
5.1 The expression of ANXA5 in nerve cells
Giambanco et al. investigated the cellular distribution of ANXA5 in rat tissue utilizing immunohistochemistry, revealing a pronounced positivity in glial cells within both the cerebellum and optic nerve (Giambanco et al., 1991). Additionally, Gotow et al. examined the central nervous tissue of rats using biochemical and morphological techniques. Their findings from immunoblotting and immunoelectron microscopy revealed that ANXA5 is present in neurons, with a focus on axonal terminals and synaptic vesicles. The immunoreactivity for ANXA5 is primarily localized around the cell bodies and dendrites of neurons, as observed through fluorescence and confocal laser scanning microscopy (Gotow et al., 1996).
5.2 The protective function of ANXA5 for neurons
Neurons are present in both the central nervous system and the peripheral nervous system and constitute the building blocks of the functional units of the nervous system. Takei N et al.'s study added recombinant human ANXA5 to embryonic rat neurons. As the amount of recombinant human ANXA5 increased, the neuron survival rate rose, reaching saturation at 30 ng/mL, demonstrating nutritional activity on neurons. Moreover, the addition of an anti-ANXA5 antibody completely inhibited this neural nutritional effect, suggesting that ANXA5 enhances neuron survival in vitro and functions as a paracrine neural nutrient factor in the central nervous system (Takei et al., 1994). The study revealed that in traumatic brain injury (TBI) by Gao et al., the number of apoptotic neurons in the TBI + ANXA5 group was significantly lower than that in the TBI group, indicating that ANXA5 can reduce neuronal apoptosis and exert a neuroprotective role after TBI. Furthermore, they confirmed that ANXA5 alleviates neural inflammation, oxidative stress, and iron-dependent apoptosis by regulating the NF-kB/HMGB1 pathway and the Nrf2/HO-1 antioxidant system (Gao et al., 2023). Current reports focus on neurons in the central nervous system, but there is a lack of studies regarding its role in the peripheral nervous system or its role in bone formation.
6 Potential role of ANXA5 in clinical disease treatment
6.1 Potential role of ANXA5 in bone-related disease treatment
In articles related to the use of ANXA5 for treating bone-related diseases, it is currently in the animal experimental stage, with no reports yet on its application in the clinical stage. Studies on osteoporotic mice have shown that ANXA5 treatment can relieve bone loss caused by osteoporosis, offering a novel strategy for therapeutic intervention for bone loss (Su et al., 2023). Experiments by Zhuoxuan Jia et al. demonstrated that in osteoarthritis rats induced with monosodium iodoacetate, treatment with ANXA5 effectively reduced pain symptoms and inhibited inflammation. These findings suggest new directions for treating osteoarthritis (Jia et al., 2024).
6.2 Potential role of ANXA5 in other diseases treatment
ANXA5 has been reported in multiple clinical trials, primarily focusing on thrombotic diseases (Quan et al., 2022), retinal vein occlusion (Wautier et al., 2011), sepsis and COVID-19 treatment (Martin et al., 2023; Mui et al., 2021). It also exhibits significant clinical potential in targeted drug delivery (Kang et al., 2020), immunotherapy in tumors (Woodward et al., 2022), treatment with systemic lupus erythematosus (Cederholm and Frostegård, 2005), atherosclerosis (Cederholm and Frostegård, 2007) and other diseases. Furthermore, ANXA5 levels may serve as a potential biomarker for preventing asthma (Lee et al., 2018), neurodegenerative disorders (Yamaguchi et al., 2010), intrauterine growth restriction and preeclampsia (Peng et al., 2022). Additionally, its levels could predict mortality in heart failure patients (Schurgers et al., 2016), evaluate lymph node metastasis and tumor grading in colon cancer patients (Sun et al., 2017) and evaluate prognosis evaluation in oral squamous cell carcinoma (Zhou et al., 2024).
7 Mechanisms through which ANXA5 exerts its biological functions
ANXA5 not only promotes osteogenic differentiation, prevents the occurrence of osteoporosis, and facilitates chondrocyte mineralization and apoptosis but also enhances angiogenesis in the vascular endothelium and protects nerve cells. These critical physiological processes are integral to both bone formation and bone repair, as illustrated in Figure 3. ANXA5 also fulfills diverse functions in other normal cells, including alleviating brain injury and intestinal injury, regulating the production of testosterone and the proliferation of interstitial cells in the testis. In tumor cells, ANXA5 enhances the invasive ability of hepatocellular carcinoma cells and inhibits the expression of cyclooxygenase in prostate cancer cells. A summary of the more relevant functions and corresponding signaling pathways (or related molecular mechanism) of ANXA5 in normal tissue and tumor cells is presented below, followed by a detailed account (Table 1).
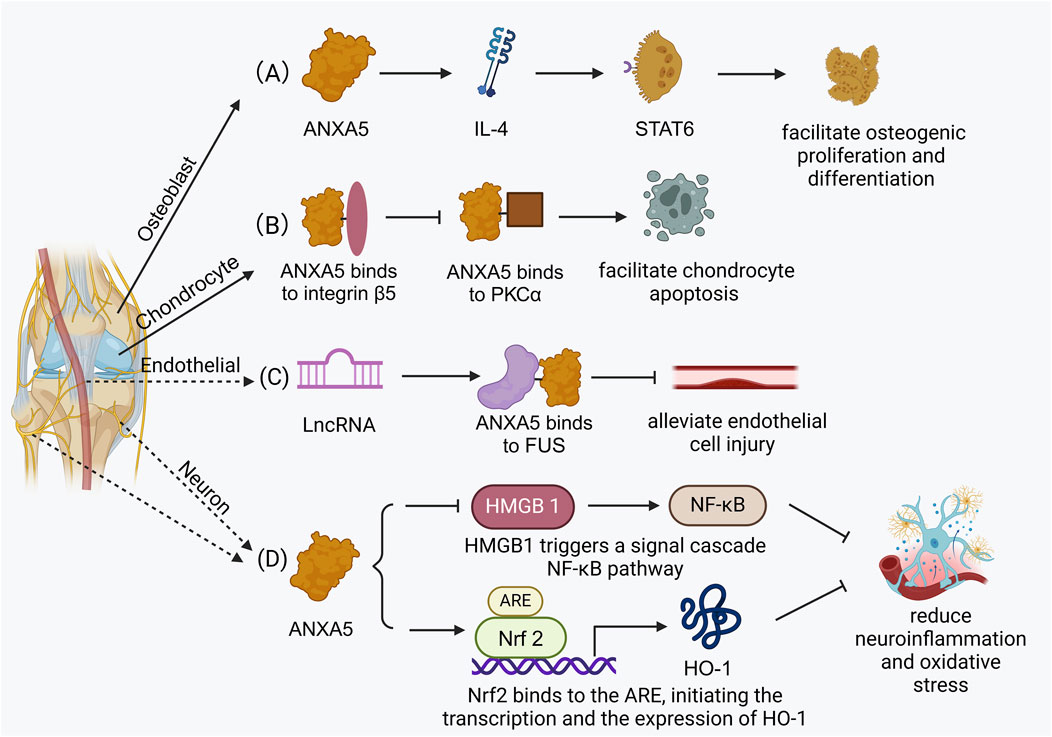
Figure 3. The related biological functions in bone tissue. (A) ANXA5 facilitates osteogenic differentiation via the STAT6 signaling pathway. (B) ANXA5 facilitates chondrocyte apoptosis by integrin β5/ANXA5/PKCβ signaling pathway. (C) ANXA5 alleviates endothelial cell injury and atherosclerosis progression through FUS/ANXA5 signaling pathway. (D) ANXA5 reduces injury-induced neuroinflammation and oxidative stress via NF-ĸB/HMGB1 signaling pathway and Nrf2/HO-1 signaling pathway.
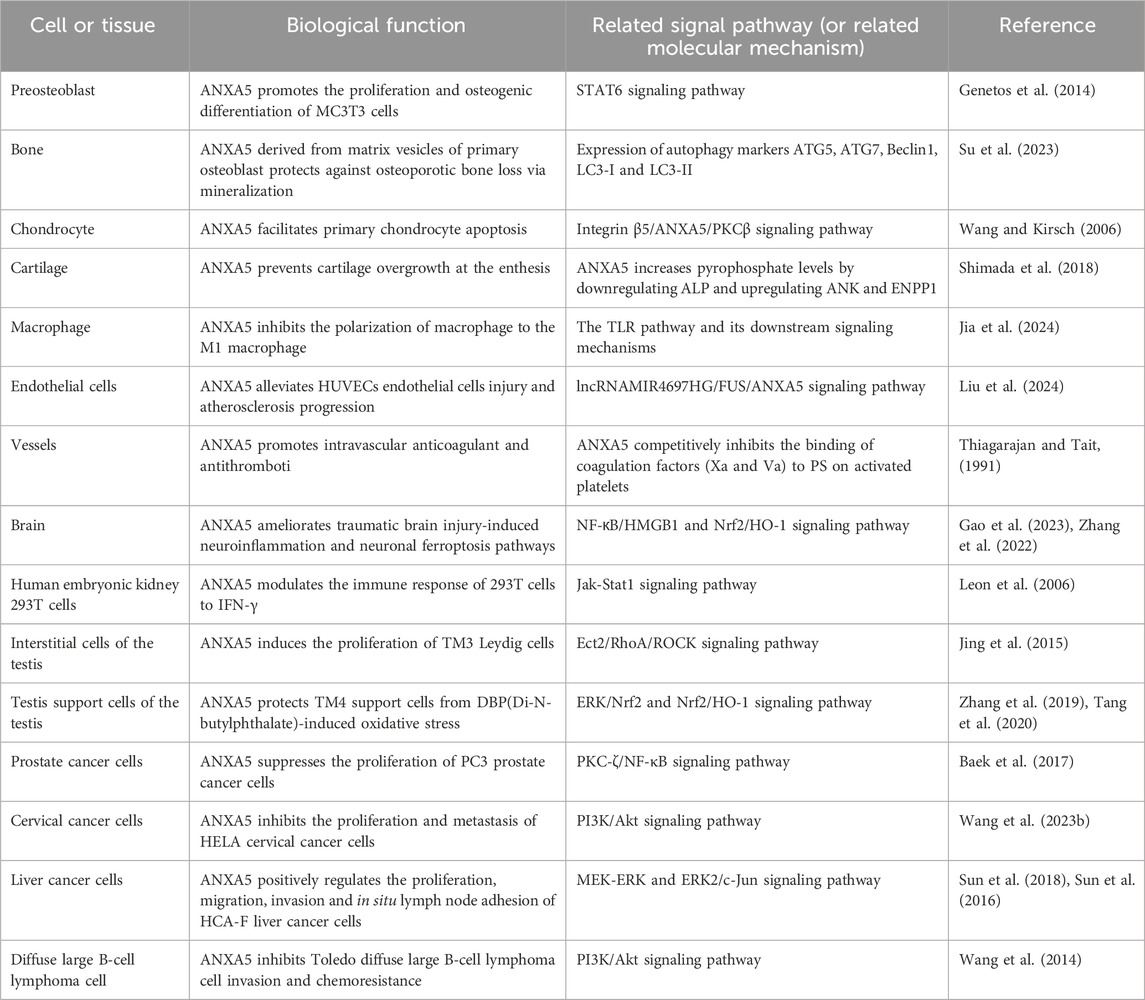
Table 1. Biological functions and related signaling pathways (or related molecular mechanism) involved in ANXA5.
8 Conclusion and perspectives
ANXA5 is expressed in bone, cartilage, vessels, and nerves. ANXA5 facilitates osteoblast differentiation. It can also enhance bone mineralization through the interaction between MVs and type I collagen, ultimately preventing the occurrence of osteoporosis. In chondrocytes, ANXA5 interacts with collagen II and collagen X via MVs to promote chondrocyte mineralization and result in chondrocyte apoptosis. Additionally, ANXA5 inhibits the apoptosis of vascular endothelial cells, and its antibody expression is associated with multiple immune system cardiovascular diseases. ANXA5 also stimulates vascular differentiation and angiogenesis. Besides, ANXA5 promotes intravascular anticoagulant and antithrombotic effects by preventing the binding of blood clotting factors to platelets. Hence, a variety of antithrombotic preparations based on ANXA5 have been developed. Furthermore, ANXA5 has a protective effect on neurons, demonstrating the properties of neurotrophic factors.
Osteogenesis represents a series of complex biological functions, such as bone and cartilage formation and revascularization, as well as the development, maintenance, and regeneration of nerves. The majority of current studies on ANXA5 mainly concentrate on immune disorders, pregnancy disorders, and apoptosis detection. Based on the relevant literature cited in this paper, future research should focus on angiogenesis and neurogenesis in the domain of bone tissue, which are crucial directions for further exploration of bone formation and the development of bone tissue engineering scaffolds.
Author contributions
MJ: Writing – original draft, Writing – review and editing. JZ: Writing – original draft, Writing – review and editing. YS: Writing – review and editing. GL: Writing – review and editing. XW: Funding acquisition, Supervision, Writing – review and editing.
Funding
The author(s) declare that financial support was received for the research and/or publication of this article. This work was supported by the Dalian University Interdisciplinary Projects (DLUXK-2023-QN-014), and the Science & Technological Convenience Foundation of Dalian (No. 2020JJ27SN076).
Conflict of interest
The authors declare that the research was conducted in the absence of any commercial or financial relationships that could be construed as a potential conflict of interest.
Generative AI statement
The author(s) declare that no Generative AI was used in the creation of this manuscript.
Publisher’s note
All claims expressed in this article are solely those of the authors and do not necessarily represent those of their affiliated organizations, or those of the publisher, the editors and the reviewers. Any product that may be evaluated in this article, or claim that may be made by its manufacturer, is not guaranteed or endorsed by the publisher.
References
Anderson, H. C. (2003). Matrix vesicles and calcification. Curr. Rheumatol. Rep. 5 (3), 222–226. doi:10.1007/s11926-003-0071-z
Ang, K. C., Kathirgamanathan, S., Ch'ng, E. S., Lee, Y. Y., Roslani, A. L., Naidu, B., et al. (2017). Genetic analysis of the M2/ANXA5 haplotype as recurrent pregnancy loss predisposition in the Malay population. J. Assist. Reprod. Genet. 34 (4), 517–524. doi:10.1007/s10815-017-0871-0
Ansari, S., de Wildt, B. W. M., Vis, M. A. M., de Korte, C. E., Ito, K., Hofmann, S., et al. (2021). Matrix vesicles: role in bone mineralization and potential use as therapeutics. Pharm. (Basel). 14 (4), 289. doi:10.3390/ph14040289
Aranda, F., Udry, S., Perés Wingeyer, S., Amshoff, L. C., Bogdanova, N., Wieacker, P., et al. (2018). Maternal carriers of the ANXA5 M2 haplotype are exposed to a greater risk for placenta-mediated pregnancy complications. J. Assist. Reprod. Genet. 35 (5), 921–928. doi:10.1007/s10815-018-1142-4
Baek, H. S., Park, N., Kwon, Y. J., Ye, D. J., Shin, S., and Chun, Y. J. (2017). Annexin A5 suppresses cyclooxygenase-2 expression by downregulating the protein kinase C-ζ-nuclear factor-κB signaling pathway in prostate cancer cells. Oncotarget 8 (43), 74263–74275. doi:10.18632/oncotarget.19392
Bartolome, F., Krzyzanowska, A., de la Cueva, M., Pascual, C., Antequera, D., Spuch, C., et al. (2020). Annexin A5 prevents amyloid-β-induced toxicity in choroid plexus: implication for Alzheimer's disease. Sci. Rep. 10 (1), 9391. doi:10.1038/s41598-020-66177-5
Bećarević, M. (2016). The IgG and IgM isotypes of anti-annexin A5 antibodies: relevance for primary antiphospholipid syndrome. J. Thromb. Thrombolysis 42 (4), 552–557. doi:10.1007/s11239-016-1389-5
Boersma, H. H., Kietselaer, B. L., Stolk, L. M., Bennaghmouch, A., Hofstra, L., Narula, J., et al. (2005). Past, present, and future of annexin A5: from protein discovery to clinical applications. J. Nucl. Med. 46 (12), 2035–2050.
Bogdanova, N., Baleva, M., Kremensky, I., and Markoff, A. (2012). The annexin A5 protective shield model revisited: inherited carriage of the M2/ANXA5 haplotype in placenta as a predisposing factor for the development of obstetric antiphospholipid antibodies. Lupus. 21 (7), 796–798. doi:10.1177/0961203312438235
Bolean, M., Izzi, B., van Kerckhoven, S., Bottini, M., Ramos, A. P., Millán, J. L., et al. (2020). Matrix vesicle biomimetics harboring Annexin A5 and alkaline phosphatase bind to the native collagen matrix produced by mineralizing vascular smooth muscle cells. Biochim. Biophys. Acta Gen. Subj. 1864 (8), 129629. doi:10.1016/j.bbagen.2020.129629
Bolean, M., Simão, A. M. S., Barioni, M. B., Favarin, B. Z., Sebinelli, H. G., Veschi, E. A., et al. (2017). Biophysical aspects of biomineralization. Biophys. Rev. 9 (5), 747–760. doi:10.1007/s12551-017-0315-1
Bondanza, A., Zimmermann, V. S., Rovere-Querini, P., Turnay, J., Dumitriu, I. E., Stach, C. M., et al. (2004). Inhibition of phosphatidylserine recognition heightens the immunogenicity of irradiated lymphoma cells in vivo. J. Exp. Med. 200 (9), 1157–1165. doi:10.1084/jem.20040327
Böttcher, A., Gaipl, U. S., Fürnrohr, B. G., Herrmann, M., Girkontaite, I., Kalden, J. R., et al. (2006). Involvement of phosphatidylserine, alphavbeta3, CD14, CD36, and complement C1q in the phagocytosis of primary necrotic lymphocytes by macrophages. Arthritis Rheum. 54 (3), 927–938. doi:10.1002/art.21660
Boyan, B. D., Asmussen, N. C., Lin, Z., and Schwartz, Z. (2022). The role of matrix-bound extracellular vesicles in the regulation of endochondral bone formation. Cells 11 (10), 1619. doi:10.3390/cells11101619
Brachvogel, B., Dikschas, J., Moch, H., Welzel, H., von der Mark, K., Hofmann, C., et al. (2003). Annexin A5 is not essential for skeletal development. Mol. Cell. Biol. 23 (8), 2907–2913. doi:10.1128/mcb.23.8.2907-2913.2003
Brachvogel, B., Moch, H., Pausch, F., Schlötzer-Schrehardt, U., Hofmann, C., Hallmann, R., et al. (2005). Perivascular cells expressing annexin A5 define a novel mesenchymal stem cell-like population with the capacity to differentiate into multiple mesenchymal lineages. Development 132 (11), 2657–2668. doi:10.1242/dev.01846
Brachvogel, B., Pausch, F., Farlie, P., Gaipl, U., Etich, J., Zhou, Z., et al. (2007). Isolated Anxa5+/Sca-1+ perivascular cells from mouse meningeal vasculature retain their perivascular phenotype in vitro and in vivo. Exp. Cell. Res. 313 (12), 2730–2743. doi:10.1016/j.yexcr.2007.04.031
Brachvogel, B., Welzel, H., Moch, H., von der Mark, K., Hofmann, C., and Pöschl, E. (2001). Sequential expression of annexin A5 in the vasculature and skeletal elements during mouse development. Mech. Dev. 109 (2), 389–393. doi:10.1016/s0925-4773(01)00532-9
Burgmaier, M., Schutters, K., Willems, B., van der Vorst, E. P., Kusters, D., Chatrou, M., et al. (2014). AnxA5 reduces plaque inflammation of advanced atherosclerotic lesions in apoE(-/-) mice. J. Cell. Mol. Med. 18 (10), 2117–2124. doi:10.1111/jcmm.12374
Castagnola, P., and Cancedda, R. (1991). Expression of anchorin CII mRNA by cultured chondrocytes. Cytotechnology 5 (Suppl. 1), 41–44. doi:10.1007/bf00736804
Cederholm, A., and Frostegård, J. (2005). Annexin A5 in cardiovascular disease and systemic lupus erythematosus. Immunobiology 210 (10), 761–768. doi:10.1016/j.imbio.2005.10.007
Cederholm, A., and Frostegård, J. (2007). Annexin A5 multitasking: a potentially novel antiatherothrombotic agent? Drug News Perspect. 20 (5), 321–326. doi:10.1358/dnp.2007.20.5.1120220
Chaudhary, S. C., Kuzynski, M., Bottini, M., Beniash, E., Dokland, T., Mobley, C. G., et al. (2016). Phosphate induces formation of matrix vesicles during odontoblast-initiated mineralization in vitro. Matrix Biol. 52-54, 284–300. doi:10.1016/j.matbio.2016.02.003
Chaurio, R. A., Janko, C., Muñoz, L. E., Frey, B., Herrmann, M., and Gaipl, U. S. (2009). Phospholipids: key players in apoptosis and immune regulation. Molecules 14 (12), 4892–4914. doi:10.3390/molecules14124892
Connor, D. E., Exner, T., Ma, D. D., and Joseph, J. E. (2010). The majority of circulating platelet-derived microparticles fail to bind annexin V, lack phospholipid-dependent procoagulant activity and demonstrate greater expression of glycoprotein Ib. Thromb. Haemost. 103 (5), 1044–1052. doi:10.1160/th09-09-0644
Ewing, M. M., de Vries, M. R., Nordzell, M., Pettersson, K., de Boer, H. C., van Zonneveld, A. J., et al. (2011). Annexin A5 therapy attenuates vascular inflammation and remodeling and improves endothelial function in mice. Arterioscler. Thromb. Vasc. Biol. 31 (1), 95–101. doi:10.1161/atvbaha.110.216747
Ewing, M. M., Karper, J. C., Sampietro, M. L., de Vries, M. R., Pettersson, K., Jukema, J. W., et al. (2012). Annexin A5 prevents post-interventional accelerated atherosclerosis development in a dose-dependent fashion in mice. Atherosclerosis 221 (2), 333–340. doi:10.1016/j.atherosclerosis.2012.01.037
Frey, B., Schildkopf, P., Rödel, F., Weiss, E. M., Munoz, L. E., Herrmann, M., et al. (2009). AnnexinA5 renders dead tumor cells immunogenic--implications for multimodal cancer therapies. J. Immunotoxicol. 6 (4), 209–216. doi:10.3109/15476910903204058
Gao, Y., Zhang, H., Wang, J., Li, F., Li, X., Li, T., et al. (2023). Annexin A5 ameliorates traumatic brain injury-induced neuroinflammation and neuronal ferroptosis by modulating the NF-ĸB/HMGB1 and Nrf2/HO-1 pathways. Int. Immunopharmacol. 114, 109619. doi:10.1016/j.intimp.2022.109619
Genetos, D. C., Wong, A., Weber, T. J., Karin, N. J., and Yellowley, C. E. (2014). Impaired osteoblast differentiation in annexin A2-and -A5-deficient cells. PLoS One 9 (9), e107482. doi:10.1371/journal.pone.0107482
Genge, B. R., Cao, X., Wu, L. N., Buzzi, W. R., Showman, R. W., Arsenault, A. L., et al. (1992). Establishment of the primary structure of the major lipid-dependent Ca2+ binding proteins of chicken growth plate cartilage matrix vesicles: identity with anchorin CII (annexin V) and annexin II. J. Bone Min. Res. 7 (7), 807–819. doi:10.1002/jbmr.5650070710
Genge, B. R., Wu, L. N., and Wuthier, R. E. (2007). In vitro modeling of matrix vesicle nucleation: synergistic stimulation of mineral formation by annexin A5 and phosphatidylserine. J. Biol. Chem. 282 (36), 26035–26045. doi:10.1074/jbc.M701057200
Genge, B. R., Wu, L. N. Y., and Wuthier, R. E. (2008). Mineralization of annexin-5-containing lipid-calcium-phosphate complexes: modulation by varying lipid composition and incubation with cartilage collagens. J. Biol. Chem. 283 (15), 9737–9748. doi:10.1074/jbc.M706523200
Gerke, V., Creutz, C. E., and Moss, S. E. (2005). Annexins: linking Ca2+ signalling to membrane dynamics. Nat. Rev. Mol. Cell. Biol. 6 (6), 449–461. doi:10.1038/nrm1661
Gerke, V., and Moss, S. E. (2002). Annexins: from structure to function. Physiol. Rev. 82 (2), 331–371. doi:10.1152/physrev.00030.2001
Giambanco, I., Pula, G., Ceccarelli, P., Bianchi, R., and Donato, R. (1991). Immunohistochemical localization of annexin V (CaBP33) in rat organs. J. Histochem Cytochem 39 (9), 1189–1198. doi:10.1177/39.9.1833446
Gotow, T., Sakata, M., Funakoshi, T., and Uchiyama, Y. (1996). Preferential localization of annexin V to the axon terminal. Neuroscience 75 (2), 507–521. doi:10.1016/0306-4522(96)00295-3
Gray, M. J., Gong, J., Hatch, M. M., Nguyen, V., Hughes, C. C., Hutchins, J. T., et al. (2016). Phosphatidylserine-targeting antibodies augment the anti-tumorigenic activity of anti-PD-1 therapy by enhancing immune activation and downregulating pro-oncogenic factors induced by T-cell checkpoint inhibition in murine triple-negative breast cancers. Breast Cancer Res. 18 (1), 50. doi:10.1186/s13058-016-0708-2
Habeeb, R. A., Mansour, H. E., Abdeldayem, A. M., Abo-Shady, R. A., Hassan, I. A., Saafan, N. K., et al. (2010). Anti-annexin v antibodies: association with vascular involvement and disease outcome in patients with systemic sclerosis. Clin. Med. Insights Arthritis Musculoskelet. Disord. 3, 15–23. doi:10.4137/cmamd.s4503
Huang, X., Ding, W. Q., Vaught, J. L., Wolf, R. F., Morrissey, J. H., Harrison, R. G., et al. (2006). A soluble tissue factor-annexin V chimeric protein has both procoagulant and anticoagulant properties. Blood 107 (3), 980–986. doi:10.1182/blood-2005-07-2733
Inaba, N., Sato, N., Ijichi, M., Fukazawa, I., Nito, A., Takamizawa, H., et al. (1984). The immunocytochemical location of two membrane-associated placental tissue proteins in human and cynomolgus monkey placentae. Tumour Biol. 5 (2), 75–85.
Jia, Z., Kang, B., Dong, Y., Fan, M., Li, W., and Zhang, W. (2024). Annexin A5 derived from cell-free fat extract attenuates osteoarthritis via macrophage regulation. Int. J. Biol. Sci. 20 (8), 2994–3007. doi:10.7150/ijbs.92802
Jing, J. (2024). The relevance, predictability, and utility of annexin A5 for human physiopathology. Int. J. Mol. Sci. 25 (5), 2865. doi:10.3390/ijms25052865
Jing, J., Chen, L., Fu, H. Y., Fan, K., Yao, Q., Ge, Y. F., et al. (2015). Annexin V-induced rat Leydig cell proliferation involves Ect2 via RhoA/ROCK signaling pathway. Sci. Rep. 5, 9437. doi:10.1038/srep09437
Ju, C. W., Wang, L. S., Yang, X., Ma, G. S., Hua, Z. C., and Gao, X. Y. (2004). A study of human annexin V derivative: its effects of anticoagulation and antithrombosis. Zhonghua Xue Ye Xue Za Zhi 25 (9), 540–543.
Kang, T. H., Park, J. H., Yang, A., Park, H. J., Lee, S. E., Kim, Y. S., et al. (2020). Annexin A5 as an immune checkpoint inhibitor and tumor-homing molecule for cancer treatment. Nat. Commun. 11 (1), 1137. doi:10.1038/s41467-020-14821-z
Kim, H. J., and Kirsch, T. (2008). Collagen/annexin V interactions regulate chondrocyte mineralization. J. Biol. Chem. 283 (16), 10310–10317. doi:10.1074/jbc.M708456200
King, K. B., Chubinskaya, S., Reid, D. L., Madsen, L. H., and Mollenhauer, J. (1997). Absence of cell-surface annexin V is accompanied by defective collagen matrix binding in the Swarm rat chondrosarcoma. J. Cell. Biochem. 65 (2), 131–144. doi:10.1002/(sici)1097-4644(199705)65:2<131::aid-jcb1>3.0.co;2-v
Kirsch, T., Nah, H. D., Demuth, D. R., Harrison, G., Golub, E. E., Adams, S. L., et al. (1997). Annexin V-mediated calcium flux across membranes is dependent on the lipid composition: implications for cartilage mineralization. Biochemistry 36 (11), 3359–3367. doi:10.1021/bi9626867
Kirsch, T., and Pfäffle, M. (1992). Selective binding of anchorin CII (annexin V) to type II and X collagen and to chondrocalcin (C-propeptide of type II collagen). Implications for anchoring function between matrix vesicles and matrix proteins. FEBS Lett. 310 (2), 143–147. doi:10.1016/0014-5793(92)81316-e
Kirsch, T., Swoboda, B., and Nah, H. (2000). Activation of annexin II and V expression, terminal differentiation, mineralization and apoptosis in human osteoarthritic cartilage. Osteoarthr. Cartil. 8 (4), 294–302. doi:10.1053/joca.1999.0304
Köhler, G., Hering, U., Zschörnig, O., and Arnold, K. (1997). Annexin V interaction with phosphatidylserine-containing vesicles at low and neutral pH. Biochemistry 36 (26), 8189–8194. doi:10.1021/bi9703960
Krey, J. F., Drummond, M., Foster, S., Porsov, E., Vijayakumar, S., Choi, D., et al. (2016). Annexin A5 is the most abundant membrane-associated protein in stereocilia but is dispensable for hair-bundle development and function. Sci. Rep. 6, 27221. doi:10.1038/srep27221
Krysko, O., De Ridder, L., and Cornelissen, M. (2004). Phosphatidylserine exposure during early primary necrosis (oncosis) in JB6 cells as evidenced by immunogold labeling technique. Apoptosis 9 (4), 495–500. doi:10.1023/b:Appt.0000031452.75162.75
Kumar, R., Saneja, A., and Panda, A. K. (2021). An annexin V-FITC-Propidium iodide-based method for detecting apoptosis in a non-small cell lung cancer cell line. Methods Mol. Biol. 2279, 213–223. doi:10.1007/978-1-0716-1278-1_17
Kusumbe, A. P., Ramasamy, S. K., and Adams, R. H. (2014). Coupling of angiogenesis and osteogenesis by a specific vessel subtype in bone. Nature 507 (7492), 323–328. doi:10.1038/nature13145
Laufer, E. M., Reutelingsperger, C. P., Narula, J., and Hofstra, L. (2008). Annexin A5: an imaging biomarker of cardiovascular risk. Basic Res. Cardiol. 103 (2), 95–104. doi:10.1007/s00395-008-0701-8
Lee, G., Pollard, H. B., and Arispe, N. (2002). Annexin 5 and apolipoprotein E2 protect against Alzheimer's amyloid-beta-peptide cytotoxicity by competitive inhibition at a common phosphatidylserine interaction site. Peptides 23 (7), 1249–1263. doi:10.1016/s0196-9781(02)00060-8
Lee, S. H., Lee, P. H., Kim, B. G., Hong, J., and Jang, A. S. (2018). Annexin A5 protein as a potential biomarker for the diagnosis of asthma. Lung 196 (6), 681–689. doi:10.1007/s00408-018-0159-x
Leon, C., Nandan, D., Lopez, M., Moeenrezakhanlou, A., and Reiner, N. E. (2006). Annexin V associates with the IFN-gamma receptor and regulates IFN-gamma signaling. J. Immunol. 176 (10), 5934–5942. doi:10.4049/jimmunol.176.10.5934
Li, J., Chen, T., Wang, D. M., Song, Y. F., and Hong, M. (2009). Annexin A5 inhibits homocysteine-induced tissue factor expression and activity in vascular smooth muscle cells. Zhonghua Xin Xue Guan Bing Za Zhi 37 (11), 1039–1043.
Liu, X., Huang, R., Wan, J., and Niu, T. (2024). LncRNA MIR4697HG alleviates endothelial cell injury and atherosclerosis progression in mice via the FUS/ANXA5 Axis. Biochem. Genet. 62 (4), 3155–3173. doi:10.1007/s10528-023-10542-2
Lizarbe, M. A., Barrasa, J. I., Olmo, N., Gavilanes, F., and Turnay, J. (2013). Annexin-phospholipid interactions. Functional implications. Int. J. Mol. Sci. 14 (2), 2652–2683. doi:10.3390/ijms14022652
Lucic, D., Mollenhauer, J., Kilpatrick, K. E., and Cole, A. A. (2003). N-telopeptide of type II collagen interacts with annexin V on human chondrocytes. Connect. Tissue Res. 44 (5), 225–239. doi:10.1080/03008200390248632
Martin, C. M., Slessarev, M., Campbell, E., Basmaji, J., Ball, I., Fraser, D. D., et al. (2023). Annexin A5 in patients with severe COVID-19 disease: a single-center, randomized, double-blind, placebo-controlled feasibility trial. Crit. Care Explor 5 (10), e0986. doi:10.1097/cce.0000000000000986
Mebarek, S., Buchet, R., Pikula, S., Strzelecka-Kiliszek, A., Brizuela, L., Corti, G., et al. (2023). Do media extracellular vesicles and extracellular vesicles bound to the extracellular matrix represent distinct types of vesicles? Biomolecules 14 (1), 42. doi:10.3390/biom14010042
Millán, J. L. (2013). The role of phosphatases in the initiation of skeletal mineralization. Calcif. Tissue Int. 93 (4), 299–306. doi:10.1007/s00223-012-9672-8
Mineo, C., Shaul, P. W., and Bermas, B. L. (2023). The pathogenesis of obstetric APS: a 2023 update. Clin. Immunol. 255, 109745. doi:10.1016/j.clim.2023.109745
Miyagishima, K. J., Nadal-Nicolás, F. M., Ma, W., and Li, W. (2024). Annexin-V binds subpopulation of immune cells altering its interpretation as an in vivo biomarker for apoptosis in the retina. Int. J. Biol. Sci. 20 (15), 6073–6089. doi:10.7150/ijbs.102551
Mohiti, J., Caswell, A. M., and Walker, J. H. (1995). Calcium-induced relocation of annexins IV and V in the human osteosarcoma cell line MG-63. Mol. Membr. Biol. 12 (4), 321–329. doi:10.3109/09687689509072434
Mollenhauer, J., Bee, J. A., Lizarbe, M. A., and von der Mark, K. (1984). Role of anchorin CII, a 31,000-mol-wt membrane protein, in the interaction of chondrocytes with type II collagen. J. Cell. Biol. 98 (4), 1572–1579. doi:10.1083/jcb.98.4.1572
Mollenhauer, J., Mok, M. T., King, K. B., Gupta, M., Chubinskaya, S., Koepp, H., et al. (1999). Expression of anchorin CII (cartilage annexin V) in human young, normal adult, and osteoarthritic cartilage. J. Histochem Cytochem 47 (2), 209–220. doi:10.1177/002215549904700209
Moss, S. E., and Morgan, R. O. (2004). The annexins. Genome Biol. 5 (4), 219. doi:10.1186/gb-2004-5-4-219
Mui, L., Martin, C. M., Tschirhart, B. J., and Feng, Q. (2021). Therapeutic potential of annexins in sepsis and COVID-19. Front. Pharmacol. 12, 735472. doi:10.3389/fphar.2021.735472
Munoz, L. E., Frey, B., Pausch, F., Baum, W., Mueller, R. B., Brachvogel, B., et al. (2007). The role of annexin A5 in the modulation of the immune response against dying and dead cells. Curr. Med. Chem. 14 (3), 271–277. doi:10.2174/092986707779941131
Peng, B., Guo, C., Guan, H., Liu, S., and Sun, M. Z. (2014). Annexin A5 as a potential marker in tumors. Clin. Chim. Acta 427, 42–48. doi:10.1016/j.cca.2013.09.048
Peng, L., Yang, W., Deng, X., and Bao, S. (2022). Research progress on ANXA5 in recurrent pregnancy loss. J. Reprod. Immunol. 153, 103679. doi:10.1016/j.jri.2022.103679
Pfäffle, M., Ruggiero, F., Hofmann, H., Fernández, M. P., Selmin, O., Yamada, Y., et al. (1988). Biosynthesis, secretion and extracellular localization of anchorin CII, a collagen-binding protein of the calpactin family. Embo J. 7 (8), 2335–2342. doi:10.1002/j.1460-2075.1988.tb03077.x
Qi, H., Liu, S., Guo, C., Wang, J., Greenaway, F. T., and Sun, M. Z. (2015). Role of annexin A6 in cancer. Oncol. Lett. 10 (4), 1947–1952. doi:10.3892/ol.2015.3498
Quan, X., Han, Y., Lu, P., Ding, Y., Wang, Q., Li, Y., et al. (2022). Annexin V-modified platelet-biomimetic nanomedicine for targeted therapy of acute ischemic stroke. Adv. Healthc. Mater 11 (16), e2200416. doi:10.1002/adhm.202200416
Rahman, M. M., Iida, H., and Shibata, Y. (1997). Expression and localization of annexin V and annexin VI during limb bud formation in the rat fetus. Anat. Embryol. Berl. 195 (1), 31–39. doi:10.1007/s004290050022
Rand, J. H., Wu, X. X., Quinn, A. S., and Taatjes, D. J. (2010). The annexin A5-mediated pathogenic mechanism in the antiphospholipid syndrome: role in pregnancy losses and thrombosis. Lupus. 19 (4), 460–469. doi:10.1177/0961203310361485
Ravanat, C., Archipoff, G., Beretz, A., Freund, G., Cazenave, J. P., and Freyssinet, J. M. (1992). Use of annexin-V to demonstrate the role of phosphatidylserine exposure in the maintenance of haemostatic balance by endothelial cells. Biochem. J. 282 (Pt 1), 7–13. doi:10.1042/bj2820007
Reddy, E. C., and Rand, M. L. (2020). Procoagulant phosphatidylserine-exposing platelets in vitro and in vivo. Front. Cardiovasc Med. 7, 15. doi:10.3389/fcvm.2020.00015
Rescher, U., and Gerke, V. (2004). Annexins--unique membrane binding proteins with diverse functions. J. Cell. Sci. 117 (Pt 13), 2631–2639. doi:10.1242/jcs.01245
Rieger, A. M., Nelson, K. L., Konowalchuk, J. D., and Barreda, D. R. (2011). Modified annexin V/propidium iodide apoptosis assay for accurate assessment of cell death. J. Vis. Exp. (50), 2597. doi:10.3791/2597
Rintala-Dempsey, A. C., Rezvanpour, A., and Shaw, G. S. (2008). S100-annexin complexes--structural insights. Febs J. 275 (20), 4956–4966. doi:10.1111/j.1742-4658.2008.06654.x
Rogenhofer, N., Nienaber, L. R. M., Amshoff, L. C., Bogdanova, N., Petroff, D., Wieacker, P., et al. (2018). Assessment of M2/ANXA5 haplotype as a risk factor in couples with placenta-mediated pregnancy complications. J. Assist. Reprod. Genet. 35 (1), 157–163. doi:10.1007/s10815-017-1041-0
Salhotra, A., Shah, H. N., Levi, B., and Longaker, M. T. (2020). Mechanisms of bone development and repair. Nat. Rev. Mol. Cell. Biol. 21 (11), 696–711. doi:10.1038/s41580-020-00279-w
Sang, Y., Roest, M., de Laat, B., de Groot, P. G., and Huskens, D. (2021). Interplay between platelets and coagulation. Blood Rev. 46, 100733. doi:10.1016/j.blre.2020.100733
Schurgers, L. J., Burgmaier, M., Ueland, T., Schutters, K., Aakhus, S., Hofstra, L., et al. (2016). Circulating annexin A5 predicts mortality in patients with heart failure. J. Intern Med. 279 (1), 89–97. doi:10.1111/joim.12396
Schutters, K., and Reutelingsperger, C. (2010). Phosphatidylserine targeting for diagnosis and treatment of human diseases. Apoptosis 15 (9), 1072–1082. doi:10.1007/s10495-010-0503-y
Shimada, A., Ideno, H., Arai, Y., Komatsu, K., Wada, S., Yamashita, T., et al. (2018). Annexin A5 involvement in bone overgrowth at the enthesis. J. Bone Min. Res. 33 (8), 1532–1543. doi:10.1002/jbmr.3453
Stach, C. M., Turnay, X., Voll, R. E., Kern, P. M., Kolowos, W., Beyer, T. D., et al. (2000). Treatment with annexin V increases immunogenicity of apoptotic human T-cells in Balb/c mice. Cell. Death Differ. 7 (10), 911–915. doi:10.1038/sj.cdd.4400715
Su, G., Zhang, D., Li, T., Pei, T., Yang, J., Tu, S., et al. (2023). Annexin A5 derived from matrix vesicles protects against osteoporotic bone loss via mineralization. Bone Res. 11 (1), 60. doi:10.1038/s41413-023-00290-9
Suarez, F., Rothhut, B., Comera, C., Touqui, L., Marie, F. R., and Silve, C. (1993). Expression of annexin I, II, V, and VI by rat osteoblasts in primary culture: stimulation of annexin I expression by dexamethasone. J. Bone Min. Res. 8 (10), 1201–1210. doi:10.1002/jbmr.5650081007
Sugiura, K., and Muro, Y. (1999). Anti-annexin V antibodies and digital ischemia in patients with scleroderma. J. Rheumatol. 26 (10), 2168–2172.
Sun, C. B., Zhao, A. Y., Ji, S., Han, X. Q., Sun, Z. C., Wang, M. C., et al. (2017). Expression of annexin A5 in serum and tumor tissue of patients with colon cancer and its clinical significance. World J. Gastroenterol. 23 (39), 7168–7173. doi:10.3748/wjg.v23.i39.7168
Sun, X., Liu, S., Wang, J., Wei, B., Guo, C., Chen, C., et al. (2018). Annexin A5 regulates hepatocarcinoma malignancy via CRKI/II-DOCK180-RAC1 integrin and MEK-ERK pathways. Cell. Death Dis. 9 (6), 637. doi:10.1038/s41419-018-0685-8
Sun, X., Wei, B., Liu, S., Guo, C., Wu, N., Liu, Q., et al. (2016). Anxa5 mediates the in vitro malignant behaviours of murine hepatocarcinoma Hca-F cells with high lymph node metastasis potential preferentially via ERK2/p-ERK2/c-Jun/p-c-Jun(Ser73) and E-cadherin. Biomed. Pharmacother. 84, 645–654. doi:10.1016/j.biopha.2016.09.086
Tait, J. F., Frankenberry, D. A., Shiang, R., Murray, J. C., Adler, D. A., and Disteche, C. M. (1991). Chromosomal localization of the human gene for annexin V (placental anticoagulant protein I) to 4q26----q28. Cytogenet Cell. Genet. 57 (4), 187–192. doi:10.1159/000133143
Takei, N., Ohsawa, K., Imai, Y., Nakao, H., Iwasaki, A., and Kohsaka, S. (1994). Neurotrophic effects of annexin V on cultured neurons from embryonic rat brain. Neurosci. Lett. 171 (1-2), 59–62. doi:10.1016/0304-3940(94)90604-1
Tang, M., Zhang, L., Zhu, Z., Li, R., Wang, S., Wang, W., et al. (2020). Overexpression of miR-506-3p aggravates DBP-induced testicular oxidative stress in rats by downregulating ANXA5 via Nrf2/HO-1 signaling pathway. Oxid. Med. Cell. Longev. 2020, 4640605. doi:10.1155/2020/4640605
Thiagarajan, P., and Benedict, C. R. (1997). Inhibition of arterial thrombosis by recombinant annexin V in a rabbit carotid artery injury model. Circulation 96 (7), 2339–2347. doi:10.1161/01.cir.96.7.2339
Thiagarajan, P., and Tait, J. F. (1990). Binding of annexin V/placental anticoagulant protein I to platelets. Evidence for phosphatidylserine exposure in the procoagulant response of activated platelets. J. Biol. Chem. 265 (29), 17420–17423. doi:10.1016/s0021-9258(18)38177-8
Thiagarajan, P., and Tait, J. F. (1991). Collagen-induced exposure of anionic phospholipid in platelets and platelet-derived microparticles. J. Biol. Chem. 266 (36), 24302–24307. doi:10.1016/s0021-9258(18)54228-9
Tripathy, N. K., Sinha, N., and Nityanand, S. (2003). Anti-annexin V antibodies in Takayasu's arteritis: prevalence and relationship with disease activity. Clin. Exp. Immunol. 134 (2), 360–364. doi:10.1046/j.1365-2249.2003.02282.x
Tschirhart, B. J., Lu, X., Gomes, J., Chandrabalan, A., Bell, G., Hess, D. A., et al. (2023). Annexin A5 inhibits endothelial inflammation induced by lipopolysaccharide-activated platelets and microvesicles via phosphatidylserine binding. Pharm. (Basel). 16 (6), 837. doi:10.3390/ph16060837
van Heerde, W. L., Poort, S., van 't Veer, C., Reutelingsperger, C. P., and de Groot, P. G. (1994). Binding of recombinant annexin V to endothelial cells: effect of annexin V binding on endothelial-cell-mediated thrombin formation. Biochem. J. 302 (Pt 1), 305–312. doi:10.1042/bj3020305
Van Ryn-McKenna, J., Merk, H., Müller, T. H., Buchanan, M. R., and Eisert, W. G. (1993). The effects of heparin and annexin V on fibrin accretion after injury in the jugular veins of rabbits. Thromb. Haemost. 69 (3), 227–230. doi:10.1055/s-0038-1651585
von der Mark, K., and Mollenhauer, J. (1997). Annexin V interactions with collagen. Cell. Mol. Life Sci. 53 (6), 539–545. doi:10.1007/s000180050069
Wang, J., Zhang, Y., Liu, X., Ma, J., Liu, P., Hu, C., et al. (2014). Annexin A5 inhibits diffuse large B-cell lymphoma cell invasion and chemoresistance through phosphatidylinositol 3-kinase signaling. Oncol. Rep. 32 (6), 2557–2563. doi:10.3892/or.2014.3547
Wang, W., and Kirsch, T. (2006). Annexin V/beta5 integrin interactions regulate apoptosis of growth plate chondrocytes. J. Biol. Chem. 281 (41), 30848–30856. doi:10.1074/jbc.M605937200
Wang, W., Xu, J., and Kirsch, T. (2005). Annexin V and terminal differentiation of growth plate chondrocytes. Exp. Cell. Res. 305 (1), 156–165. doi:10.1016/j.yexcr.2004.12.022
Wang, X., Dai, Y., Zhang, J., and Li, X. (2023a). Annexin A5 suppression promotes the progression of cervical cancer. Arch. Gynecol. Obstet. 307 (3), 937–943. doi:10.1007/s00404-022-06524-1
Wang, X., Wu, Q., Zhang, R., Fan, Z., Li, W., Mao, R., et al. (2023b). Stage-specific and location-specific cartilage calcification in osteoarthritis development. Ann. Rheum. Dis. 82 (3), 393–402. doi:10.1136/ard-2022-222944
Wautier, M. P., Héron, E., Picot, J., Colin, Y., Hermine, O., and Wautier, J. L. (2011). Red blood cell phosphatidylserine exposure is responsible for increased erythrocyte adhesion to endothelium in central retinal vein occlusion. J. Thromb. Haemost. 9 (5), 1049–1055. doi:10.1111/j.1538-7836.2011.04251.x
Woodward, A., Faria, G. N. F., and Harrison, R. G. (2022). Annexin A5 as a targeting agent for cancer treatment. Cancer Lett. 547, 215857. doi:10.1016/j.canlet.2022.215857
Wuthier, R. E., and Lipscomb, G. F. (2011). Matrix vesicles: structure, composition, formation and function in calcification. Front. Biosci. Landmark Ed. 16 (8), 2812–2902. doi:10.2741/3887
Xi, Y., Ju, R., and Wang, Y. (2020). Roles of Annexin A protein family in autophagy regulation and therapy. Biomed. Pharmacother. 130, 110591. doi:10.1016/j.biopha.2020.110591
Yamaguchi, M., Kokai, Y., Imai, S., Utsumi, K., Matsumoto, K., Honda, H., et al. (2010). Investigation of annexin A5 as a biomarker for Alzheimer's disease using neuronal cell culture and mouse model. J. Neurosci. Res. 88 (12), 2682–2692. doi:10.1002/jnr.22427
Yan, X., Doffek, K., Yin, C., Krein, M., Phillips, M., Sugg, S. L., et al. (2012). Annexin-V promotes anti-tumor immunity and inhibits neuroblastoma growth in vivo. Cancer Immunol. Immunother. 61 (11), 1917–1927. doi:10.1007/s00262-012-1250-4
Zhang, H., Gao, Y., Li, T., Li, F., Peng, R., Wang, C., et al. (2022). Recombinant human annexin A5 alleviated traumatic-brain-injury induced intestinal injury by regulating the Nrf2/HO-1/HMGB1 pathway. Molecules 27 (18), 5755. doi:10.3390/molecules27185755
Zhang, L., Qin, Z., Li, R., Wang, S., Wang, W., Tang, M., et al. (2019). The role of ANXA5 in DBP-induced oxidative stress through ERK/Nrf2 pathway. Environ. Toxicol. Pharmacol. 72, 103236. doi:10.1016/j.etap.2019.103236
Zhao, L., Bi, Y., Kou, J., Shi, J., and Piao, D. (2016). Phosphatidylserine exposing-platelets and microparticles promote procoagulant activity in colon cancer patients. J. Exp. Clin. Cancer Res. 35, 54. doi:10.1186/s13046-016-0328-9
Keywords: ANXA5, phosphatidylserine, bone regeneration, biological functions, signaling pathways
Citation: Jin M, Zhang J, Sun Y, Liu G and Wei X (2025) ANXA5: related mechanisms of osteogenesis and additional biological functions. Front. Cell Dev. Biol. 13:1553683. doi: 10.3389/fcell.2025.1553683
Received: 31 December 2024; Accepted: 31 March 2025;
Published: 24 April 2025.
Edited by:
Lynda Bourebaba, Wroclaw University of Environmental and Life Sciences, PolandReviewed by:
Magdalena Zyzak, Wroclaw University of Environmental and Life Sciences, PolandHongchi Yu, Sichuan University, China
Copyright © 2025 Jin, Zhang, Sun, Liu and Wei. This is an open-access article distributed under the terms of the Creative Commons Attribution License (CC BY). The use, distribution or reproduction in other forums is permitted, provided the original author(s) and the copyright owner(s) are credited and that the original publication in this journal is cited, in accordance with accepted academic practice. No use, distribution or reproduction is permitted which does not comply with these terms.
*Correspondence: Xiaowei Wei, YW5nZWx3ZWkyMDAzQDE2My5jb20=
†These authors have contributed equally to this work